Abstract
1. The excitation—contraction coupling mechanism in the smooth muscle of the guinea-pig mesenteric artery was studied using intact and chemically skinned muscle cells.
2. The mean membrane potential of the intact smooth muscle was -65.8 ± 2.4 mV. It was electrically quiescent. Caffeine (5 mm), procaine (> 1 mm) and TEA (> 1 mm) depolarized the membrane, increased the membrane resistance and in their presence, outward current pulses evoked action potentials with overshoot. These potential changes were still observed in Na-deficient solution but were abolished in the presence of 3 mm-MnCl2.
3. Caffeine (5 mm) and TEA (1 mm) produced contractions in the intact muscle which were suppressed by procaine (5-10 mm). Caffeine (5 mm) continued to produce contraction even after prolonged exposure to Ca-free solution (containing 2 mm-EGTA) and this contraction was suppressed by procaine (5 mm). On the other hand, the K-induced contraction was rapidly abolished in 0-Ca.
4. Electrical stimulation (1 sec) in the presence of TTX (10-7 m) evoked a contraction. Caffeine (5 mm) and TEA (5 mm) enhanced but procaine (5 mm) suppressed the contraction.
5. Chemically skinned smooth muscle cells were prepared by adding saponin, 50 μg/ml., to the relaxing solution. The minimum concentration