Abstract
1. When coherent light was passed through isolated isometric cardiac muscles during the diastolic or resting period, intensity fluctuations were observed in the scattered field. The frequency of these intensity fluctuations (f½) varied with many experimental interventions known to enhance Ca2+ flux into the cell.
2. In rat muscles stimulated at low frequencies (0.1 ± 2.0 min-1) stepwise increases (0.4-10 mm) of [Ca2+] in the bathing fluid ([Ca2+]e), or addition of ouabain (10-6-6 × 10-4 m) to the perfusate caused stepwise increases in f½. These were paralleled by increments in resting force (RF) such that the changes in f½ and RF were highly correlated. Substitution of K+ for Na+ in the perfusate resulted in parallel transients in RF and f½.
3. In contrast to the rat, most cat muscles stimulated at low frequencies in the steady state exhibited neither diastolic intensity fluctuations nor Ca2+-dependent changes in RF in [Ca2+]e of 10 mm or less; when [Ca2+]e was increased to 12-32 mm, however, steady-state Ca2+-dependent f½ and RF were observed. In a given [Ca2+]e reduction of [Na+]e increased f½. In the transient state following cessation of regular stimulation at more rapid rates (12-96 min-1) intensity fluctuations were present in all [Ca2+]e and decayed with time (seconds to minutes); the f½ and time course of the decay of the fluctuations were determined by the rate of prior stimulation and [Ca2+]e.
4. Maximum potentiation of twitch force in response to the above inotropic interventions was associated with an optimal level of f½ which was similar in both species; when higher levels of f½ were produced by more intense inotropic intervention, twitch force