Abstract
We have determined the relationship between microtubule length and translocation velocity from recordings of bovine brain microtubules translocating over a Paramecium 22S dynein substratum in an in vitro assay chamber. For comparison with untreated samples, the 22S dynein has been subjected to detergent and/or to pretreatments that induce phosphorylation of an associated 29 kDa light chain. Control and treated dyneins have been used at the same densities in the translocation assays. In any given condition, translocation velocity (v) shows an initial increase with microtubule length (L) and then reaches a plateau. This situation may be represented by a hyperbola of the general form v = aL/(L+b), which is formally analogous to the Briggs-Haldane relationship, which we have used to interpret our data. The results indicate that the maximum translocation velocity Vo(= a) is increased by pretreatment, whereas the length constant KL(= b), which corresponds to Km, does not change with pretreatment, implying that the mechanochemical properties of the pretreated dyneins differ from those of control dyneins. The conclusion that KL is constant for defined in vitro assays rules out the possibility that the velocity changes seen are caused by changes in geometry in the translocation assays or by the numbers of dyneins or dynein heads needed to produce maximal translocational velocity. From our analysis, we determine that f, the fraction of cycle time during which the dynein is in the force-generating state, is small--roughly 0.01, comparable to the f determined previously for heavy meromyosin. The practical limits of these mechanochemical changes imply that the maximum possible ciliary beat frequency is about 120 Hz, and that in the physiological range of 5-60 Hz, beat frequency could be controlled by varying the numbers of phosphorylated outer arm dyneins along an axonemal microtubule.
Full text
PDF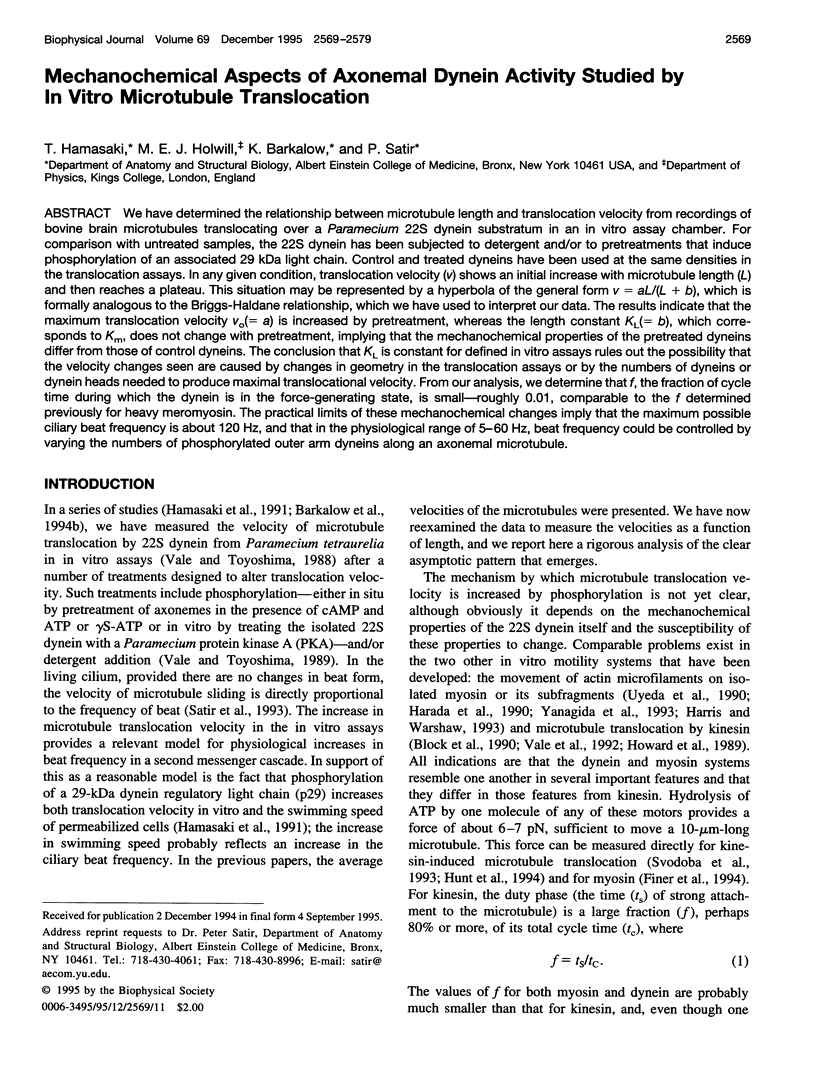
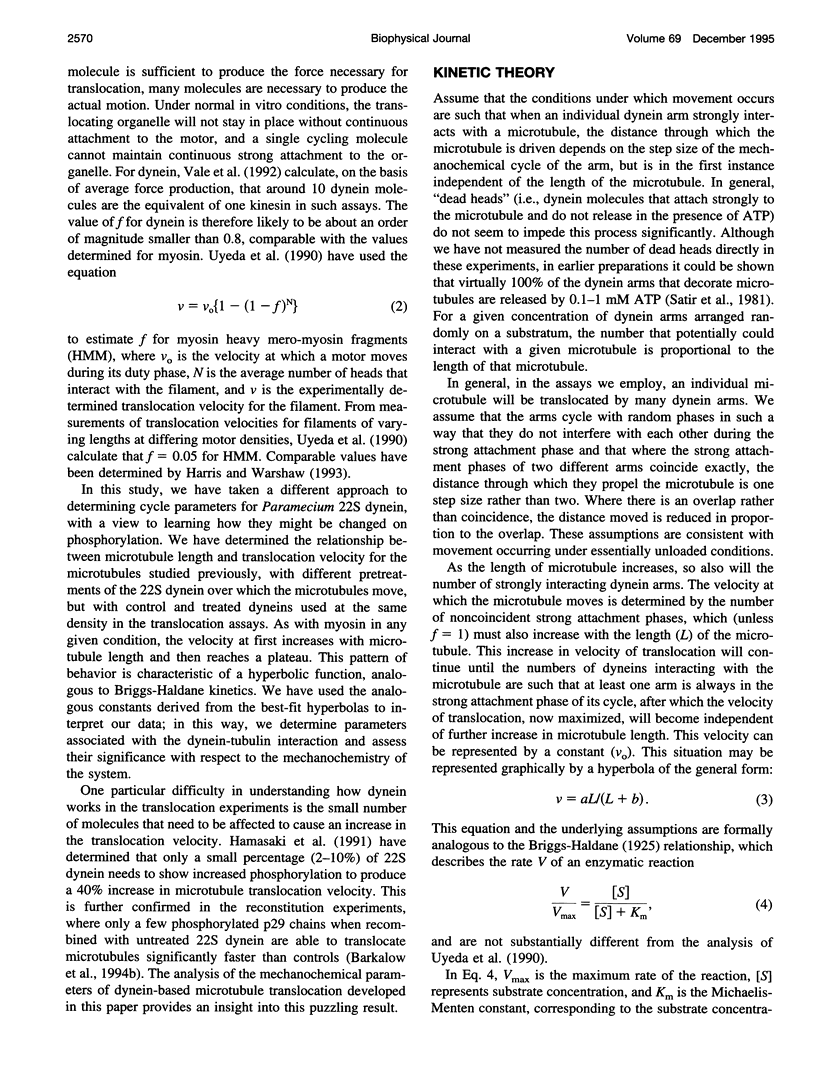
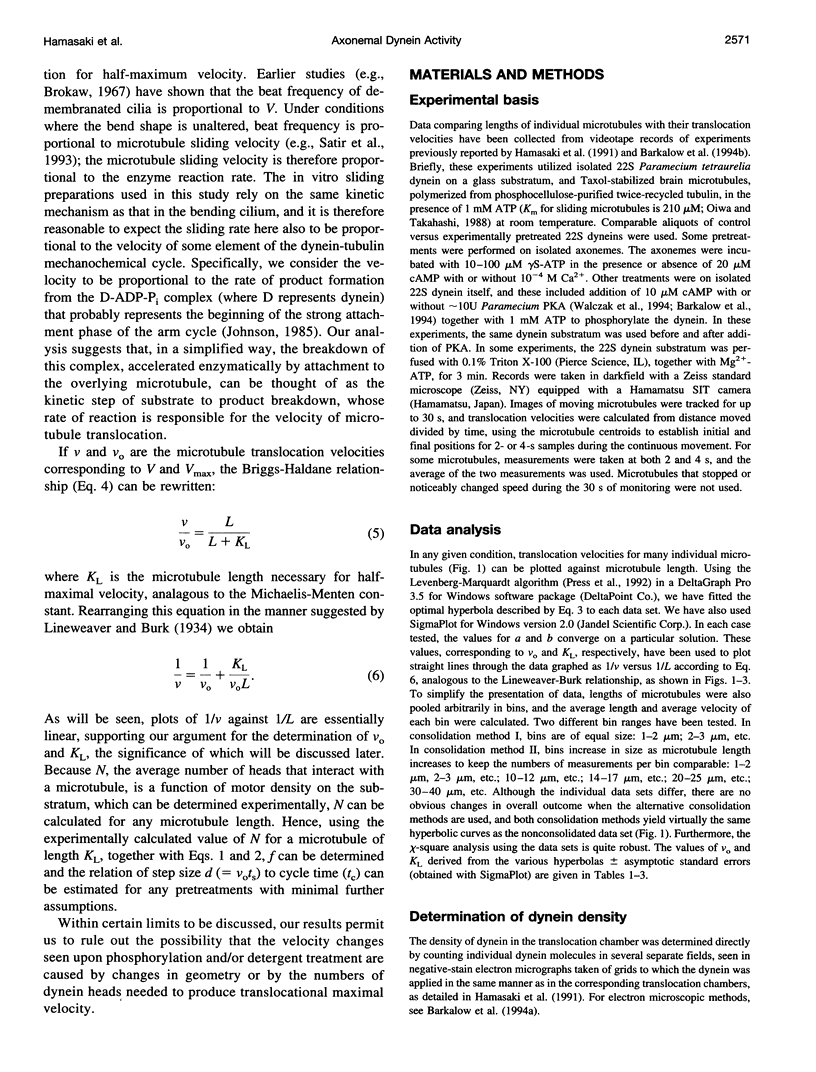
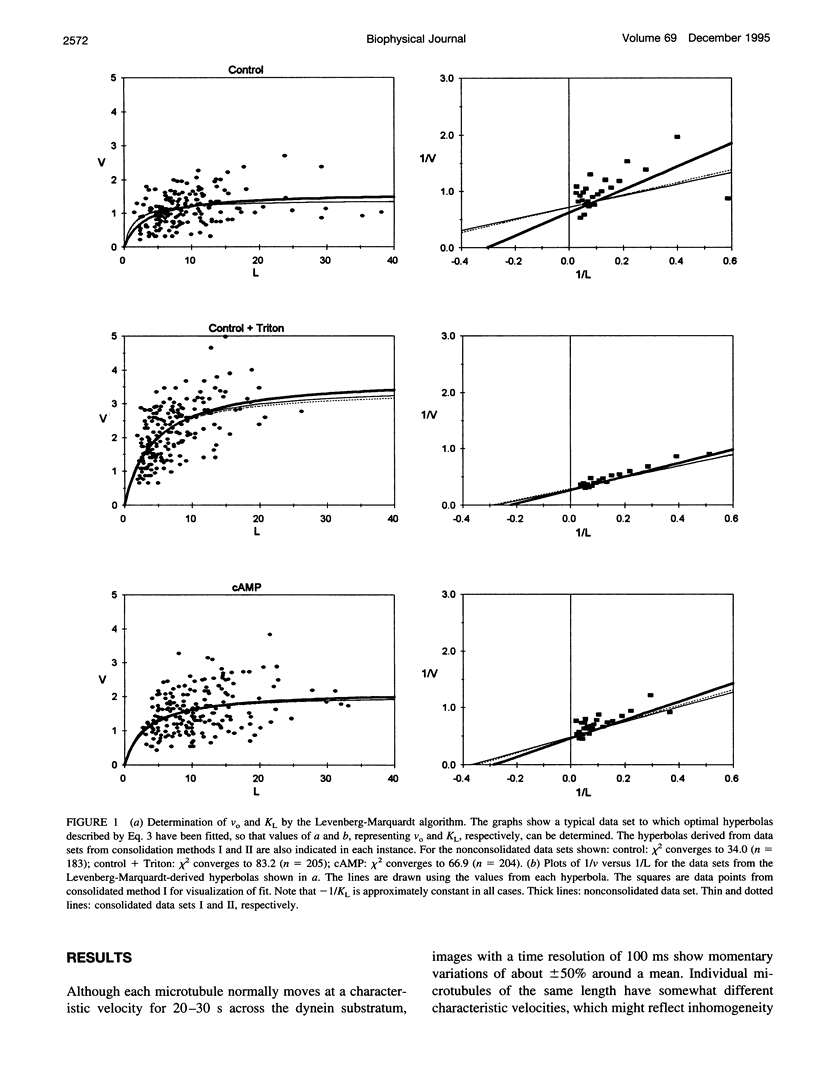
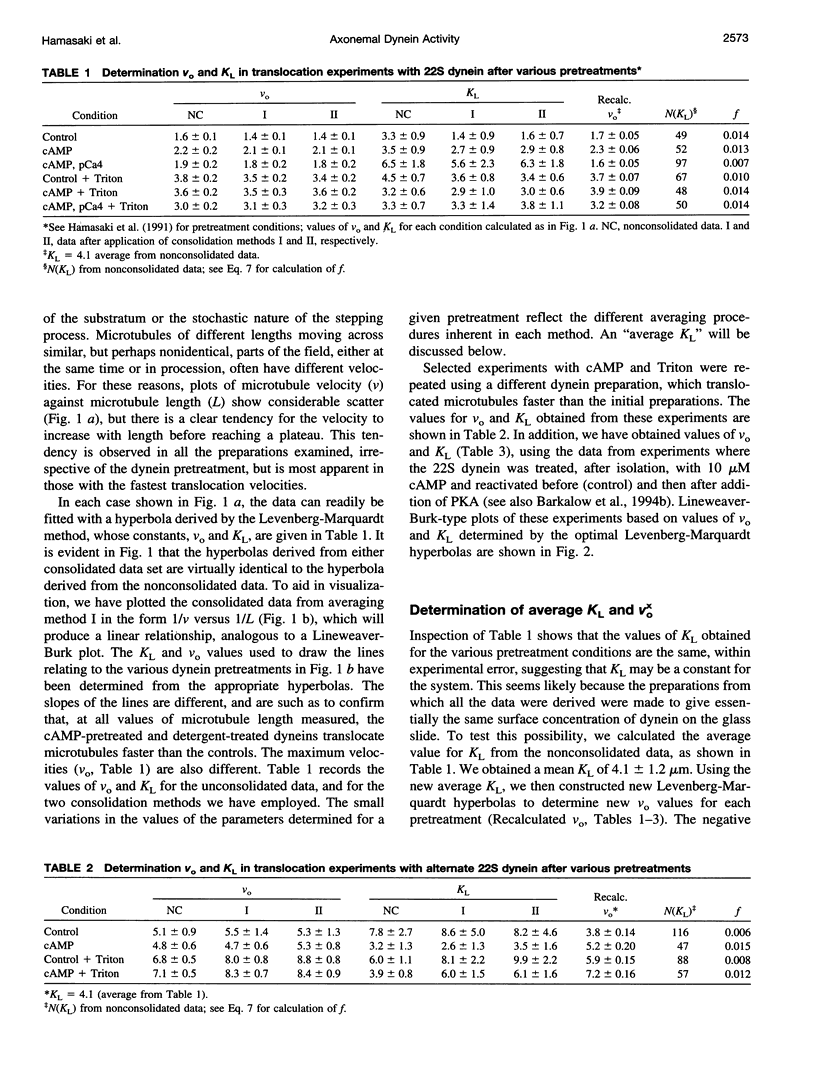
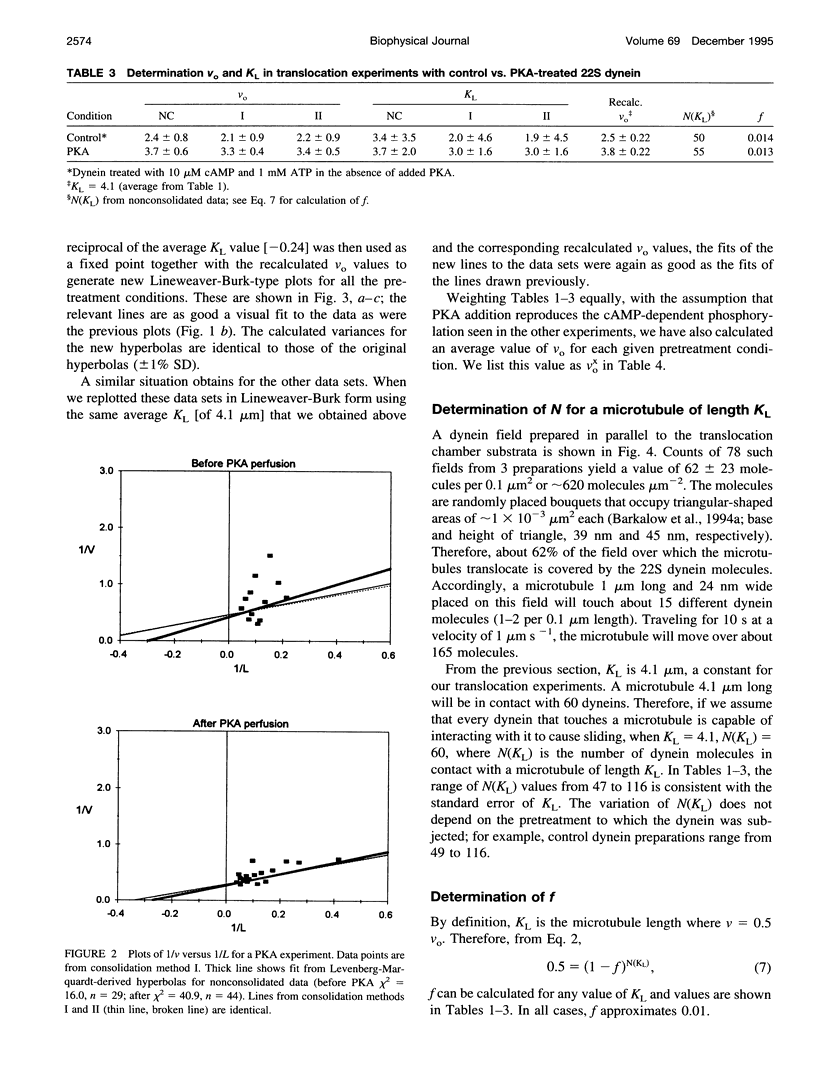