of free Ca required to evoke contraction in skinned muscle cells was 1-2 × 10-7 m and the maximum contraction was produced at 10-5 m. When Ca was replaced with Sr, the above relationship also shifted to the right (ED50 for Ca is 4.4 × 10-7 m and that for Sr is 1.5 × 10-5 m). Treatment with high concentrations of caffeine and procaine had no effect on the pCa—tension relationship.
6. Caffeine induced contraction in skinned muscle cells preloaded with Ca, and this contraction was markedly suppressed by procaine (5-10 mm).
7. In skinned muscles, depolarization of the internal membrane by replacement of K with choline (116 mm) in the relaxing solution produced contraction, but the amplitude was much smaller than the caffeine-induced contraction.
8. The relationship between the amplitude of caffeine-induced contraction and the duration of preincubation in various Ca concentrations was observed in skinned muscles. The minimum concentration of Ca required to produce a subsequent caffeine-induced contraction was itself below threshold for contraction. The results also indicate that the Ca-induced Ca release mechanism appears to modify the amount of Ca stored by preincubation in over 3 × 10-7 m free Ca.
9. When the amount of Ca stored in intact cells was estimated from the caffeine-induced contraction evoked in Ca-free solution following preincubation with Ca, Ca applied simultaneously with procaine increased and Ca with caffeine reduced the Ca stored in the cell. After preincubation in 2.5 mm-[Ca]o with 1 mm-procaine for 5 min, the amplitude of the subsequently generated caffeine-induced contraction (5 mm) in Ca-free solution (2 min) was much the same as that observed in 118 mm-[K]o.
10. The results support the view that the excitation—contraction coupling mechanism in the mesenteric artery may be as follows; the Ca inward current generated at the myoplasmic membrane may not directly provide the free Ca