Abstract
The effects of ionic strength (10-1,000 mM) on the gating of batrachotoxin-activated rat brain sodium channels were studied in neutral and in negatively charged lipid bilayers. In neutral bilayers, increasing the ionic strength of the extracellular solution, shifted the voltage dependence of the open probability (gating curve) of the sodium channel to more positive membrane potentials. On the other hand, increasing the intracellular ionic strength shifted the gating curve to more negative membrane potentials. Ionic strength shifted the voltage dependence of both opening and closing rate constants of the channel in analogous ways to its effects on gating curves. The voltage sensitivities of the rate constants were not affected by ionic strength. The effects of ionic strength on the gating of sodium channels reconstituted in negatively charged bilayers were qualitatively the same as in neutral bilayers. However, important quantitative differences were noticed: in low ionic strength conditions (10-150 mM), the presence of negative charges on the membrane surface induced an extra voltage shift on the gating curve of sodium channels in relation to neutral bilayers. It is concluded that: (a) asymmetric negative surface charge densities in the extracellular (1e-/533A2) and intracellular (1e-/1,231A2) sides of the sodium channel could explain the voltage shifts caused by ionic strength on the gating curve of the channel in neutral bilayers. These surface charges create negative electric fields in both the extracellular and intracellular sides of the channel. Said electric fields interfere with gating charge movements that occur during the opening and closing of sodium channels; (b) the voltage shifts caused by ionic strength on the gating curve of sodium channels can