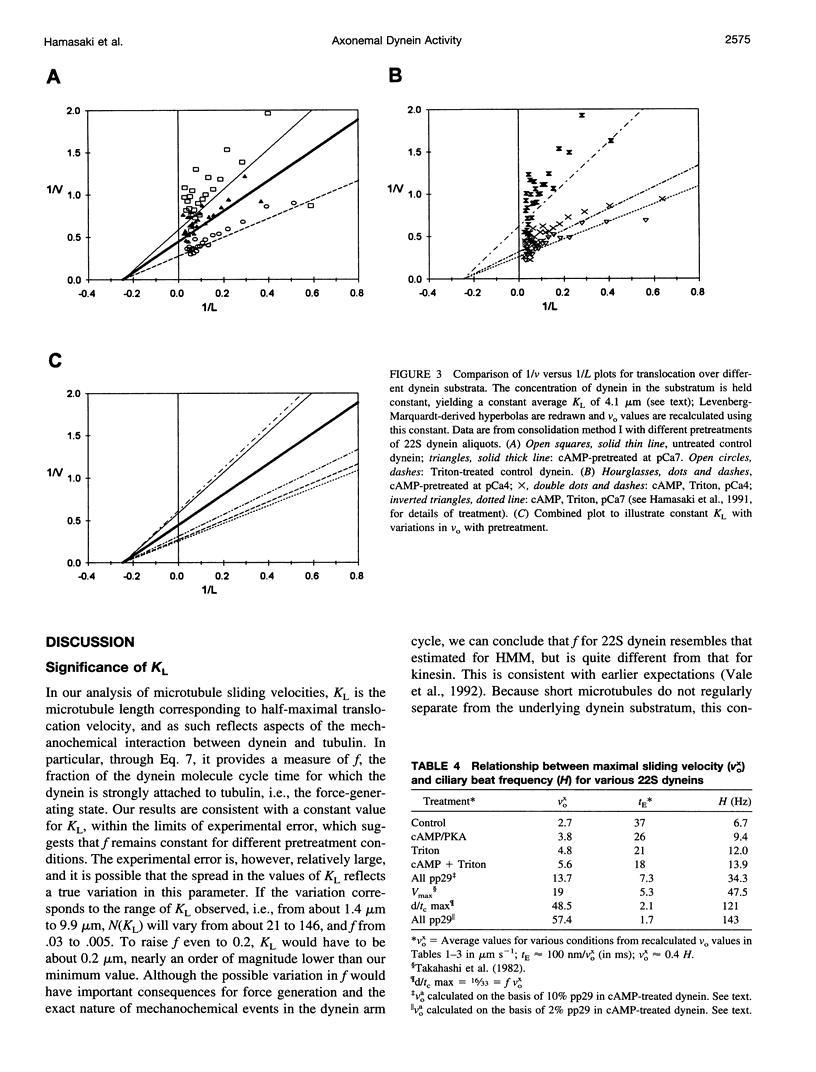
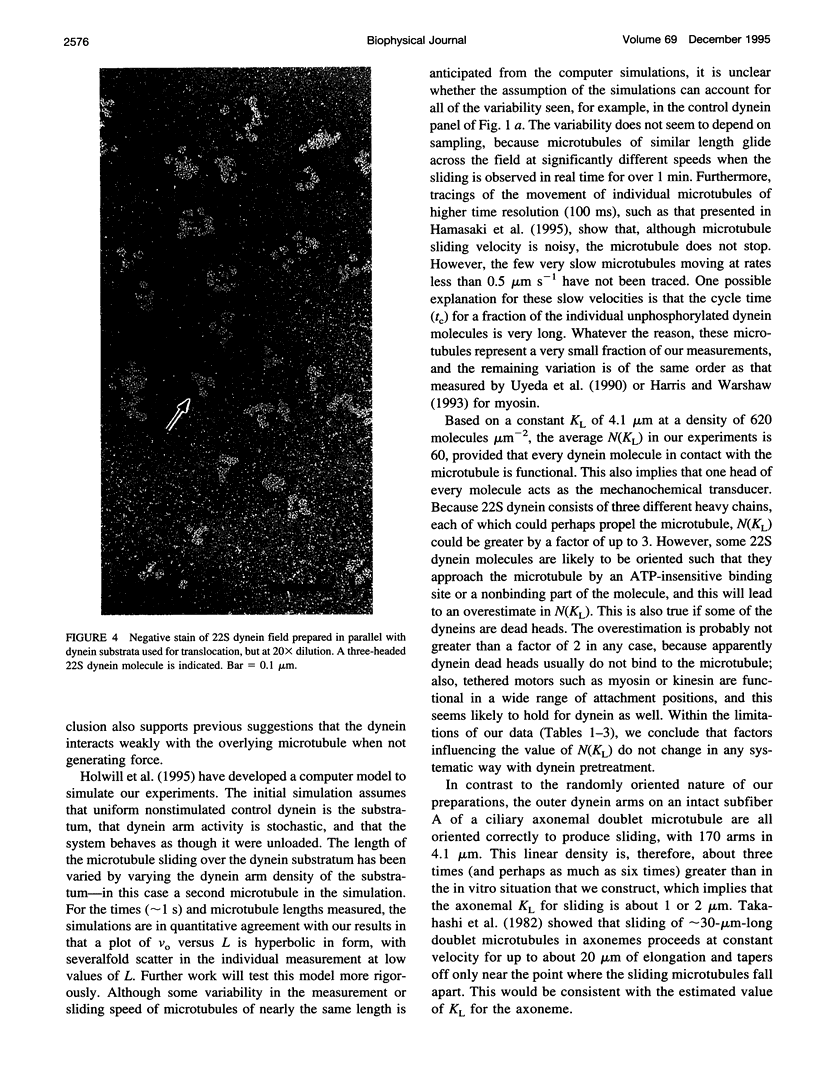
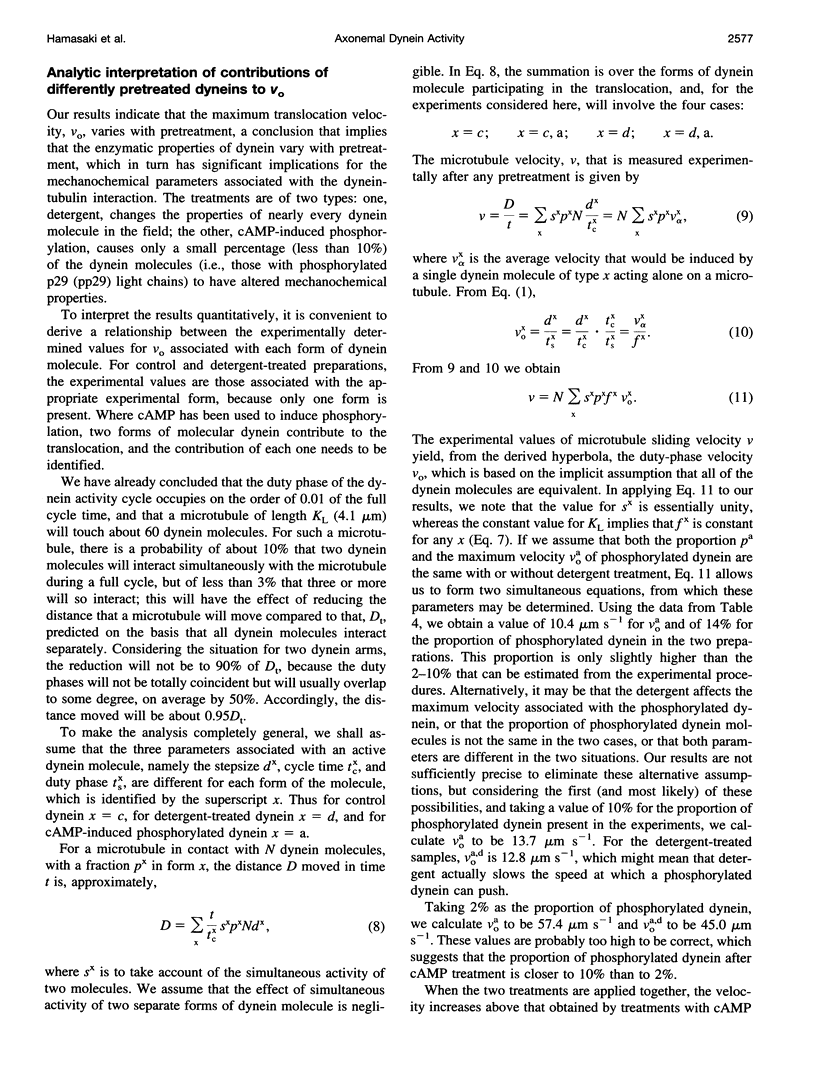
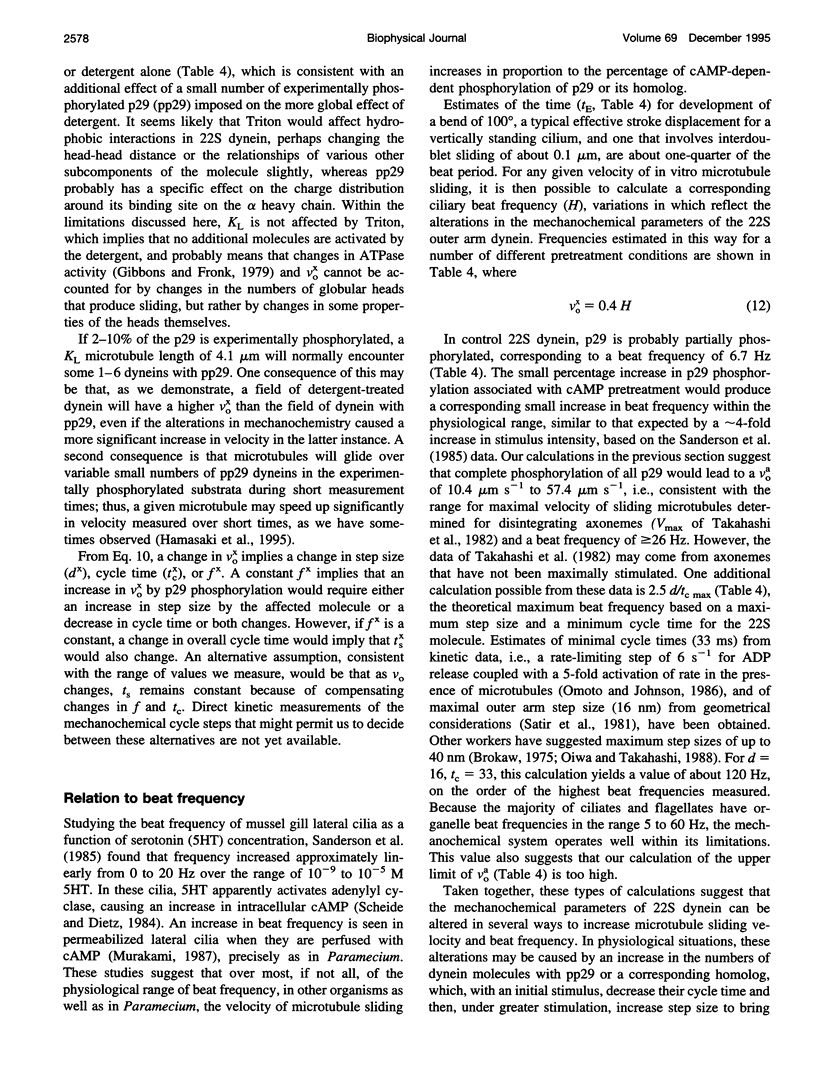
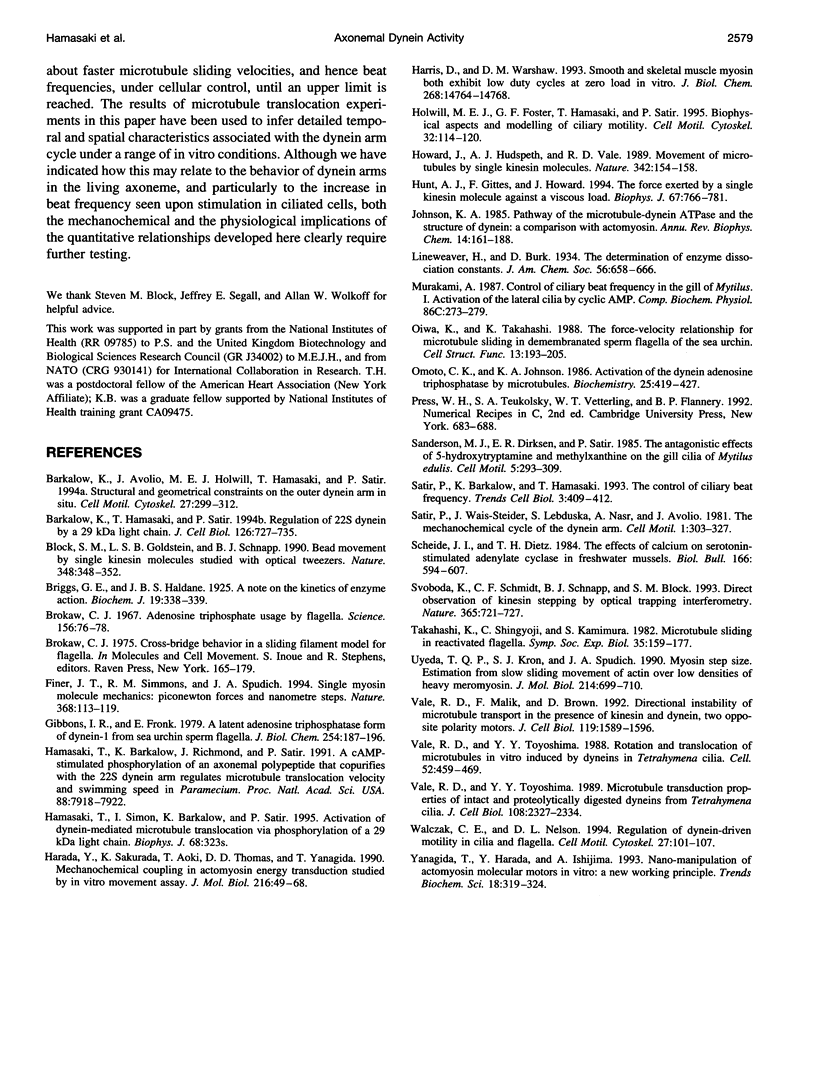
Images in this article
Selected References
These references are in PubMed. This may not be the complete list of references from this article.
- Barkalow K., Avolio J., Holwill M. E., Hamasaki T., Satir P. Structural and geometrical constraints on the outer dynein arm in situ. Cell Motil Cytoskeleton. 1994;27(4):299–312. doi: 10.1002/cm.970270403. [DOI] [PubMed] [Google Scholar]
- Barkalow K., Hamasaki T., Satir P. Regulation of 22S dynein by a 29-kD light chain. J Cell Biol. 1994 Aug;126(3):727–735. doi: 10.1083/jcb.126.3.727. [DOI] [PMC free article] [PubMed] [Google Scholar]
- Block S. M., Goldstein L. S., Schnapp B. J. Bead movement by single kinesin molecules studied with optical tweezers. Nature. 1990 Nov 22;348(6299):348–352. doi: 10.1038/348348a0. [DOI] [PubMed] [Google Scholar]