declined. Over the range of inotropic intervention up to and including that at which maximum twitch potentiation occurred, the increase in diastolic f½ predicted the extent of twitch potentiation with a high degree of accuracy (r > 0.97) both in the transient and steady states.
5. In contrast to the other inotropic interventions studied, catecholamines were unique in that neither f½ nor RF increased over a full range of concentrations that resulted in maximum potentiation of the twitch.
6. It is concluded from these observations that f½ reflects diastolic Ca2+-dependent myofilament interaction; the increase in the extent of this interaction by inotropic interventions that do not alter the affinity of the myofilaments for Ca2+ probably reflects an increase in diastolic myoplasmic [Ca2+], an optimal level of which is associated with maximal potentiation of twitch force; the difference in f½ in rat and cat muscles under a given set of in vitro conditions may be related to the marked species difference in the effectiveness of excitation—contraction coupling.
Full text
PDF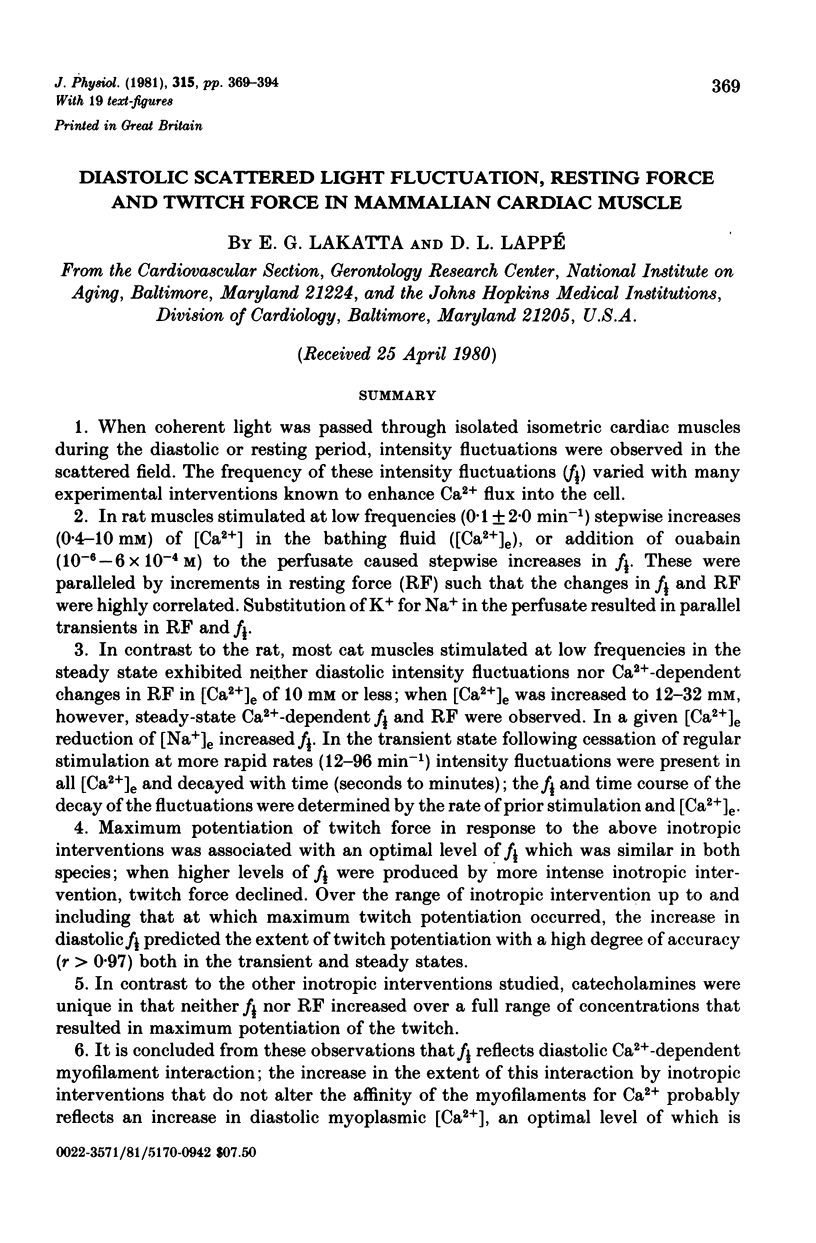
