Abstract
1. The sensation of stereoscopic depth rests on the central neural processing of signals evoked by the two retinal images of a single object in space. It was our purpose in this study to investigate in the behaving monkey the binocular cortical mechanisms that might underlie the ability to recognize the relative position and motion of objects in three-dimensional space.
2. The large majority of neurones studied in A17 (n = 245), and all neurones studied in A18 (n = 21), were functionally connected to both eyes, and a substantial proportion (75%) of these neurones were sensitive to positional binocular disparity. On the basis of their depth sensitivity profile, four types of stereoscopic neurones were recognized, each type characteristically sensitive to visual contours appearing in depth farther than, at, or nearer than the point of binocular fixation.
3. Tuned excitatory and tuned inhibitory neurones display binocular facilitation and binocular suppression respectively, to stimuli over a narrow range of small disparities, including zero disparity, with more or less pronounced reciprocal responses to stimuli with larger disparities. These neurones, the tuned excitatory in particular, may be considered to be the substrate for central fusion of slightly disparate retinal images, and to provide the basis for the neural mechanisms leading to three-dimensional perception of objects with high stereoacuity (fine stereopsis).
4. Two other sets of reciprocally organized neurones, near and far neurones, respond differentially to wider ranges of crossed and uncrossed disparities. The near neurones are activated by stimuli in front of and inhibited by stimuli behind fixation. The far neurones have the reciprocal depth sensitivity. These neural elements may be regarded as active in the processing of binocular information leading to qualitative depth estimates in the presence of double vision (coarse stereopsis).
5. Binocular response selectivity for the direction of object motion-in-depth depends chiefly upon monocular sensitivity to the direction of retinal image motion, a property we observed in about one half of the foveal neurones. Cortical neurones with the same directional sensitivity for monocular stimuli in both eyes display coarse binocular selectivity for the trajectory of object motion but provide unambiguous signals for the direction