Abstract
1. Experiments were performed to determine whether a decrease in tissue ATP contributes to the rapid failure of cerebral synaptic transmission during hypoxia. Transmission between the perforant path and the dentate granule cells in the in vitro hippocampus was studied.
2. Hippocampal slice ATP is decreased by ∼ 15% at the time that the evoked response begins to diminish in standard Krebs bicarbonate buffer. This is about 2 min after the onset of hypoxia.
3. When transmission failure is accelerated by increasing extracellular K+ from 4·4 to 13·4 mM, the evoked response begins to decay about 30 sec after exposure to hypoxia. There is no decrease in hippocampal slice ATP at this time.
4. However, ATP in the molecular layer (the synaptic region of the tissue) is decreased by ∼ 15% at the time the evoked response begins to decay in the slices exposed to elevated K+ concentration.
5. Exposing the hippocampal slice to 25 mM-creatine for 3 hr elevates molecular layer phosphocreatine fourfold. Synaptic transmission during hypoxia survives three times as long as it does in the absence of creatine.
6. In the creatine fortified medium, molecular layer ATP no longer declines within 30 sec of hypoxia. However the molecular layer ATP does decline within 90 sec of hypoxia, the time at which the evoked response begins to decay in this creatine-fortified buffer.
7. The results establish that ATP in the region of the active synapses is lowered when the first signs of electrophysiological failure appear