- Briggs G. E., Haldane J. B. A Note on the Kinetics of Enzyme Action. Biochem J. 1925;19(2):338–339. doi: 10.1042/bj0190338. [DOI] [PMC free article] [PubMed] [Google Scholar]
- Brokaw C. J. Adenosine triphosphate usage by flagella. Science. 1967 Apr 7;156(3771):76–78. doi: 10.1126/science.156.3771.76. [DOI] [PubMed] [Google Scholar]
- Finer J. T., Simmons R. M., Spudich J. A. Single myosin molecule mechanics: piconewton forces and nanometre steps. Nature. 1994 Mar 10;368(6467):113–119. doi: 10.1038/368113a0. [DOI] [PubMed] [Google Scholar]
- Gibbons I. R., Fronk E. A latent adenosine triphosphatase form of dynein 1 from sea urchin sperm flagella. J Biol Chem. 1979 Jan 10;254(1):187–196. [PubMed] [Google Scholar]
- Hamasaki T., Barkalow K., Richmond J., Satir P. cAMP-stimulated phosphorylation of an axonemal polypeptide that copurifies with the 22S dynein arm regulates microtubule translocation velocity and swimming speed in Paramecium. Proc Natl Acad Sci U S A. 1991 Sep 15;88(18):7918–7922. doi: 10.1073/pnas.88.18.7918. [DOI] [PMC free article] [PubMed] [Google Scholar]