of motion, towards the right or towards the left within the depth domain of the neurone. A small group of neurones (3%) displays opposite and opponent directional sensitivity for stimuli in the two eyes. Their binocular response, therefore, is best when the two retinal images move in opposite directions at the same time, a condition that obtains with motion directly towards or away from the animal with little or no lateral movement. These directionally dual-opponent cells usually have coarse or no selectivity for position-in-depth.
6. The results of this study indicate that basic mechanisms for the stereoscopic analysis of the position (static) and motion (dynamic) of objects in space relative to one another are present at early stages of binocular interaction in the visual cortex of primates, and that they are in effective action during normal binocular vision.
Full text
PDF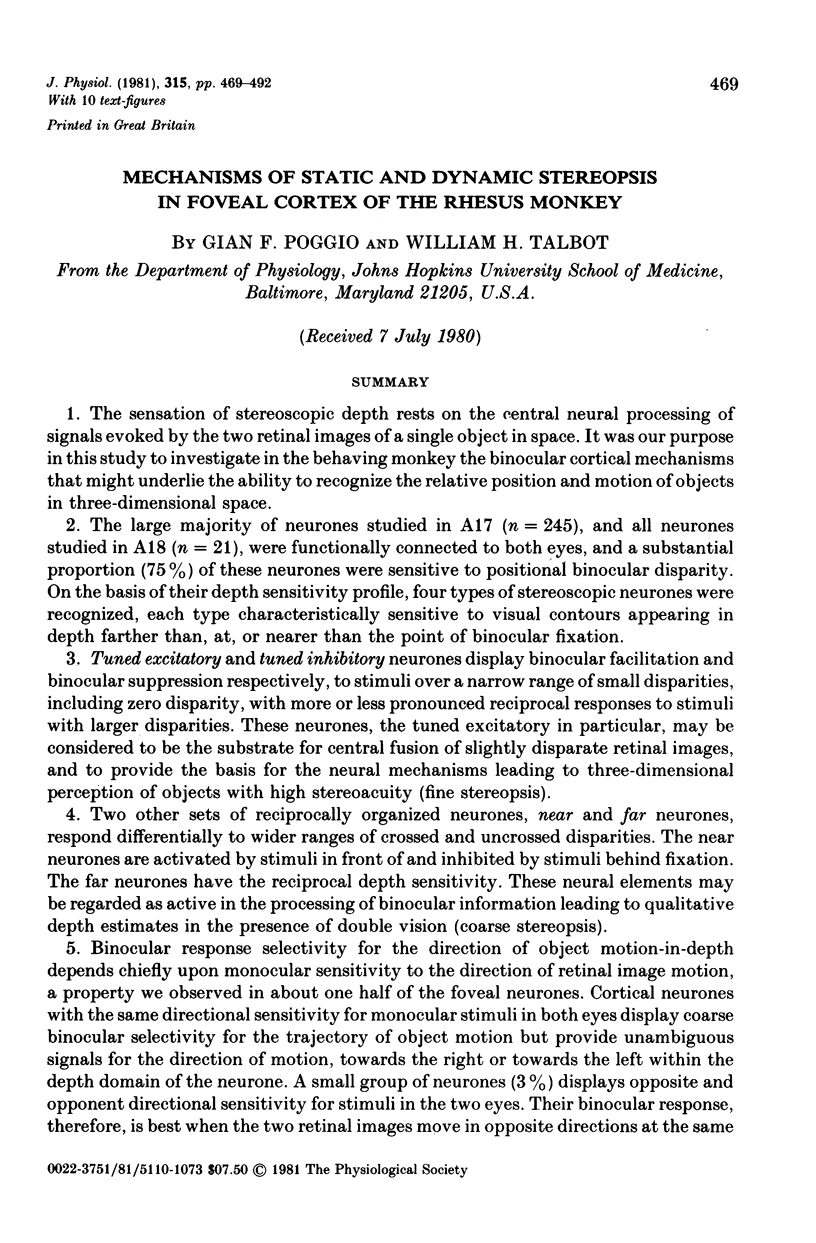
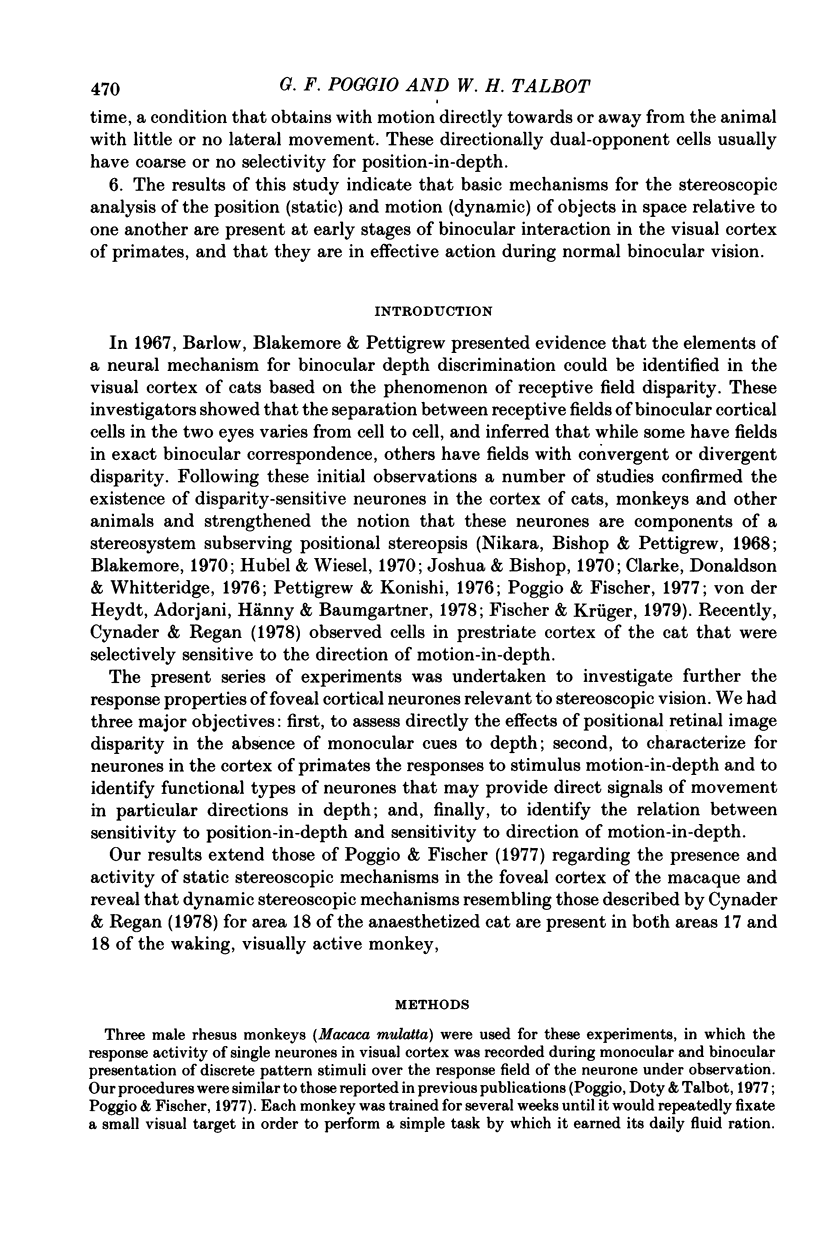
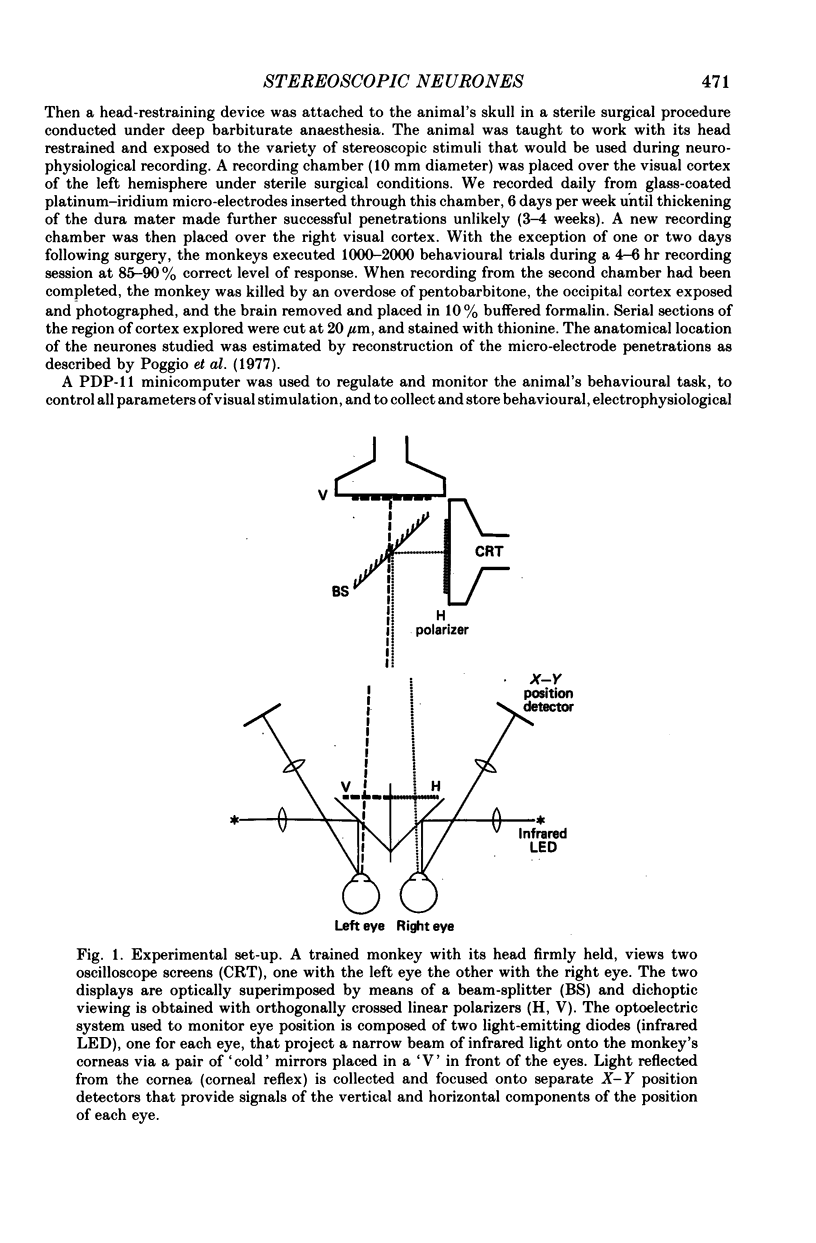
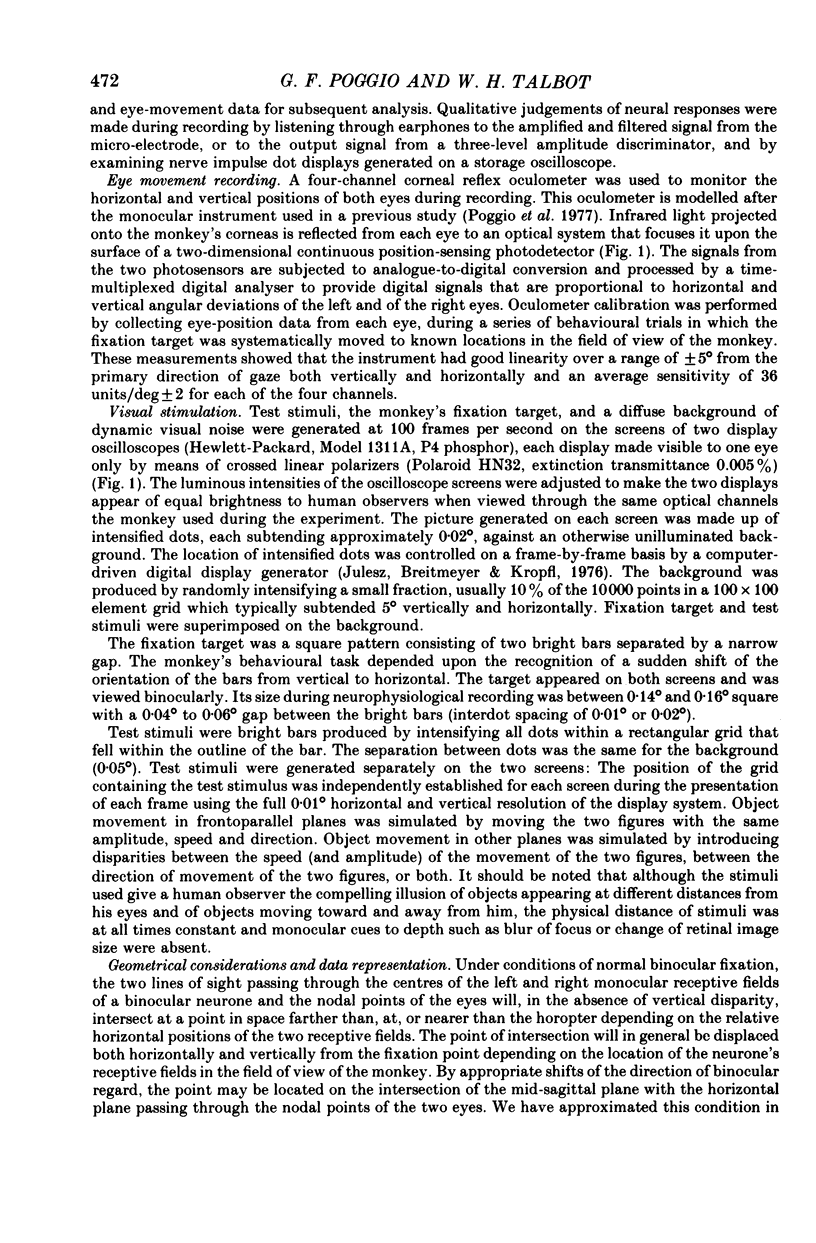
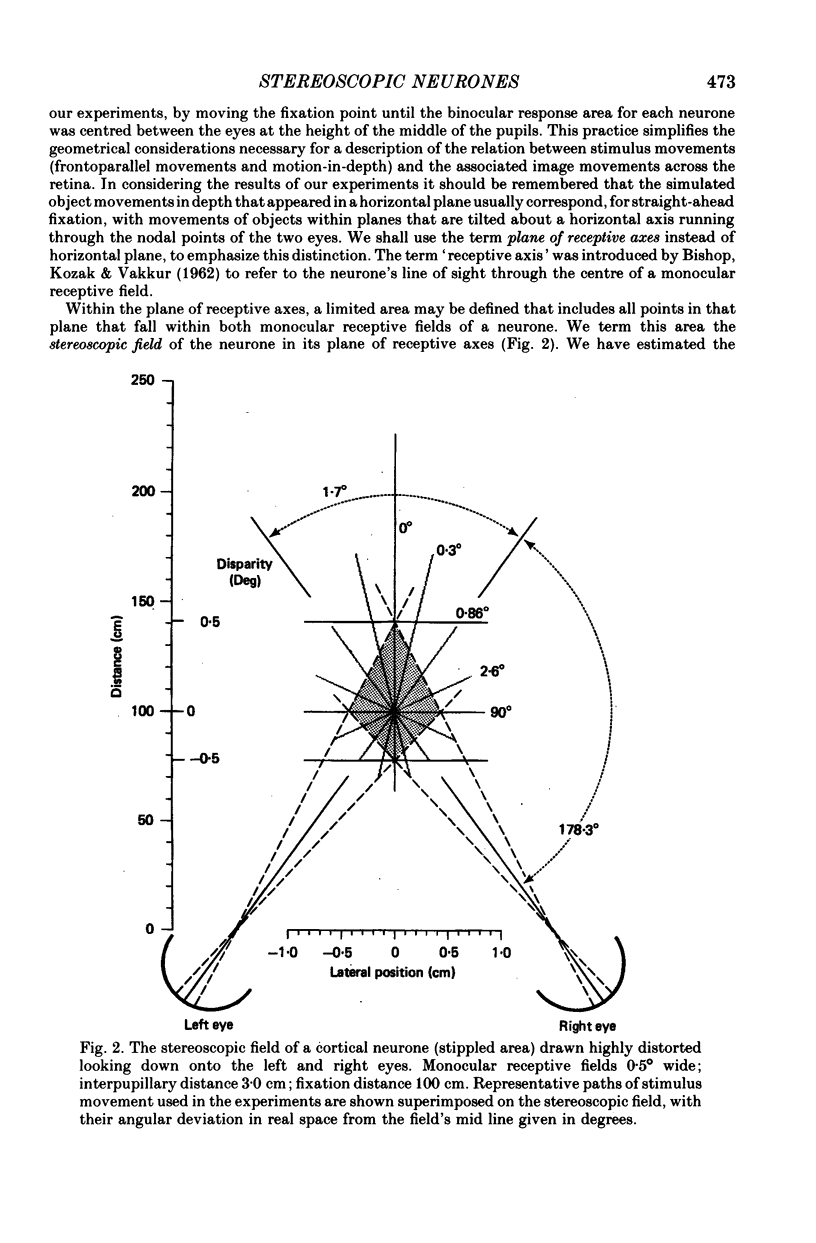
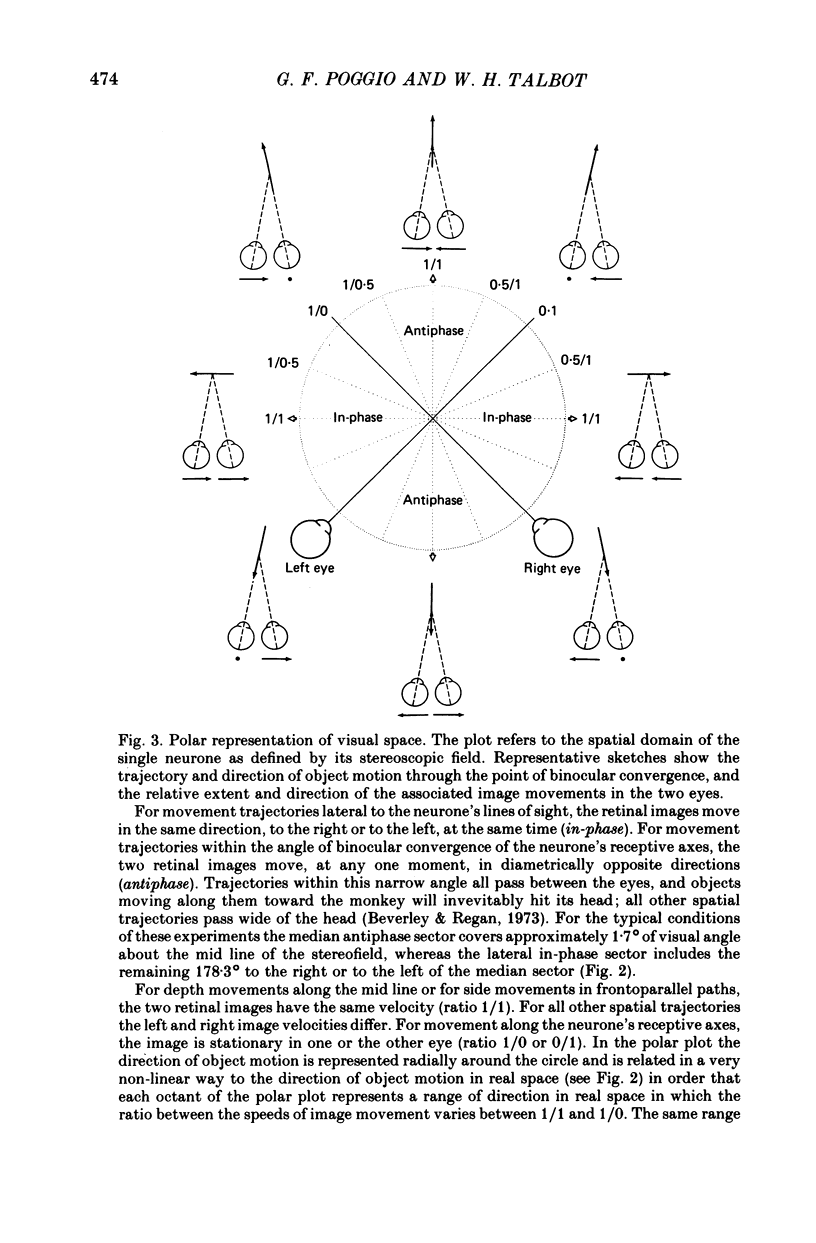
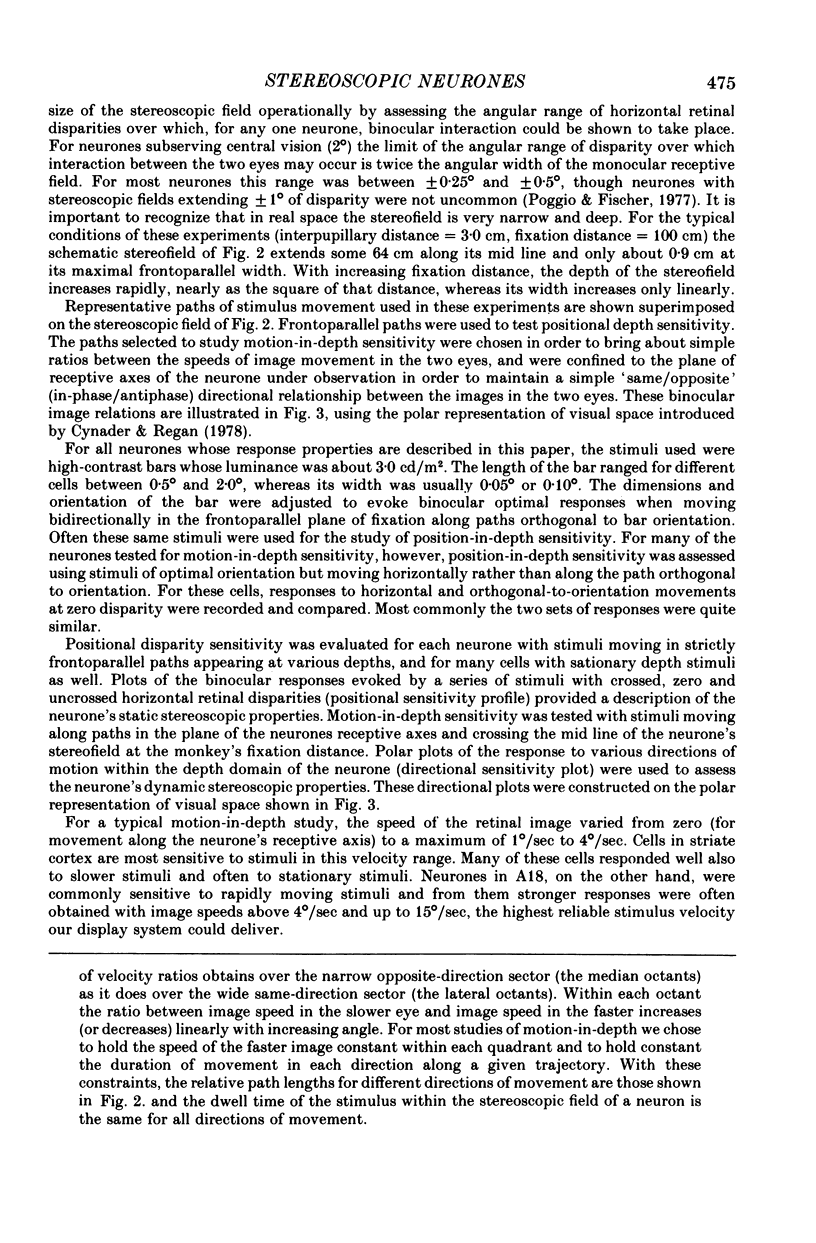