during hypoxia. They also show that maintaining ATP for longer than normal during hypoxia is associated with a prolonged maintenance of the evoked response. They thus suggest that a decline in ATP is one factor causing hypoxic block of synaptic transmission.
8. It is further suggested that the very rapid failure of the electroencephalogram during anoxia may also result from a decline in ATP.
Full text
PDF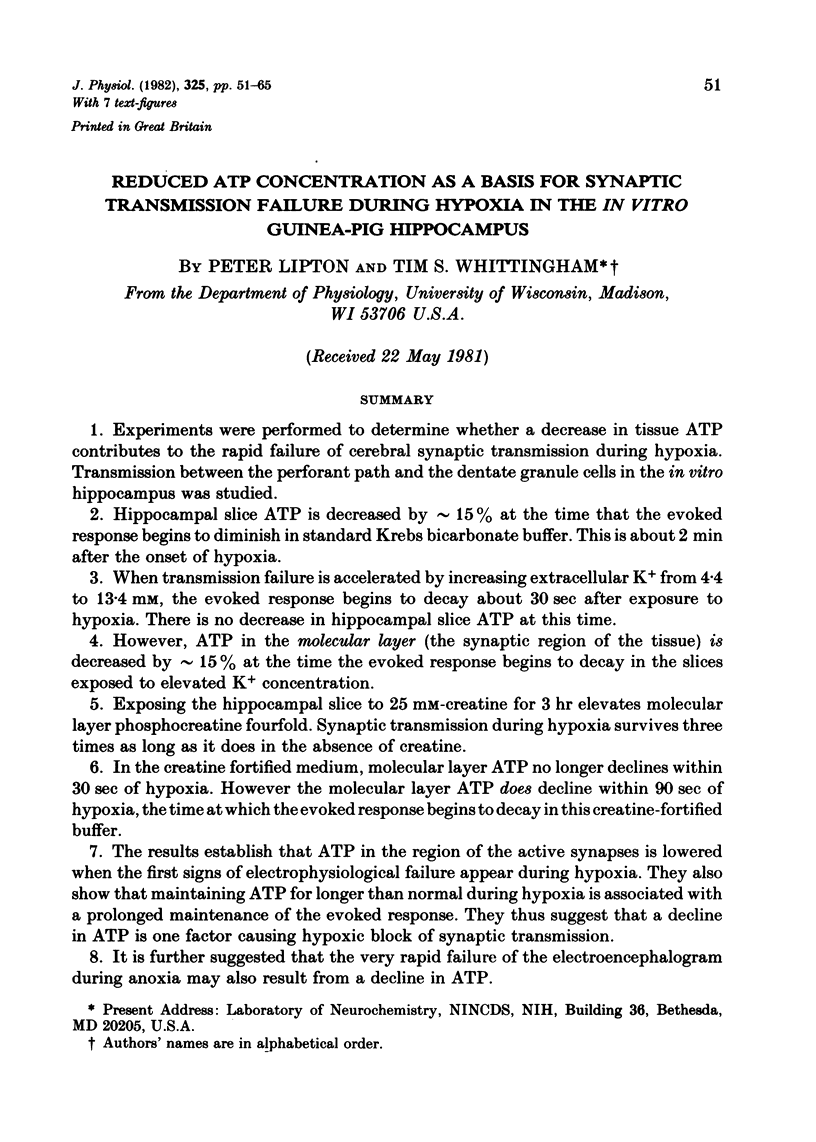
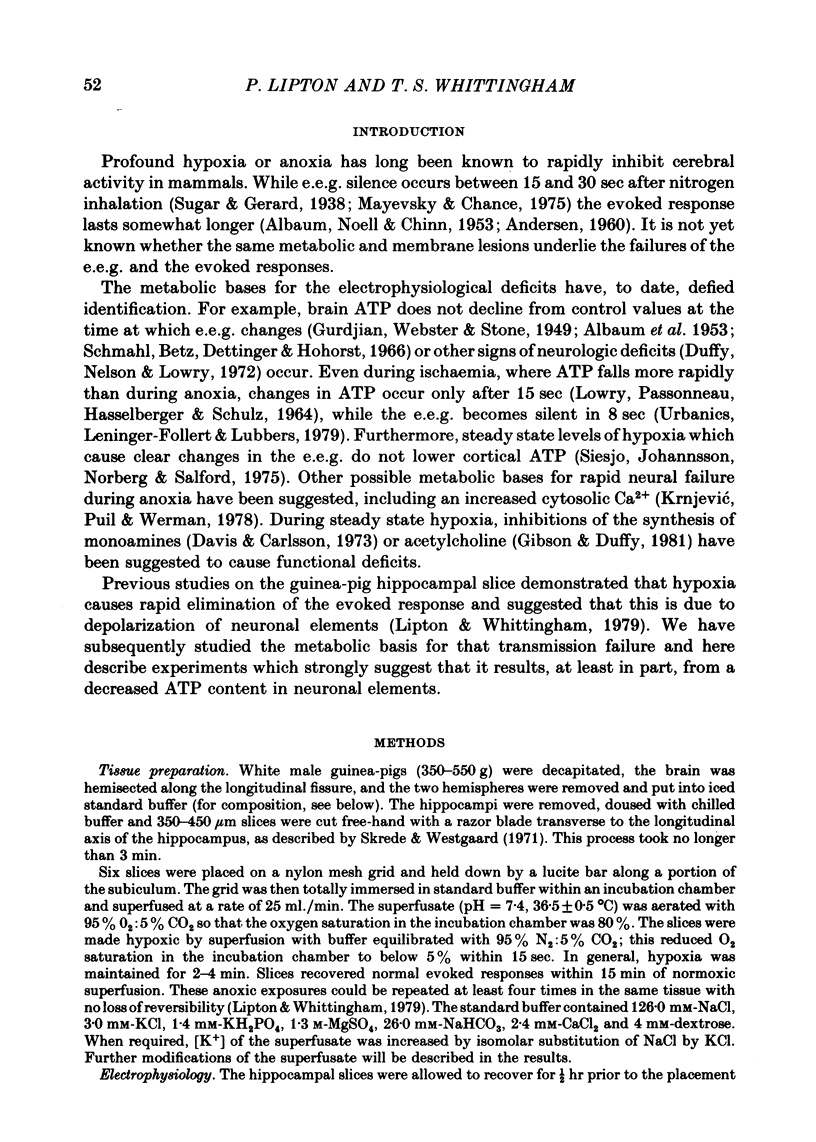
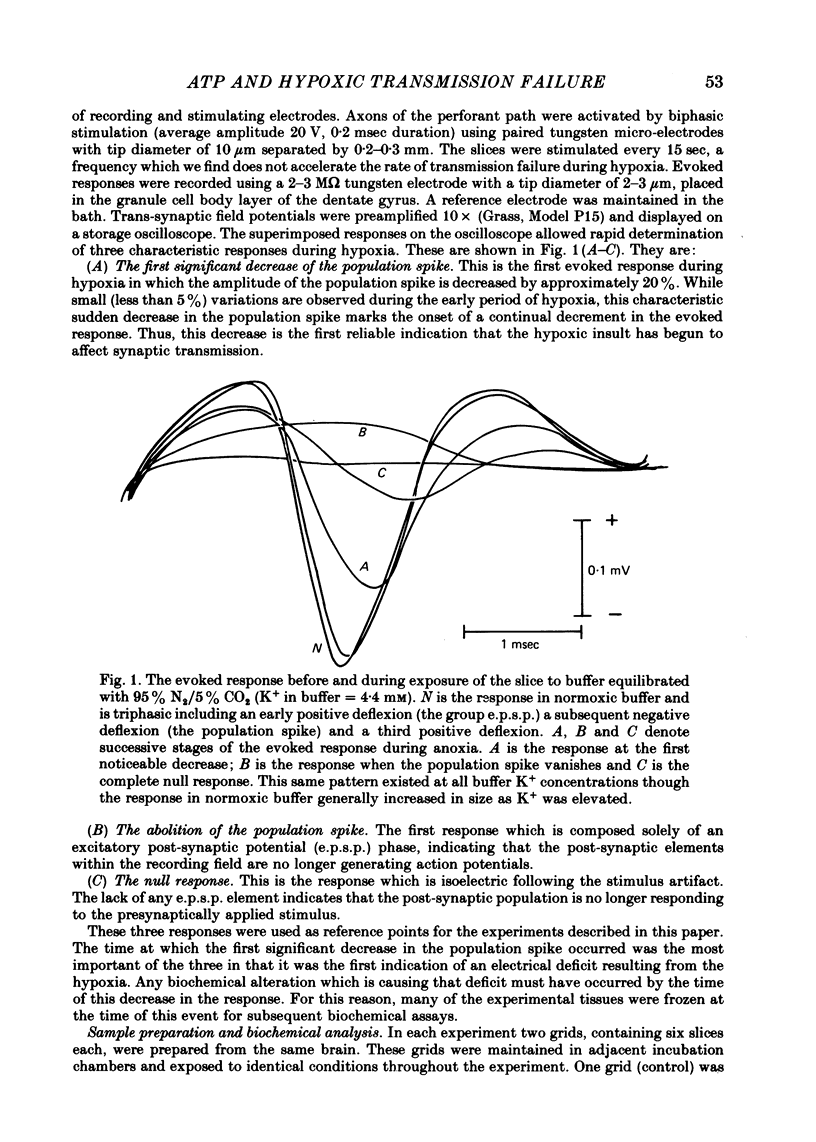
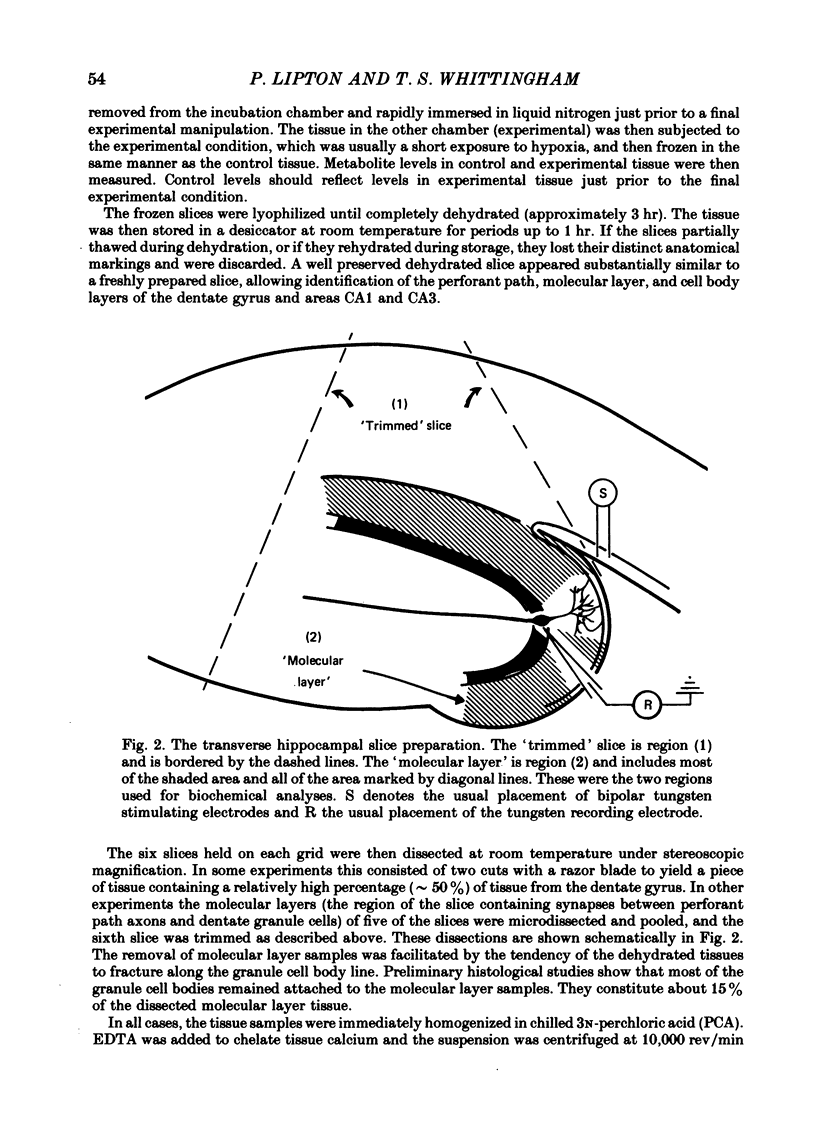
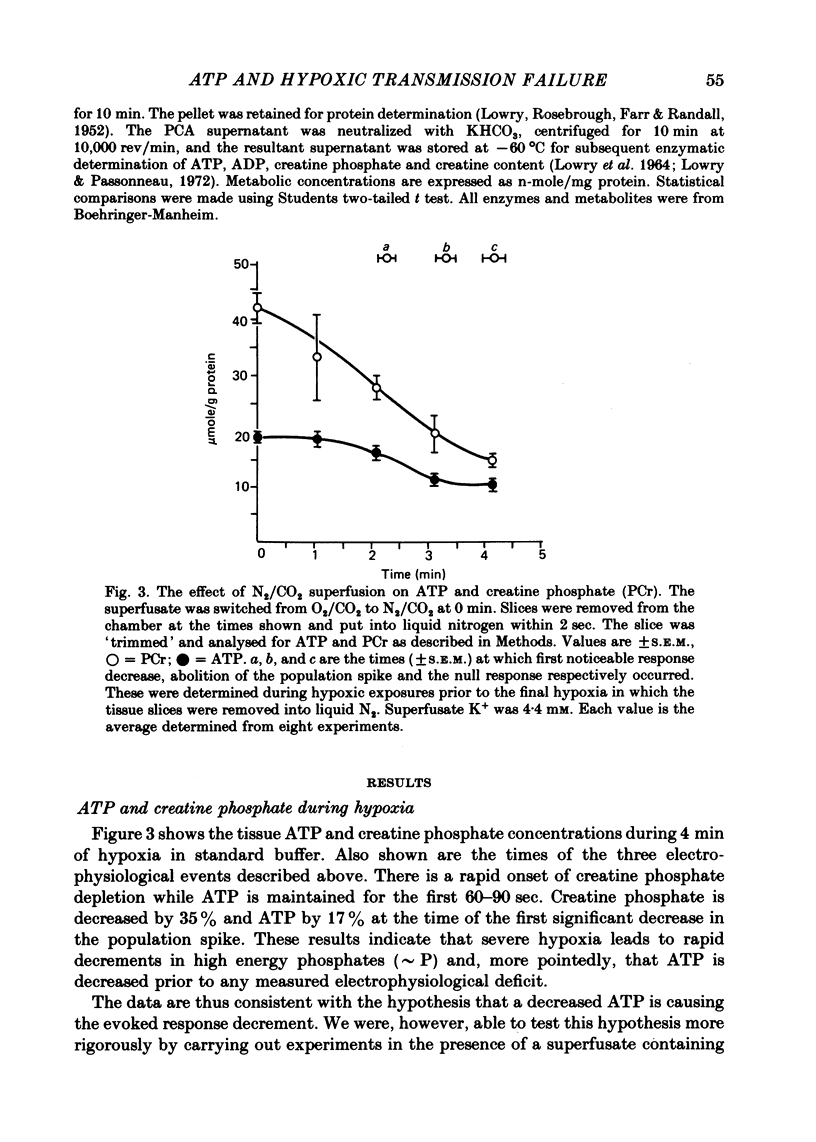
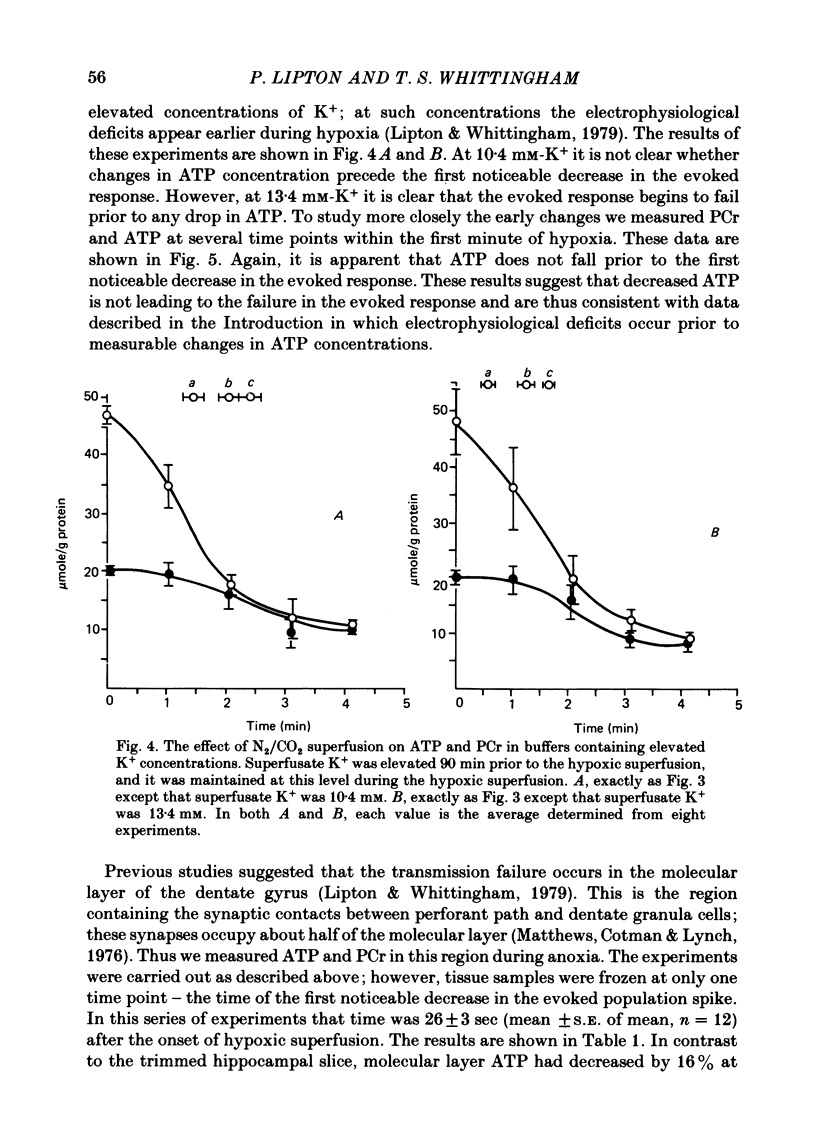
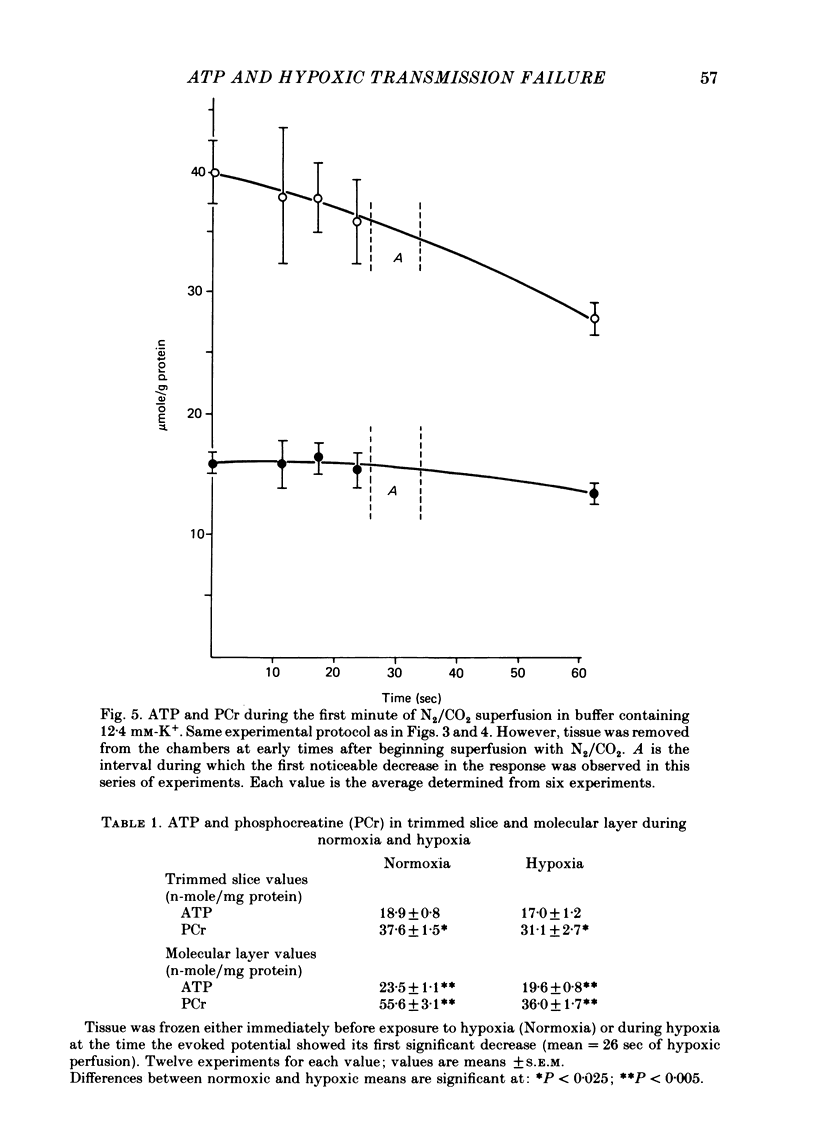
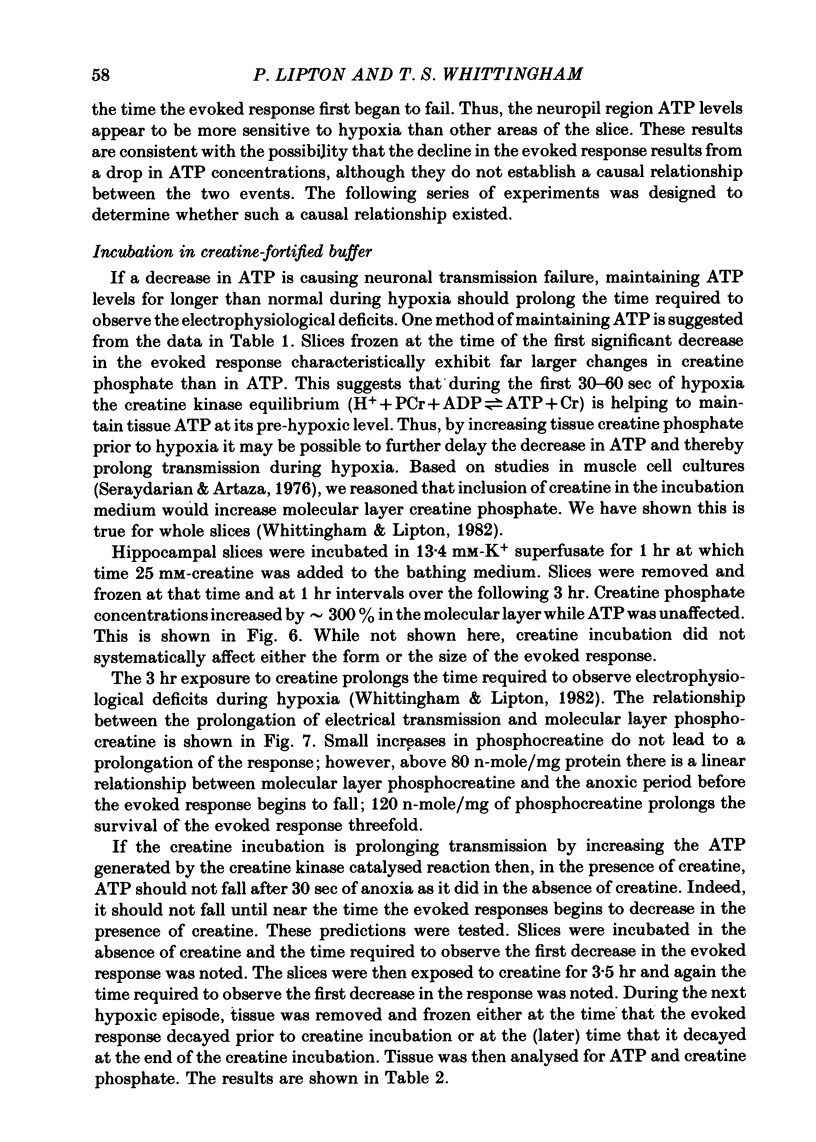
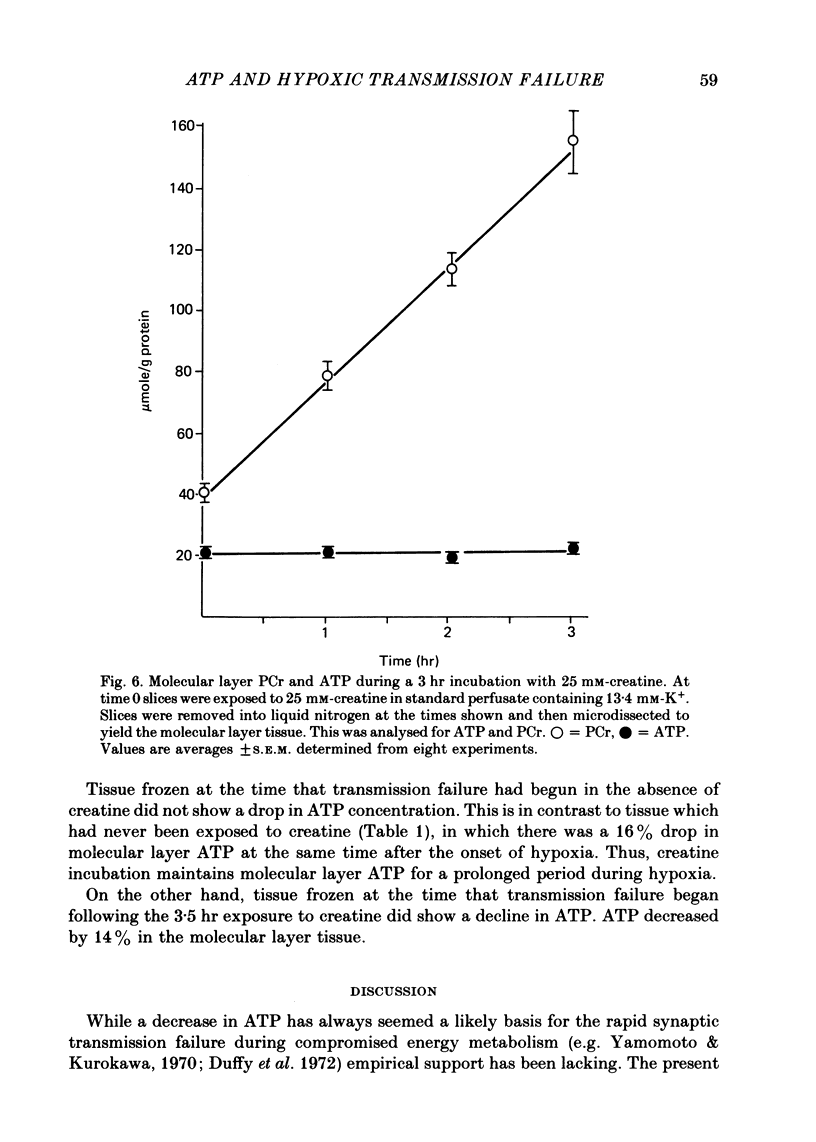