required to activate the Ca-receptor of the contractile protein, but the Ca carrying the inward current may first be sequestered inside the cell and activate a Ca release mechanism which in turn leads to contraction.
Full text
PDF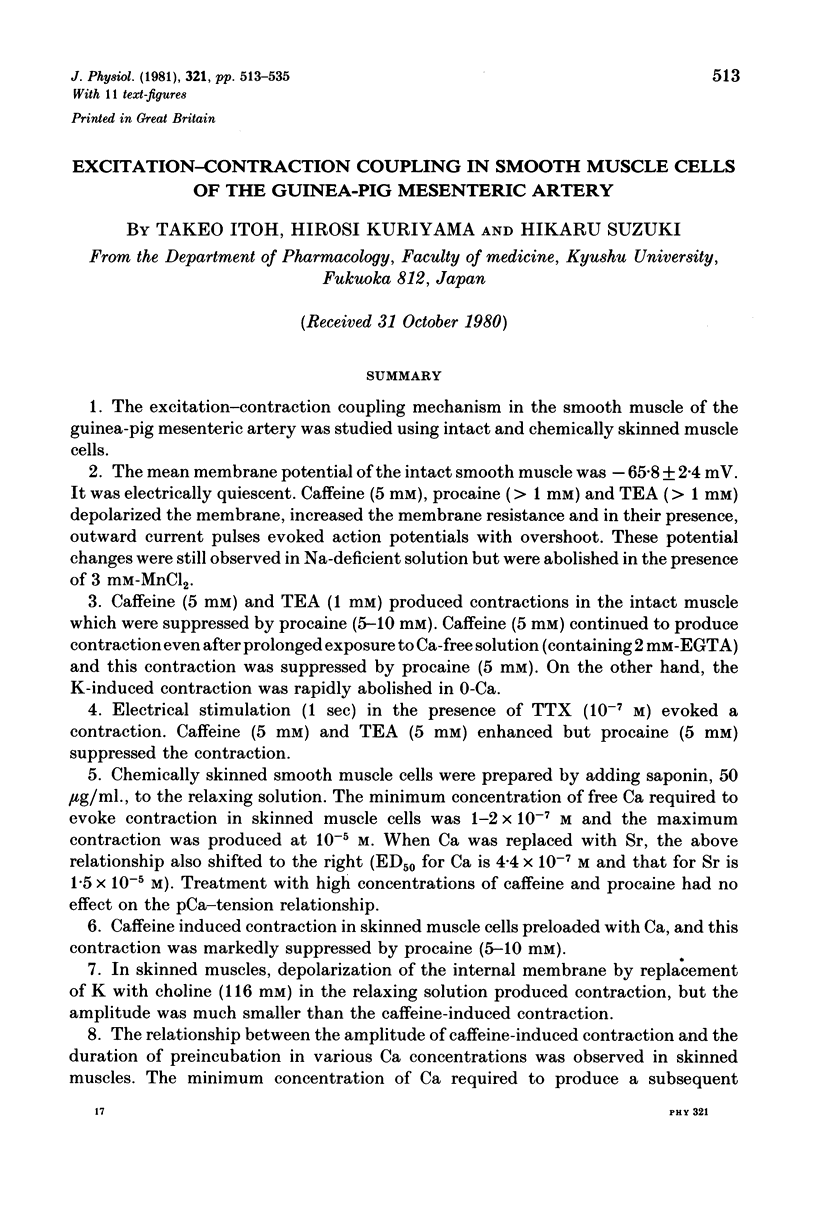
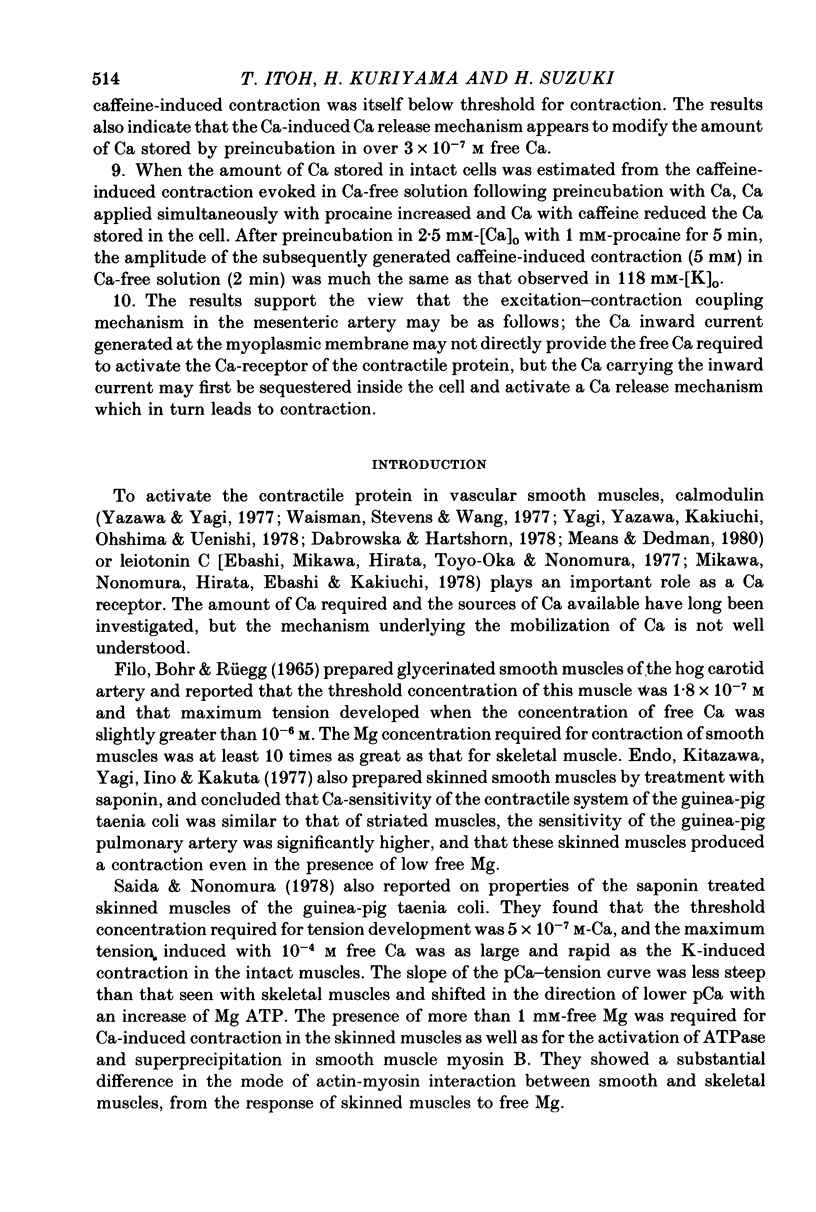
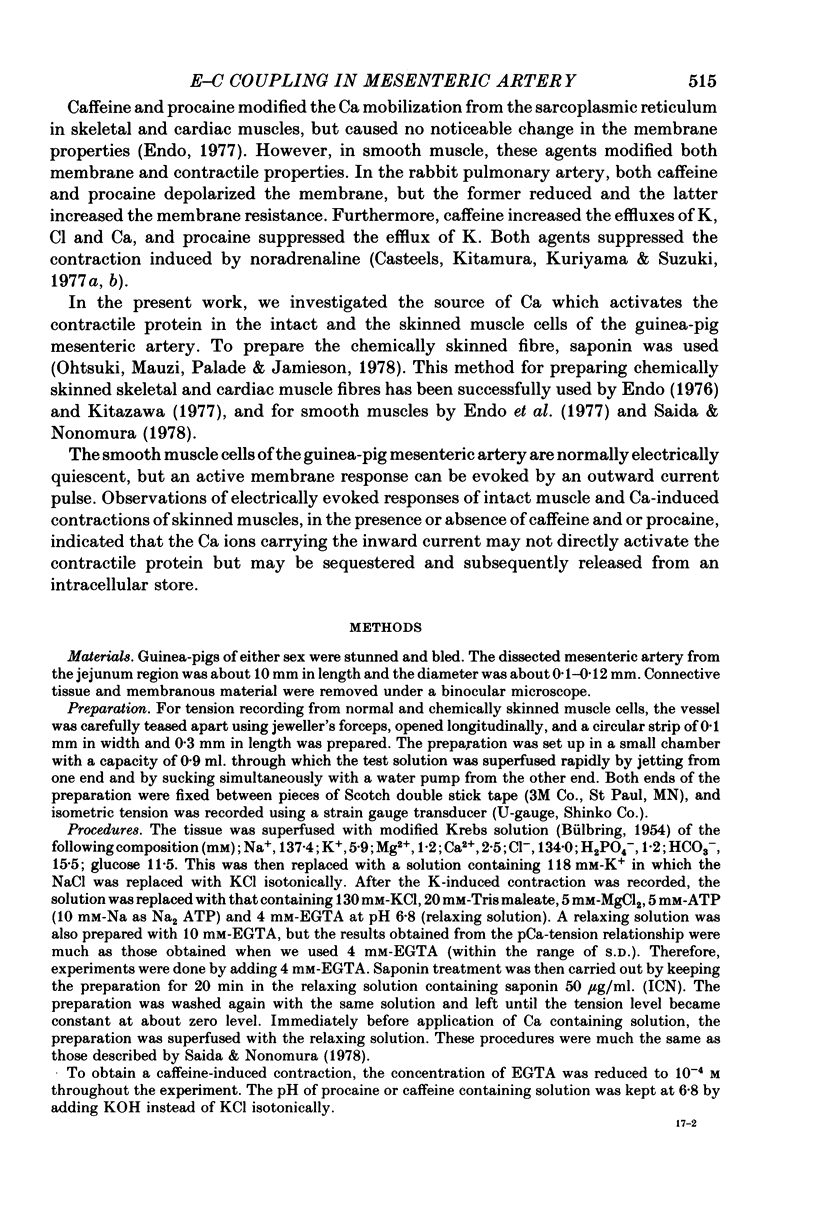
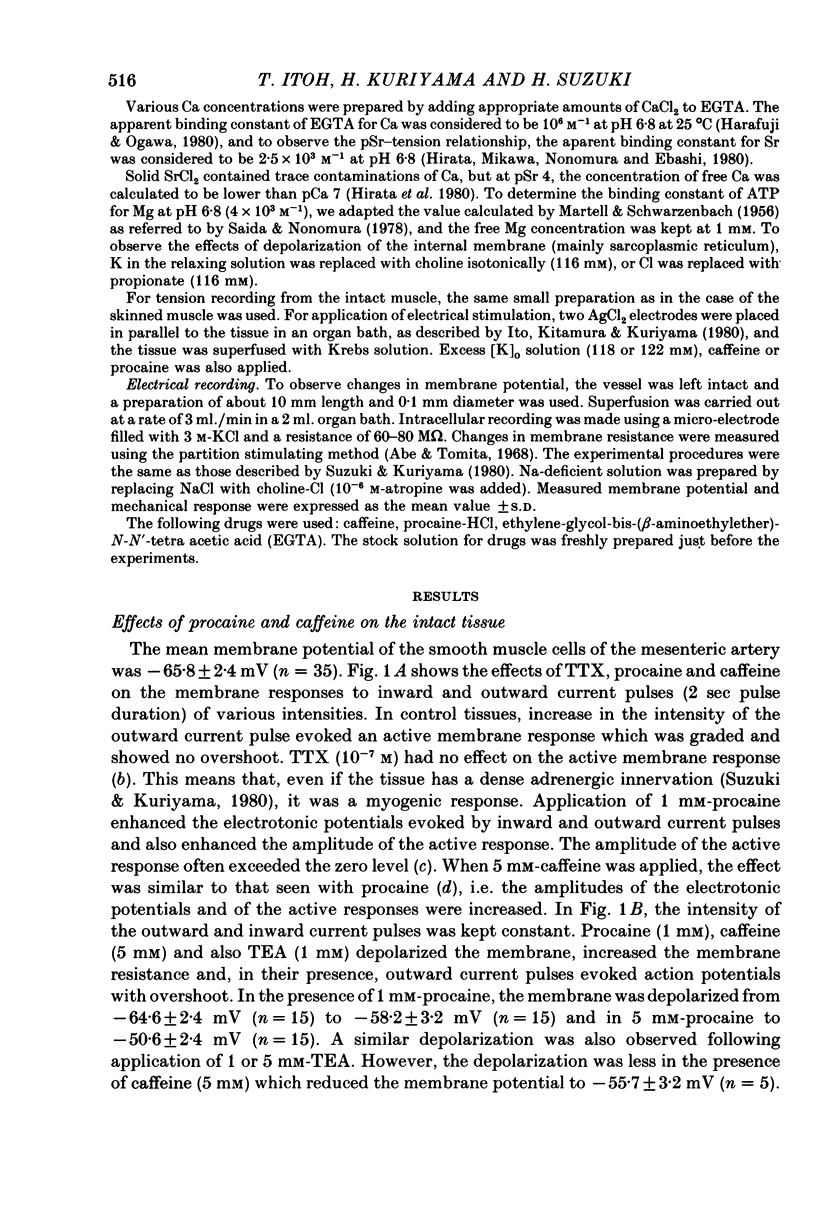
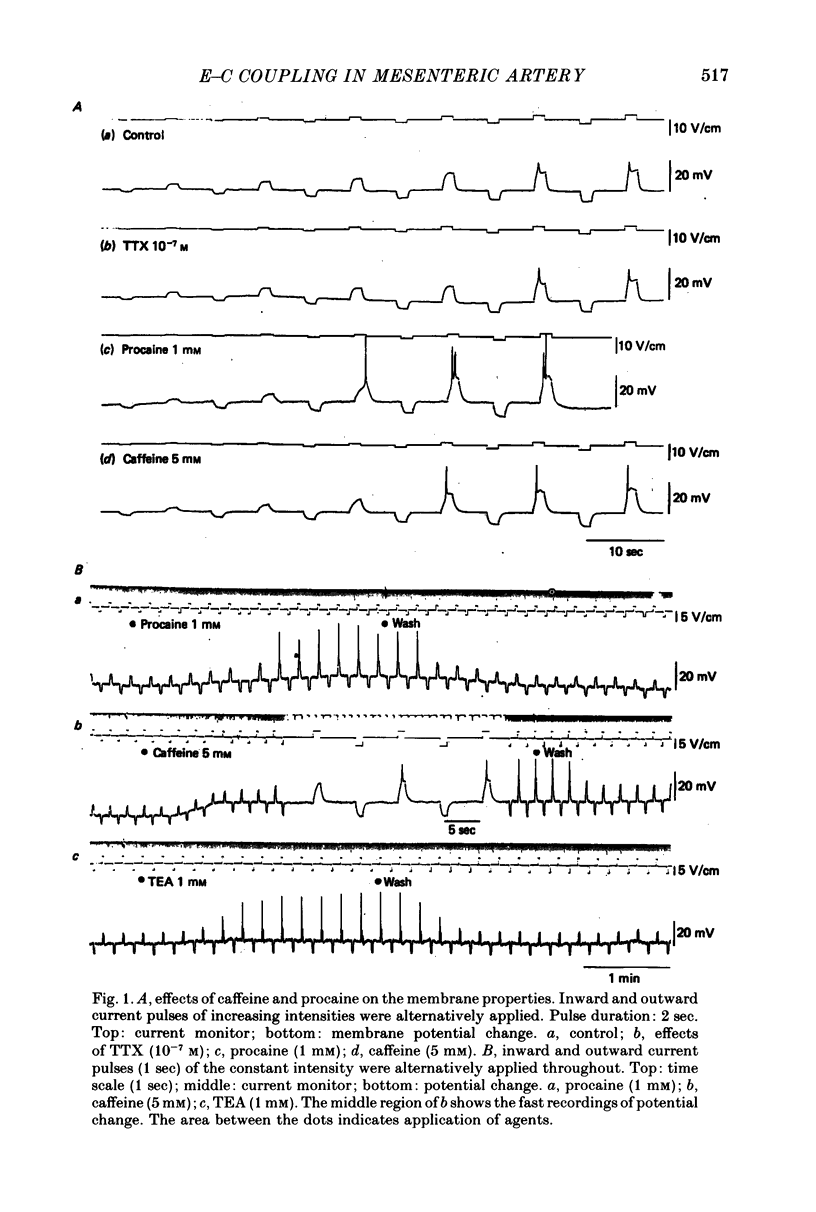
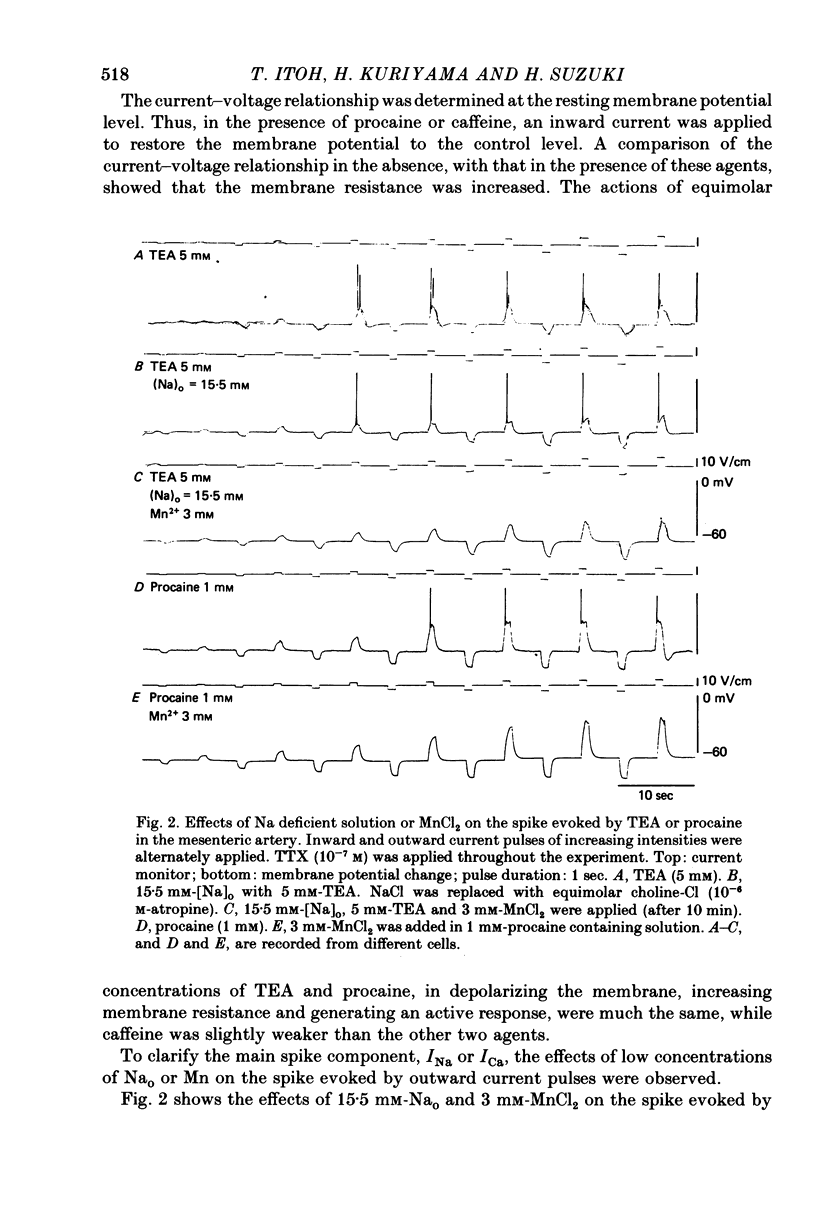
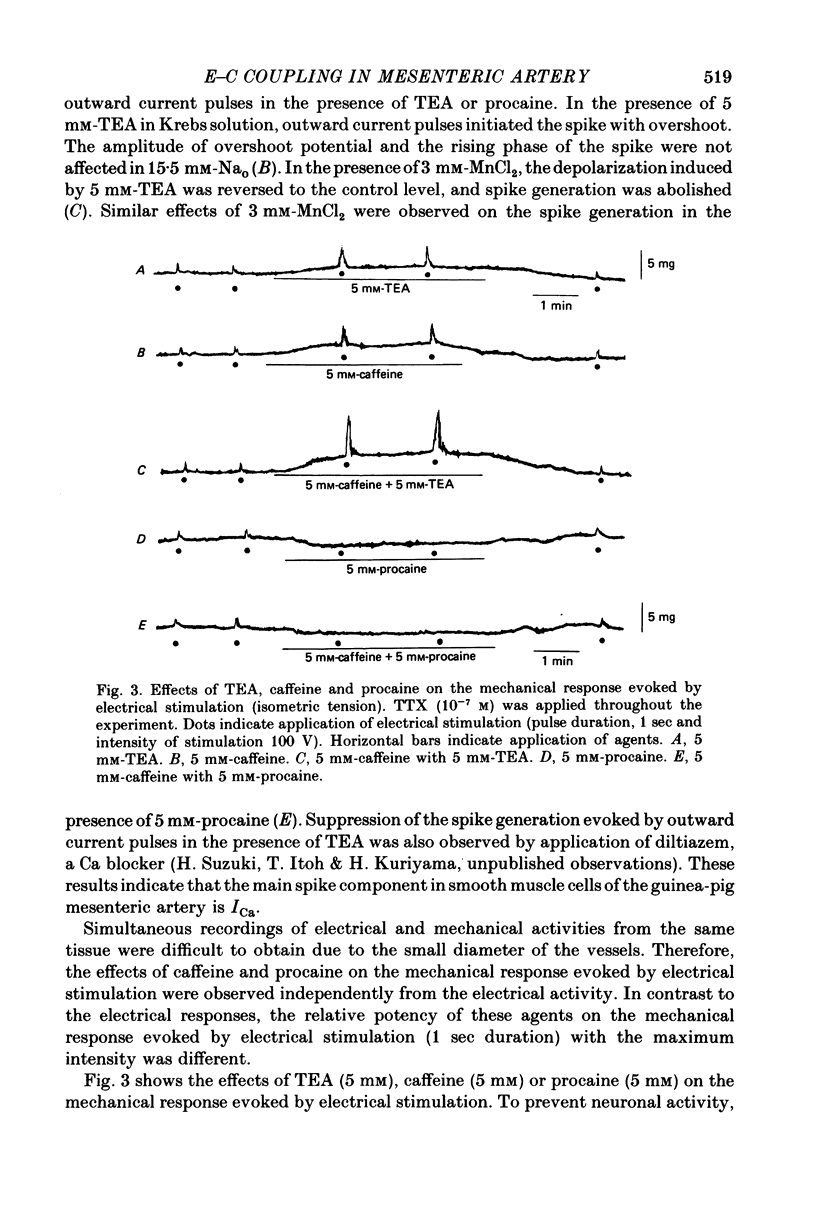
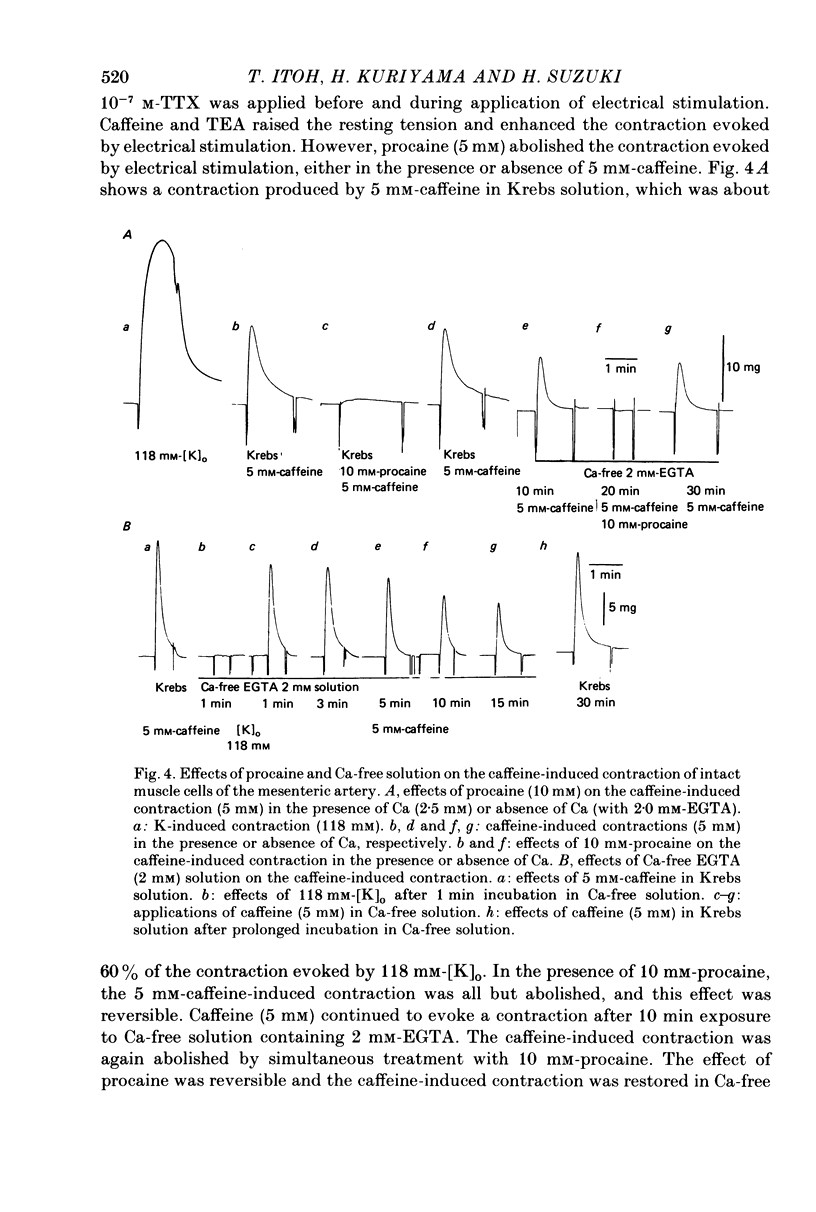
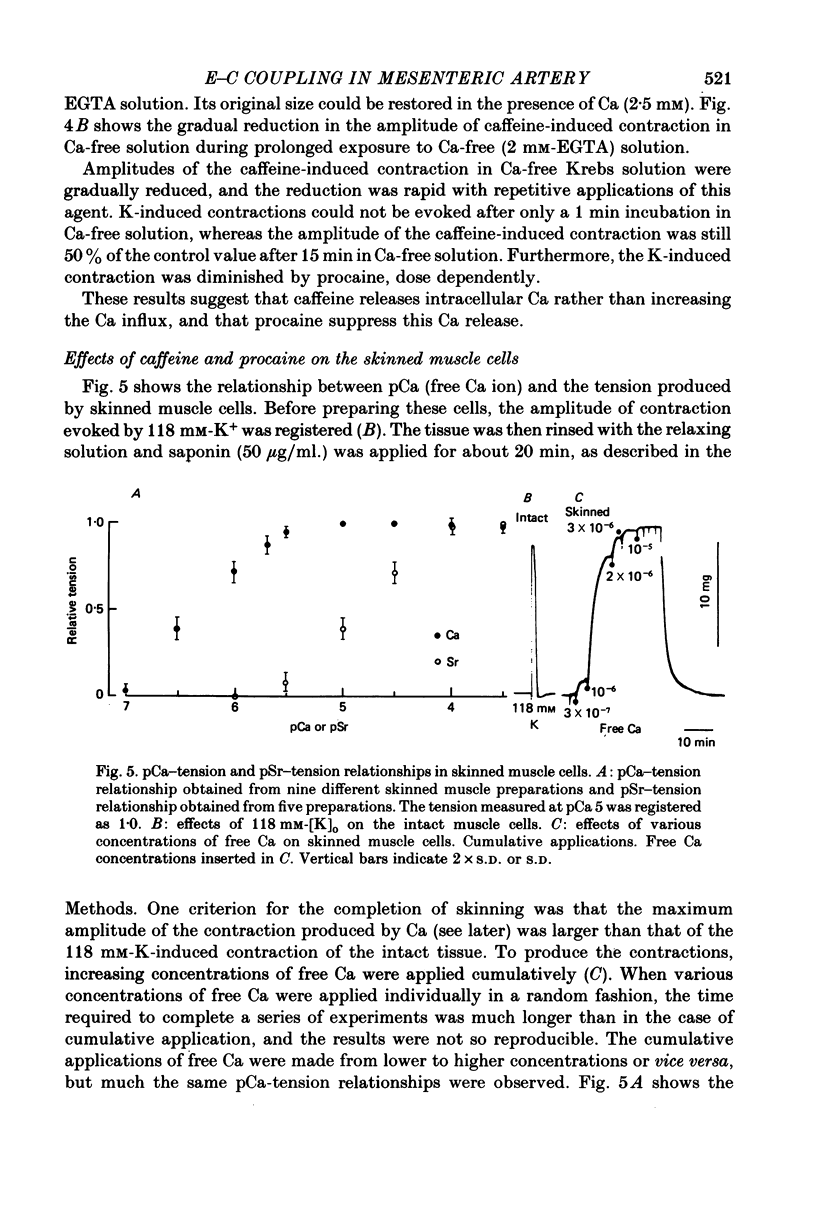
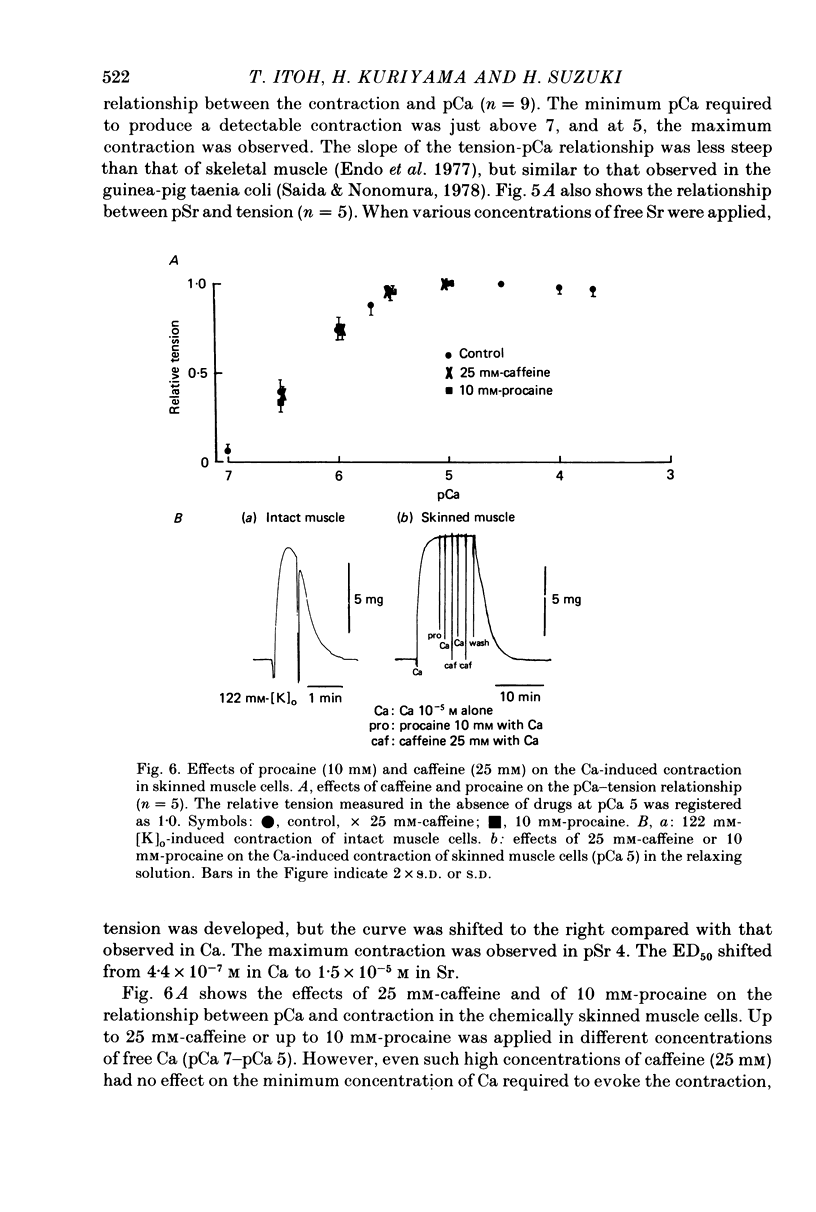