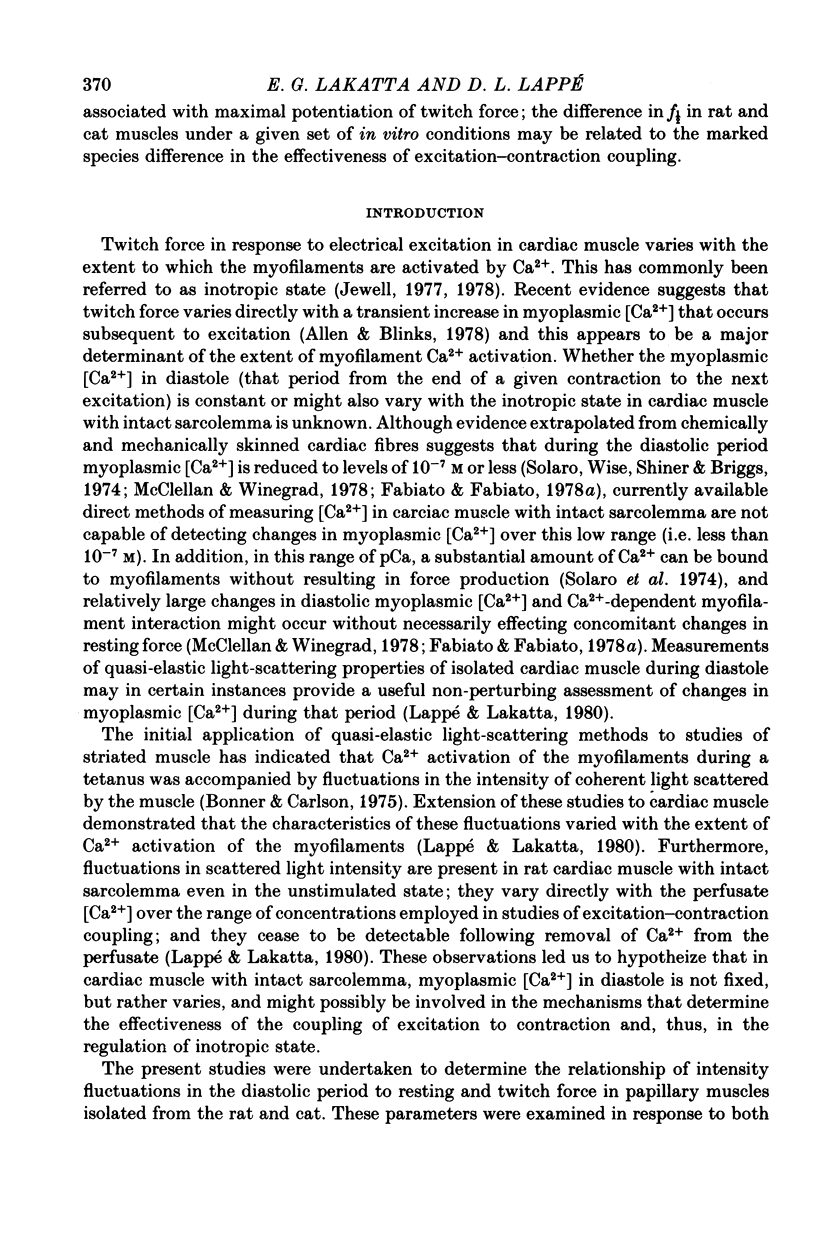
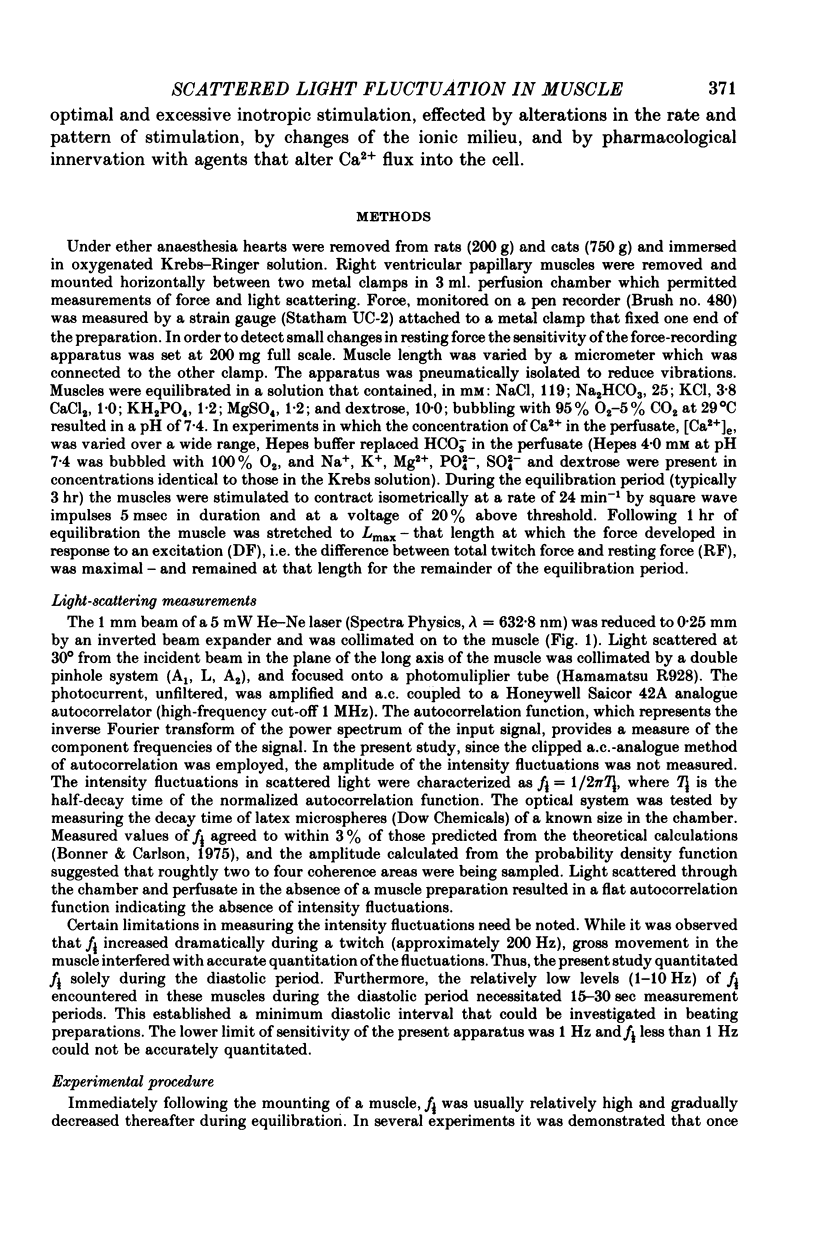
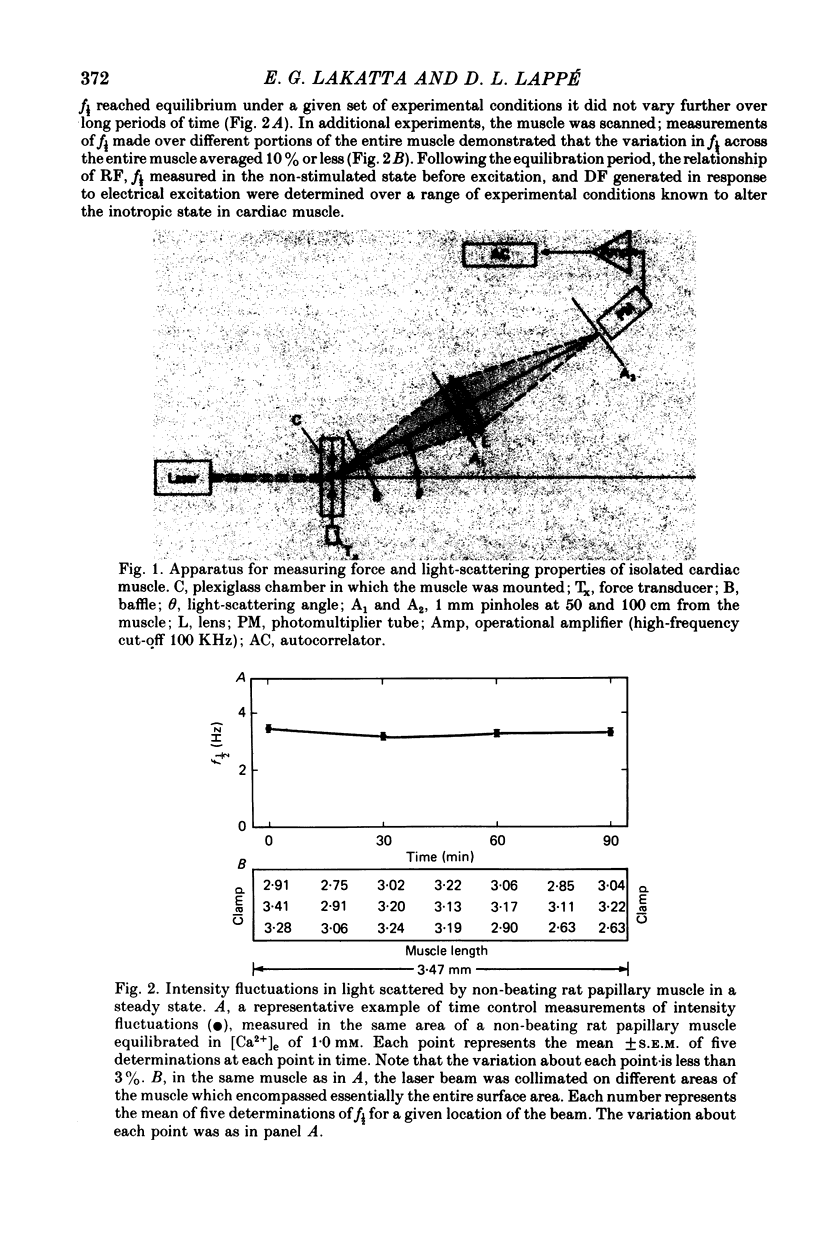
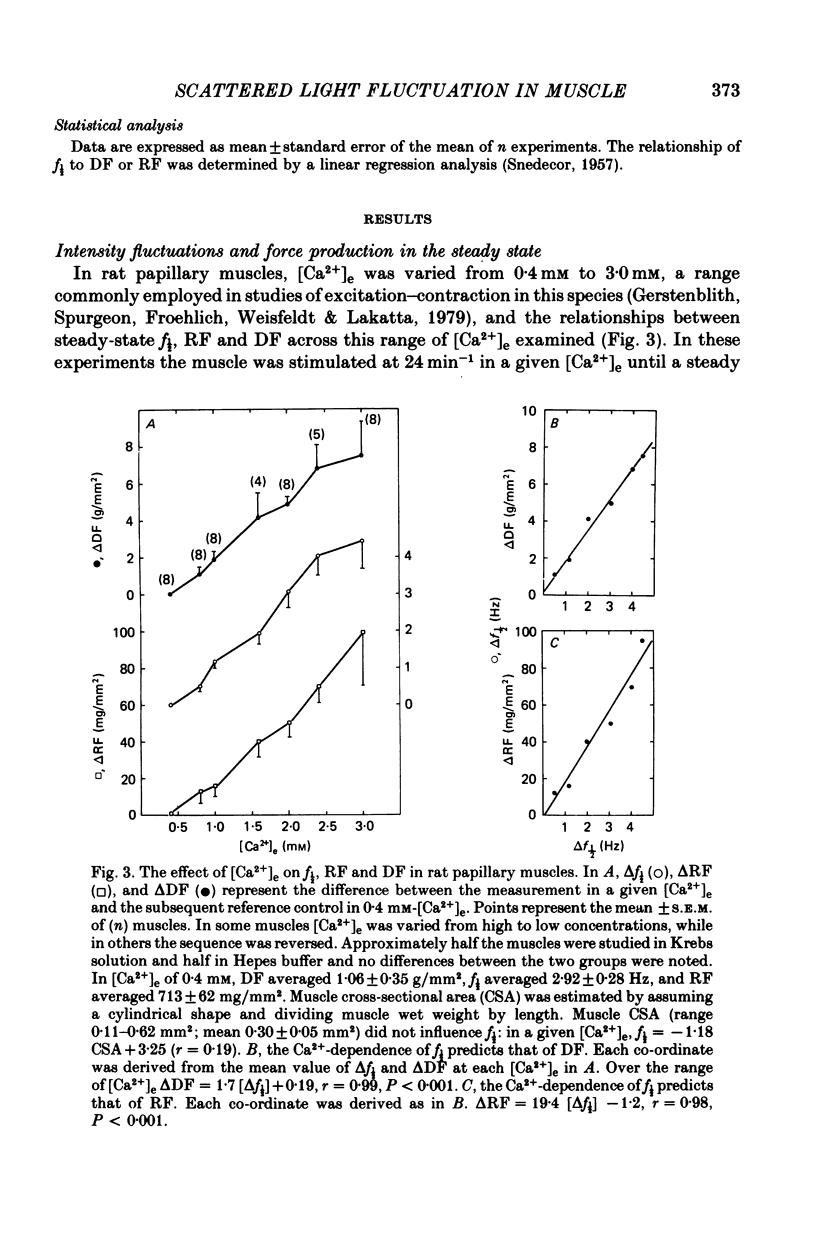
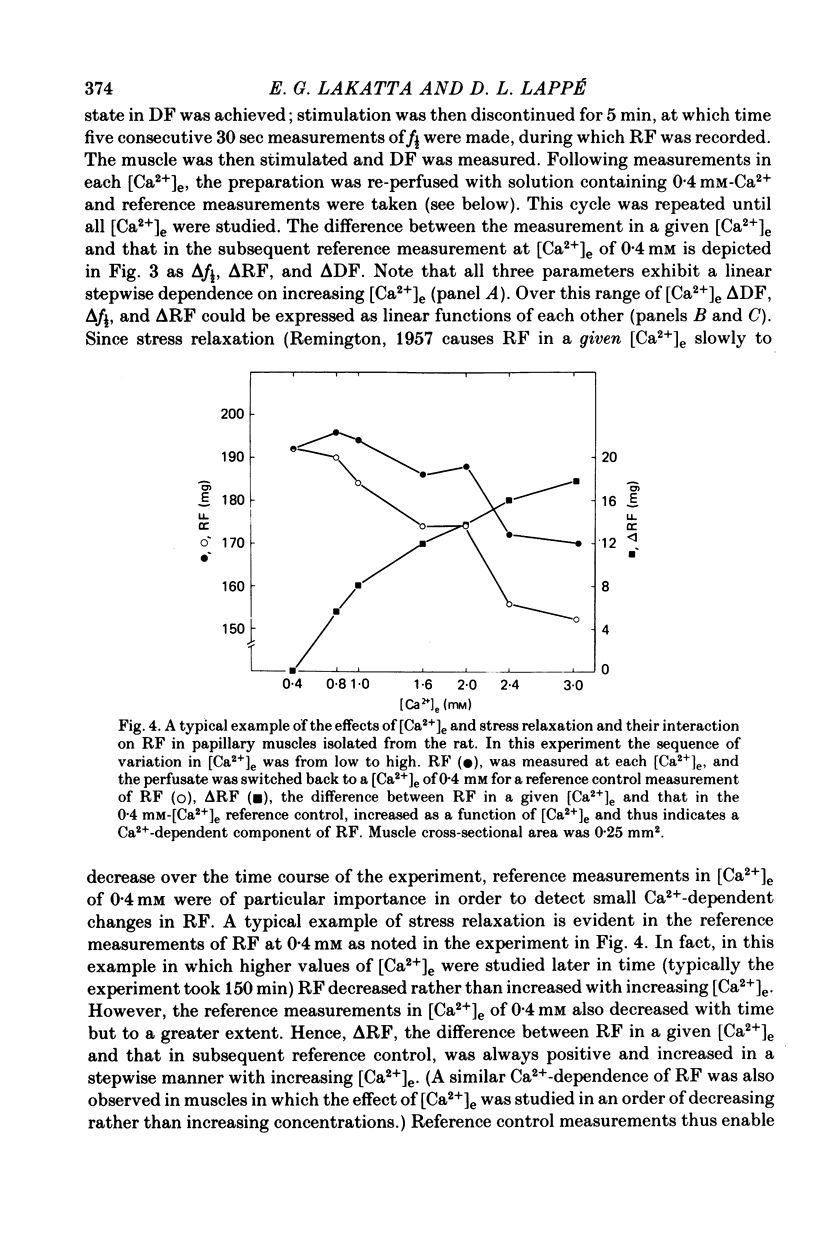
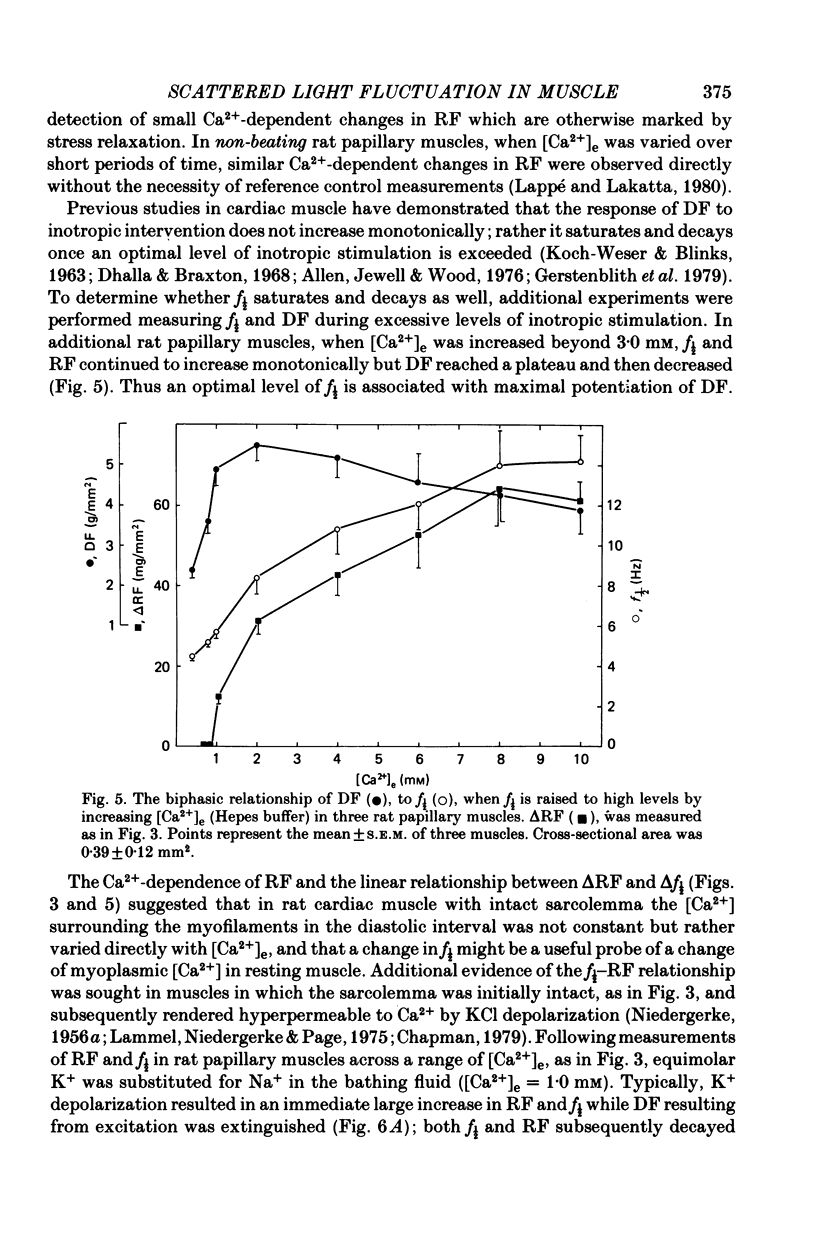
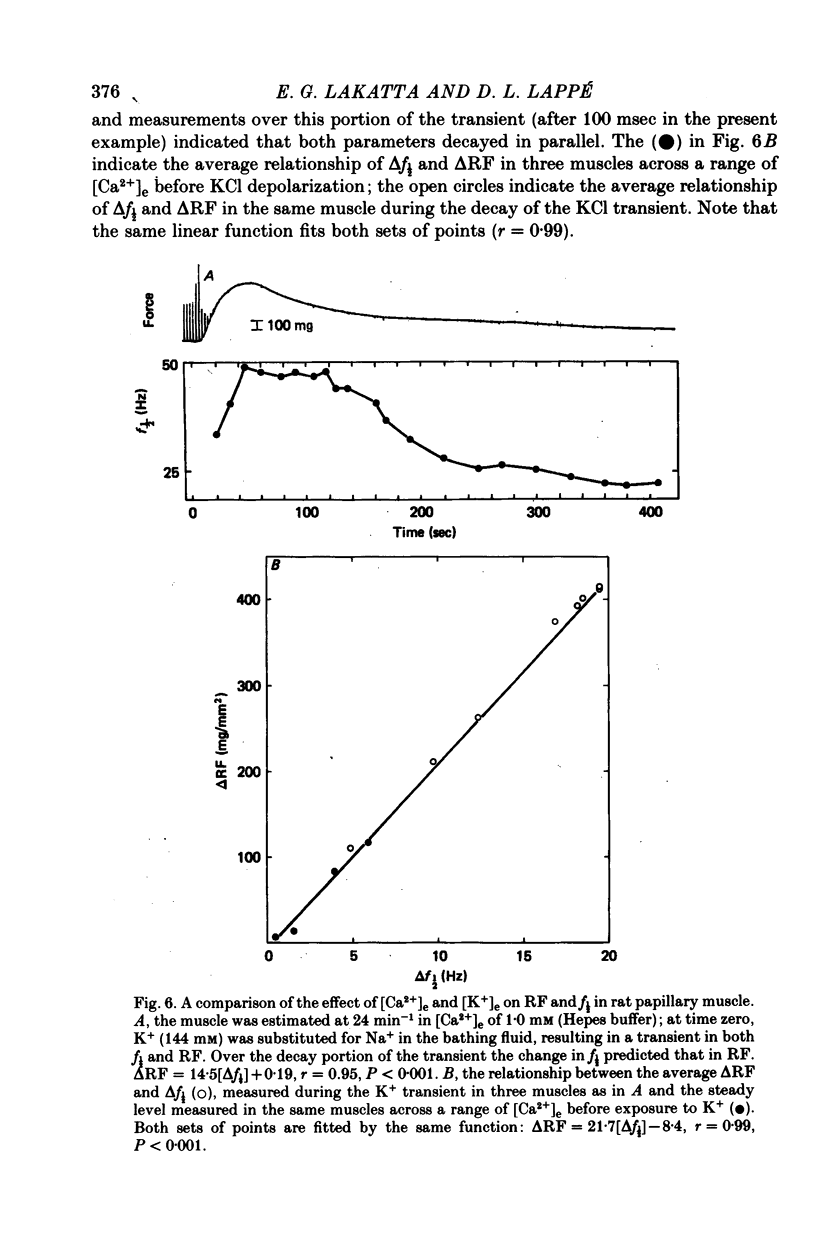
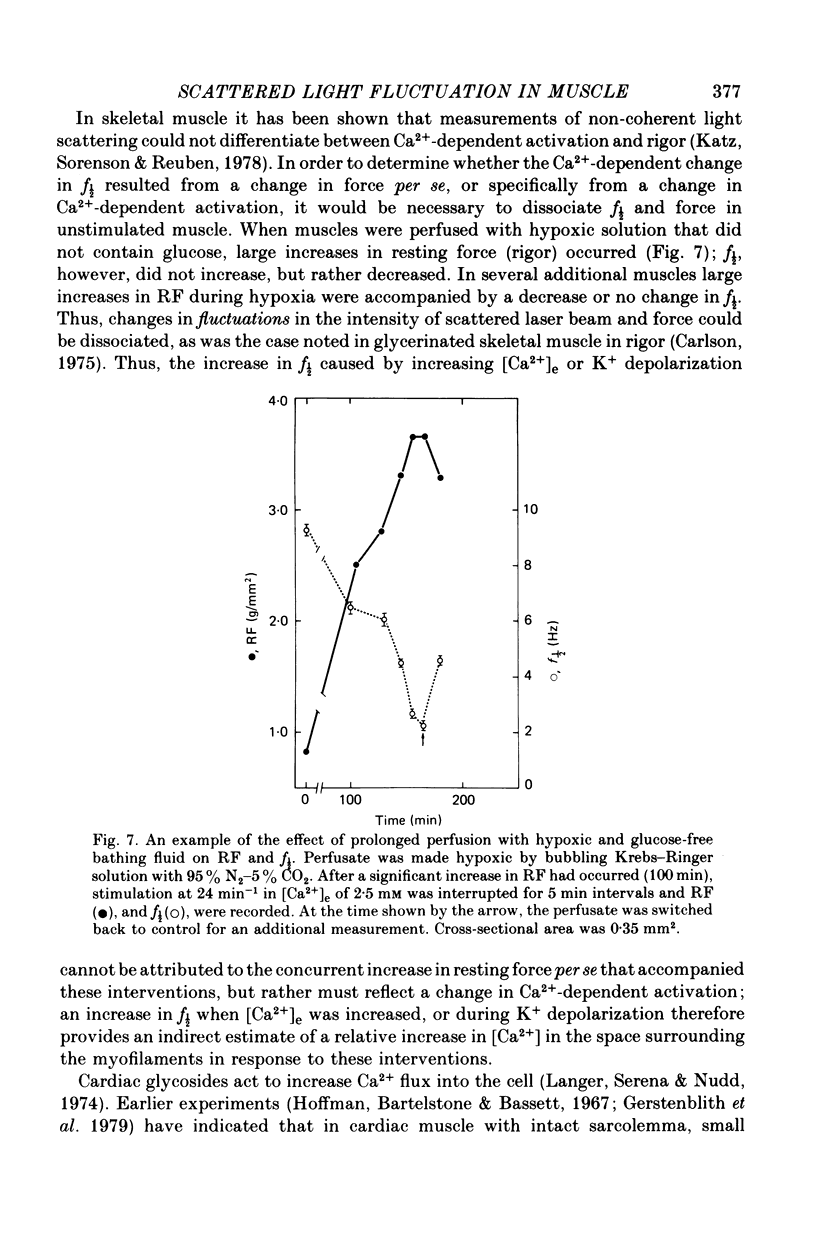
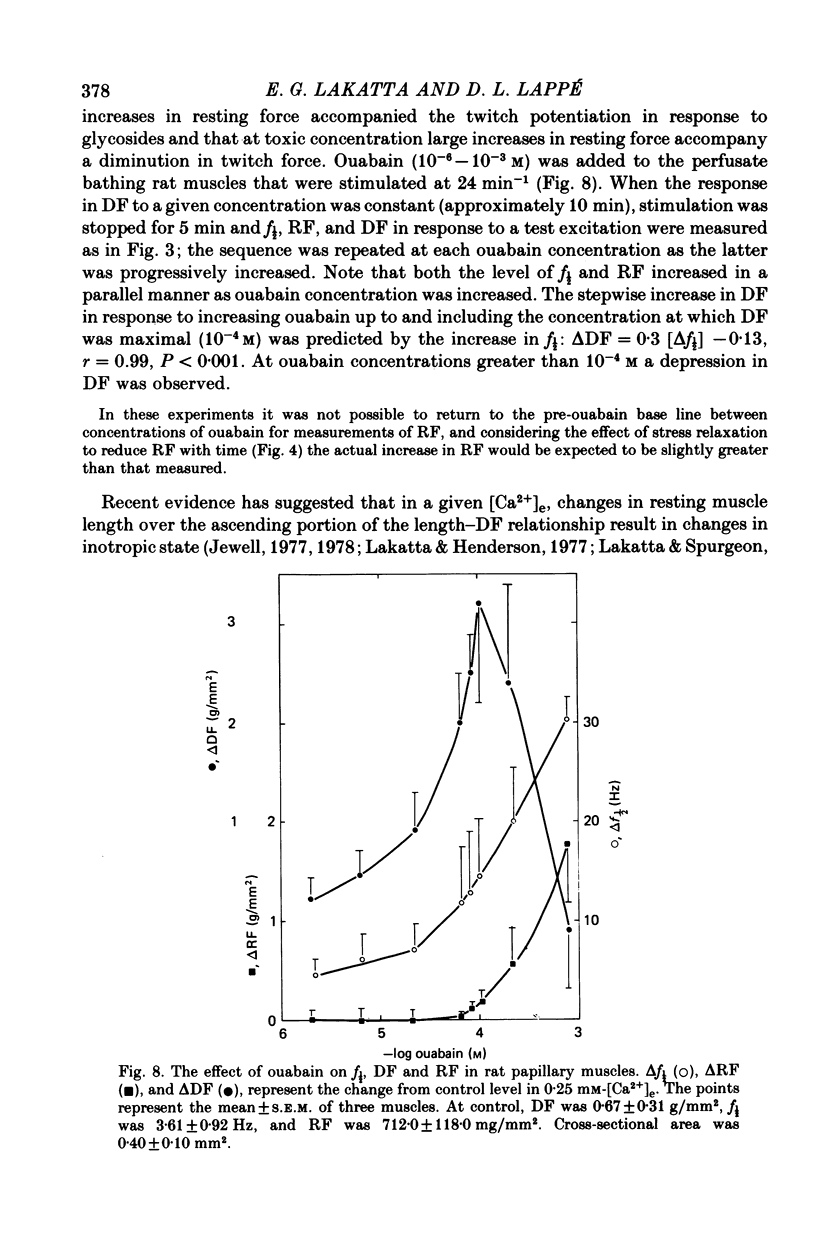
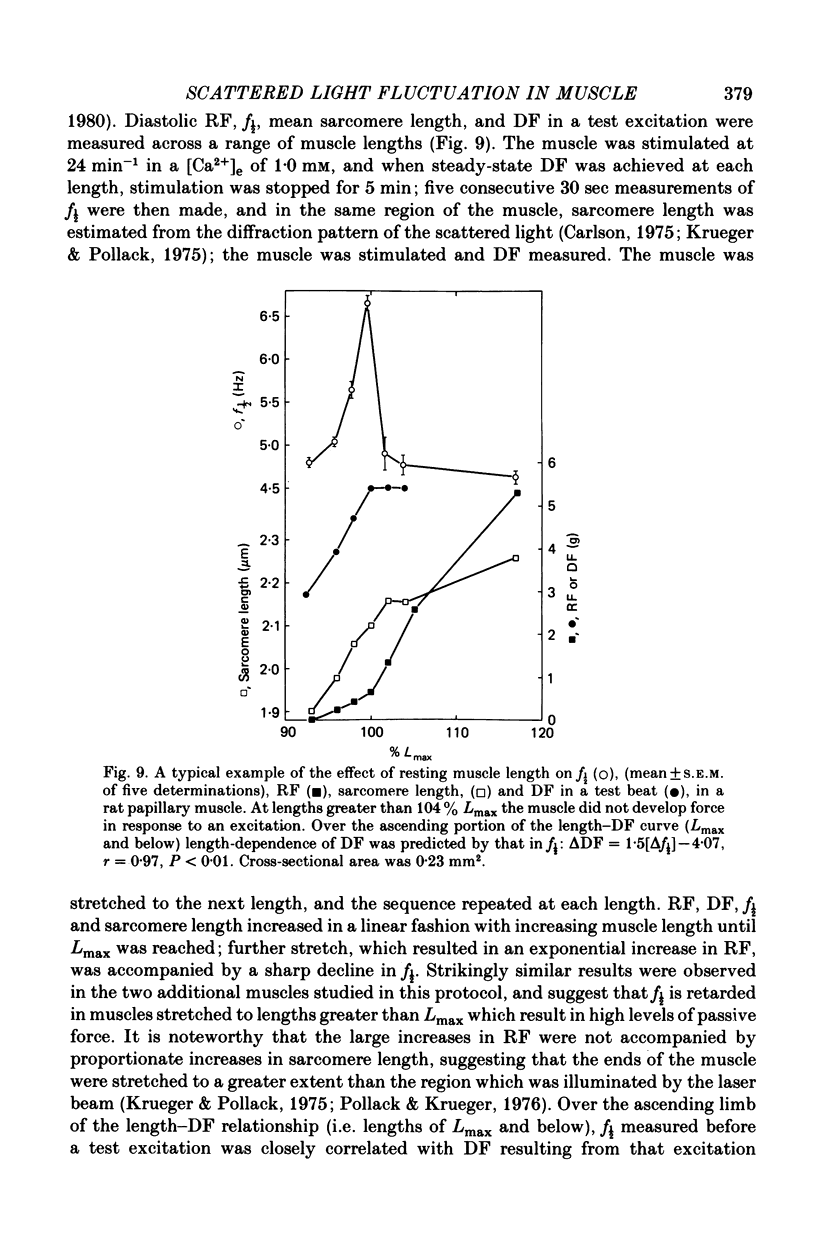
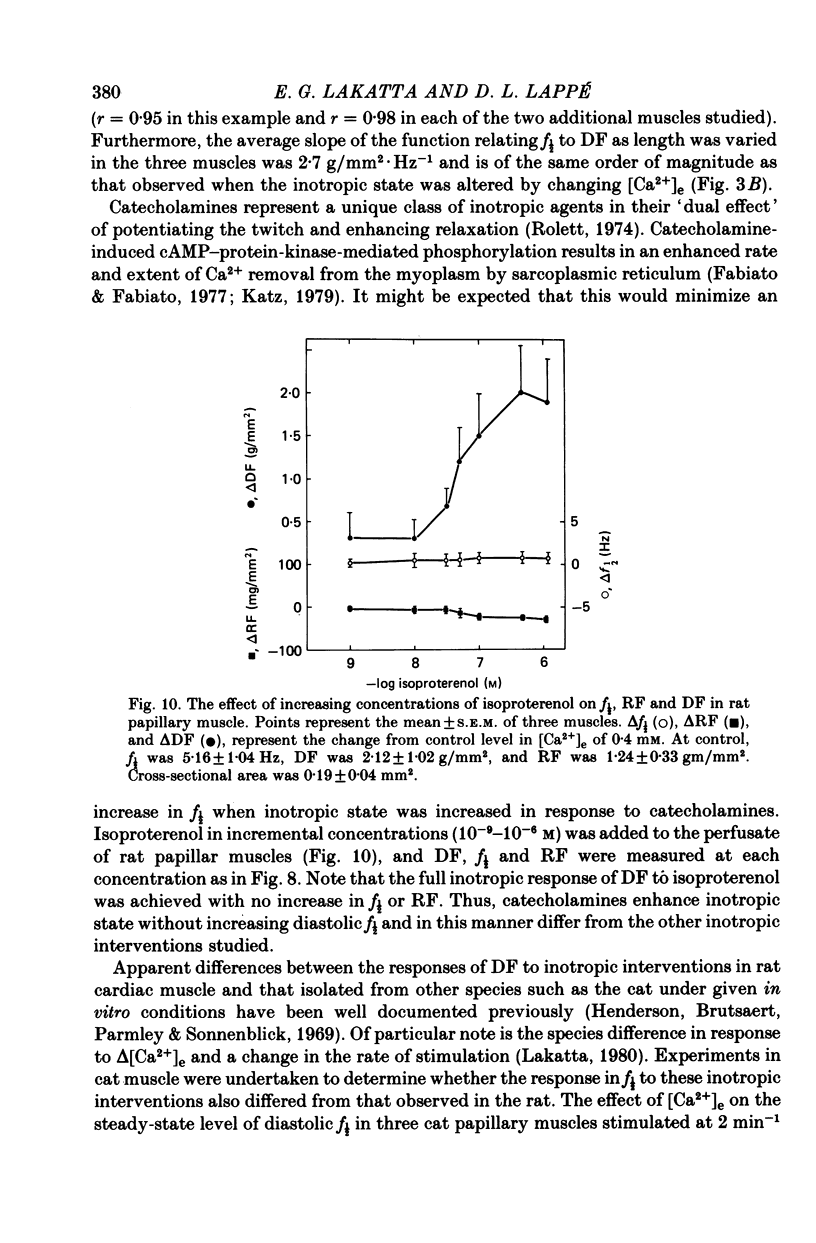
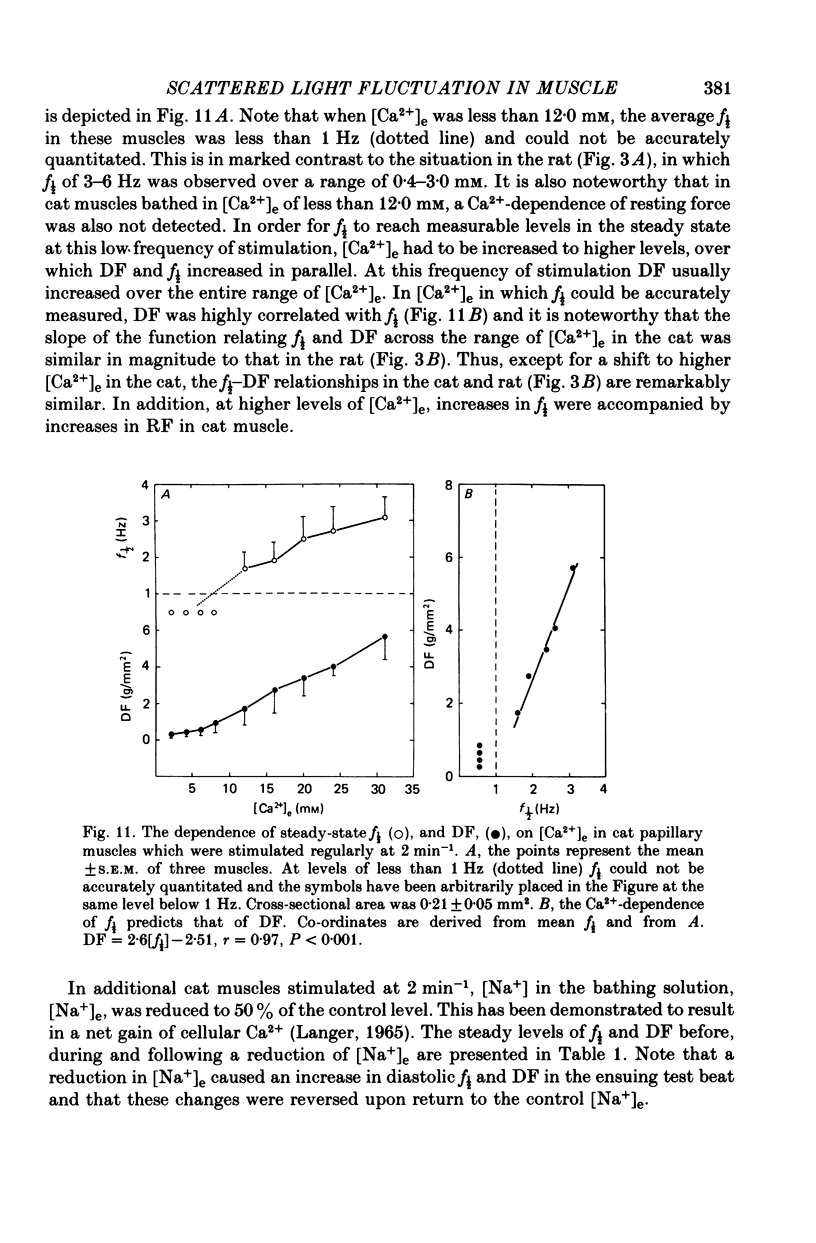
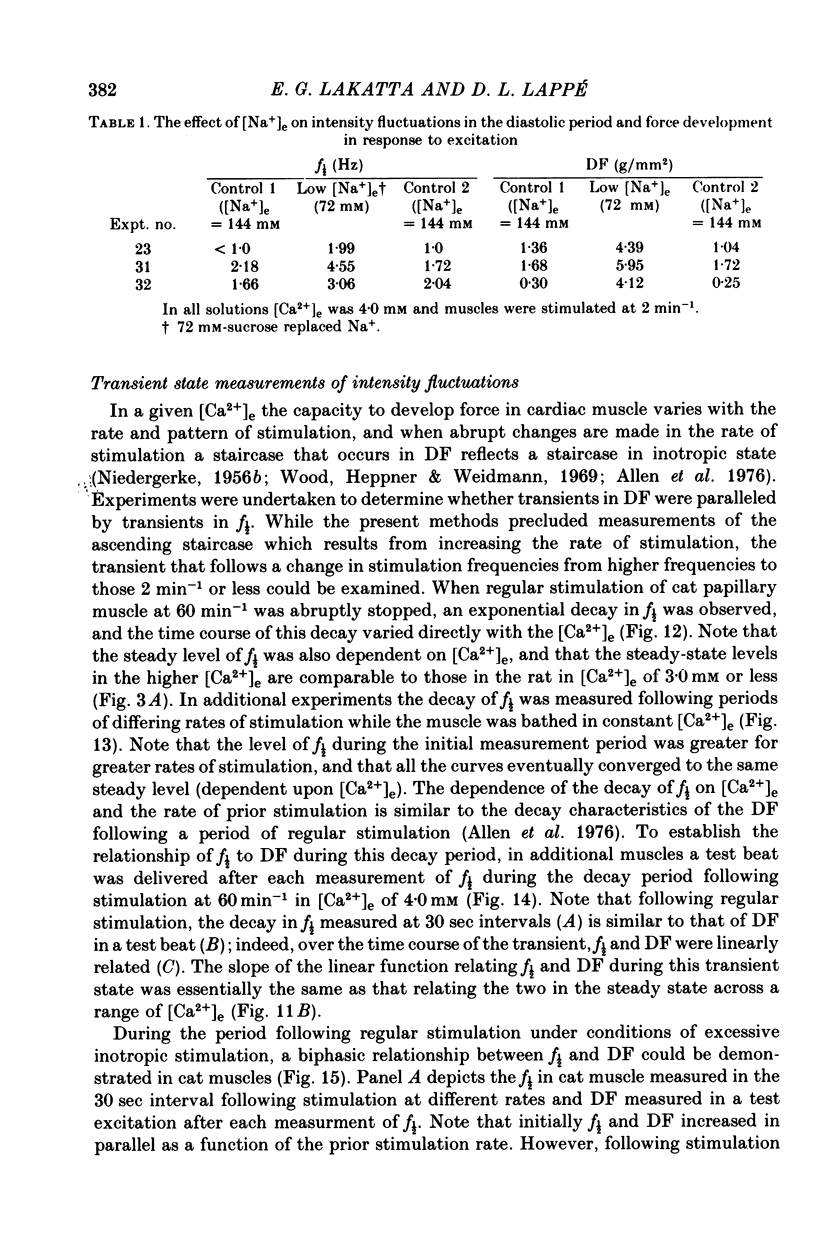
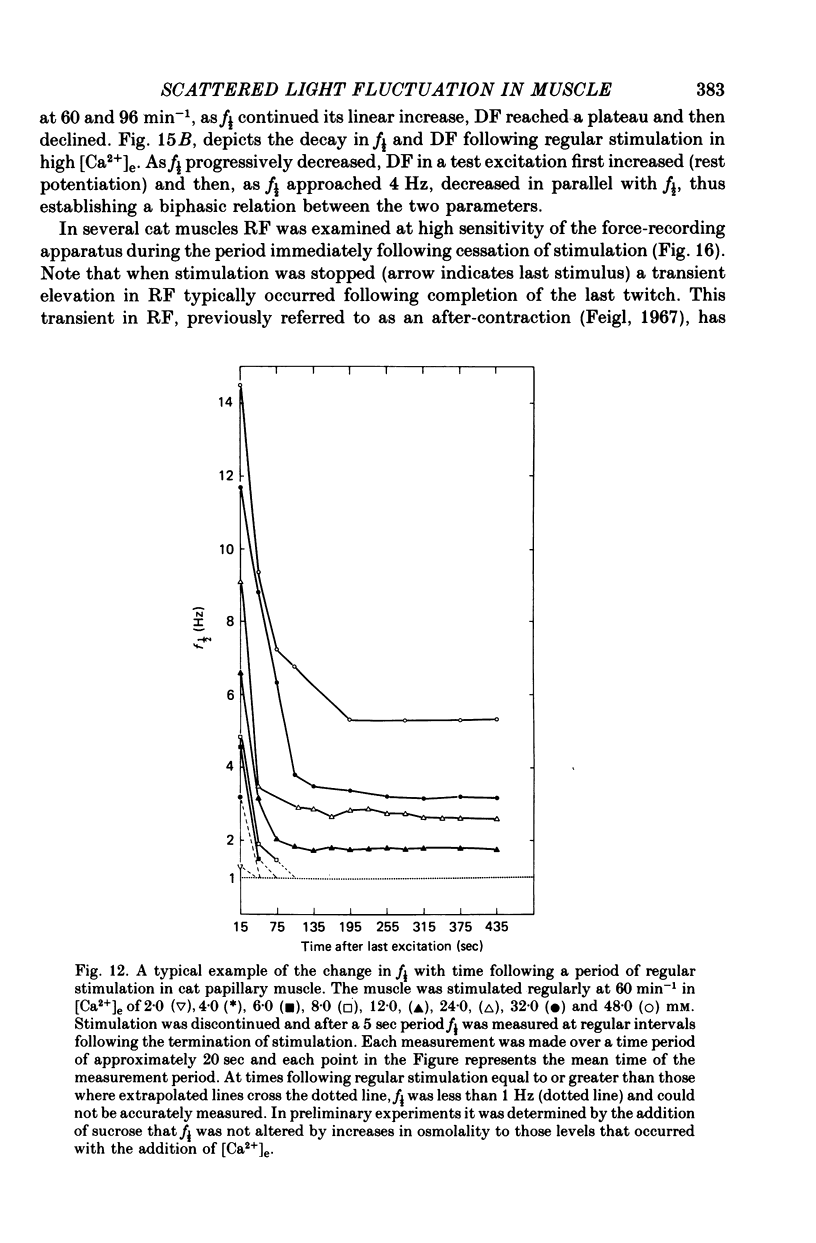