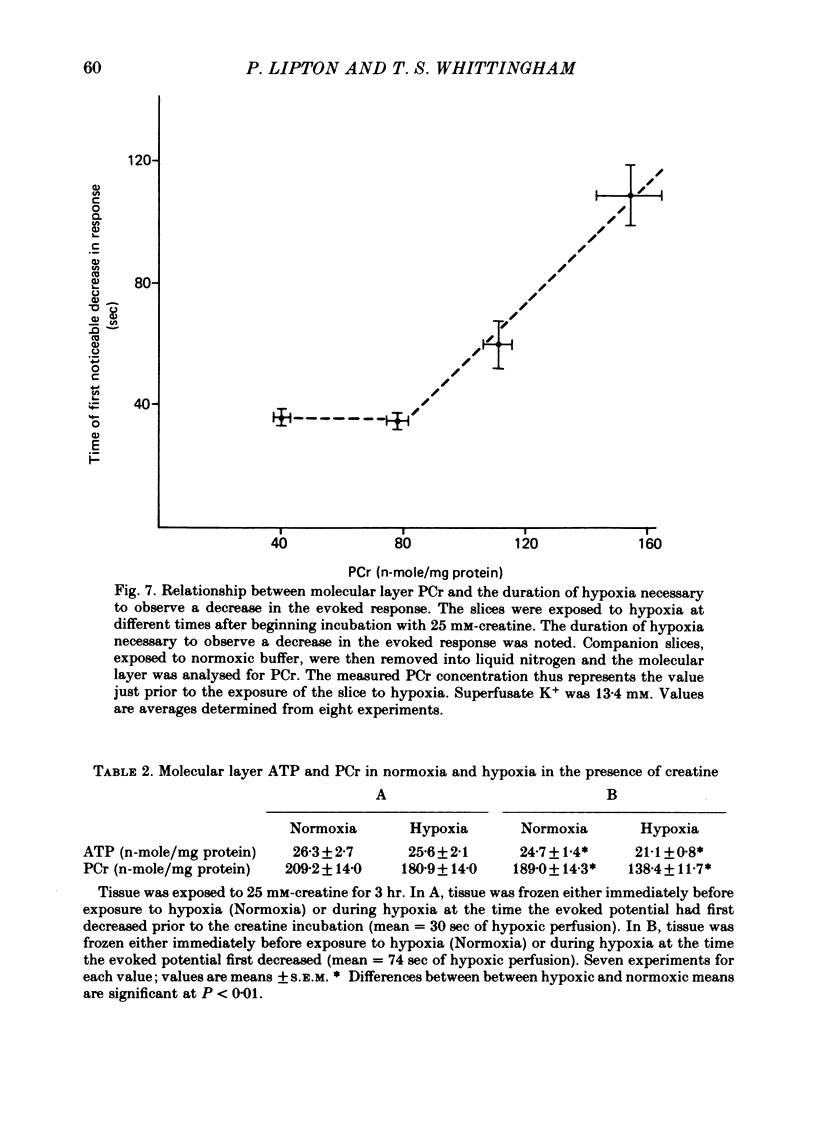
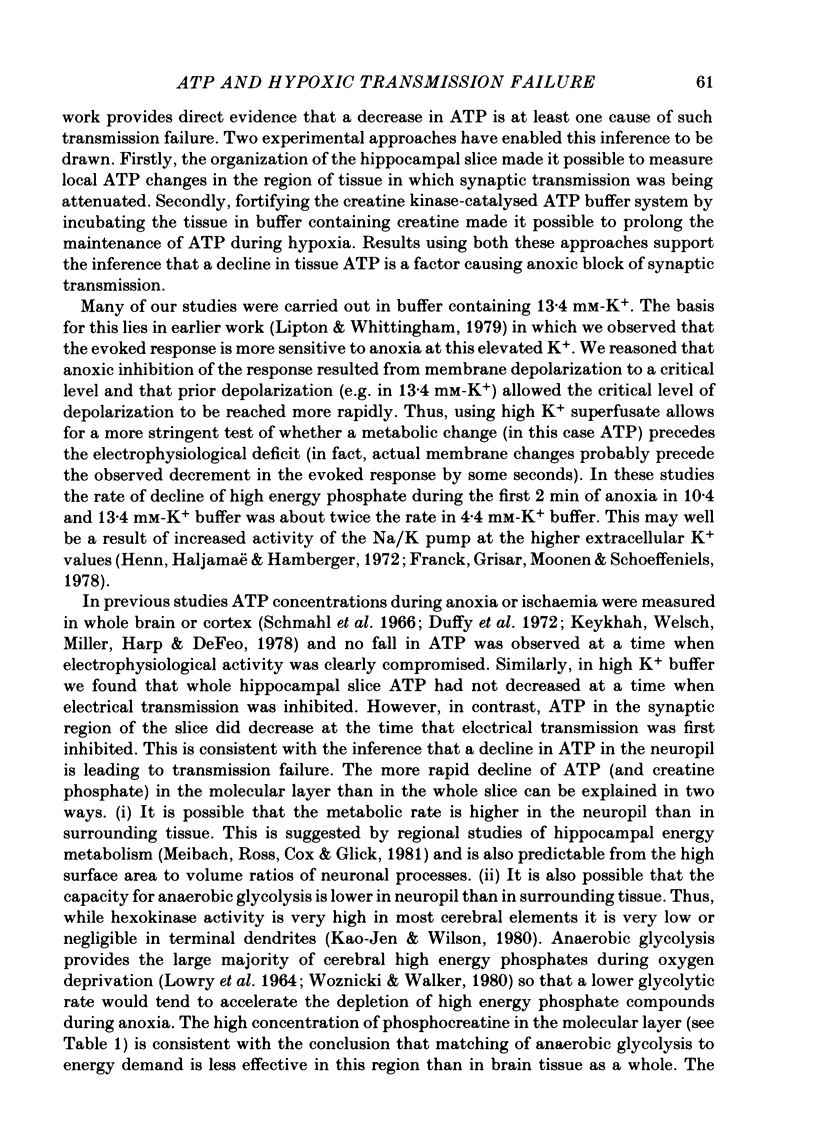
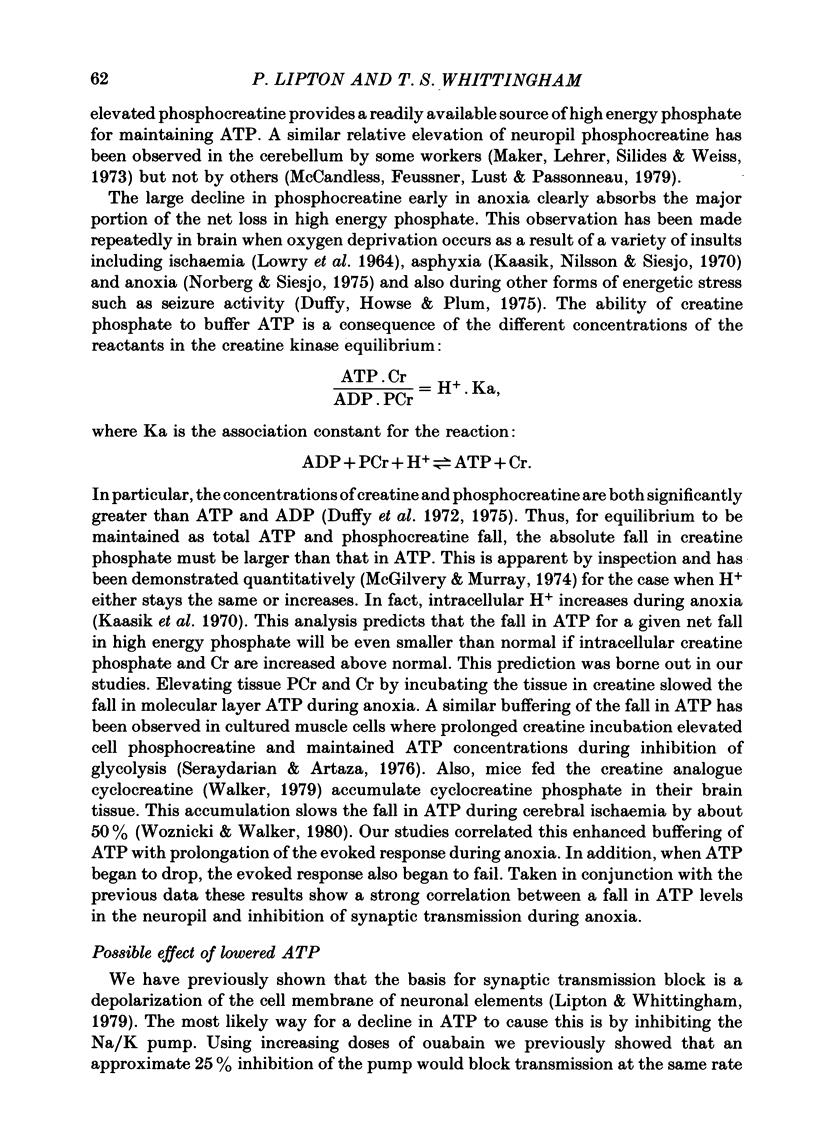
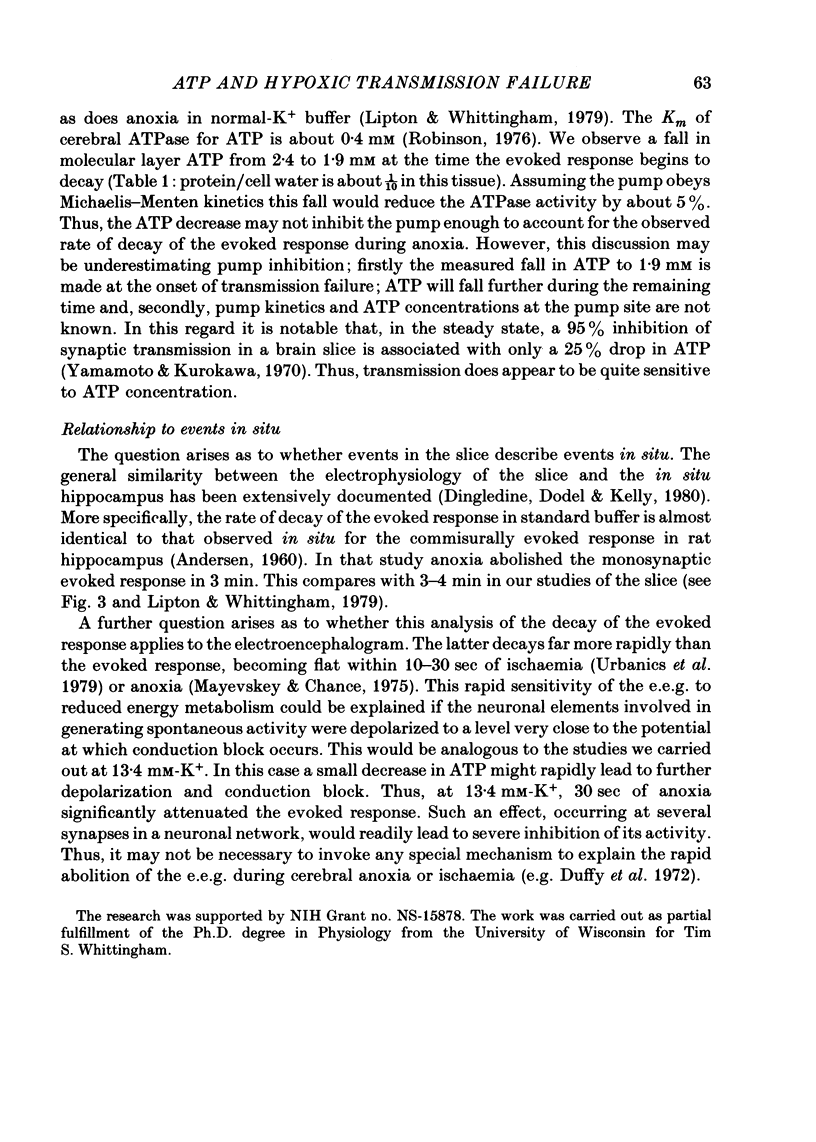
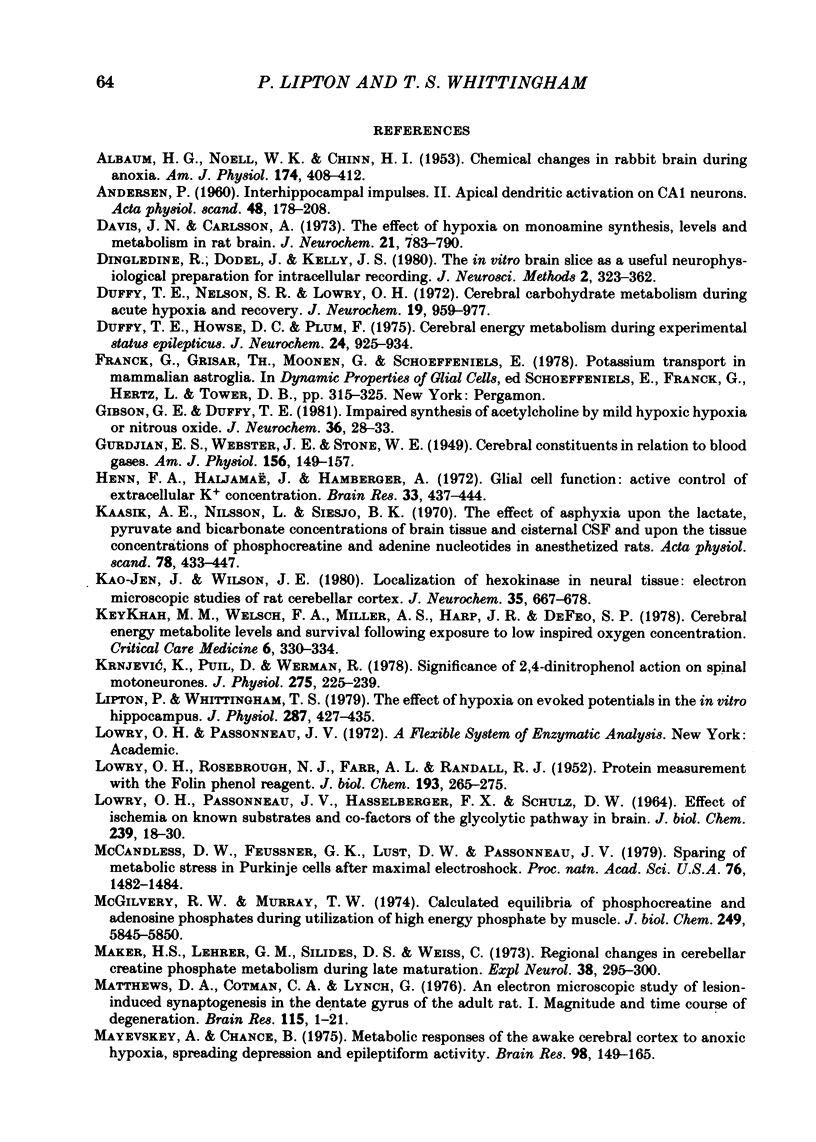
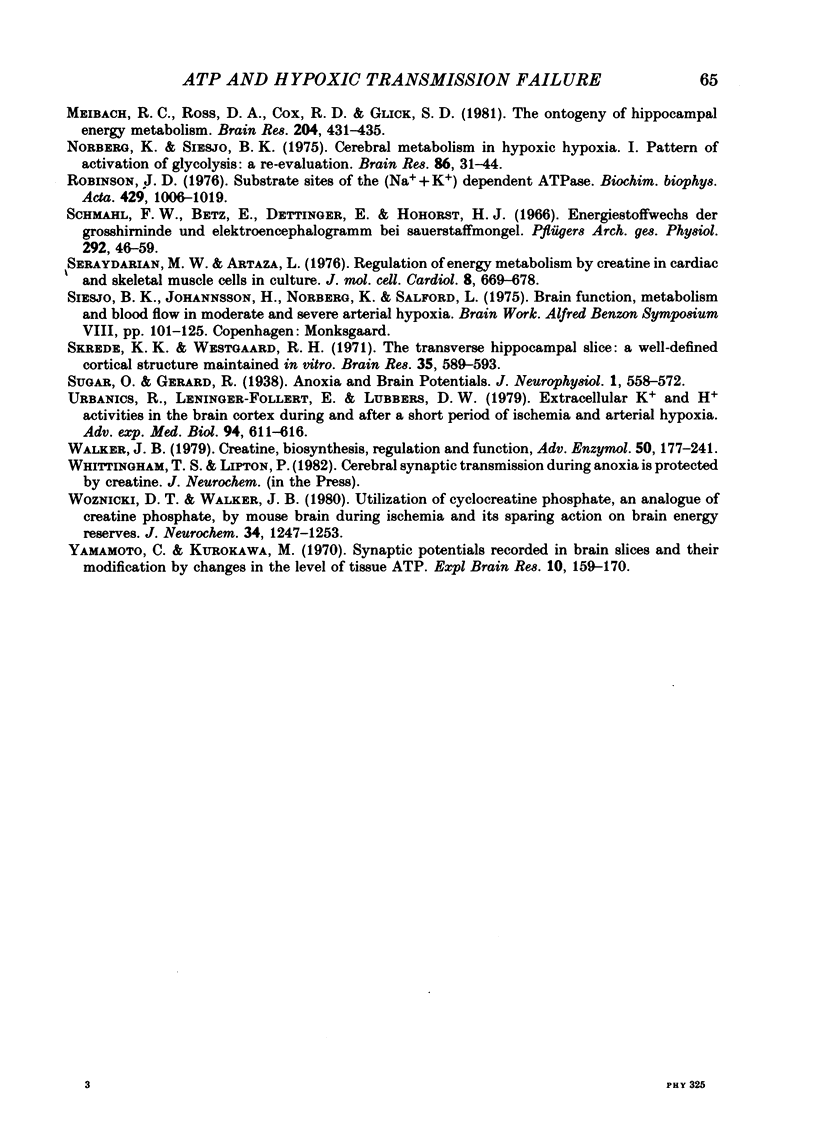
Selected References
These references are in PubMed. This may not be the complete list of references from this article.