- Harada Y., Sakurada K., Aoki T., Thomas D. D., Yanagida T. Mechanochemical coupling in actomyosin energy transduction studied by in vitro movement assay. J Mol Biol. 1990 Nov 5;216(1):49–68. doi: 10.1016/S0022-2836(05)80060-9. [DOI] [PubMed] [Google Scholar]
- Harris D. E., Warshaw D. M. Smooth and skeletal muscle myosin both exhibit low duty cycles at zero load in vitro. J Biol Chem. 1993 Jul 15;268(20):14764–14768. [PubMed] [Google Scholar]
- Holwill M. E., Foster G. F., Hamasaki T., Satir P. Biophysical aspects and modelling of ciliary motility. Cell Motil Cytoskeleton. 1995;32(2):114–120. doi: 10.1002/cm.970320209. [DOI] [PubMed] [Google Scholar]
- Howard J., Hudspeth A. J., Vale R. D. Movement of microtubules by single kinesin molecules. Nature. 1989 Nov 9;342(6246):154–158. doi: 10.1038/342154a0. [DOI] [PubMed] [Google Scholar]
- Hunt A. J., Gittes F., Howard J. The force exerted by a single kinesin molecule against a viscous load. Biophys J. 1994 Aug;67(2):766–781. doi: 10.1016/S0006-3495(94)80537-5. [DOI] [PMC free article] [PubMed] [Google Scholar]