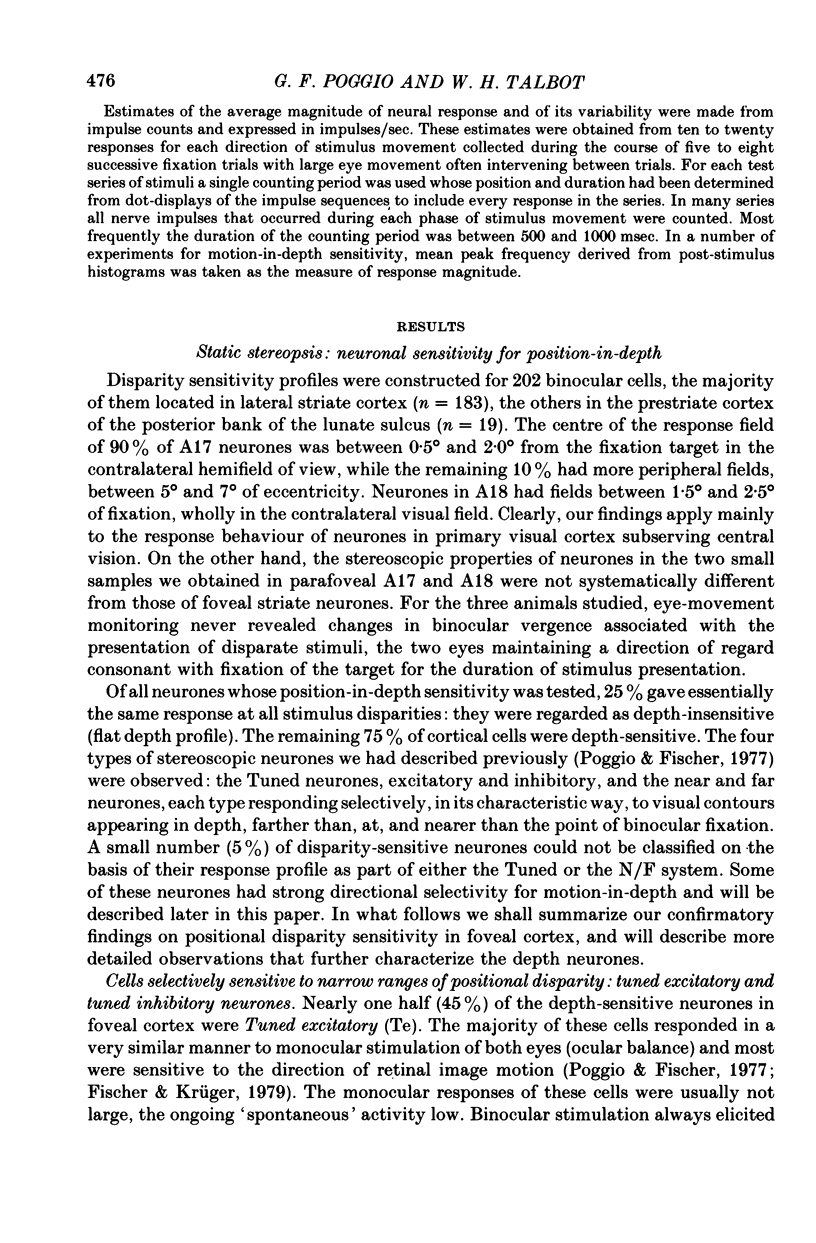
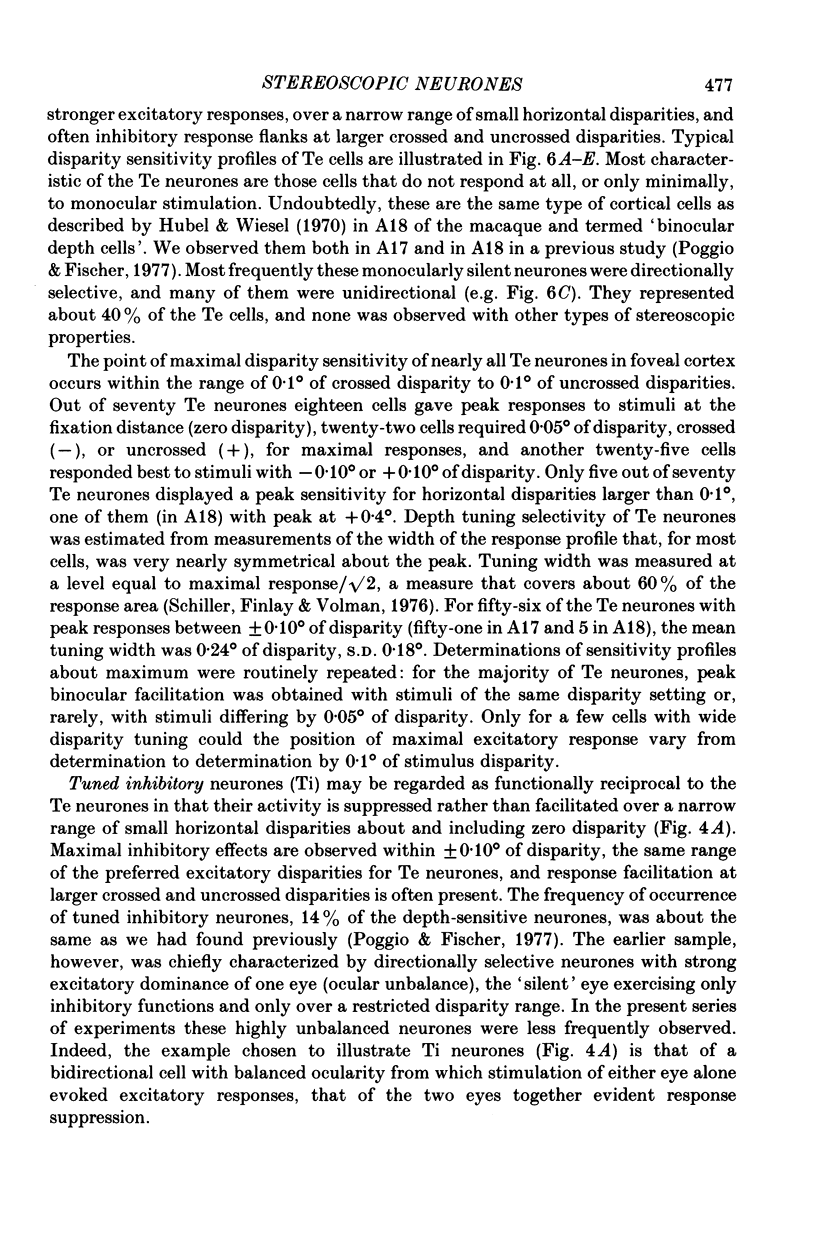
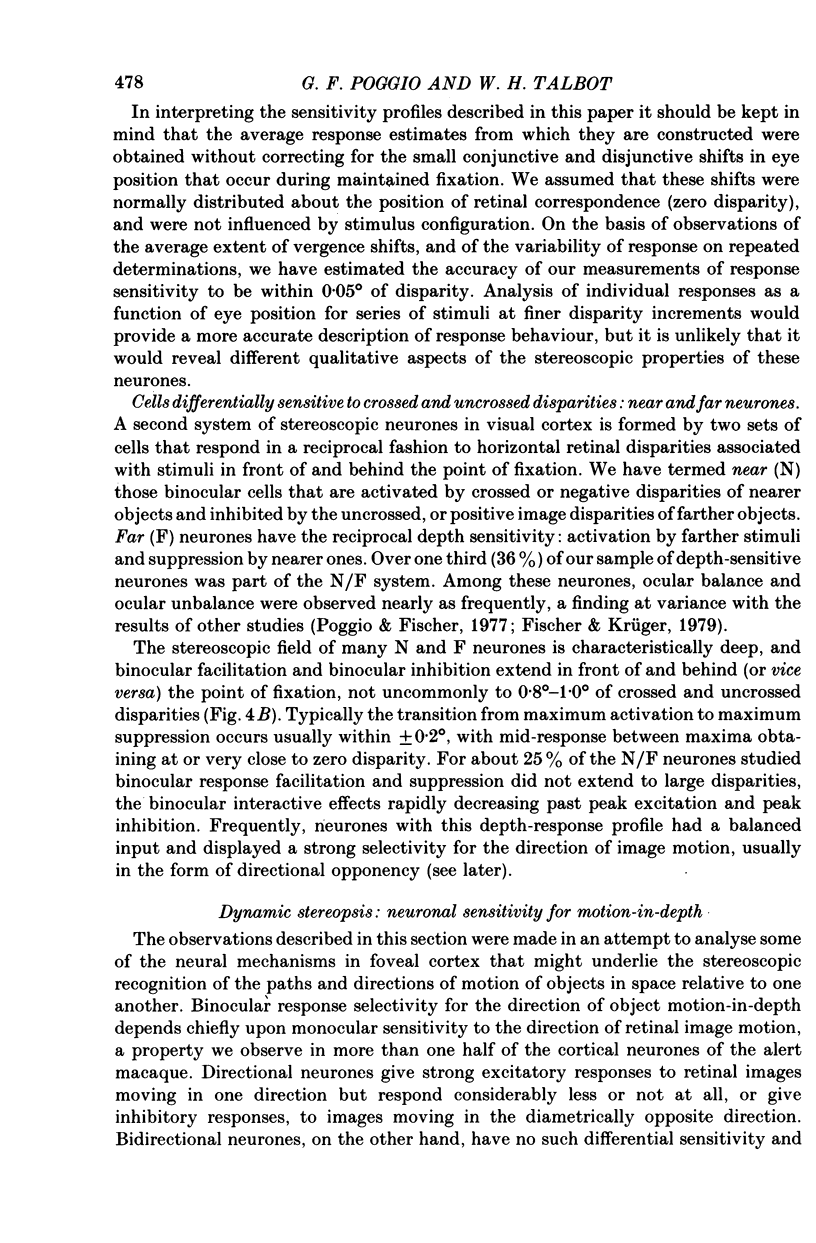
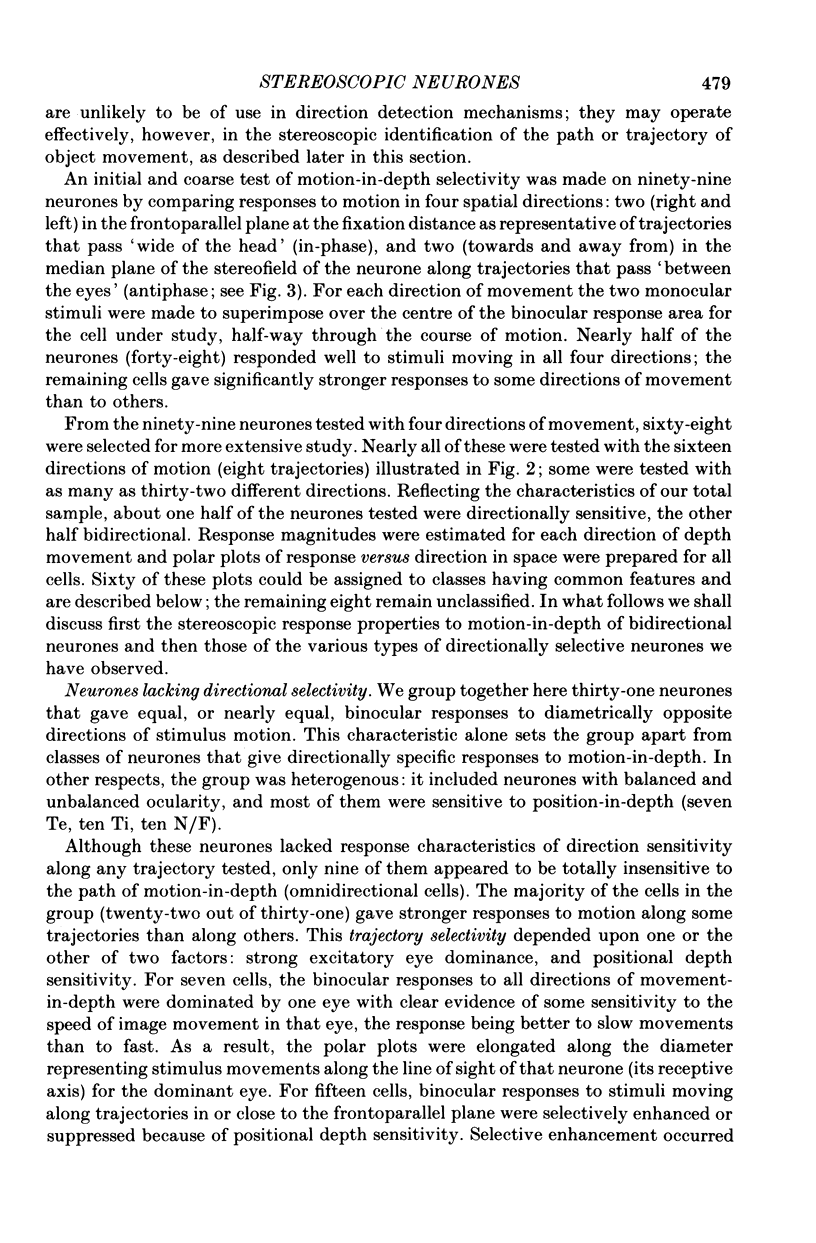
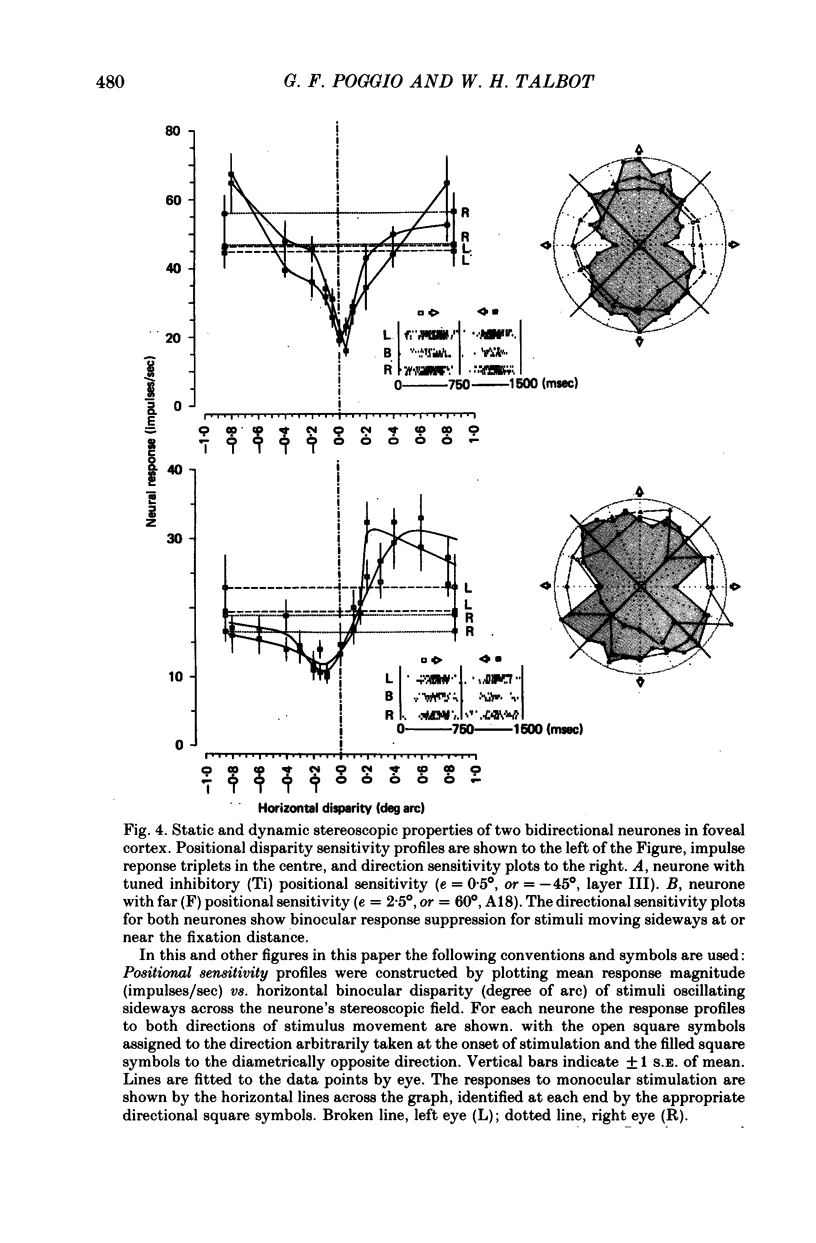
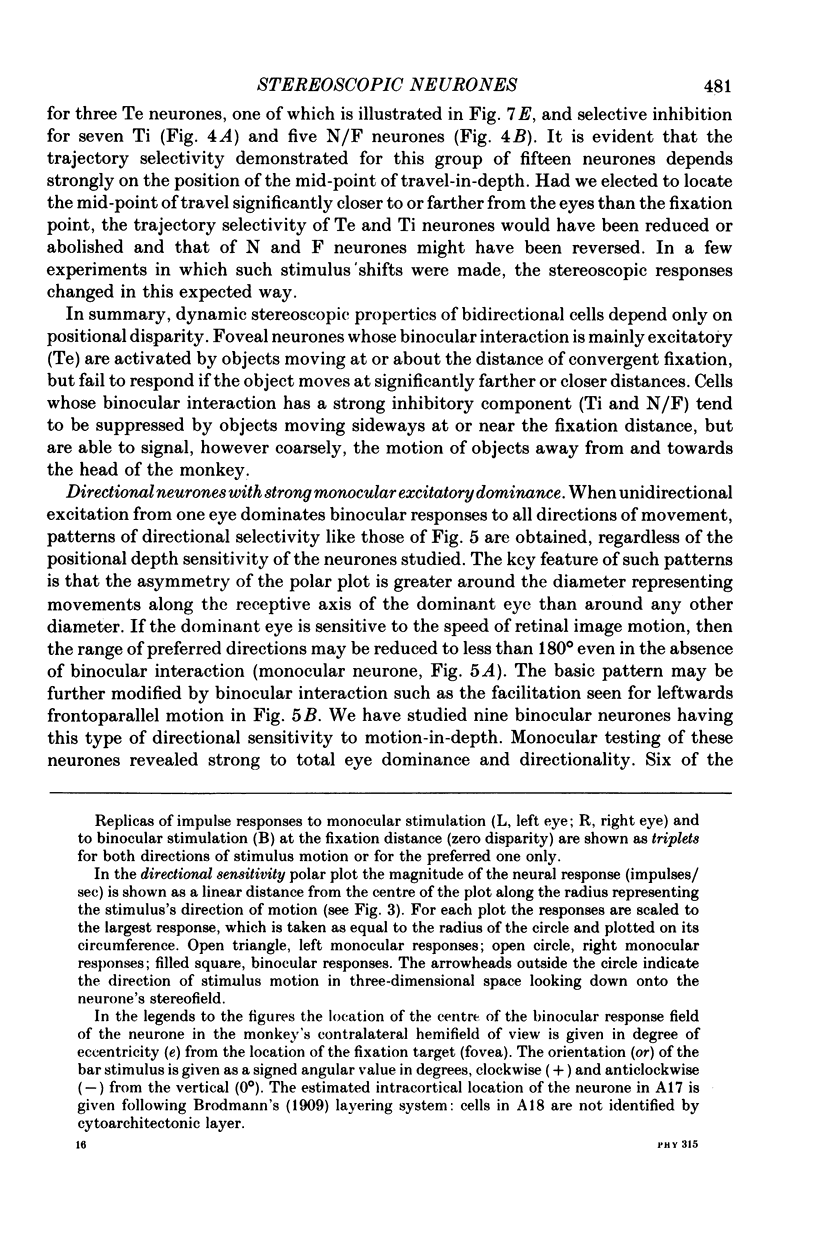
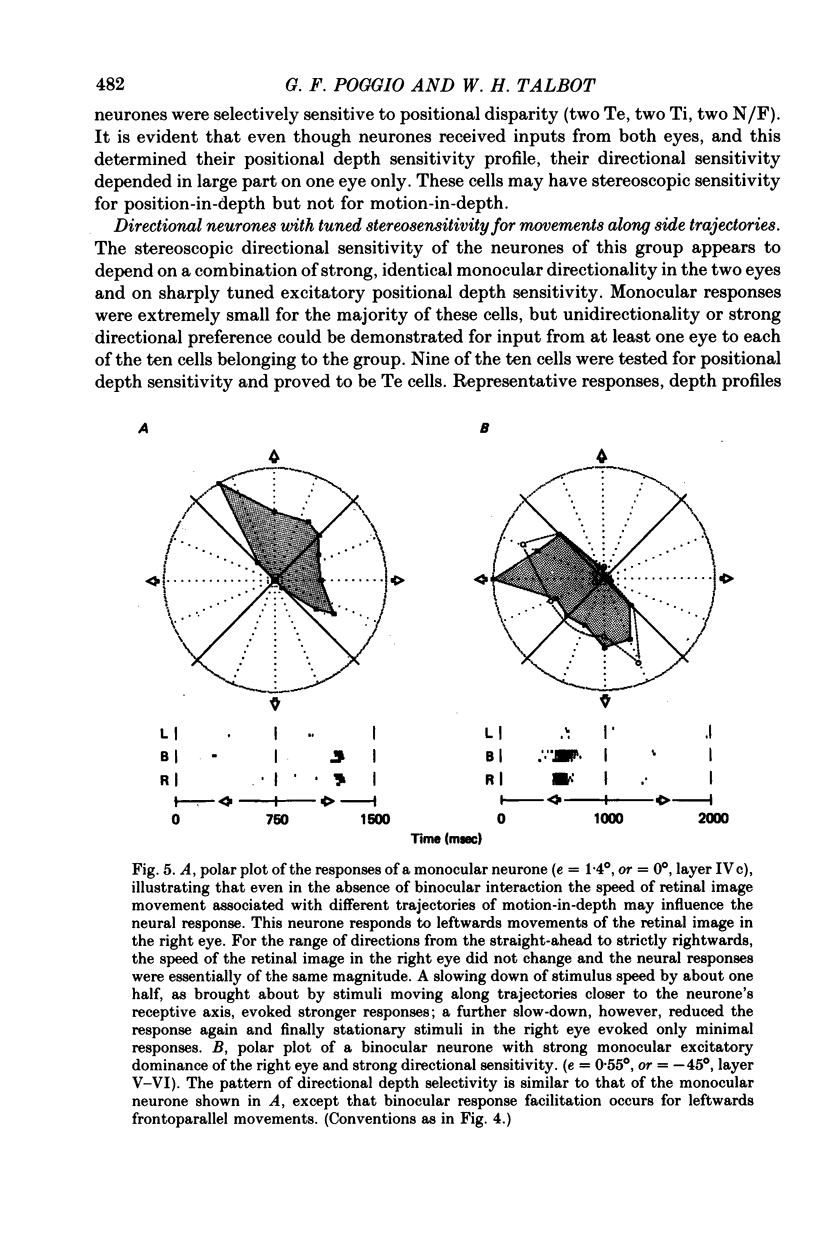
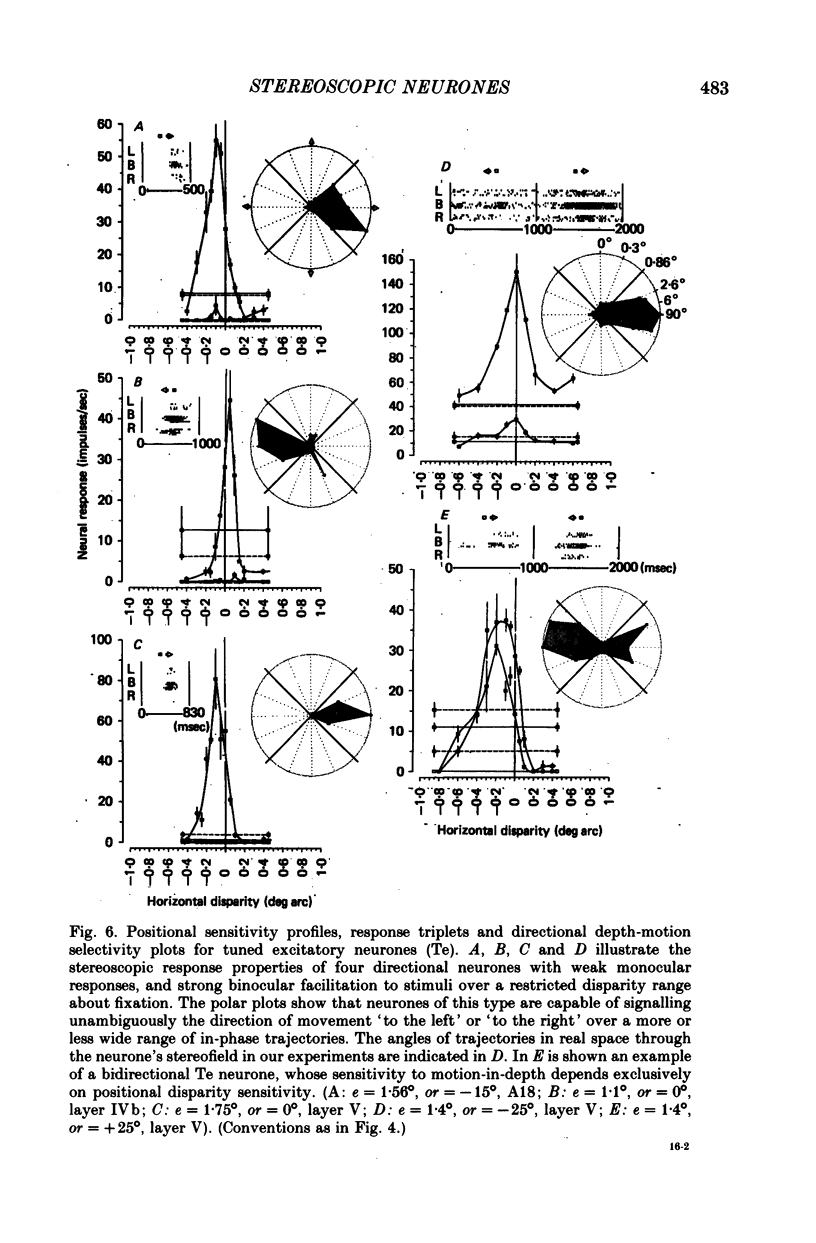
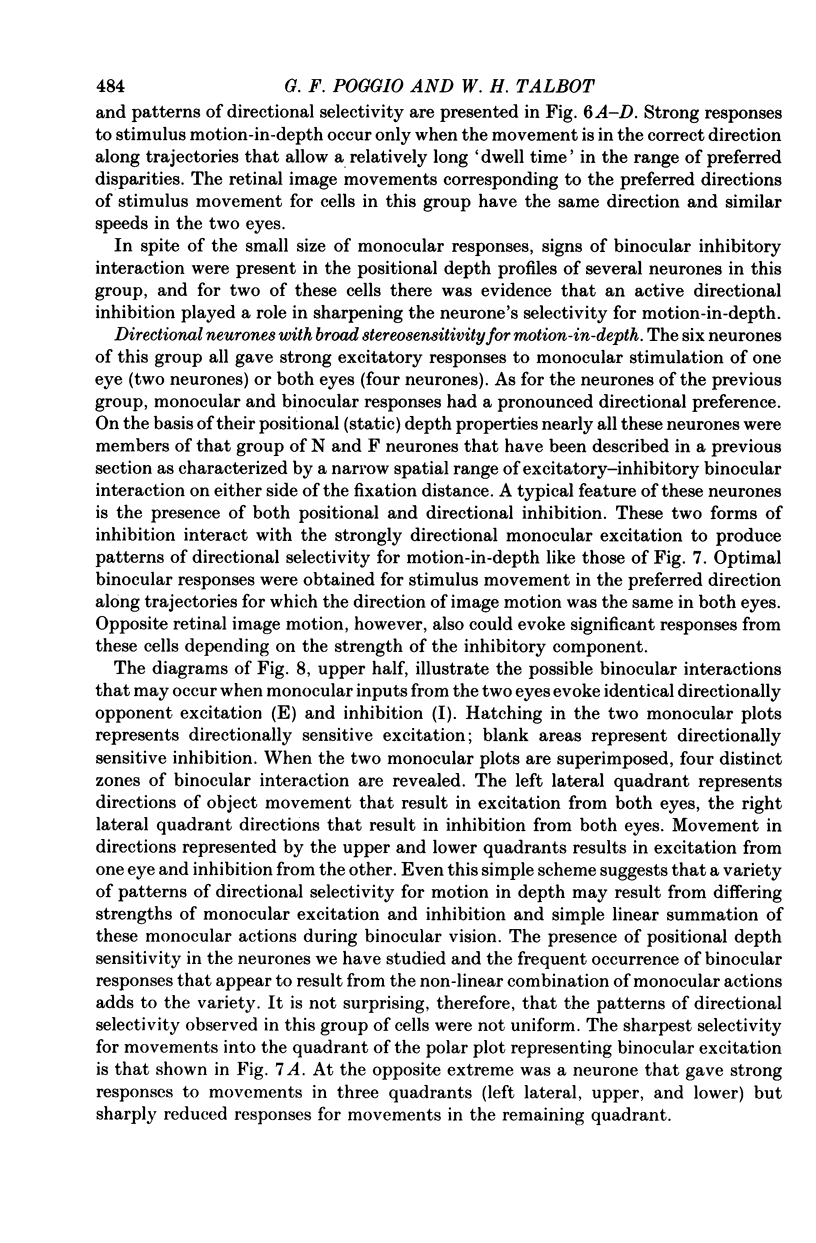
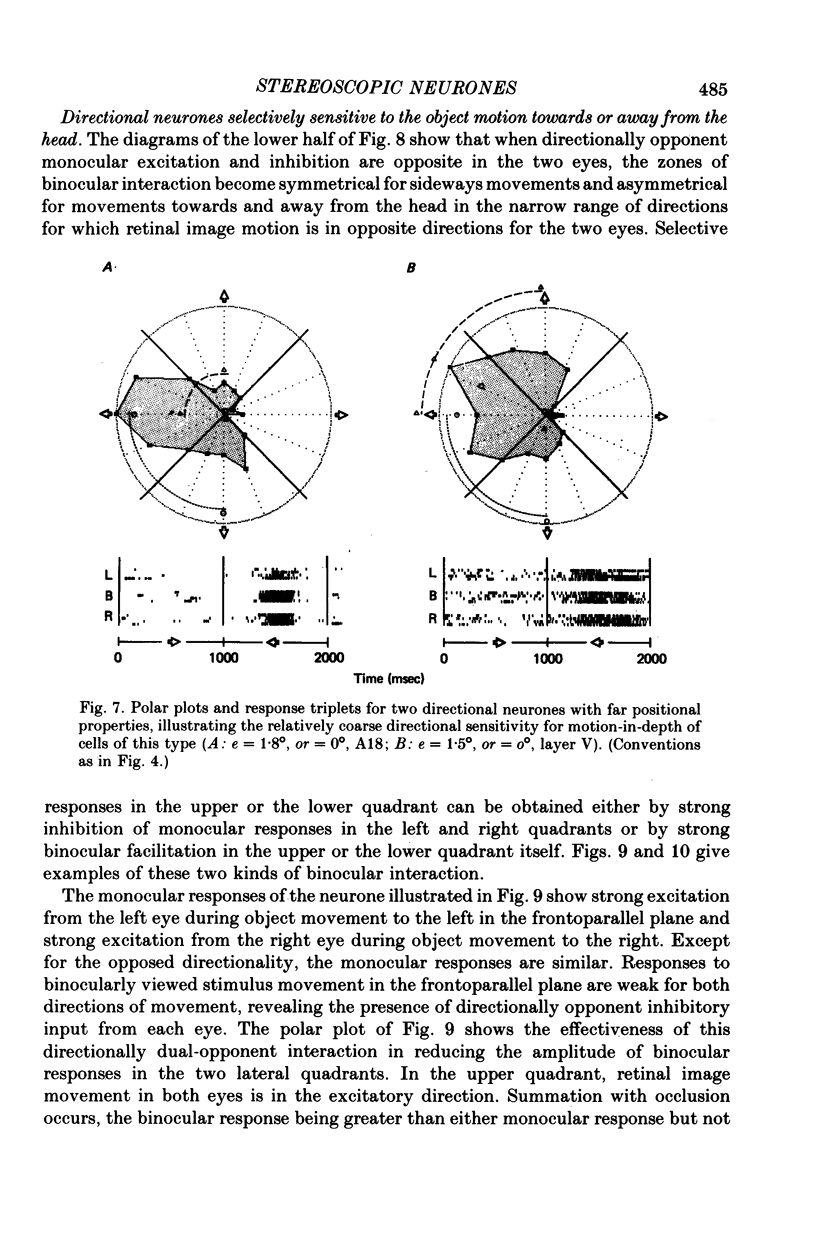
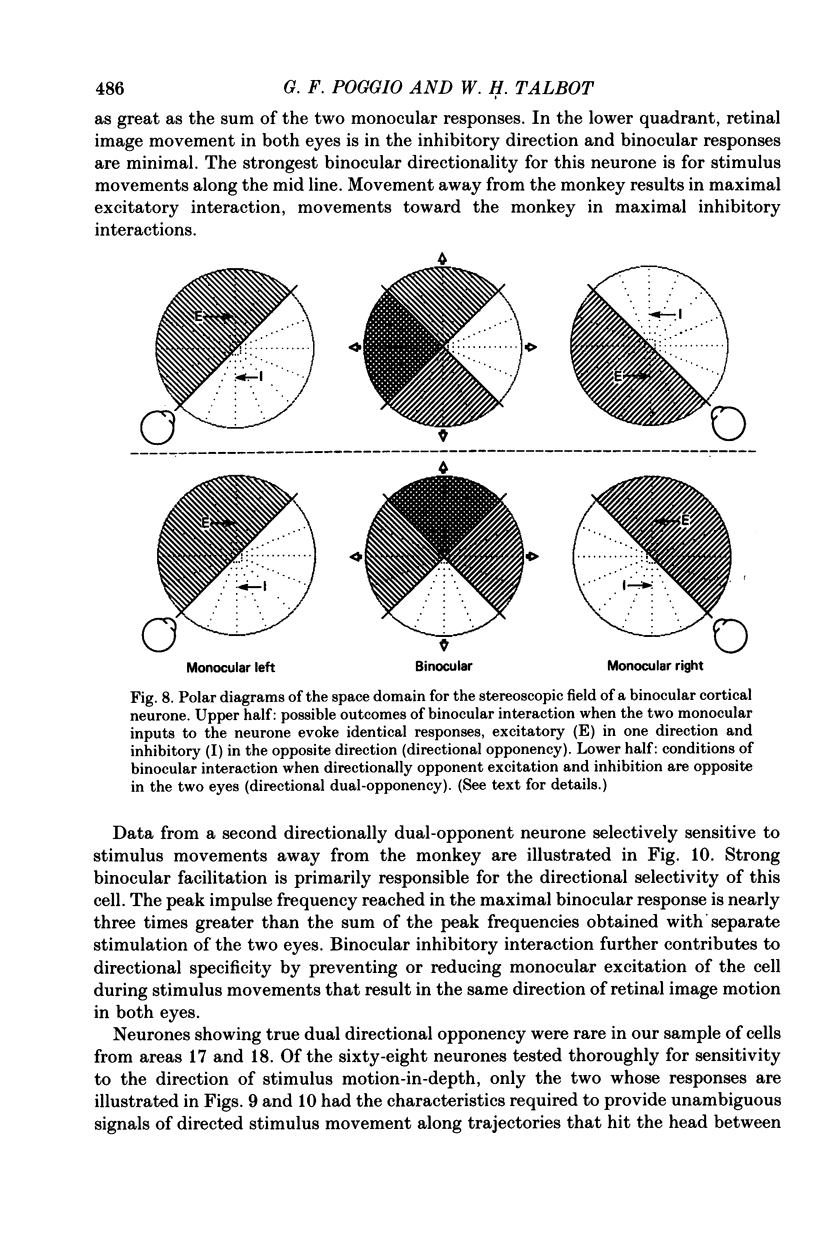
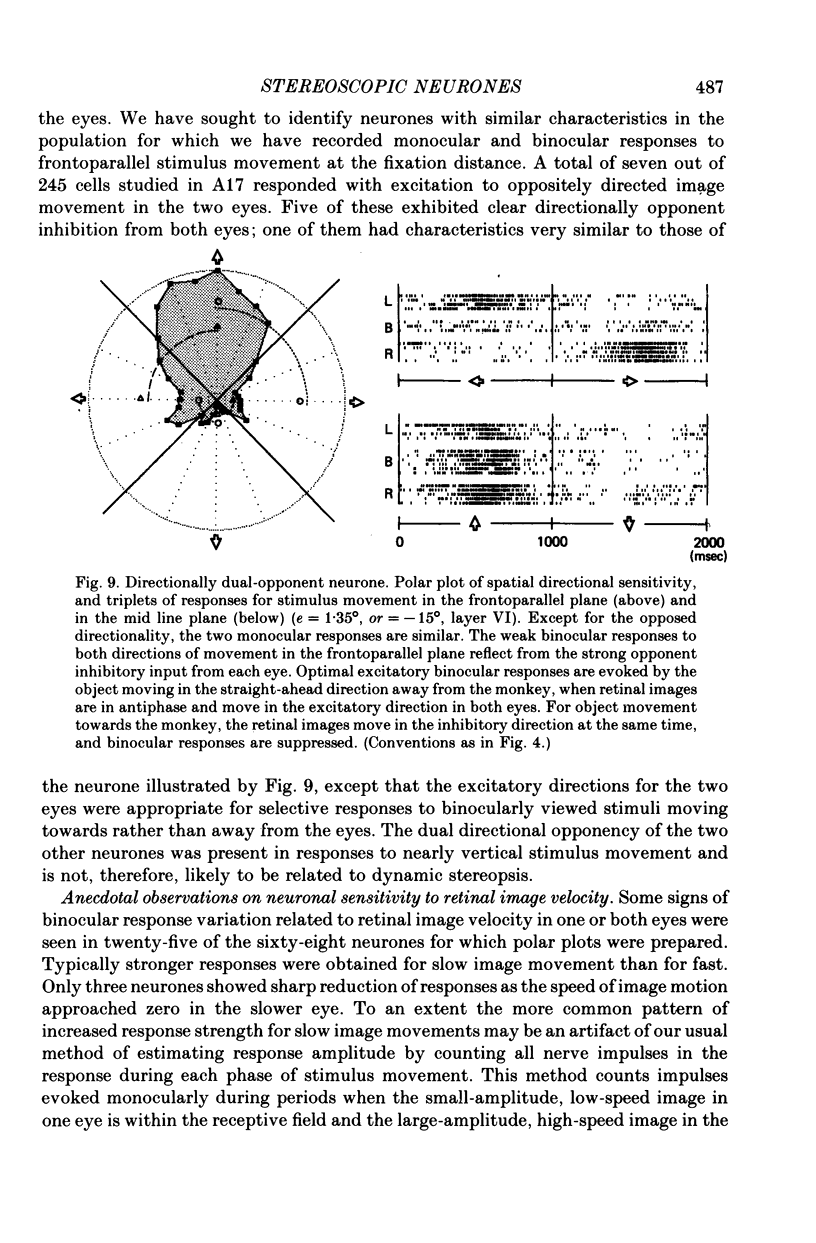
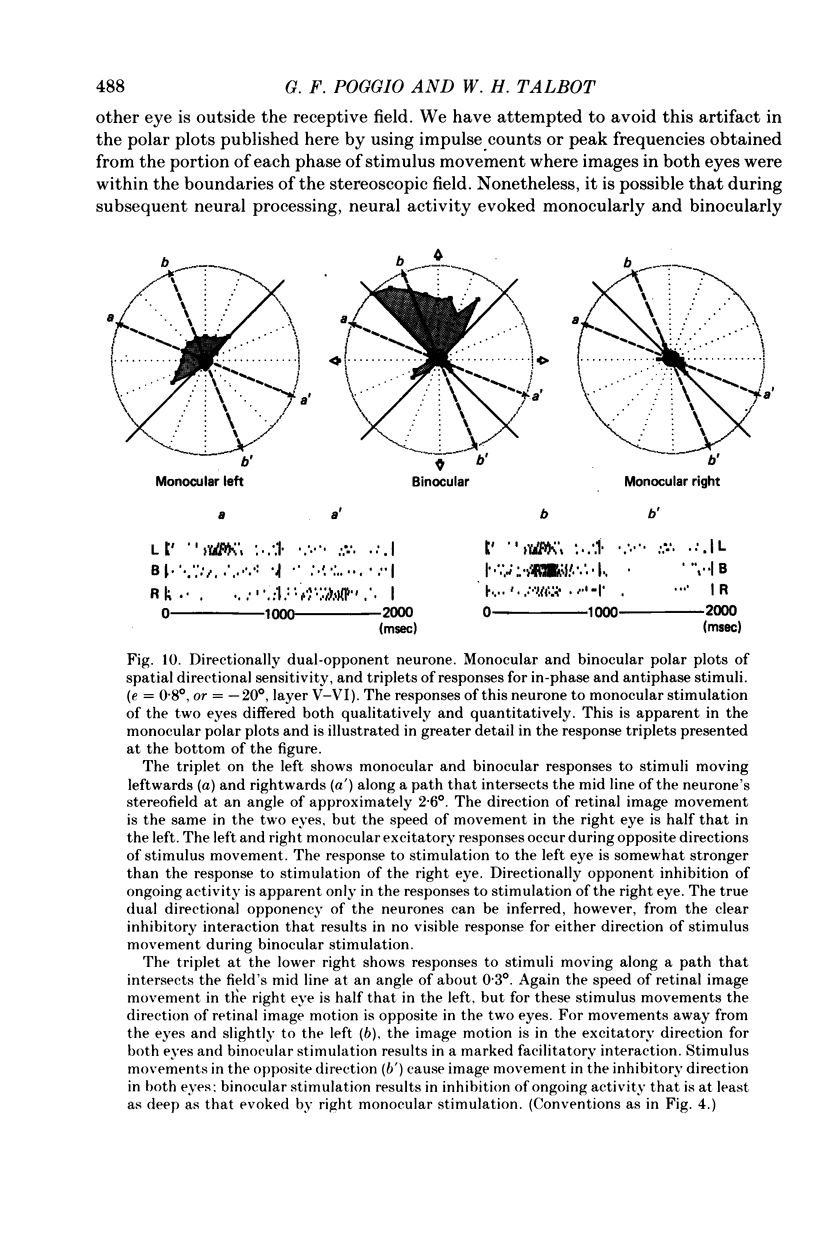
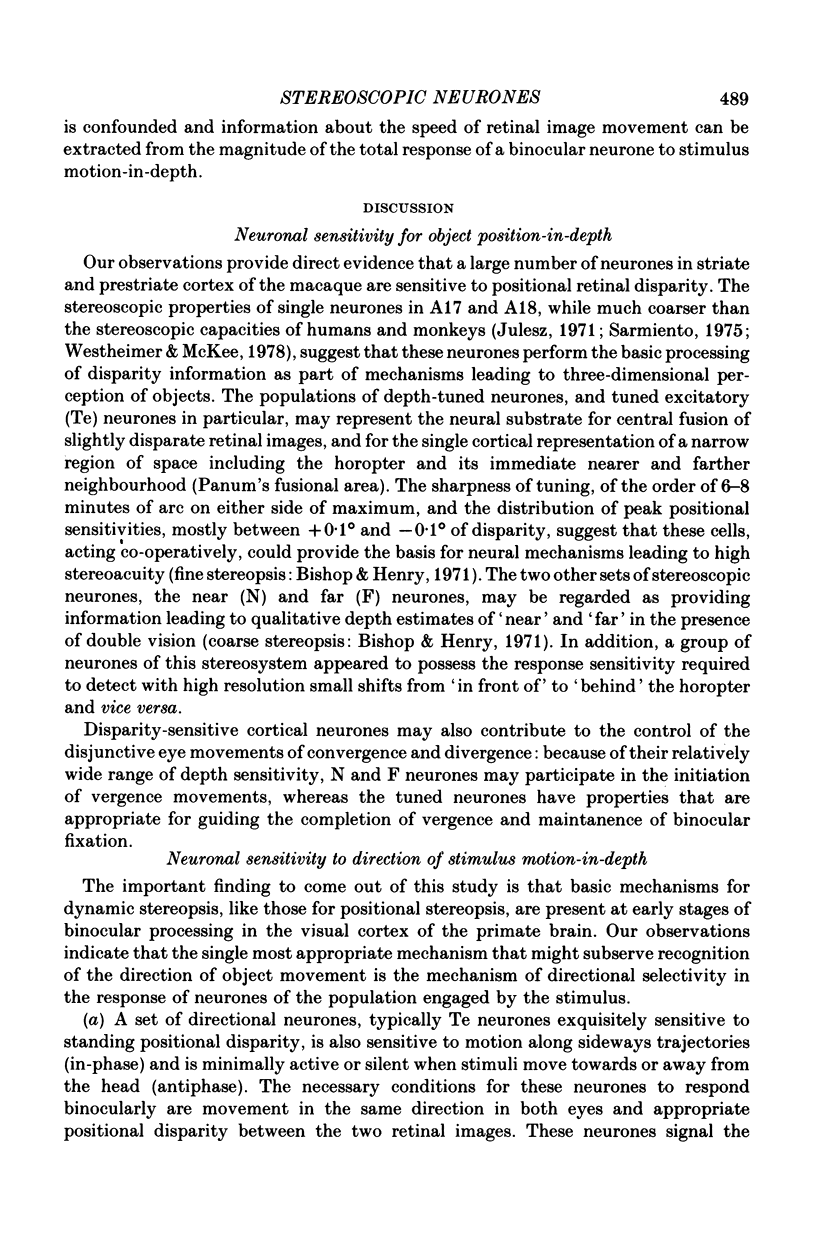
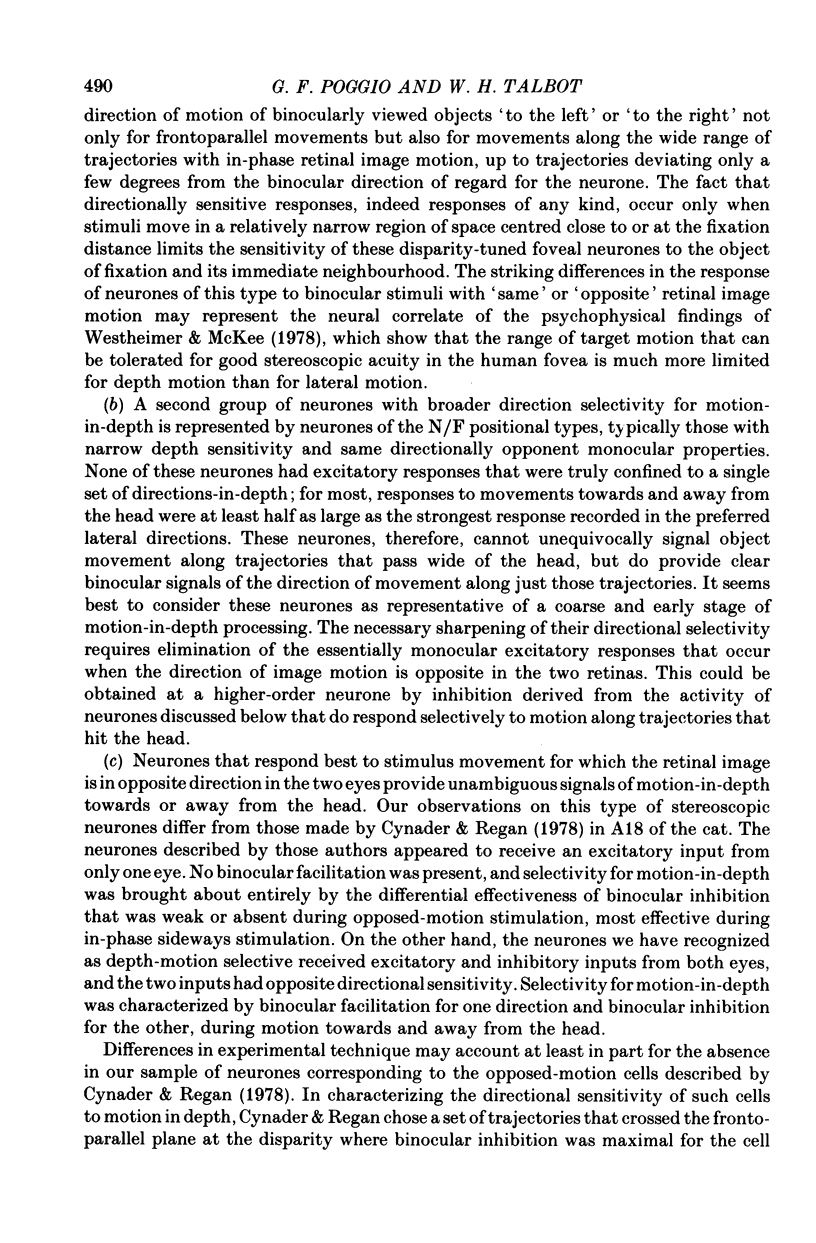
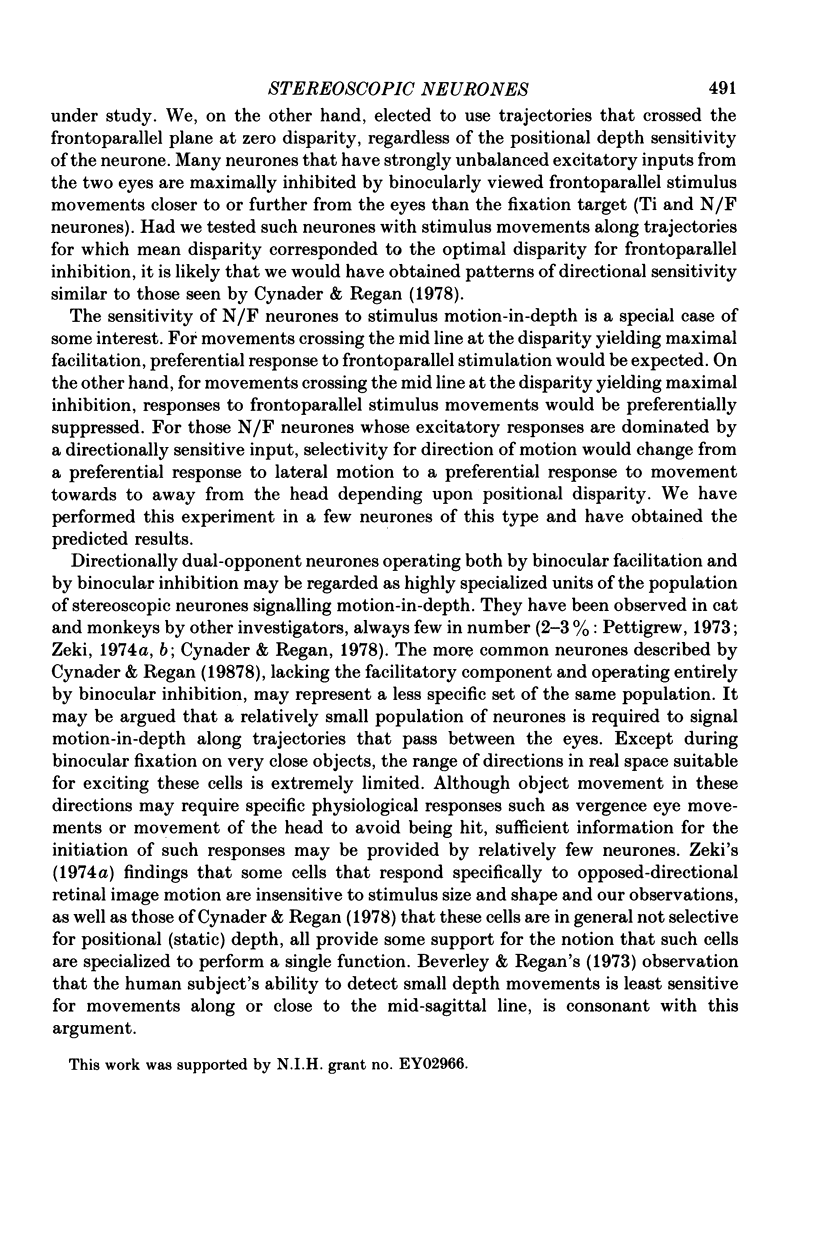
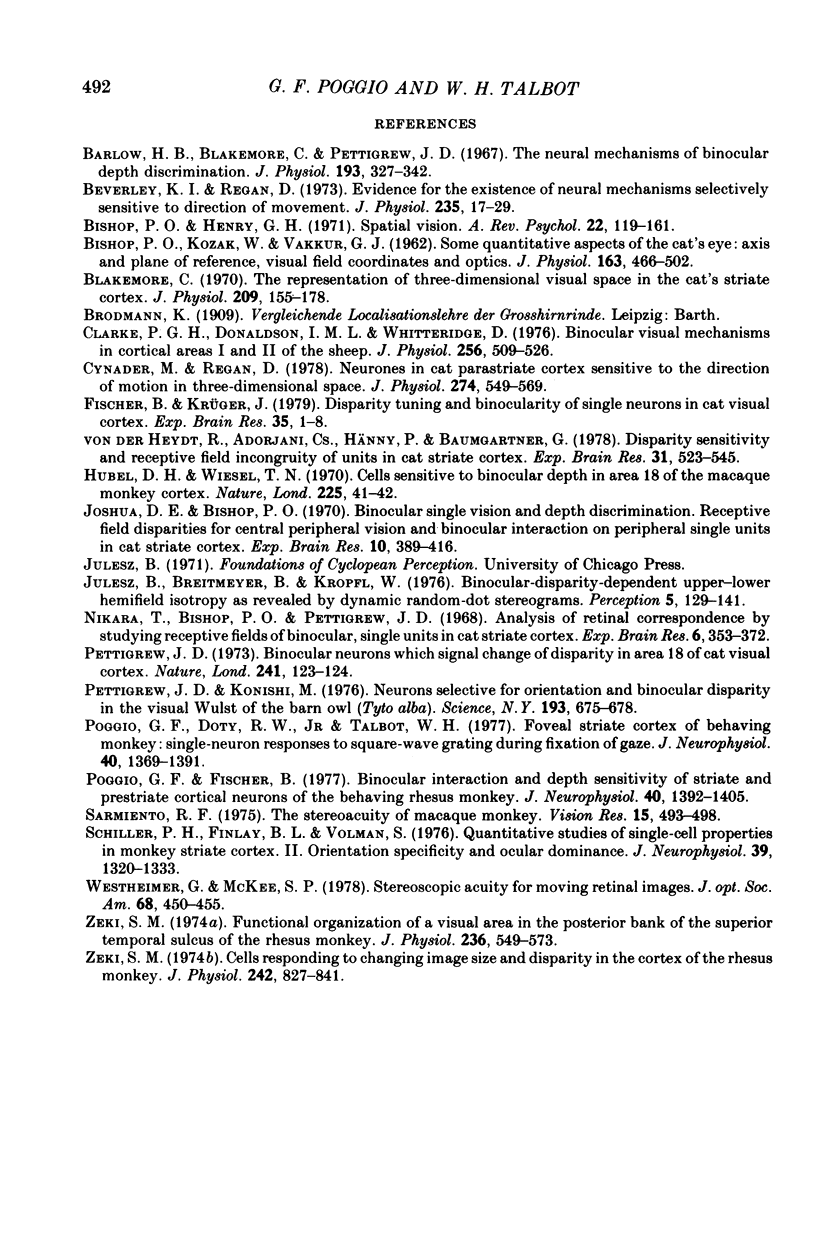
Images in this article
Selected References
These references are in PubMed. This may not be the complete list of references from this article.
- BISHOP P. O., KOZAK W., VAKKUR G. J. Some quantitative aspects of the cat's eye: axis and plane of reference, visual field co-ordinates and optics. J Physiol. 1962 Oct;163:466–502. doi: 10.1113/jphysiol.1962.sp006990. [DOI] [PMC free article] [PubMed] [Google Scholar]