- ALBAUM H. G., NOELL W. K., CHINN H. I. Chemical changes in rabbit brain during anoxia. Am J Physiol. 1953 Sep;174(3):408–412. doi: 10.1152/ajplegacy.1953.174.3.408. [DOI] [PubMed] [Google Scholar]
- ANDERSEN P. Interhippocampal impulses. II. Apical dendritic activation of CAI neurons. Acta Physiol Scand. 1960 Mar 18;48:178–208. doi: 10.1111/j.1748-1716.1960.tb01858.x. [DOI] [PubMed] [Google Scholar]
- Davis J. N., Carlsson A. The effect of hypoxia on monoamine synthesis, levels and metabolism in rat brain. J Neurochem. 1973 Oct;21(4):783–790. doi: 10.1111/j.1471-4159.1973.tb07522.x. [DOI] [PubMed] [Google Scholar]
- Dingledine R., Dodd J., Kelly J. S. The in vitro brain slice as a useful neurophysiological preparation for intracellular recording. J Neurosci Methods. 1980 Aug;2(4):323–362. doi: 10.1016/0165-0270(80)90002-3. [DOI] [PubMed] [Google Scholar]
- Duffy T. E., Howse D. C., Plum F. Cerebral energy metabolism during experimental status epilepticus. J Neurochem. 1975 May;24(5):925–934. doi: 10.1111/j.1471-4159.1975.tb03657.x. [DOI] [PubMed] [Google Scholar]
- Duffy T. E., Nelson S. R., Lowry O. H. Cerebral carbohydrate metabolism during acute hypoxia and recovery. J Neurochem. 1972 Apr;19(4):959–977. doi: 10.1111/j.1471-4159.1972.tb01417.x. [DOI] [PubMed] [Google Scholar]
- Gibson G. E., Duffy T. E. Impaired synthesis of acetylcholine by mild hypoxic hypoxia or nitrous oxide. J Neurochem. 1981 Jan;36(1):28–33. doi: 10.1111/j.1471-4159.1981.tb02373.x. [DOI] [PubMed] [Google Scholar]
- Henn F. A., Haljamäe H., Hamberger A. Glial cell function: active control of extracellular K + concentration. Brain Res. 1972 Aug 25;43(2):437–443. doi: 10.1016/0006-8993(72)90399-x. [DOI] [PubMed] [Google Scholar]
- Kaasik A. E., Nilsson L., Siesjö B. K. The effect of asphyxia upon the lactate, pyruvate and bicarbonate concentrations of brain tissue and cisternal CSF, and upon the tissue concentrations of phosphocreatine and adenine nucleotides in anesthetized rats. Acta Physiol Scand. 1970 Apr;78(4):433–447. doi: 10.1111/j.1748-1716.1970.tb04680.x. [DOI] [PubMed] [Google Scholar]
- Kao-Jen J., Wilson J. E. Localization of hexokinase in neural tissue: electron microscopic studies of rat cerebellar cortex. J Neurochem. 1980 Sep;35(3):667–678. doi: 10.1111/j.1471-4159.1980.tb03706.x. [DOI] [PubMed] [Google Scholar]
- Keykhah M. M., Welsh F. A., Miller A. S., Harp J. R., DeFeo S. P. Cerebral energy metabolite levels and survival following exposure to low inspired oxygen concentration. Crit Care Med. 1978 Sep-Oct;6(5):330–334. doi: 10.1097/00003246-197809000-00007. [DOI] [PubMed] [Google Scholar]
- Krnjević K., Puil E., Werman R. Significance of 2,4-dinitrophenol action on spinal motoneurones. J Physiol. 1978 Feb;275:225–239. doi: 10.1113/jphysiol.1978.sp012187. [DOI] [PMC free article] [PubMed] [Google Scholar]
- LOWRY O. H., PASSONNEAU J. V., HASSELBERGER F. X., SCHULZ D. W. EFFECT OF ISCHEMIA ON KNOWN SUBSTRATES AND COFACTORS OF THE GLYCOLYTIC PATHWAY IN BRAIN. J Biol Chem. 1964 Jan;239:18–30. [PubMed] [Google Scholar]
- LOWRY O. H., ROSEBROUGH N. J., FARR A. L., RANDALL R. J. Protein measurement with the Folin phenol reagent. J Biol Chem. 1951 Nov;193(1):265–275. [PubMed] [Google Scholar]
- Lipton P., Whittingham T. S. The effect of hypoxia on evoked potentials in the in vitro hippocampus. J Physiol. 1979 Feb;287:427–438. doi: 10.1113/jphysiol.1979.sp012668. [DOI] [PMC free article] [PubMed] [Google Scholar]
- Maker H. S., Lehrer G. M., Silides D. J., Weiss C. Regional changes in cerebellar creatine phosphate metabolism during late maturation. Exp Neurol. 1973 Feb;38(2):295–300. doi: 10.1016/0014-4886(73)90153-2. [DOI] [PubMed] [Google Scholar]
- Matthews D. A., Cotman C., Lynch G. An electron microscopic study of lesion-induced synaptogenesis in the dentate gyrus of the adult rat. I. Magnitude and time course of degeneration. Brain Res. 1976 Oct 8;115(1):1–21. doi: 10.1016/0006-8993(76)90819-2. [DOI] [PubMed] [Google Scholar]
- Mayevsky A., Chance B. Metabolic responses of the awake cerebral cortex to anoxia hypoxia spreading depression and epileptiform activity. Brain Res. 1975 Nov 7;98(1):149–165. doi: 10.1016/0006-8993(75)90515-6. [DOI] [PubMed] [Google Scholar]
- McCandless D. W., Feussner G. K., Lust W. D., Passonneau J. V. Sparing of metabolic stress in Purkinje cells after maximal electroshock. Proc Natl Acad Sci U S A. 1979 Mar;76(3):1482–1484. doi: 10.1073/pnas.76.3.1482. [DOI] [PMC free article] [PubMed] [Google Scholar]
- McGilvery R. W., Murray T. W. Calculated equilibria of phosphocreatine and adenosine phosphates during utilization of high energy phosphate by muscle. J Biol Chem. 1974 Sep 25;249(18):5845–5850. [PubMed] [Google Scholar]
- Meibach R. C., Ross D. A., Cox R. D., Glick S. D. The ontogeny of hippocampal energy metabolism. Brain Res. 1981 Jan 12;204(2):431–435. doi: 10.1016/0006-8993(81)90603-x. [DOI] [PubMed] [Google Scholar]
- Norberg K., Siesjö B. K. Cerebral metabolism in hypoxic hypoxia. I. Pattern of activation of glycolysis: a re-evaluation. Brain Res. 1975 Mar 14;86(1):31–44. doi: 10.1016/0006-8993(75)90635-6. [DOI] [PubMed] [Google Scholar]
- Robinson J. D. Substrate sites for the (Na+ + K+)-dependent ATPase. Biochim Biophys Acta. 1976 May 13;429(3):1006–1019. doi: 10.1016/0005-2744(76)90345-4. [DOI] [PubMed] [Google Scholar]
- Schmahl F. W., Betz E., Dettinger E., Hohorst H. J. Energiestoffwechsel der Grosshirnrinde und Elektrencephalogramm bei Sauerstoffmangel. Pflugers Arch Gesamte Physiol Menschen Tiere. 1966;292(1):46–59. [PubMed] [Google Scholar]
- Seraydarian M. W., Artaza L. Regulation of energy metabolism by creatine in cardiac and skeletal muscle cells in culture. J Mol Cell Cardiol. 1976 Sep;08(9):669–678. doi: 10.1016/0022-2828(76)90009-2. [DOI] [PubMed] [Google Scholar]
- Skrede K. K., Westgaard R. H. The transverse hippocampal slice: a well-defined cortical structure maintained in vitro. Brain Res. 1971 Dec 24;35(2):589–593. doi: 10.1016/0006-8993(71)90508-7. [DOI] [PubMed] [Google Scholar]
- Urbanics R., Leniger-Follert E., Lübbers D. W. Extracellular K+ and H+ activities in the brain cortex during and after a short period of ischaemia and arterial hypoxia. Adv Exp Med Biol. 1977 Jul 4;94:611–616. doi: 10.1007/978-1-4684-8890-6_83. [DOI] [PubMed] [Google Scholar]
- Walker J. B. Creatine: biosynthesis, regulation, and function. Adv Enzymol Relat Areas Mol Biol. 1979;50:177–242. doi: 10.1002/9780470122952.ch4. [DOI] [PubMed] [Google Scholar]
- Woznicki D. T., Walker J. B. Utilization of cyclocreatine phosphate, and analogue of creatine phosphate, by mouse brain during ischemia and its sparing action on brain energy reserves. J Neurochem. 1980 May;34(5):1247–1253. doi: 10.1111/j.1471-4159.1980.tb09966.x. [DOI] [PubMed] [Google Scholar]
- Yamamoto C., Kurokawa M. Synaptic potentials recorded in brain slices and their modification by changes in the level of tissue ATP. Exp Brain Res. 1970;10(2):159–170. doi: 10.1007/BF00234729. [DOI] [PubMed] [Google Scholar]