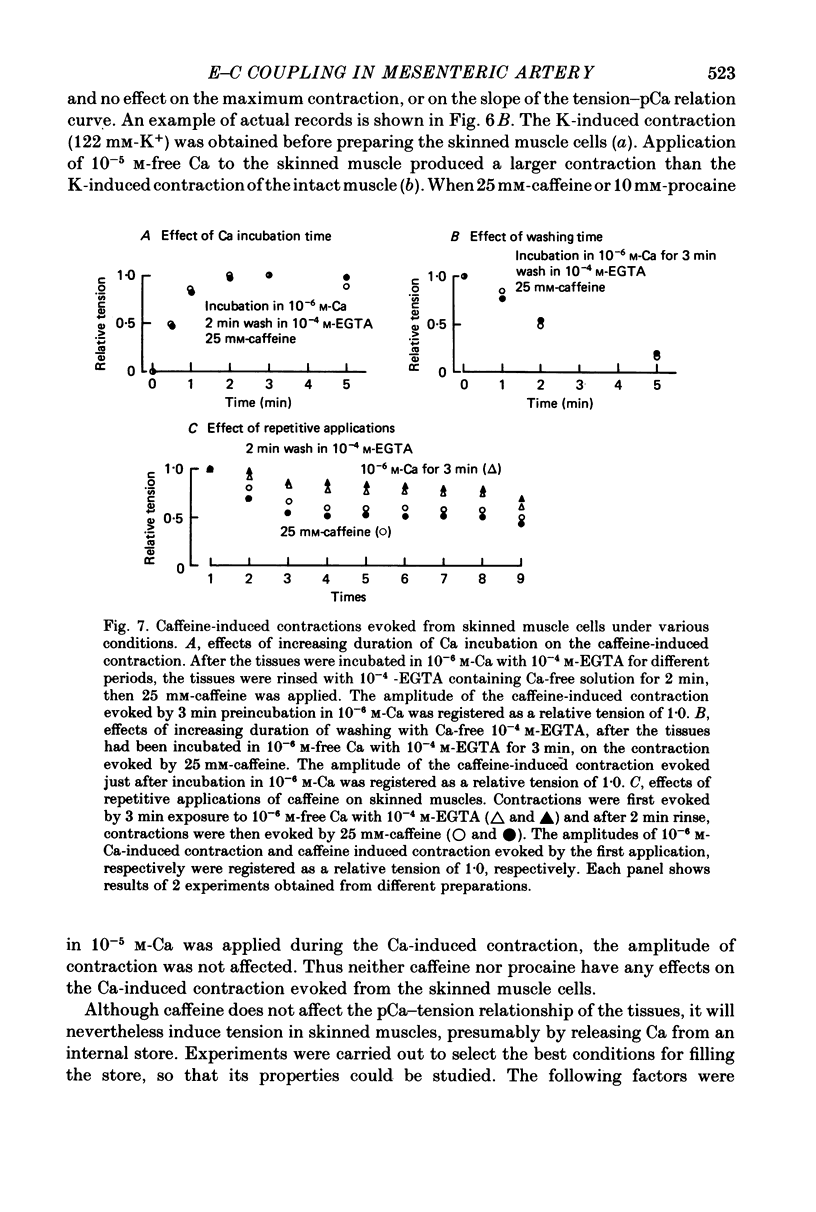
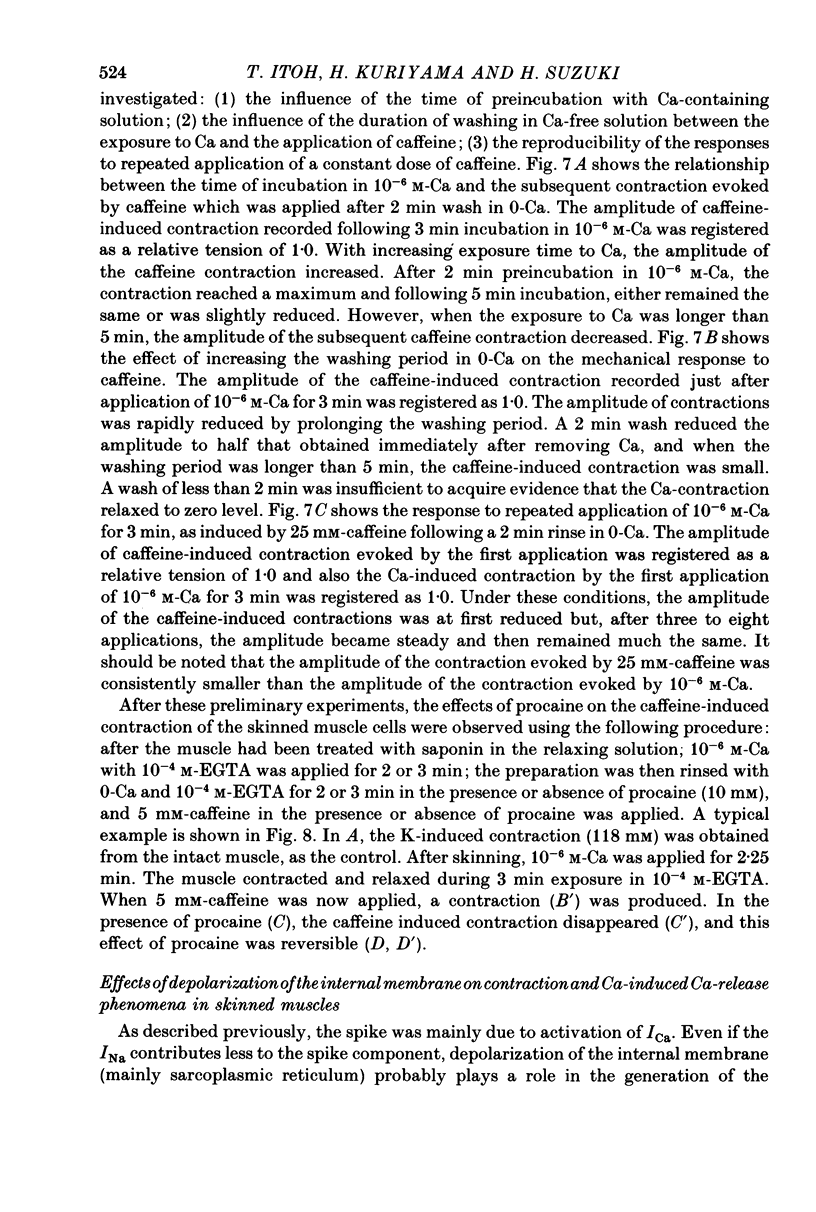
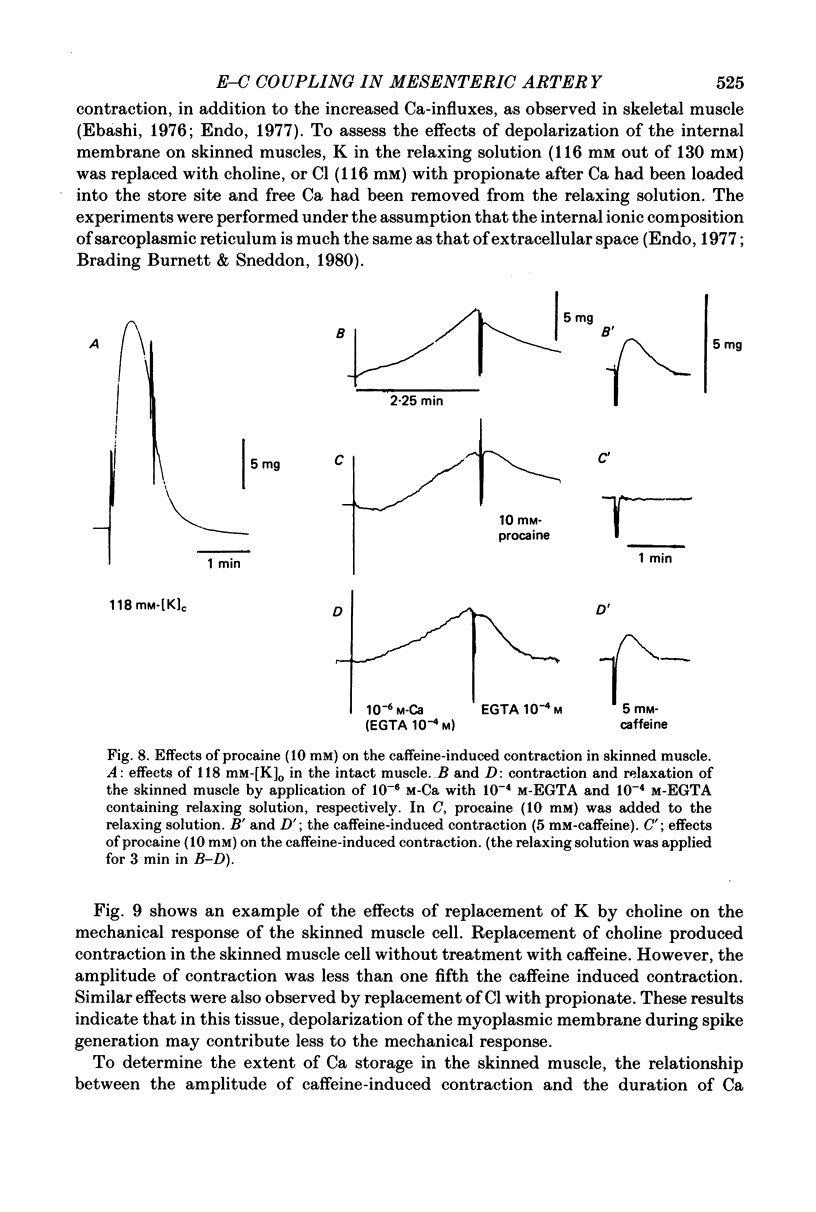
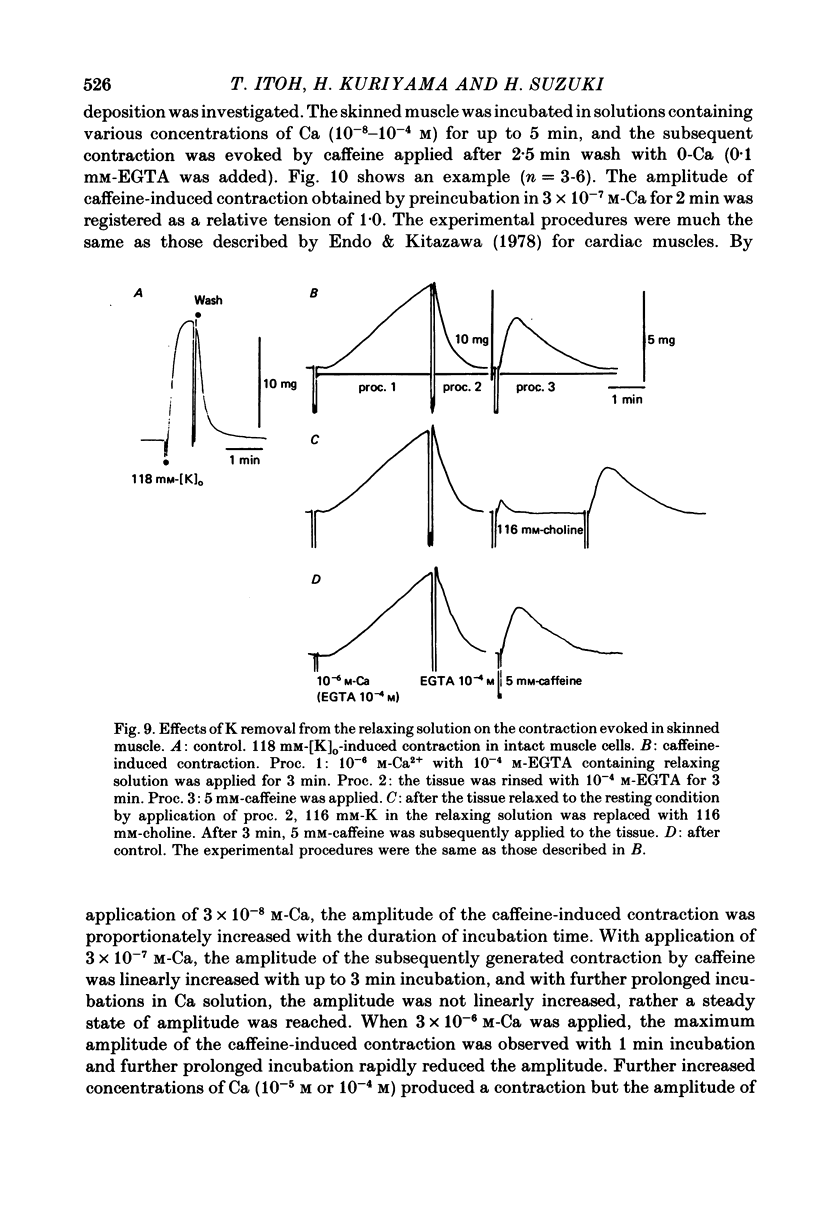
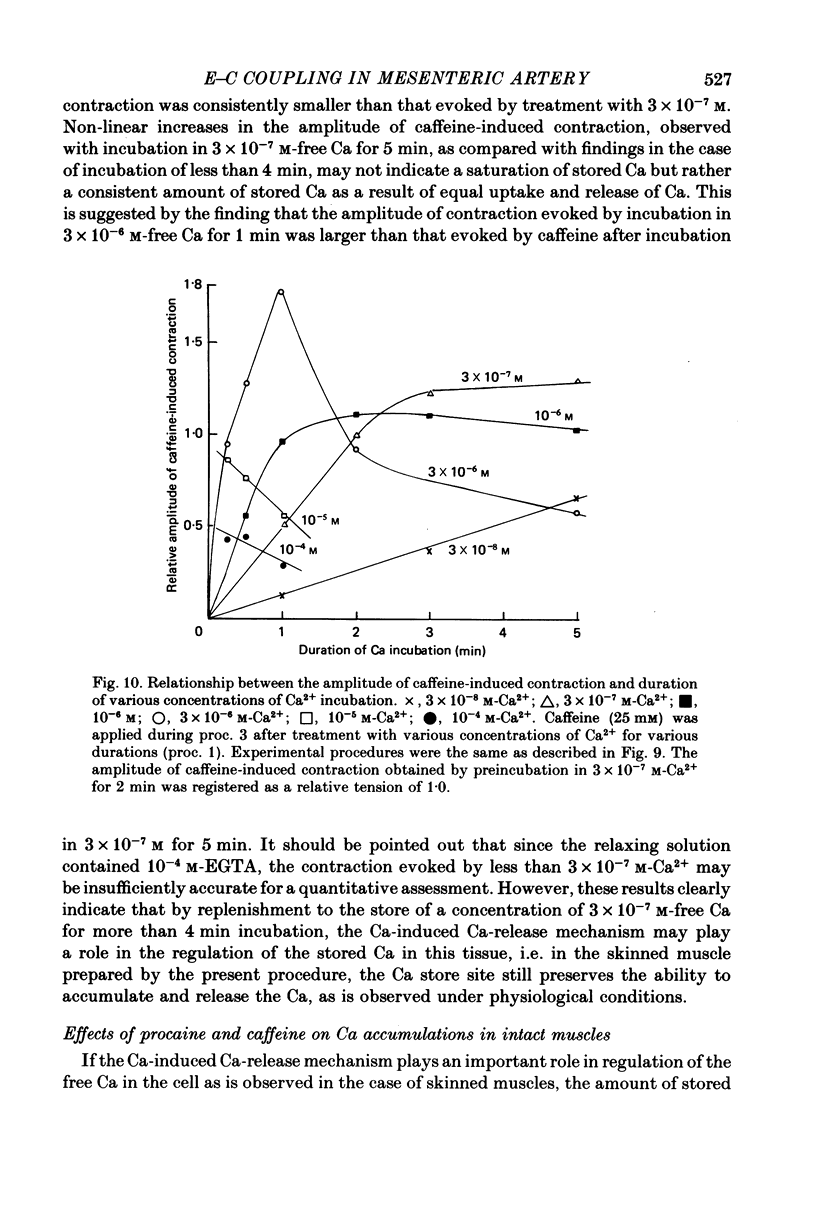
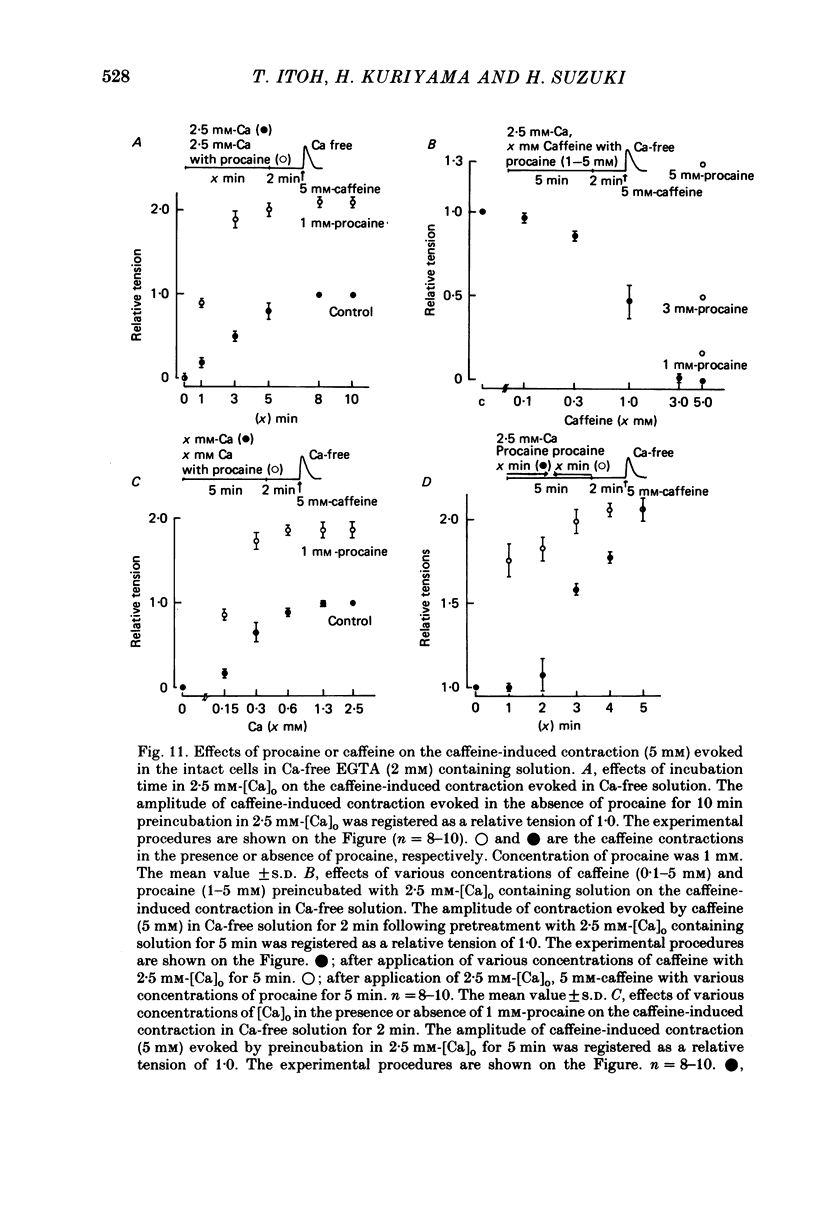
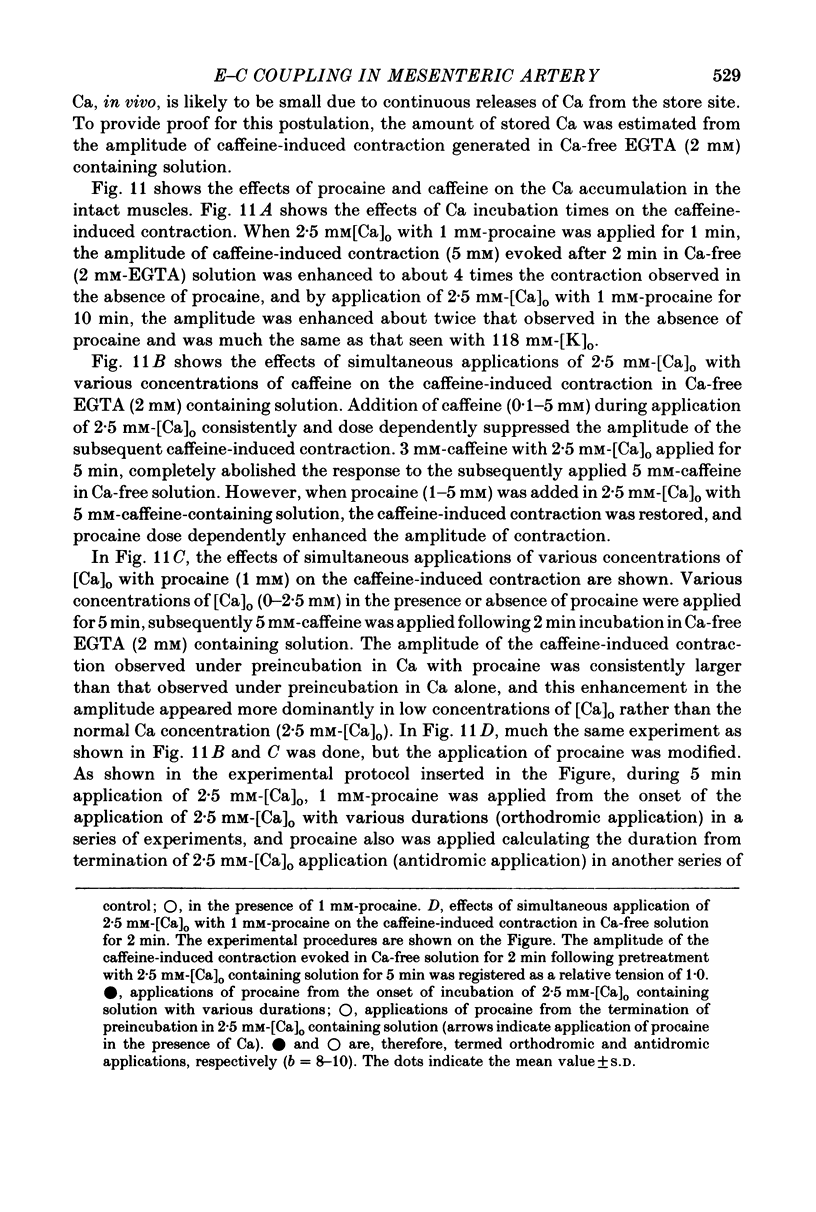
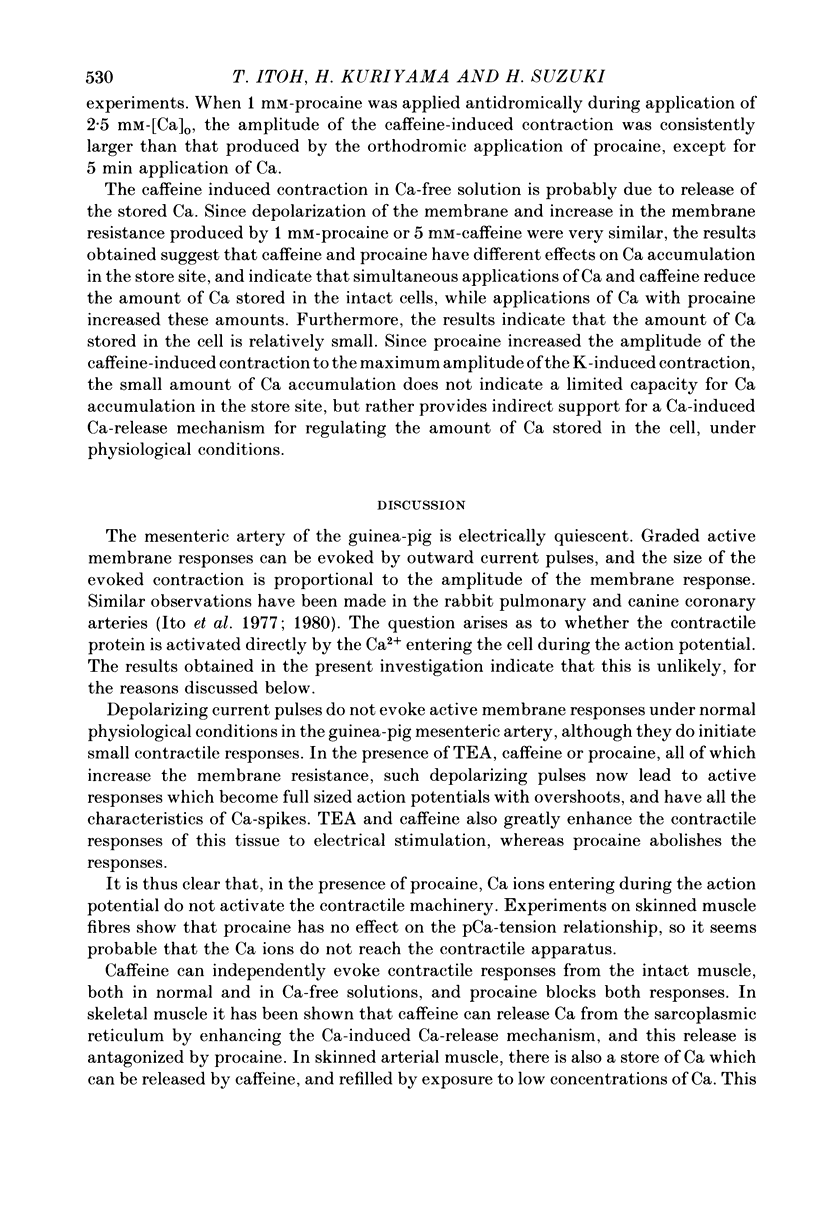
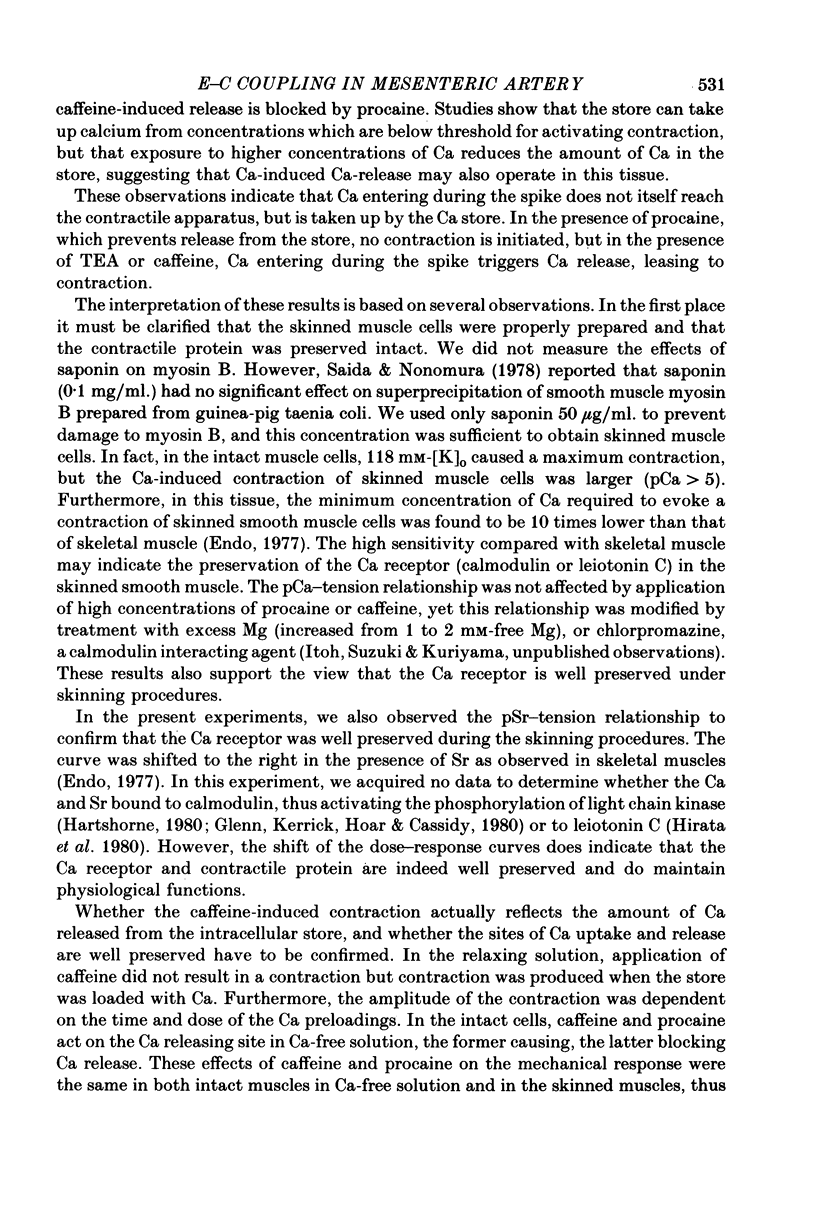
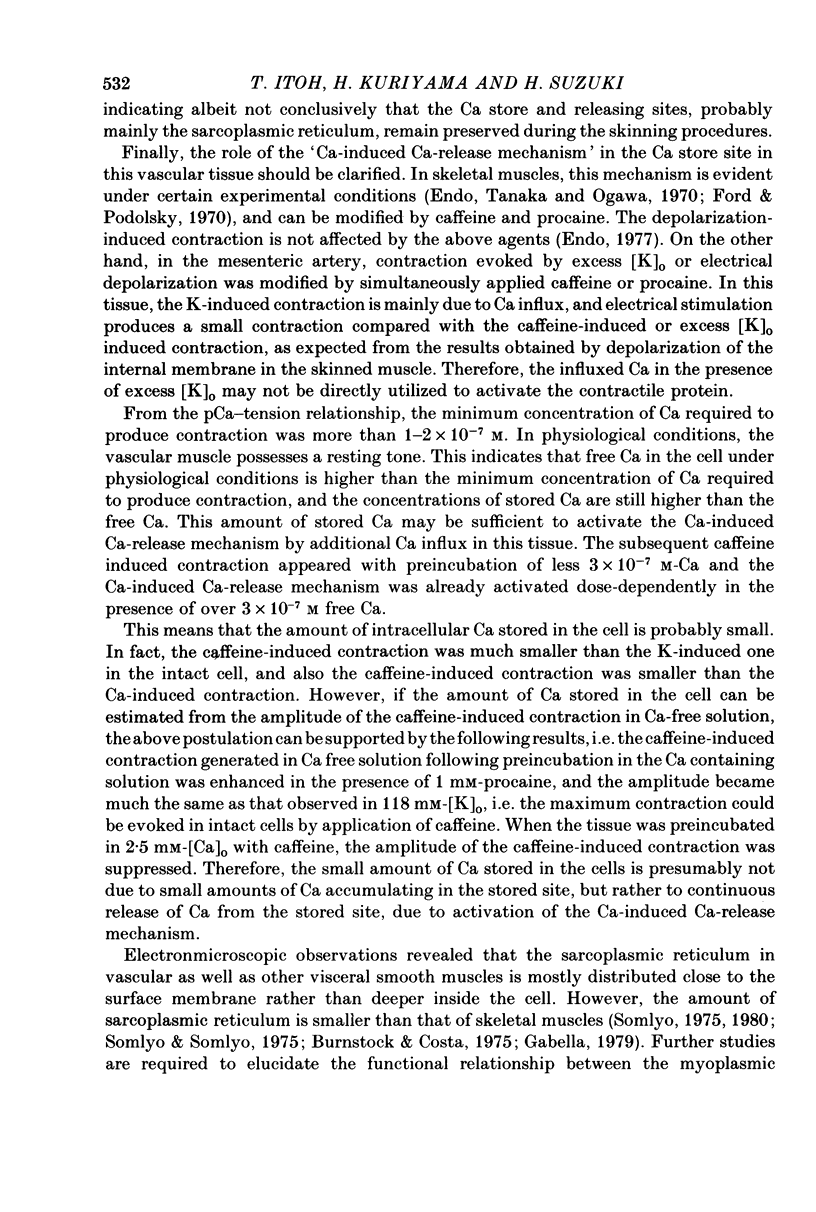
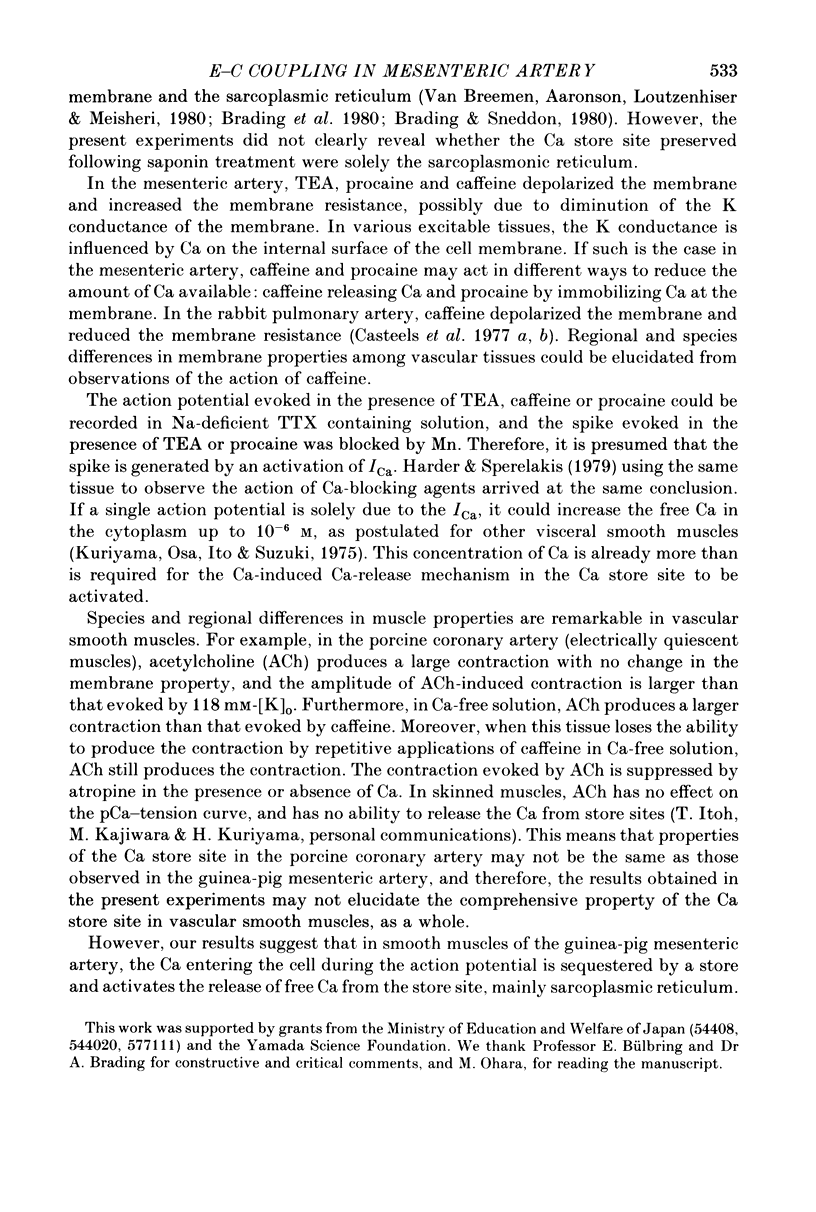
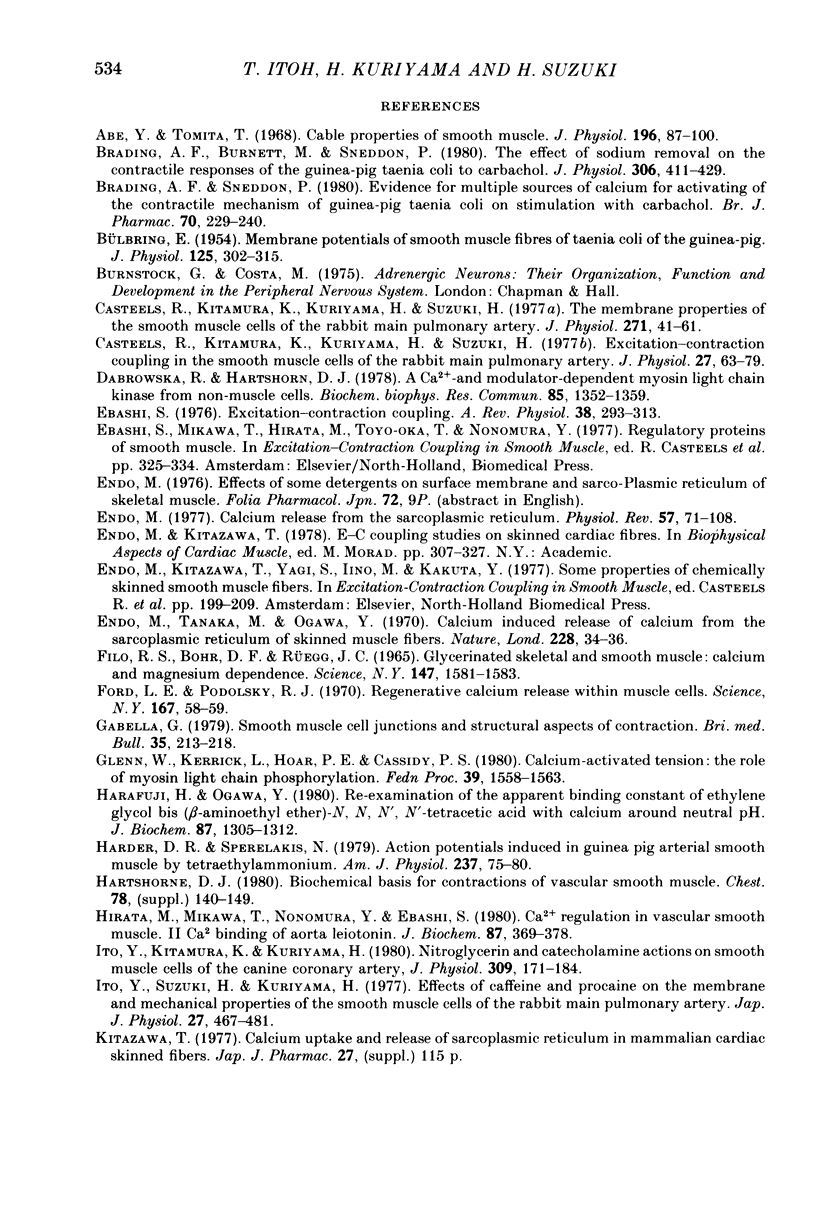
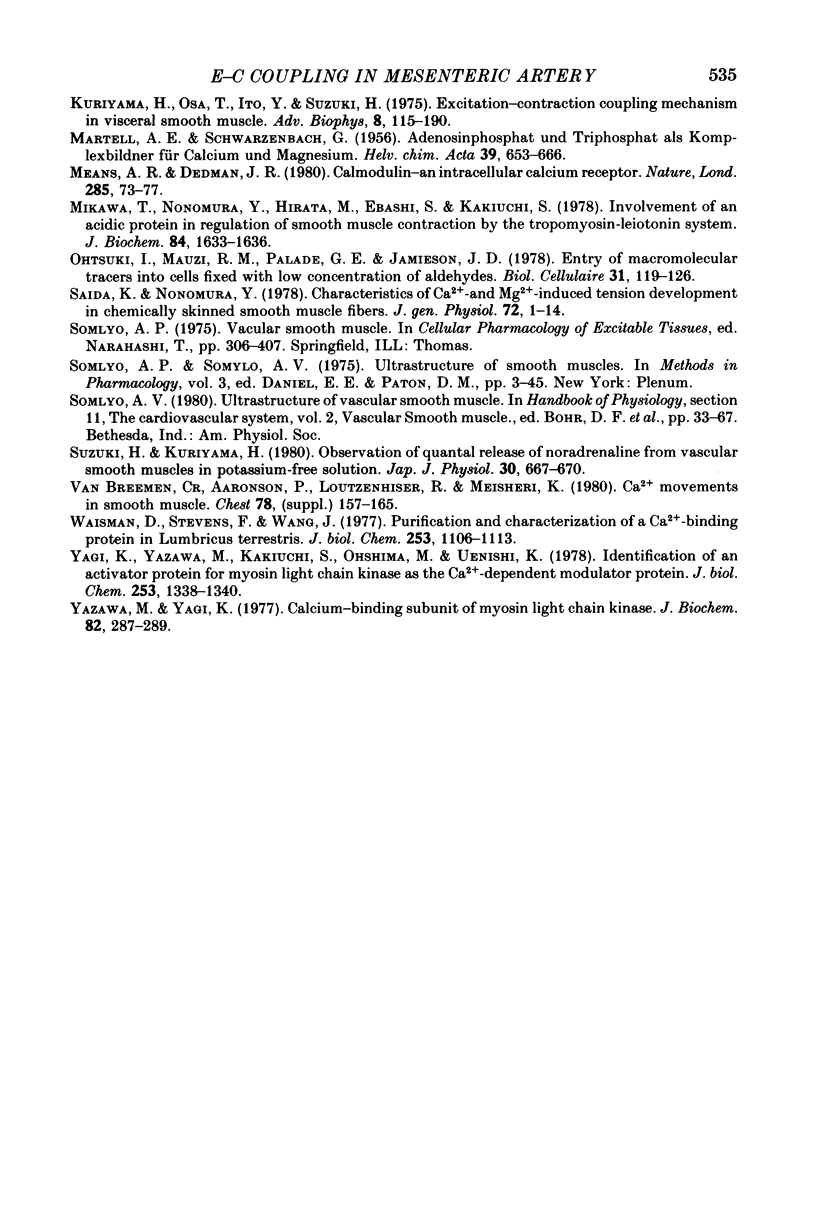
Selected References
These references are in PubMed. This may not be the complete list of references from this article.