- Barlow H. B., Blakemore C., Pettigrew J. D. The neural mechanism of binocular depth discrimination. J Physiol. 1967 Nov;193(2):327–342. doi: 10.1113/jphysiol.1967.sp008360. [DOI] [PMC free article] [PubMed] [Google Scholar]
- Beverley K. I., Regan D. Evidence for the existence of neural mechanisms selectively sensitive to the direction of movement in space. J Physiol. 1973 Nov;235(1):17–29. doi: 10.1113/jphysiol.1973.sp010376. [DOI] [PMC free article] [PubMed] [Google Scholar]
- Bishop P. O., Henry G. H. Spatial vision. Annu Rev Psychol. 1971;22:119–160. doi: 10.1146/annurev.ps.22.020171.001003. [DOI] [PubMed] [Google Scholar]
- Blakemore C. The representation of three-dimensional visual space in the cat's striate cortex. J Physiol. 1970 Jul;209(1):155–178. doi: 10.1113/jphysiol.1970.sp009160. [DOI] [PMC free article] [PubMed] [Google Scholar]
- Clarke P. G., Donaldson I. M., Whitteridge D. Binocular visual mechanisms in cortical areas I and II of the sheep. J Physiol. 1976 Apr;256(3):509–526. doi: 10.1113/jphysiol.1976.sp011336. [DOI] [PMC free article] [PubMed] [Google Scholar]
- Cynader M., Regan D. Neurones in cat parastriate cortex sensitive to the direction of motion in three-dimensional space. J Physiol. 1978 Jan;274:549–569. doi: 10.1113/jphysiol.1978.sp012166. [DOI] [PMC free article] [PubMed] [Google Scholar]