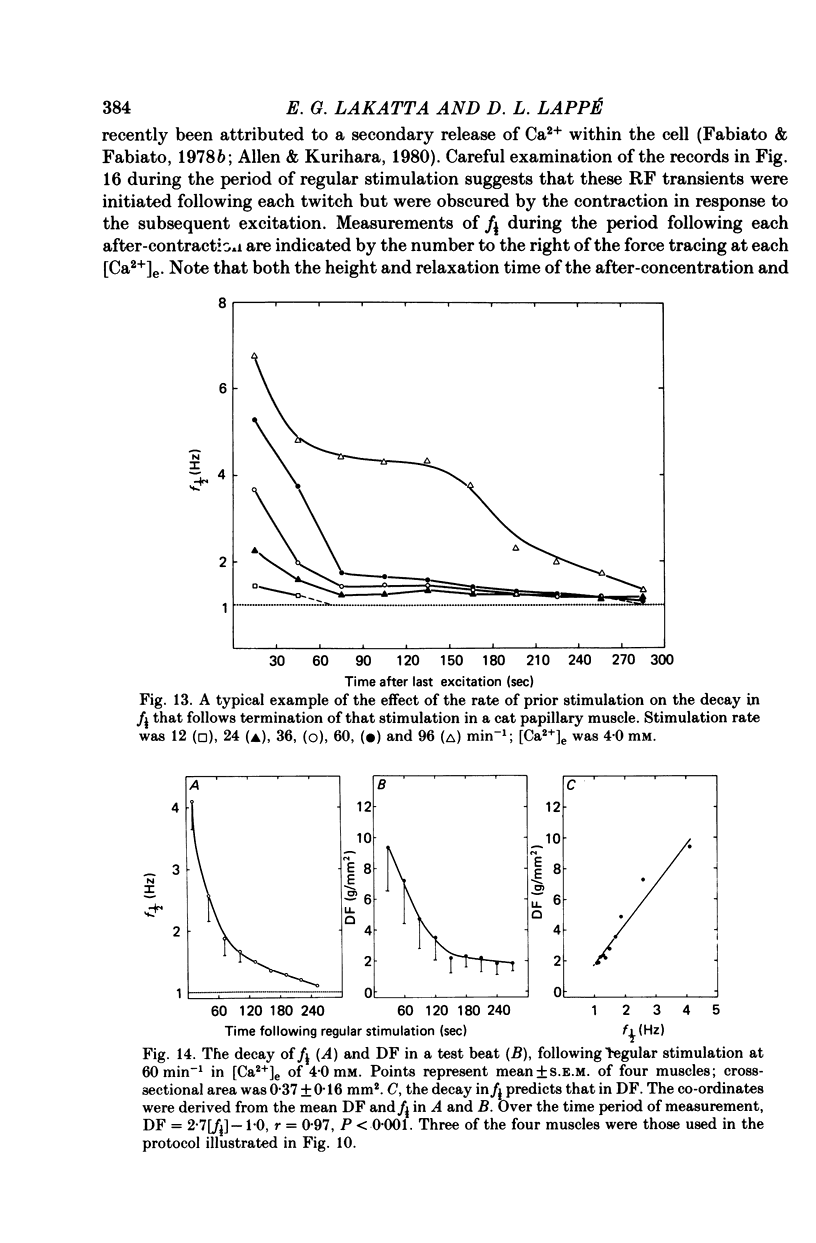
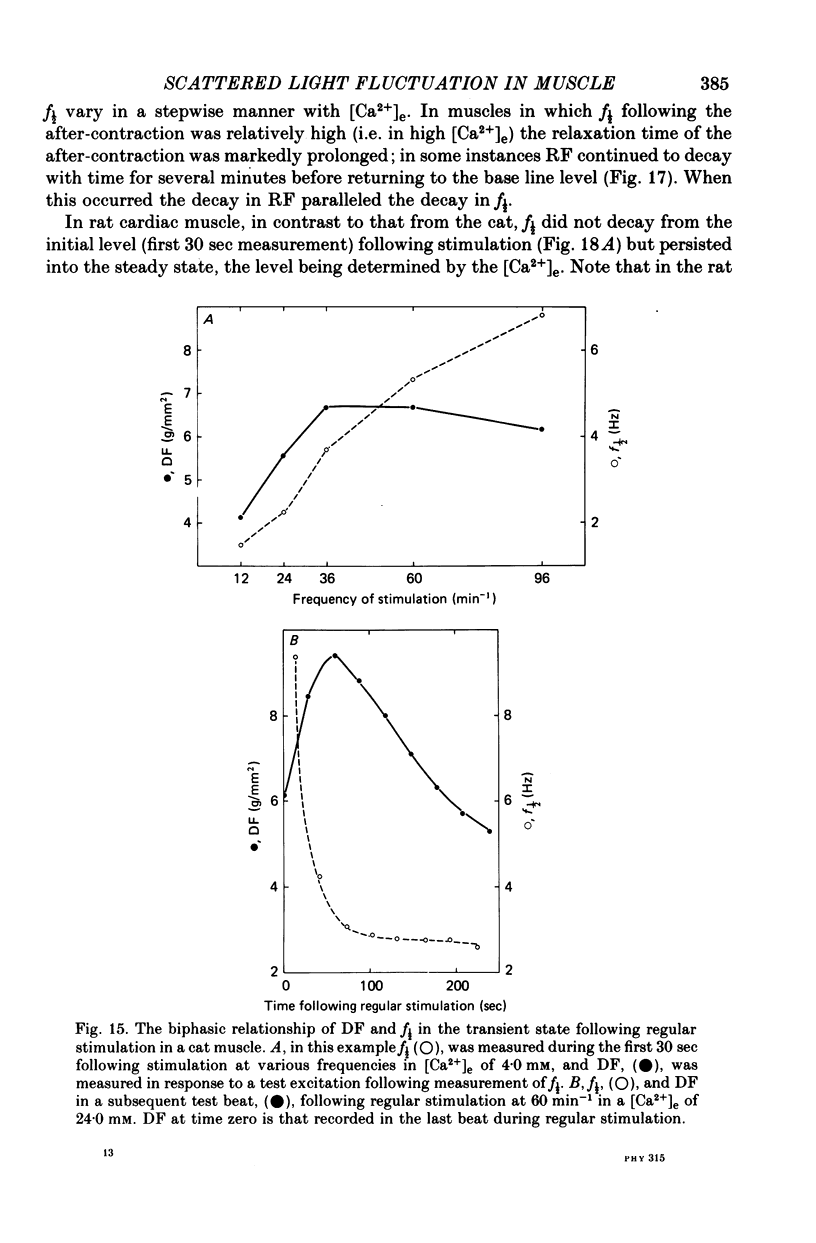
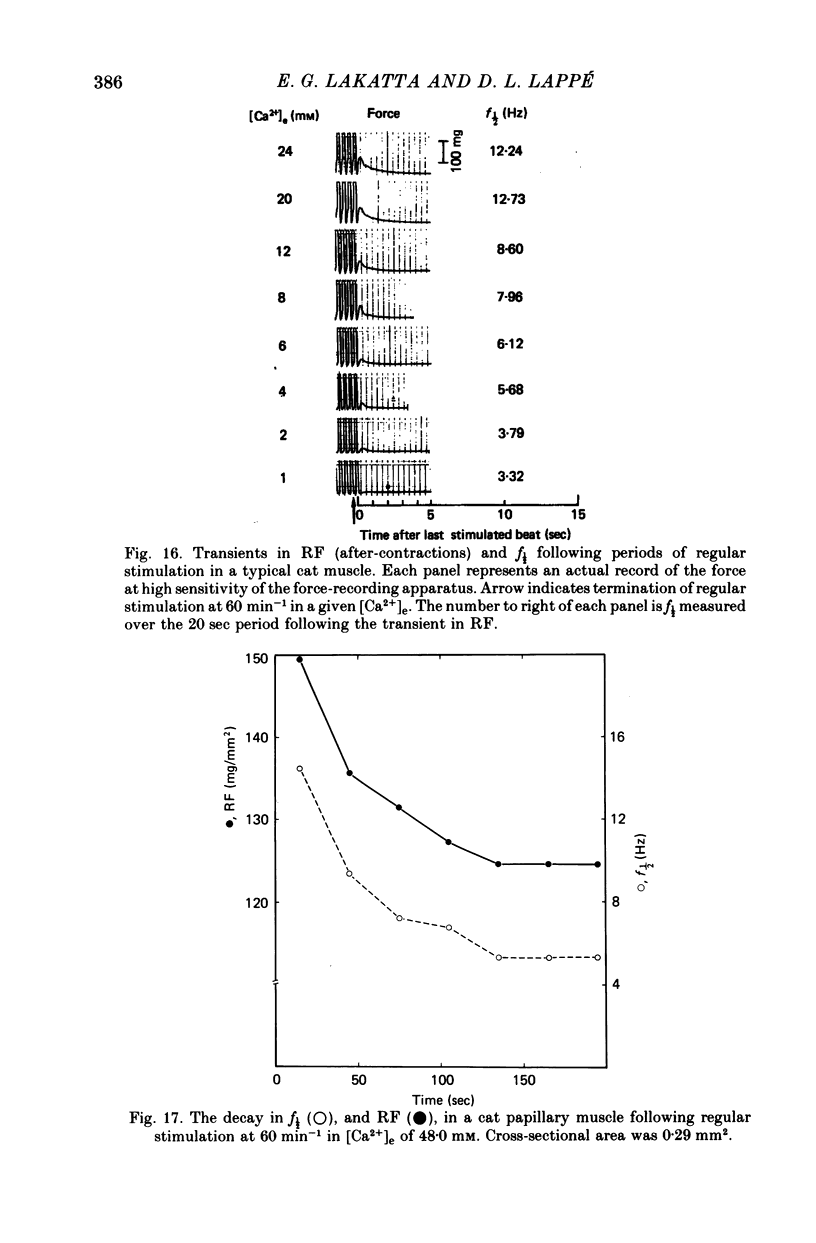
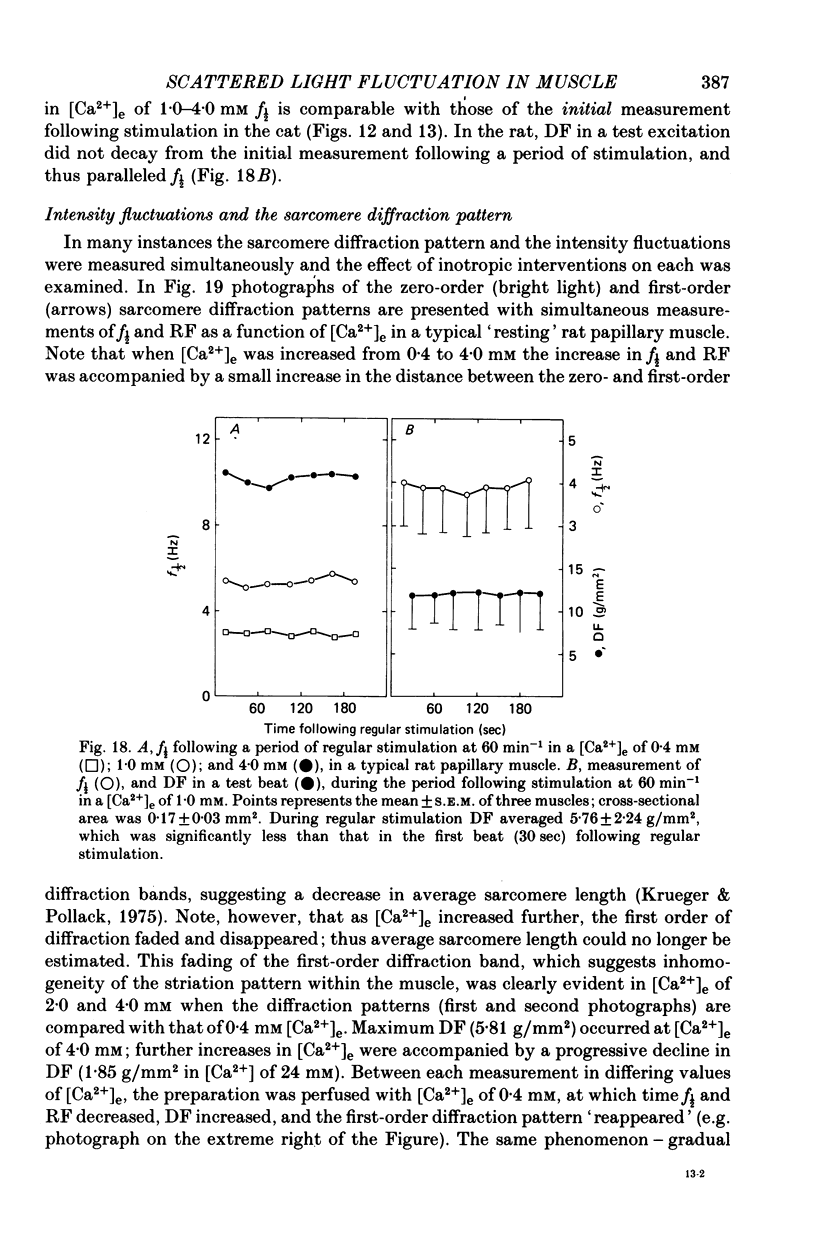
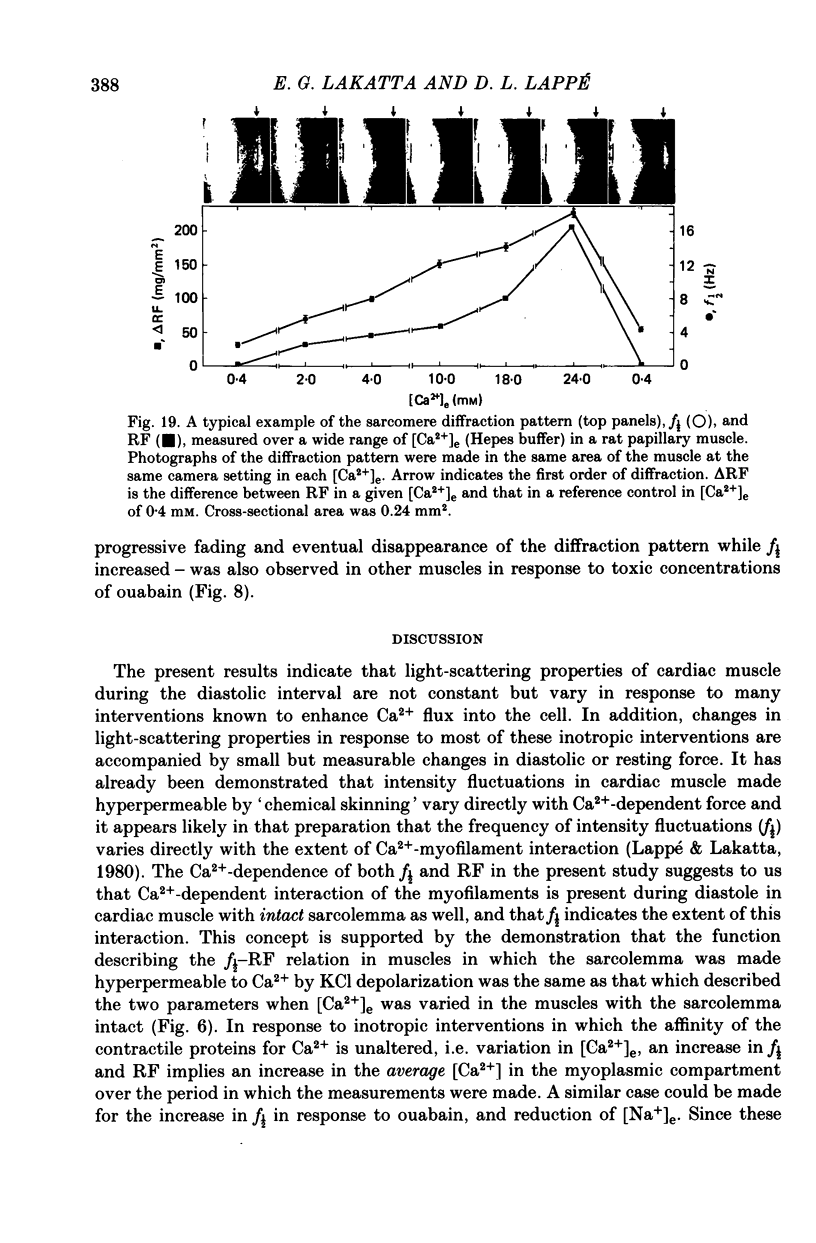
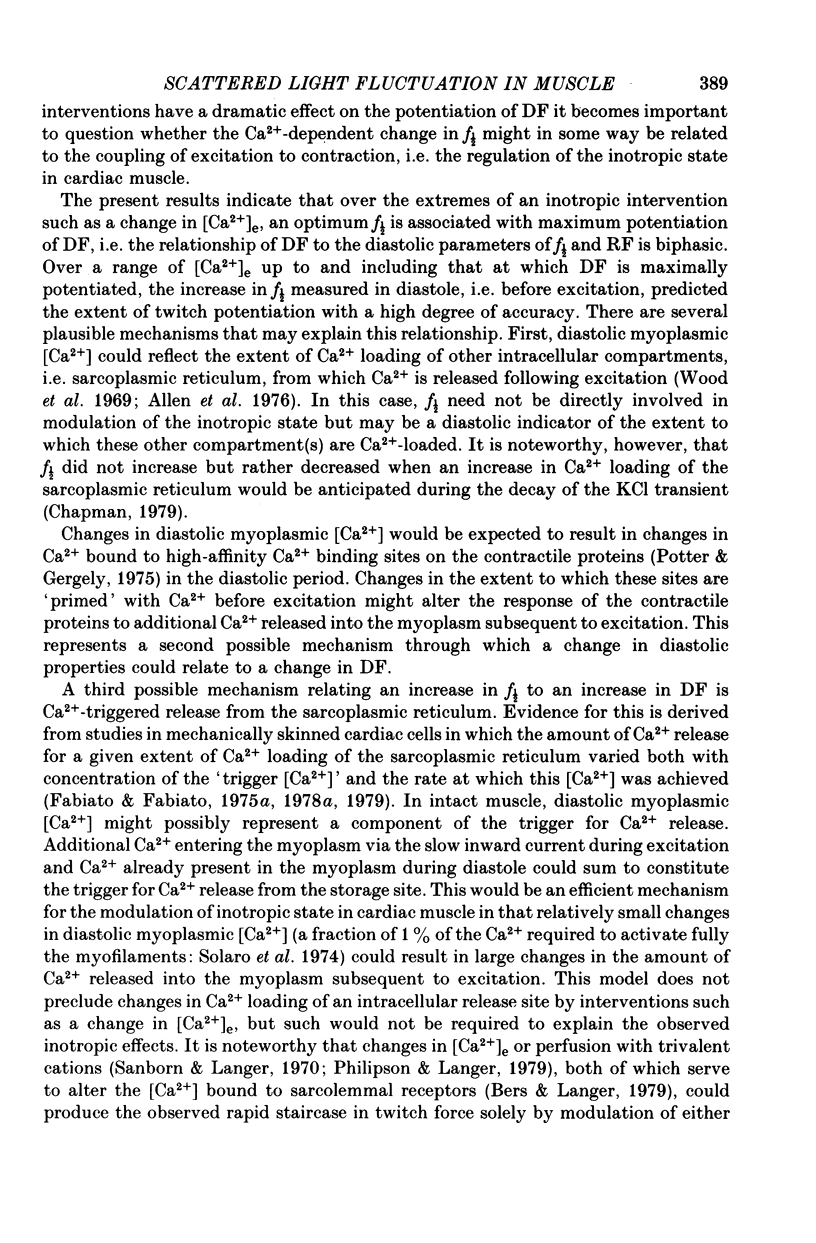
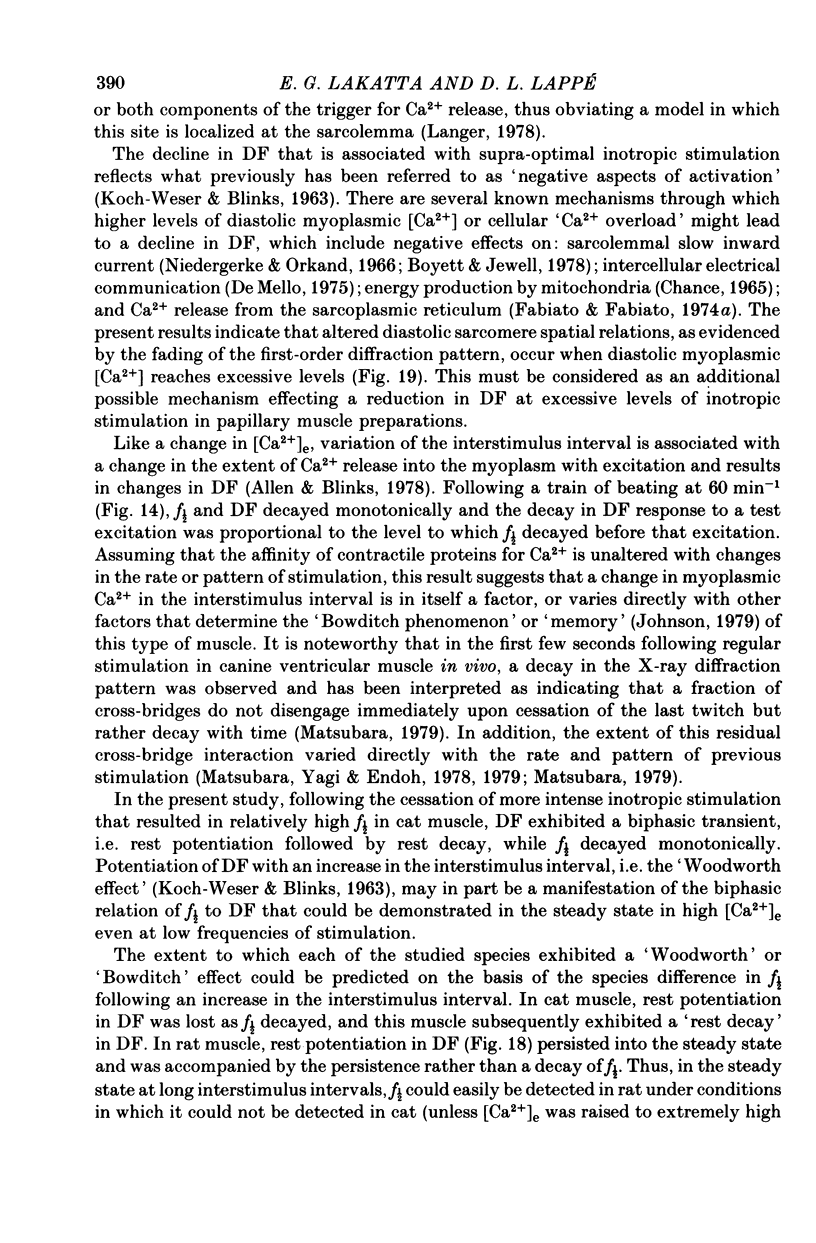
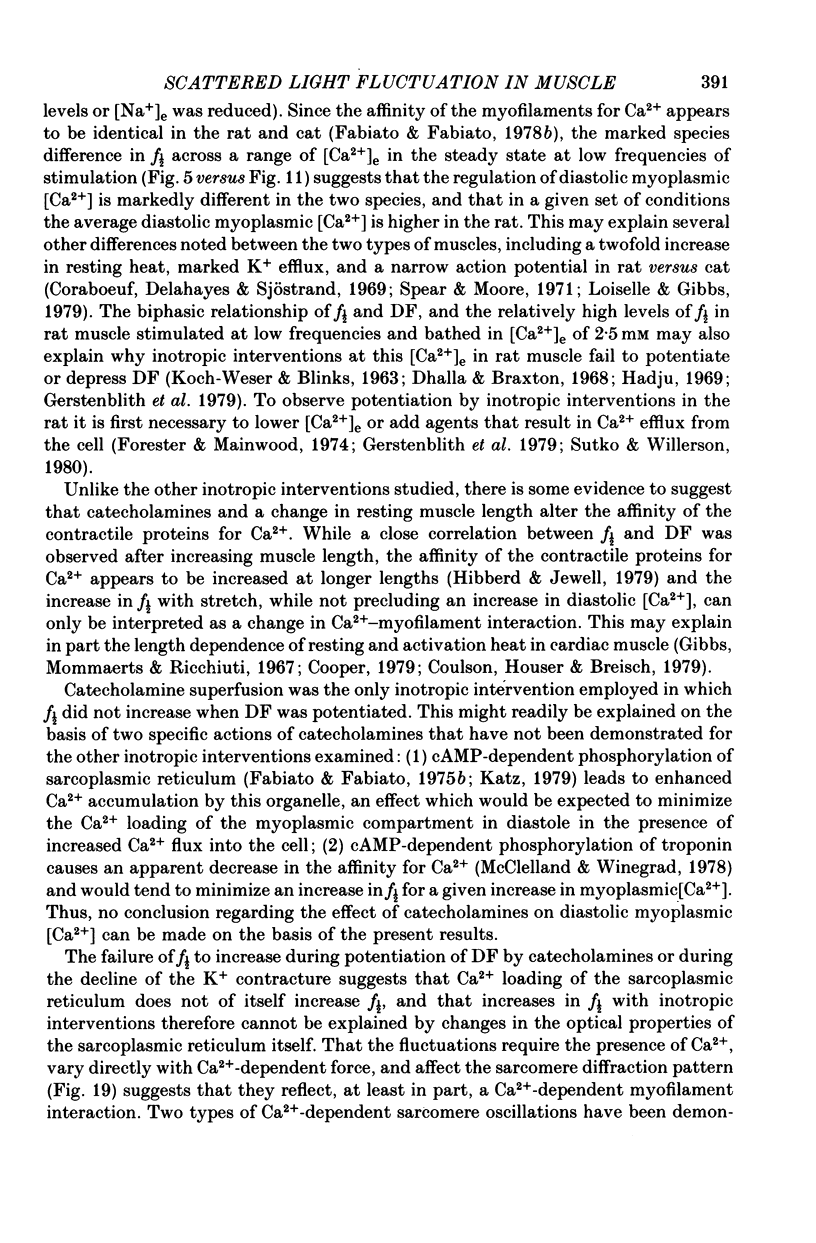
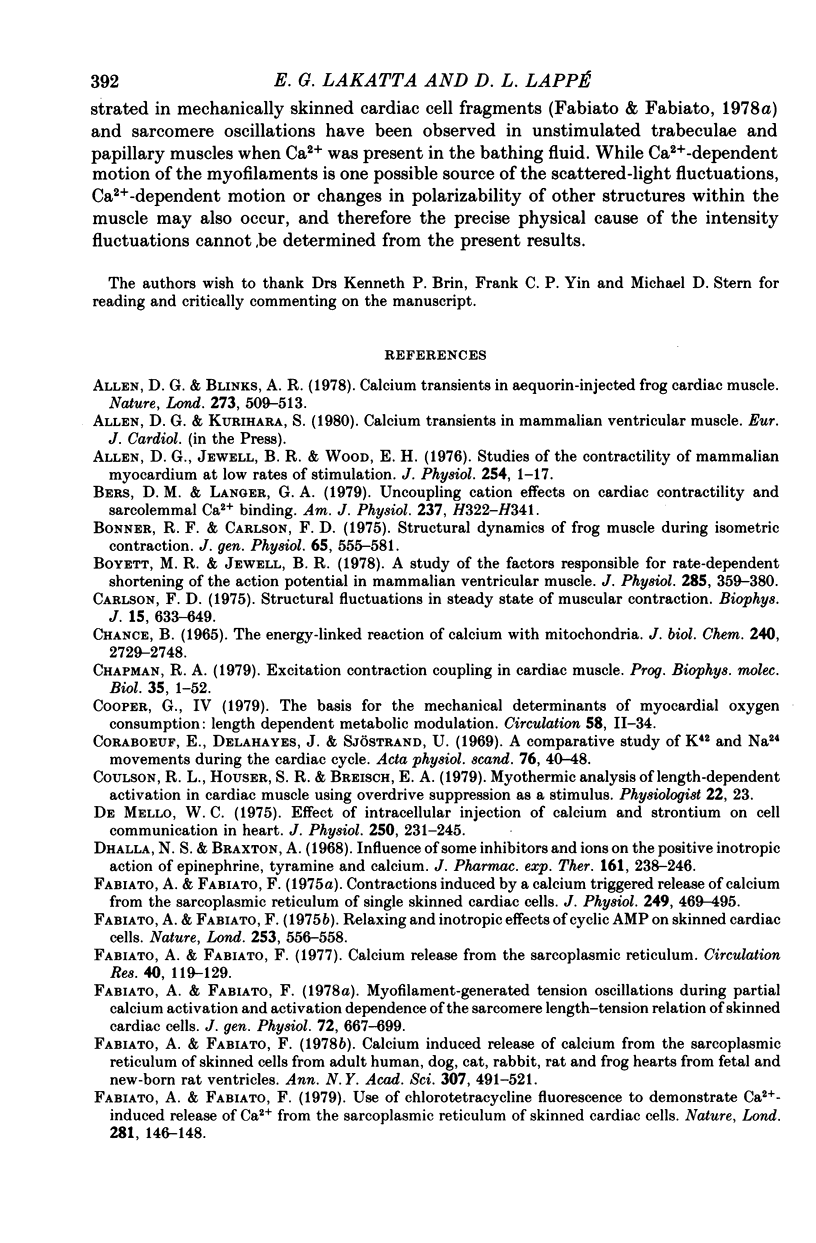
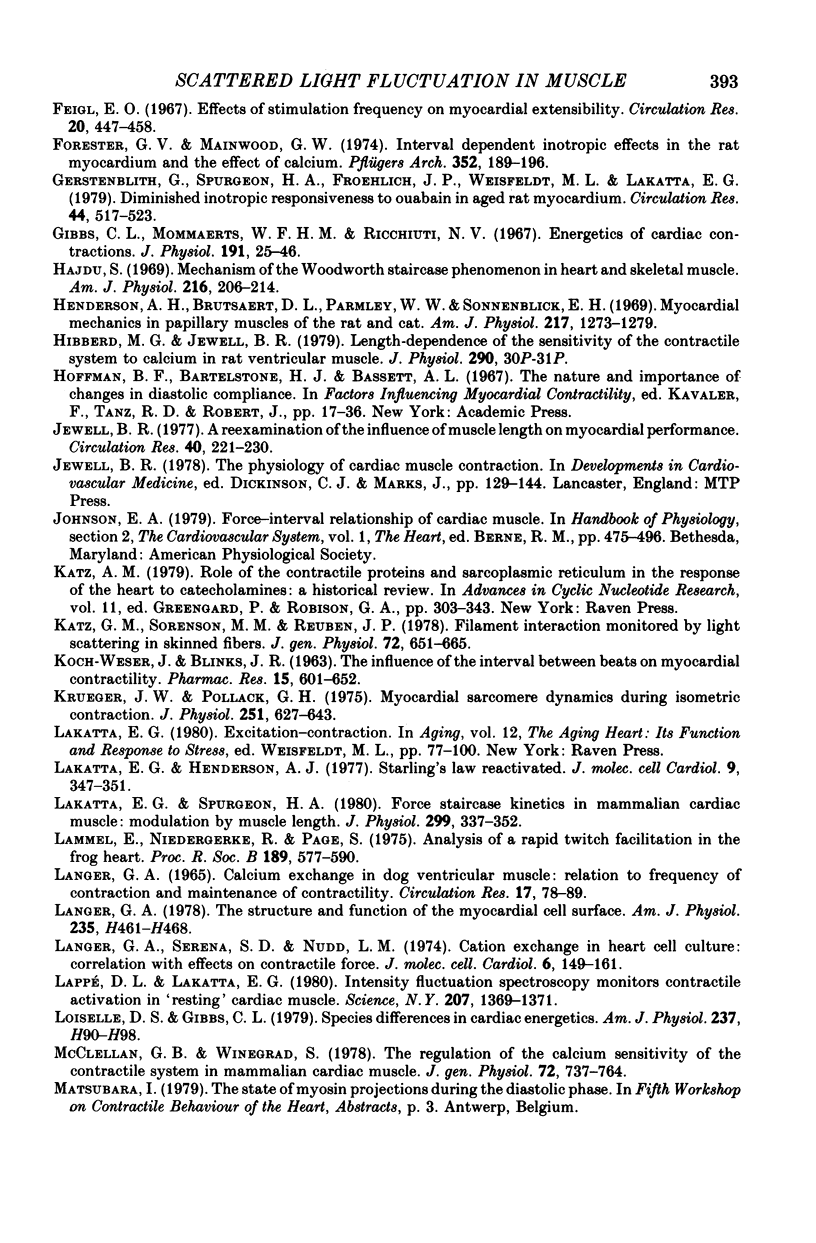
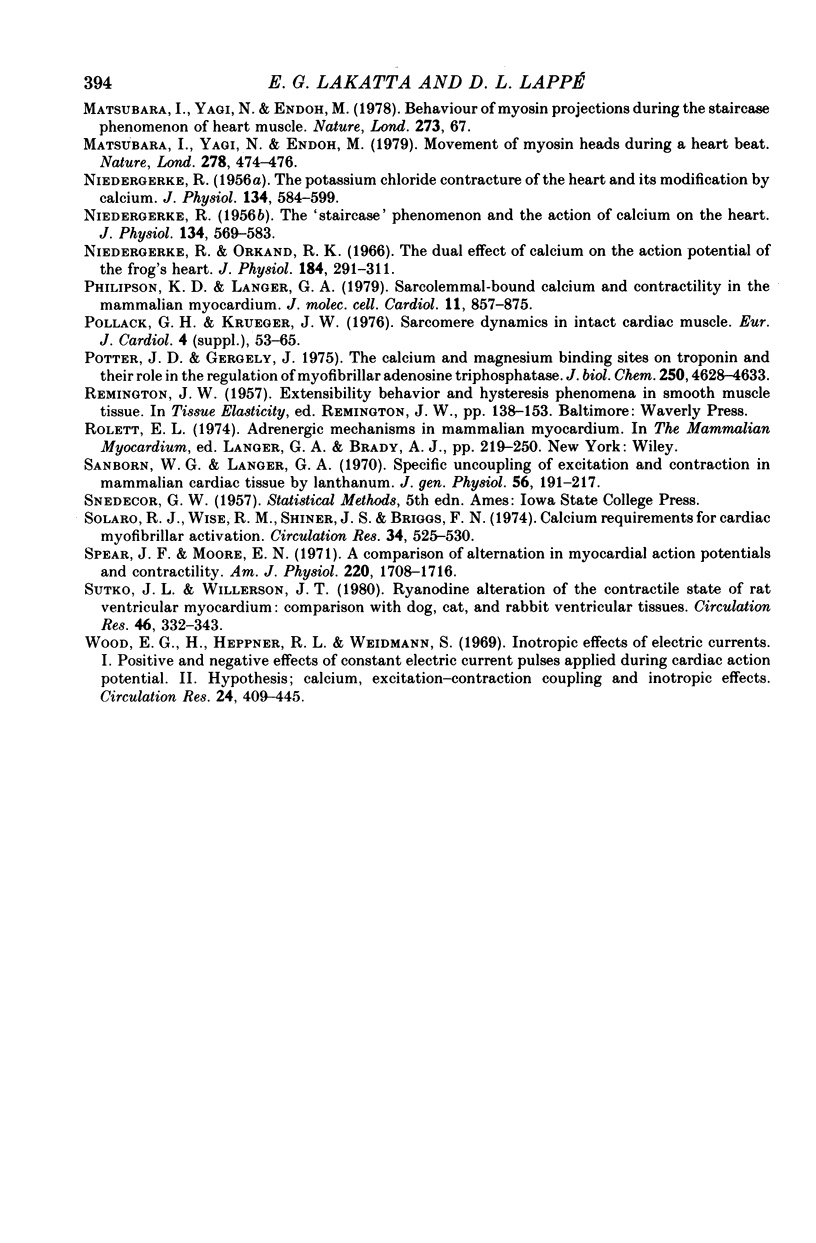
Selected References
These references are in PubMed. This may not be the complete list of references from this article.