- Johnson K. A. Pathway of the microtubule-dynein ATPase and the structure of dynein: a comparison with actomyosin. Annu Rev Biophys Biophys Chem. 1985;14:161–188. doi: 10.1146/annurev.bb.14.060185.001113. [DOI] [PubMed] [Google Scholar]
- Murakami A. Control of ciliary beat frequency in the gill of Mytilus--I. Activation of the lateral cilia by cyclic AMP. Comp Biochem Physiol C. 1987;86(2):273–279. doi: 10.1016/0742-8413(87)90079-x. [DOI] [PubMed] [Google Scholar]
- Oiwa K., Takahashi K. The force-velocity relationship for microtubule sliding in demembranated sperm flagella of the sea urchin. Cell Struct Funct. 1988 Jun;13(3):193–205. doi: 10.1247/csf.13.193. [DOI] [PubMed] [Google Scholar]
- Omoto C. K., Johnson K. A. Activation of the dynein adenosinetriphosphatase by microtubules. Biochemistry. 1986 Jan 28;25(2):419–427. doi: 10.1021/bi00350a022. [DOI] [PubMed] [Google Scholar]
- Sanderson M. J., Dirksen E. R., Satir P. The antagonistic effects of 5-hydroxytryptamine and methylxanthine on the gill cilia of Mytilus edulis. Cell Motil. 1985;5(4):293–309. doi: 10.1002/cm.970050403. [DOI] [PubMed] [Google Scholar]