- Abe Y., Tomita T. Cable properties of smooth muscle. J Physiol. 1968 May;196(1):87–100. doi: 10.1113/jphysiol.1968.sp008496. [DOI] [PMC free article] [PubMed] [Google Scholar]
- BULBRING E. Membrane potentials of smooth muscle fibres of the taenia coli of the guinea-pig. J Physiol. 1954 Aug 27;125(2):302–315. doi: 10.1113/jphysiol.1954.sp005159. [DOI] [PMC free article] [PubMed] [Google Scholar]
- Brading A. F., Burnett M., Sneddon P. The effect of sodium removal on the contractile response of the guinea-pig taenia coli to carbachol. J Physiol. 1980 Sep;306:411–429. doi: 10.1113/jphysiol.1980.sp013404. [DOI] [PMC free article] [PubMed] [Google Scholar]
- Brading A. F., Sneddon P. Evidence for multiple sources of calcium for activation of the contractile mechanism of guinea-pig taenia coli on stimulation with carbachol. Br J Pharmacol. 1980 Oct;70(2):229–240. doi: 10.1111/j.1476-5381.1980.tb07928.x. [DOI] [PMC free article] [PubMed] [Google Scholar]
- Casteels R., Kitamura K., Kuriyama H., Suzuki H. Excitation-contraction coupling in the smooth muscle cells of the rabbit main pulmonary artery. J Physiol. 1977 Sep;271(1):63–79. doi: 10.1113/jphysiol.1977.sp011990. [DOI] [PMC free article] [PubMed] [Google Scholar]
- Casteels R., Kitamura K., Kuriyama H., Suzuki H. The membrane properties of the smooth muscle cells of the rabbit main pulmonary artery. J Physiol. 1977 Sep;271(1):41–61. doi: 10.1113/jphysiol.1977.sp011989. [DOI] [PMC free article] [PubMed] [Google Scholar]
- Dabrowska R., Hartshorne D. J. A Ca2+-and modulator-dependent myosin light chain kinase from non-muscle cells. Biochem Biophys Res Commun. 1978 Dec 29;85(4):1352–1359. doi: 10.1016/0006-291x(78)91152-x. [DOI] [PubMed] [Google Scholar]
- Ebashi S. Excitation-contraction coupling. Annu Rev Physiol. 1976;38:293–313. doi: 10.1146/annurev.ph.38.030176.001453. [DOI] [PubMed] [Google Scholar]
- Endo M. Calcium release from the sarcoplasmic reticulum. Physiol Rev. 1977 Jan;57(1):71–108. doi: 10.1152/physrev.1977.57.1.71. [DOI] [PubMed] [Google Scholar]
- Endo M., Tanaka M., Ogawa Y. Calcium induced release of calcium from the sarcoplasmic reticulum of skinned skeletal muscle fibres. Nature. 1970 Oct 3;228(5266):34–36. doi: 10.1038/228034a0. [DOI] [PubMed] [Google Scholar]
- FILO R. S., BOHR D. F., RUEGG J. C. GLYCERINATED SKELETAL AND SMOOTH MUSCLE: CALCIUM AND MAGNESIUM DEPENDENCE. Science. 1965 Mar 26;147(3665):1581–1583. doi: 10.1126/science.147.3665.1581. [DOI] [PubMed] [Google Scholar]
- Ford L. E., Podolsky R. J. Regenerative calcium release within muscle cells. Science. 1970 Jan 2;167(3914):58–59. doi: 10.1126/science.167.3914.58. [DOI] [PubMed] [Google Scholar]
- Gabella G. Smooth muscle cell junctions and structural aspects of contraction. Br Med Bull. 1979 Sep;35(3):213–218. doi: 10.1093/oxfordjournals.bmb.a071580. [DOI] [PubMed] [Google Scholar]
- Harafuji H., Ogawa Y. Re-examination of the apparent binding constant of ethylene glycol bis(beta-aminoethyl ether)-N,N,N',N'-tetraacetic acid with calcium around neutral pH. J Biochem. 1980 May;87(5):1305–1312. doi: 10.1093/oxfordjournals.jbchem.a132868. [DOI] [PubMed] [Google Scholar]
- Hartshorne D. J. Biochemical basis for contraction of vascular smooth muscle. Chest. 1980 Jul;78(1 Suppl):140–149. doi: 10.1378/chest.78.1_supplement.140. [DOI] [PubMed] [Google Scholar]
- Hirata M., Mikawa T., Nonomura Y., Ebashi S. Ca2+ regulation in vascular smooth muscle. II. Ca2+ binding of aorta leiotonin. J Biochem. 1980 Feb;87(2):369–378. doi: 10.1093/oxfordjournals.jbchem.a132757. [DOI] [PubMed] [Google Scholar]
- Ito Y., Kitamura K., Kuriyama H. Nitroglycerine and catecholamine actions on smooth muscle cells of the canine coronary artery. J Physiol. 1980 Dec;309:171–183. doi: 10.1113/jphysiol.1980.sp013502. [DOI] [PMC free article] [PubMed] [Google Scholar]
- Ito Y., Suzuki H., Kuriyama H. Effects of caffeine and procaine on the membrane and mechanical properties of the smooth muscle cells of the rabbit main pulmonary artery. Jpn J Physiol. 1977;27(4):467–481. doi: 10.2170/jjphysiol.27.467. [DOI] [PubMed] [Google Scholar]
- Kerrick W. G., Hoar P. E., Cassidy P. S. Calcium-activated tension: the role of myosin light chain phosphorylation. Fed Proc. 1980 Apr;39(5):1558–1563. [PubMed] [Google Scholar]
- Kuriyama H., Osa T., Ito Y., Suzuki H. Excitation-contraction coupling mechanism in visceral smooth muscle. Adv Biophys. 1976;8:115–190. [PubMed] [Google Scholar]
- Means A. R., Dedman J. R. Calmodulin--an intracellular calcium receptor. Nature. 1980 May 8;285(5760):73–77. doi: 10.1038/285073a0. [DOI] [PubMed] [Google Scholar]
- Mikawa T., Nonomura Y., Hirata M., Ebashi S., Kakiuchi S. Involvement of an acidic protein in regulation of smooth muscle contraction by the tropomyosin-leiotonin system. J Biochem. 1978 Dec;84(6):1633–1636. doi: 10.1093/oxfordjournals.jbchem.a132290. [DOI] [PubMed] [Google Scholar]
- Saida K., Nonomura Y. Characteristics of Ca2+- and Mg2+-induced tension development in chemically skinned smooth muscle fibers. J Gen Physiol. 1978 Jul;72(1):1–14. doi: 10.1085/jgp.72.1.1. [DOI] [PMC free article] [PubMed] [Google Scholar]
- Waisman D. M., Stevens F. C., Wang J. H. Purification and characterization of a Ca2+-binding protein in Lumbricus terrestris. J Biol Chem. 1978 Feb 25;253(4):1106–1113. [PubMed] [Google Scholar]
- Yagi K., Yazawa M., Kakiuchi S., Ohshima M., Uenishi K. Identification of an activator protein for myosin light chain kinase as the Ca2+-dependent modulator protein. J Biol Chem. 1978 Mar 10;253(5):1338–1340. [PubMed] [Google Scholar]
- Yazawa M., Yagi K. Calcium-binding subunit of myosin light chain kinase. J Biochem. 1977 Jul;82(1):287–289. doi: 10.1093/oxfordjournals.jbchem.a131681. [DOI] [PubMed] [Google Scholar]
- van Breemen C., Aaronson P., Loutzenhiser R., Meisheri K. Ca2+ movements in smooth muscle. Chest. 1980 Jul;78(1 Suppl):157–165. doi: 10.1378/chest.78.1_supplement.157. [DOI] [PubMed] [Google Scholar]