- Fischer B., Krüger J. Disparity tuning and binocularity of single neurons in cat visual cortex. Exp Brain Res. 1979 Mar 9;35(1):1–8. doi: 10.1007/BF00236780. [DOI] [PubMed] [Google Scholar]
- Hubel D. H., Wiesel T. N. Stereoscopic vision in macaque monkey. Cells sensitive to binocular depth in area 18 of the macaque monkey cortex. Nature. 1970 Jan 3;225(5227):41–42. doi: 10.1038/225041a0. [DOI] [PubMed] [Google Scholar]
- Joshua D. E., Bishop P. O. Binocular single vision and depth discrimination. Receptive field disparities for central and peripheral vision and binocular interaction on peripheral single units in cat striate cortex. Exp Brain Res. 1970;10(4):389–416. doi: 10.1007/BF02324766. [DOI] [PubMed] [Google Scholar]
- Julesz B., Breitmeyer B., Kropfi W. Binocular-disparity-dependent upper-lower hemifield anisotropy and left-right hemifield isotropy as revealed by dynamic random-dot stereograms. Perception. 1976;5(2):129–141. doi: 10.1068/p050129. [DOI] [PubMed] [Google Scholar]
- Nikara T., Bishop P. O., Pettigrew J. D. Analysis of retinal correspondence by studying receptive fields of binocular single units in cat striate cortex. Exp Brain Res. 1968;6(4):353–372. doi: 10.1007/BF00233184. [DOI] [PubMed] [Google Scholar]