- Allen D. G., Blinks J. R. Calcium transients in aequorin-injected frog cardiac muscle. Nature. 1978 Jun 15;273(5663):509–513. doi: 10.1038/273509a0. [DOI] [PubMed] [Google Scholar]
- Allen D. G., Jewell B. R., Wood E. H. Studies of the contractility of mammalian myocardium at low rates of stimulation. J Physiol. 1976 Jan;254(1):1–17. doi: 10.1113/jphysiol.1976.sp011217. [DOI] [PMC free article] [PubMed] [Google Scholar]
- Bers D. M., Langer G. A. Uncoupling cation effects on cardiac contractility and sarcolemmal Ca2+ binding. Am J Physiol. 1979 Sep;237(3):H332–H341. doi: 10.1152/ajpheart.1979.237.3.H332. [DOI] [PubMed] [Google Scholar]
- Bonner R. F., Carlson F. D. Structural dynamics of frog muscle during isometric contraction. J Gen Physiol. 1975 May;65(5):555–581. doi: 10.1085/jgp.65.5.555. [DOI] [PMC free article] [PubMed] [Google Scholar]
- Boyett M. R., Jewell B. R. A study of the factors responsible for rate-dependent shortening of the action potential in mammalian ventricular muscle. J Physiol. 1978 Dec;285:359–380. doi: 10.1113/jphysiol.1978.sp012576. [DOI] [PMC free article] [PubMed] [Google Scholar]
- CHANCE B. THE ENERGY-LINKED REACTION OF CALCIUM WITH MITOCHONDRIA. J Biol Chem. 1965 Jun;240:2729–2748. [PubMed] [Google Scholar]
- Carlson F. D. Structural fluctuations in the steady state of muscular contraction. Biophys J. 1975 Jul;15(7):633–649. doi: 10.1016/S0006-3495(75)85845-0. [DOI] [PMC free article] [PubMed] [Google Scholar]
- Chapman R. A. Excitation-contraction coupling in cardiac muscle. Prog Biophys Mol Biol. 1979;35(1):1–52. doi: 10.1016/0079-6107(80)90002-4. [DOI] [PubMed] [Google Scholar]
- Coraboeuf E., Delahayes J., Sjöstrand U. A comparative study of K42 and Na24 movements during the cardiac cycle. Acta Physiol Scand. 1969 May-Jun;76(1):40–48. doi: 10.1111/j.1748-1716.1969.tb04448.x. [DOI] [PubMed] [Google Scholar]
- De Mello W. C. Effect of intracellular injection of calcium and strontium on cell communication in heart. J Physiol. 1975 Sep;250(2):231–245. doi: 10.1113/jphysiol.1975.sp011051. [DOI] [PMC free article] [PubMed] [Google Scholar]
- Dhalla N. S., Braxton A. Influence of some inhibitors and ions on the positive inotropic action of epinephrine, tyramine and calcium. J Pharmacol Exp Ther. 1968 Jun;161(2):238–246. [PubMed] [Google Scholar]
- Fabiato A., Fabiato F. Calcium release from the sarcoplasmic reticulum. Circ Res. 1977 Feb;40(2):119–129. doi: 10.1161/01.res.40.2.119. [DOI] [PubMed] [Google Scholar]
- Fabiato A., Fabiato F. Calcium-induced release of calcium from the sarcoplasmic reticulum of skinned cells from adult human, dog, cat, rabbit, rat, and frog hearts and from fetal and new-born rat ventricles. Ann N Y Acad Sci. 1978 Apr 28;307:491–522. doi: 10.1111/j.1749-6632.1978.tb41979.x. [DOI] [PubMed] [Google Scholar]
- Fabiato A., Fabiato F. Contractions induced by a calcium-triggered release of calcium from the sarcoplasmic reticulum of single skinned cardiac cells. J Physiol. 1975 Aug;249(3):469–495. doi: 10.1113/jphysiol.1975.sp011026. [DOI] [PMC free article] [PubMed] [Google Scholar]
- Fabiato A., Fabiato F. Myofilament-generated tension oscillations during partial calcium activation and activation dependence of the sarcomere length-tension relation of skinned cardiac cells. J Gen Physiol. 1978 Nov;72(5):667–699. doi: 10.1085/jgp.72.5.667. [DOI] [PMC free article] [PubMed] [Google Scholar]
- Fabiato A., Fabiato F. Relaxing and inotropic effects of cyclic AMP on skinned cardiac cells. Nature. 1975 Feb 13;253(5492):556–558. doi: 10.1038/253556b0. [DOI] [PubMed] [Google Scholar]
- Fabiato A., Fabiato F. Use of chlorotetracycline fluorescence to demonstrate Ca2+-induced release of Ca2+ from the sarcoplasmic reticulum of skinned cardiac cells. Nature. 1979 Sep 13;281(5727):146–148. doi: 10.1038/281146a0. [DOI] [PubMed] [Google Scholar]
- Feigl E. O. Effects of stimulation frequency on myocardial extensibility. Circ Res. 1967 Apr;20(4):447–458. doi: 10.1161/01.res.20.4.447. [DOI] [PubMed] [Google Scholar]
- Forester G. V., Mainwood G. W. Interval dependent inotropic effects in the rat myocardium and the effect of calcium. Pflugers Arch. 1974;352(3):189–196. doi: 10.1007/BF00590484. [DOI] [PubMed] [Google Scholar]
- Gerstenblith G., Spurgeon H. A., Froehlich J. P., Weisfeldt M. L., Lakatta E. G. Diminished inotropic responsiveness to ouabain in aged rat myocardium. Circ Res. 1979 Apr;44(4):517–523. doi: 10.1161/01.res.44.4.517. [DOI] [PubMed] [Google Scholar]
- Gibbs C. L., Mommaerts W. F., Ricchiuti N. V. Energetics of cardiac contractions. J Physiol. 1967 Jul;191(1):25–46. doi: 10.1113/jphysiol.1967.sp008235. [DOI] [PMC free article] [PubMed] [Google Scholar]
- Hajdu S. Mechanism of the Woodworth staircase phenomenon in heart and skeletal muscle. Am J Physiol. 1969 Jan;216(1):206–214. doi: 10.1152/ajplegacy.1969.216.1.206. [DOI] [PubMed] [Google Scholar]
- Henderson A. H., Brutsaert D. L., Parmley W. W., Sonnenblick E. H. Myocardial mechanics in ppillary muscles of the rat and cat. Am J Physiol. 1969 Nov;217(5):1273–1279. doi: 10.1152/ajplegacy.1969.217.5.1273. [DOI] [PubMed] [Google Scholar]
- Hibberd M. G., Jewell B. R. Length-dependence of the sensitivity of the contractile system to calcium in rat ventricular muscle [proceedings]. J Physiol. 1979 May;290(2):30P–31P. [PubMed] [Google Scholar]
- Jewell B. R. A reexamination of the influence of muscle length on myocardial performance. Circ Res. 1977 Mar;40(3):221–230. doi: 10.1161/01.res.40.3.221. [DOI] [PubMed] [Google Scholar]
- KOCH-WESER J., BLINKS J. R. THE INFLUENCE OF THE INTERVAL BETWEEN BEATS ON MYOCARDIAL CONTRACTILITY. Pharmacol Rev. 1963 Sep;15:601–652. [PubMed] [Google Scholar]
- Katz A. M. Role of the contractile proteins and sarcoplasmic reticulum in the response of the heart to catecholamines: an historical review. Adv Cyclic Nucleotide Res. 1979;11:303–343. [PubMed] [Google Scholar]
- Katz G. M., Sorenson M. M., Reuben J. P. Filament interaction monitored by light scattering in skinned fibers. J Gen Physiol. 1978 Nov;72(5):651–665. doi: 10.1085/jgp.72.5.651. [DOI] [PMC free article] [PubMed] [Google Scholar]
- Krueger J. W., Pollack G. H. Myocardial sarcomere dynamics during isometric contraction. J Physiol. 1975 Oct;251(3):627–643. doi: 10.1113/jphysiol.1975.sp011112. [DOI] [PMC free article] [PubMed] [Google Scholar]
- LANGER G. A. CALCIUM EXCHANGE IN DOG VENTRICULAR MUSCLE: RELATION TO FREQUENCY OF CONTRACTION AND MAINTENANCE OF CONTRACTILITY. Circ Res. 1965 Jul;17:78–89. doi: 10.1161/01.res.17.1.78. [DOI] [PubMed] [Google Scholar]
- Lakatta E. G., Henderson A. H. Starling's Law reactivated. J Mol Cell Cardiol. 1977 May;9(5):347–351. doi: 10.1016/s0022-2828(77)80001-1. [DOI] [PubMed] [Google Scholar]
- Lakatta E. G., Spurgeon H. A. Force staircase kinetics in mammalian cardiac muscle: modulation by muscle length. J Physiol. 1980 Feb;299:337–352. doi: 10.1113/jphysiol.1980.sp013128. [DOI] [PMC free article] [PubMed] [Google Scholar]
- Lammel E., Niedergerke R., Page S. Analysis of a rapid twitch facilitation in the frog heart. Proc R Soc Lond B Biol Sci. 1975 Jun 17;189(1097):577–590. doi: 10.1098/rspb.1975.0073. [DOI] [PubMed] [Google Scholar]
- Langer G. A., Serena S. D., Nudd L. M. Cation exchange in heart cell culture: correlation with effects on contractile force. J Mol Cell Cardiol. 1974 Apr;6(2):149–161. doi: 10.1016/0022-2828(74)90018-2. [DOI] [PubMed] [Google Scholar]
- Langer G. A. The structure and function of the myocardial cell surface. Am J Physiol. 1978 Nov;235(5):H461–H468. doi: 10.1152/ajpheart.1978.235.5.H461. [DOI] [PubMed] [Google Scholar]
- Lappé D. L., Lakatta E. G. Intensity fluctuation spectroscopy monitors contractile activation in "resting" cardiac muscle. Science. 1980 Mar 21;207(4437):1369–1371. doi: 10.1126/science.7355295. [DOI] [PubMed] [Google Scholar]
- Loiselle D. S., Gibbs C. L. Species differences in cardiac energetics. Am J Physiol. 1979 Jul;237(1):H90–H98. doi: 10.1152/ajpheart.1979.237.1.H90. [DOI] [PubMed] [Google Scholar]
- Martin J. B. Twenty third annual Bowditch Lecture. Brain mechanisms for integration of growth hormones secretion. Physiologist. 1979 Feb;22(1):23–29. [PubMed] [Google Scholar]
- Matsubara I., Yagi N., Endoh M. Behaviour of myosin projections during the staircase phenomenon of heart muscle. Nature. 1978 May 4;273(5657):67–67. doi: 10.1038/273067a0. [DOI] [PubMed] [Google Scholar]
- Matsubara I., Yagi N., Endoh M. Movement of myosin heads during a heart beat. Nature. 1979 Mar 29;278(5703):474–476. doi: 10.1038/278474a0. [DOI] [PubMed] [Google Scholar]
- McClellan G. B., Winegrad S. The regulation of the calcium sensitivity of the contractile system in mammalian cardiac muscle. J Gen Physiol. 1978 Dec;72(6):737–764. doi: 10.1085/jgp.72.6.737. [DOI] [PMC free article] [PubMed] [Google Scholar]
- NIEDERGERKE R. The potassium chloride contracture of the heart and its modification by calcium. J Physiol. 1956 Dec 28;134(3):584–599. doi: 10.1113/jphysiol.1956.sp005667. [DOI] [PMC free article] [PubMed] [Google Scholar]
- NIEDERGERKE R. The staircase phenomenon and the action of calcium on the heart. J Physiol. 1956 Dec 28;134(3):569–583. doi: 10.1113/jphysiol.1956.sp005666. [DOI] [PMC free article] [PubMed] [Google Scholar]
- Niedergerke R., Orkand R. K. The dual effect of calcium on the action potential of the frog's heart. J Physiol. 1966 May;184(2):291–311. doi: 10.1113/jphysiol.1966.sp007916. [DOI] [PMC free article] [PubMed] [Google Scholar]
- Philipson K. D., Langer G. A. Sarcolemmal-bound calcium and contractility in the mammalian myocardium. J Mol Cell Cardiol. 1979 Sep;11(9):857–875. doi: 10.1016/0022-2828(79)90481-4. [DOI] [PubMed] [Google Scholar]
- Pollack G. H., Krueger J. W. Sarcomere dynamics in intact cardiac muscle. Eur J Cardiol. 1976 May;4 (Suppl):53–65. [PubMed] [Google Scholar]
- Potter J. D., Gergely J. The calcium and magnesium binding sites on troponin and their role in the regulation of myofibrillar adenosine triphosphatase. J Biol Chem. 1975 Jun 25;250(12):4628–4633. [PubMed] [Google Scholar]
- Sanborn W. G., Langer G. A. Specific uncoupling of excitation and contraction in mammalian cardiac tissue by lanthanum. J Gen Physiol. 1970 Aug;56(2):191–217. doi: 10.1085/jgp.56.2.191. [DOI] [PMC free article] [PubMed] [Google Scholar]
- Solaro R. J., Wise R. M., Shiner J. S., Briggs F. N. Calcium requirements for cardiac myofibrillar activation. Circ Res. 1974 Apr;34(4):525–530. doi: 10.1161/01.res.34.4.525. [DOI] [PubMed] [Google Scholar]
- Spear J. F., Moore E. N. A comparison of alternation in myocardial action potentials and contractility. Am J Physiol. 1971 Jun;220(6):1708–1716. doi: 10.1152/ajplegacy.1971.220.6.1708. [DOI] [PubMed] [Google Scholar]
- Sutko J. L., Willerson J. T. Ryanodine alteration of the contractile state of rat ventricular myocardium. Comparison with dog, cat, and rabbit ventricular tissues. Circ Res. 1980 Mar;46(3):332–343. doi: 10.1161/01.res.46.3.332. [DOI] [PubMed] [Google Scholar]
- Wood E. H., Heppner R. L., Weidmann S. Inotropic effects of electric currents. I. Positive and negative effects of constant electric currents or current pulses applied during cardiac action potentials. II. Hypotheses: calcium movements, excitation-contraction coupling and inotropic effects. Circ Res. 1969 Mar;24(3):409–445. doi: 10.1161/01.res.24.3.409. [DOI] [PubMed] [Google Scholar]