- Satir P., Barkalow K., Hamasaki T. The control of ciliary beat frequency. Trends Cell Biol. 1993 Nov;3(11):409–412. doi: 10.1016/0962-8924(93)90092-f. [DOI] [PubMed] [Google Scholar]
- Satir P., Wais-Steider J., Lebduska S., Nasr A., Avolio J. The mechanochemical cycle of the dynein arm. Cell Motil. 1981;1(3):303–327. doi: 10.1002/cm.970010304. [DOI] [PubMed] [Google Scholar]
- Svoboda K., Schmidt C. F., Schnapp B. J., Block S. M. Direct observation of kinesin stepping by optical trapping interferometry. Nature. 1993 Oct 21;365(6448):721–727. doi: 10.1038/365721a0. [DOI] [PubMed] [Google Scholar]
- Takahashi K., Shingyoji C., Kamimura S. Microtubule sliding in reactivated flagella. Symp Soc Exp Biol. 1982;35:159–177. [PubMed] [Google Scholar]
- Uyeda T. Q., Kron S. J., Spudich J. A. Myosin step size. Estimation from slow sliding movement of actin over low densities of heavy meromyosin. J Mol Biol. 1990 Aug 5;214(3):699–710. doi: 10.1016/0022-2836(90)90287-V. [DOI] [PubMed] [Google Scholar]