- Pettigrew J. D. Binocular neurons which signal change of disparity in area 18 of cat visual cortex. Nat New Biol. 1973 Jan 24;241(108):123–124. doi: 10.1038/newbio241123a0. [DOI] [PubMed] [Google Scholar]
- Pettigrew J. D., Konishi M. Neurons selective for orientation and binocular disparity in the visual Wulst of the barn owl (Tyto alba). Science. 1976 Aug 20;193(4254):675–678. doi: 10.1126/science.948741. [DOI] [PubMed] [Google Scholar]
- Poggio G. F., Doty R. W., Jr, Talbot W. H. Foveal striate cortex of behaving monkey: single-neuron responses to square-wave gratings during fixation of gaze. J Neurophysiol. 1977 Nov;40(6):1369–1391. doi: 10.1152/jn.1977.40.6.1369. [DOI] [PubMed] [Google Scholar]
- Poggio G. F., Fischer B. Binocular interaction and depth sensitivity in striate and prestriate cortex of behaving rhesus monkey. J Neurophysiol. 1977 Nov;40(6):1392–1405. doi: 10.1152/jn.1977.40.6.1392. [DOI] [PubMed] [Google Scholar]
- Sarmiento R. F. The stereoacuity of macaque monkey. Vision Res. 1975 Apr;15(4):493–498. doi: 10.1016/0042-6989(75)90026-7. [DOI] [PubMed] [Google Scholar]
- Schiller P. H., Finlay B. L., Volman S. F. Quantitative studies of single-cell properties in monkey striate cortex. II. Orientation specificity and ocular dominance. J Neurophysiol. 1976 Nov;39(6):1320–1333. doi: 10.1152/jn.1976.39.6.1320. [DOI] [PubMed] [Google Scholar]
- Westheimer G., McKee S. P. Steroscopic acuity for moving retinal images. J Opt Soc Am. 1978 Apr;68(4):450–455. doi: 10.1364/josa.68.000450. [DOI] [PubMed] [Google Scholar]
- Zeki S. M. Cells responding to changing image size and disparity in the cortex of the rhesus monkey. J Physiol. 1974 Nov;242(3):827–841. doi: 10.1113/jphysiol.1974.sp010736. [DOI] [PMC free article] [PubMed] [Google Scholar]
- Zeki S. M. Functional organization of a visual area in the posterior bank of the superior temporal sulcus of the rhesus monkey. J Physiol. 1974 Feb;236(3):549–573. doi: 10.1113/jphysiol.1974.sp010452. [DOI] [PMC free article] [PubMed] [Google Scholar]
- von der Heydt R., Adorjani C., Hänny P., Baumgartner G. Disparity sensitivity and receptive field incongruity of units in the cat striate cortex. Exp Brain Res. 1978 Apr 14;31(4):523–545. doi: 10.1007/BF00239810. [DOI] [PubMed] [Google Scholar]