- Vale R. D., Malik F., Brown D. Directional instability of microtubule transport in the presence of kinesin and dynein, two opposite polarity motor proteins. J Cell Biol. 1992 Dec;119(6):1589–1596. doi: 10.1083/jcb.119.6.1589. [DOI] [PMC free article] [PubMed] [Google Scholar]
- Vale R. D., Toyoshima Y. Y. Microtubule translocation properties of intact and proteolytically digested dyneins from Tetrahymena cilia. J Cell Biol. 1989 Jun;108(6):2327–2334. doi: 10.1083/jcb.108.6.2327. [DOI] [PMC free article] [PubMed] [Google Scholar]
- Vale R. D., Toyoshima Y. Y. Rotation and translocation of microtubules in vitro induced by dyneins from Tetrahymena cilia. Cell. 1988 Feb 12;52(3):459–469. doi: 10.1016/s0092-8674(88)80038-2. [DOI] [PubMed] [Google Scholar]
- Walczak C. E., Nelson D. L. Regulation of dynein-driven motility in cilia and flagella. Cell Motil Cytoskeleton. 1994;27(2):101–107. doi: 10.1002/cm.970270202. [DOI] [PubMed] [Google Scholar]
- Yanagida T., Harada Y., Ishijima A. Nano-manipulation of actomyosin molecular motors in vitro: a new working principle. Trends Biochem Sci. 1993 Sep;18(9):319–324. doi: 10.1016/0968-0004(93)90064-t. [DOI] [PubMed] [Google Scholar]