be accounted by voltage shifts in both the opening and closing rate constants; (c) net negative surface charges on the channel's molecule do not affect the intrinsic gating properties of sodium channels but are essential in determining the relative position of the channel's gating curve; (d) provided the ionic strength is below 150 mM, the gating machinery of the sodium channel molecule is able to sense the electric field created by surface changes on the lipid membrane. I propose that during the opening and closing of sodium channels, the gating charges involved in this process are asymmetrically displaced in relation to the plane of the bilayer. Simple electrostatic calculations suggest that gating charge movements are influenced by membrane electrostatic potentials at distances of 48 and 28 A away from the plane of the membrane in the extracellular sides of the channel, respectively.
Full text
PDF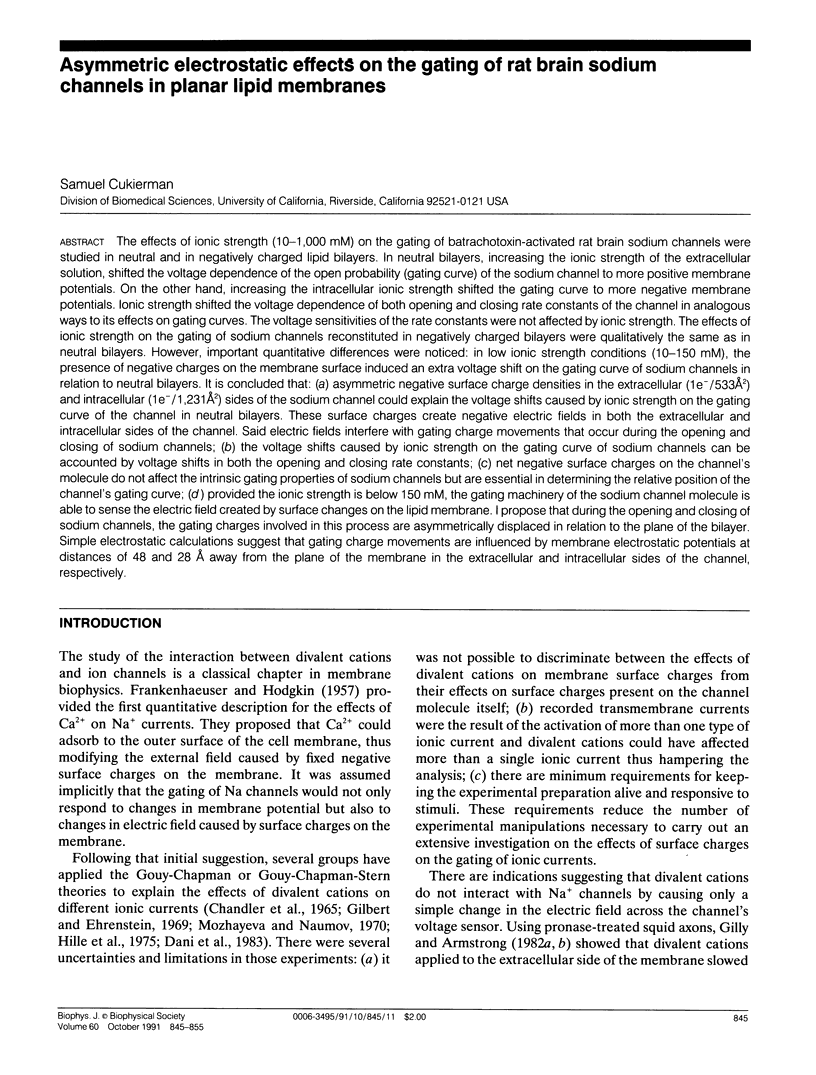
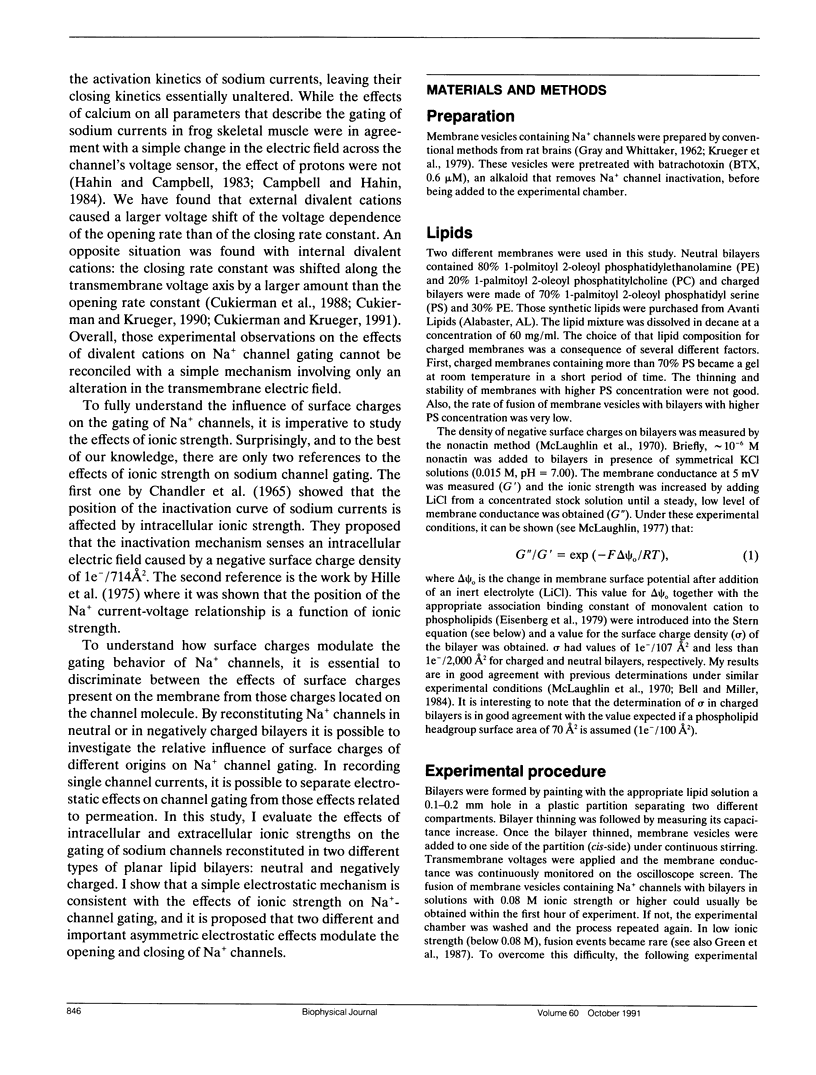
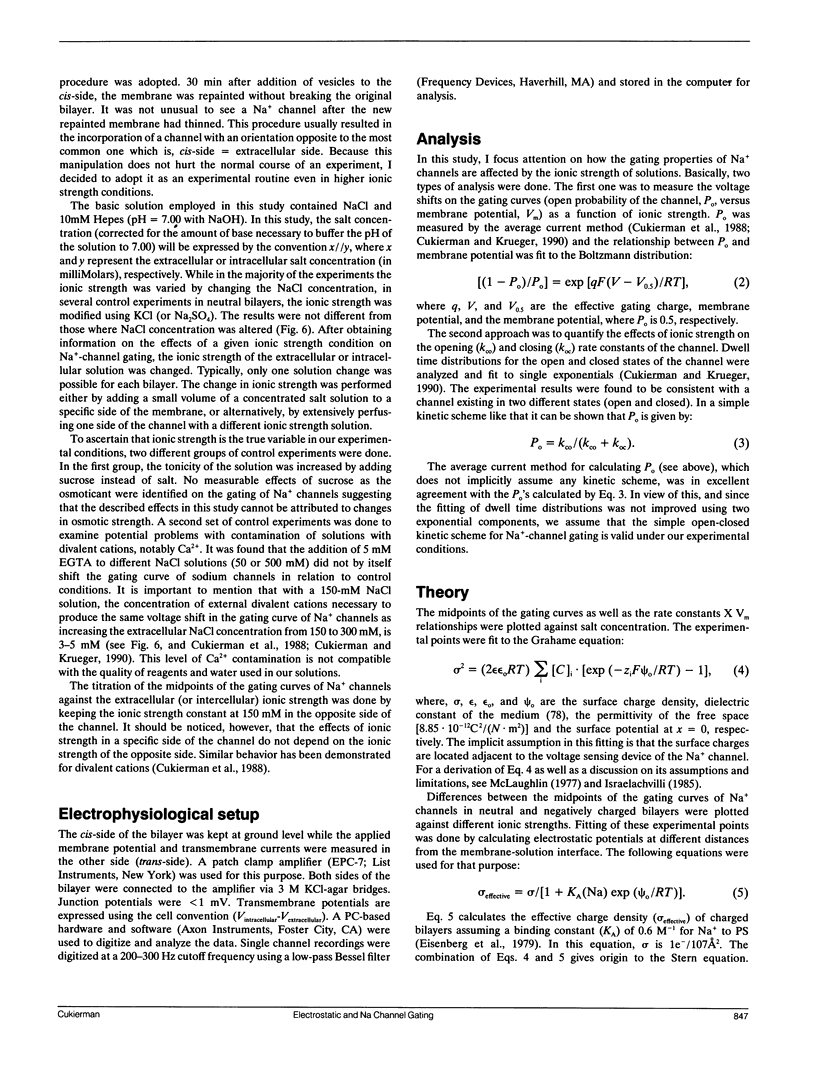
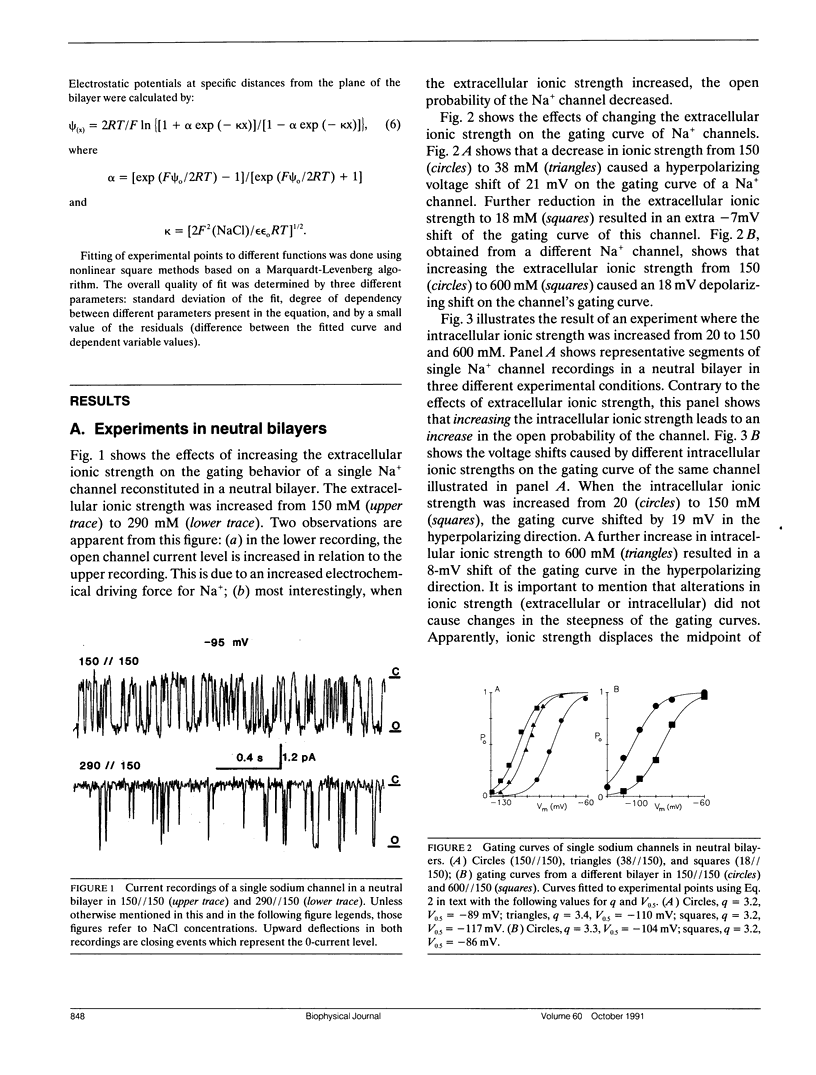
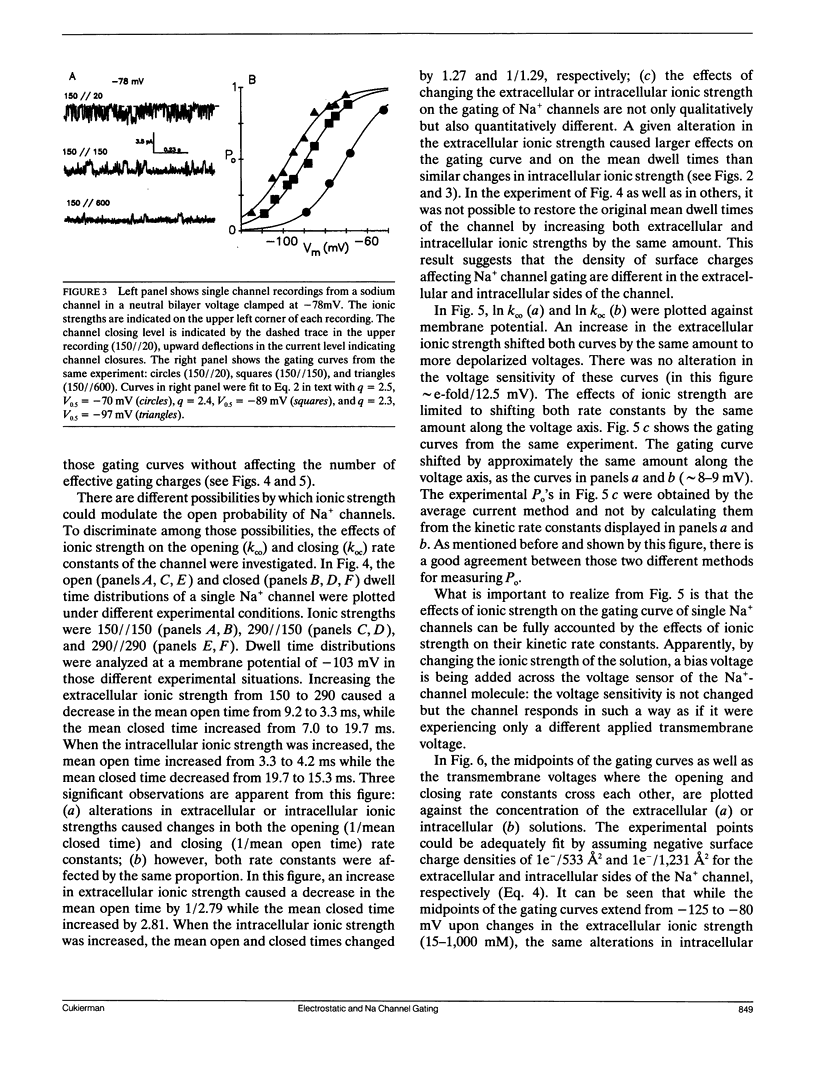
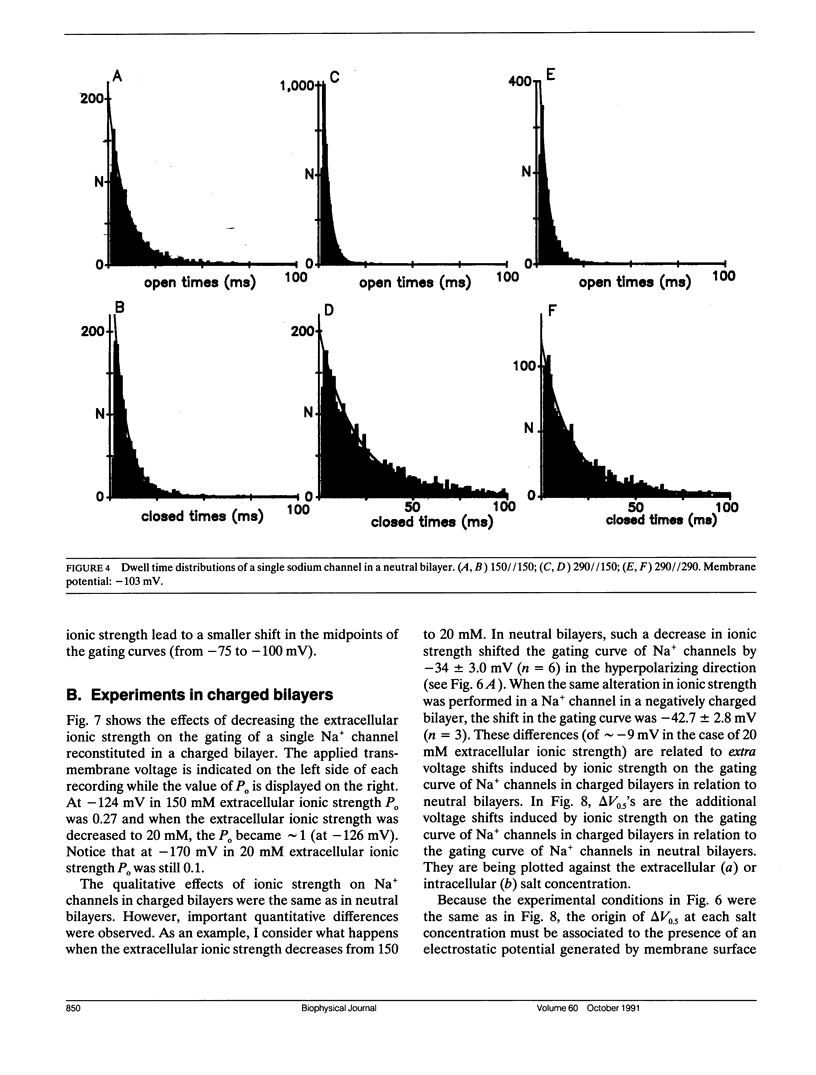
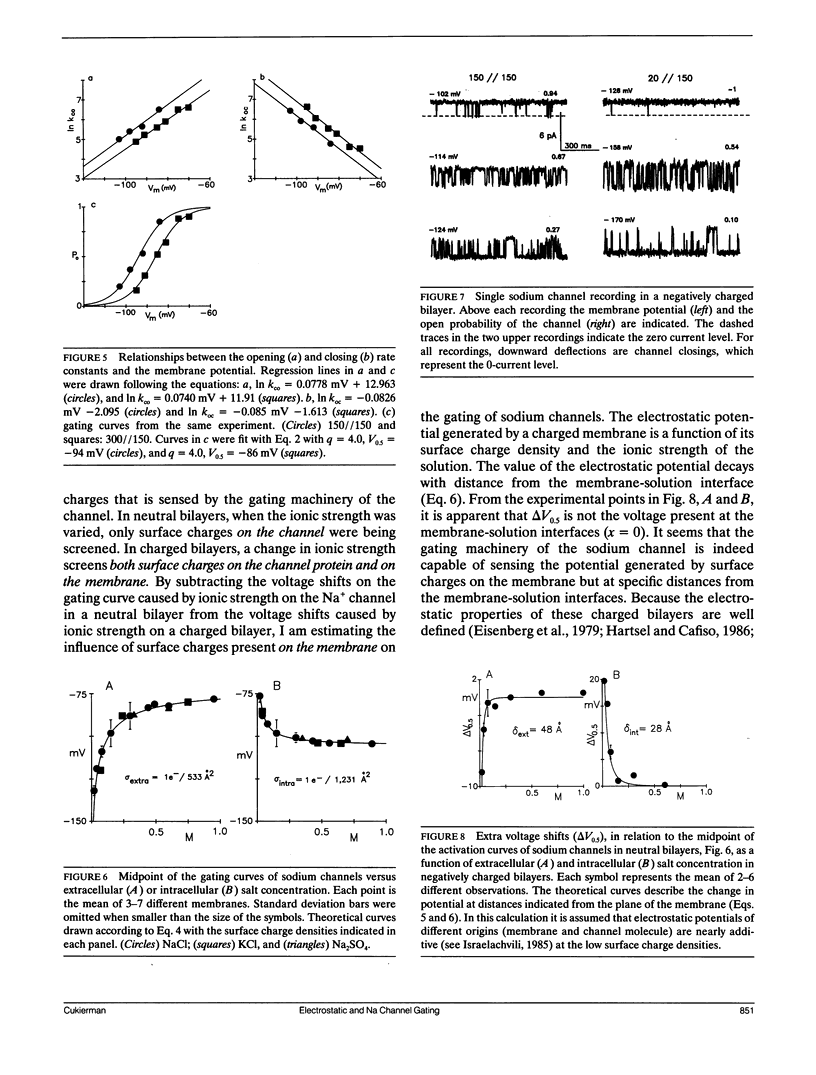
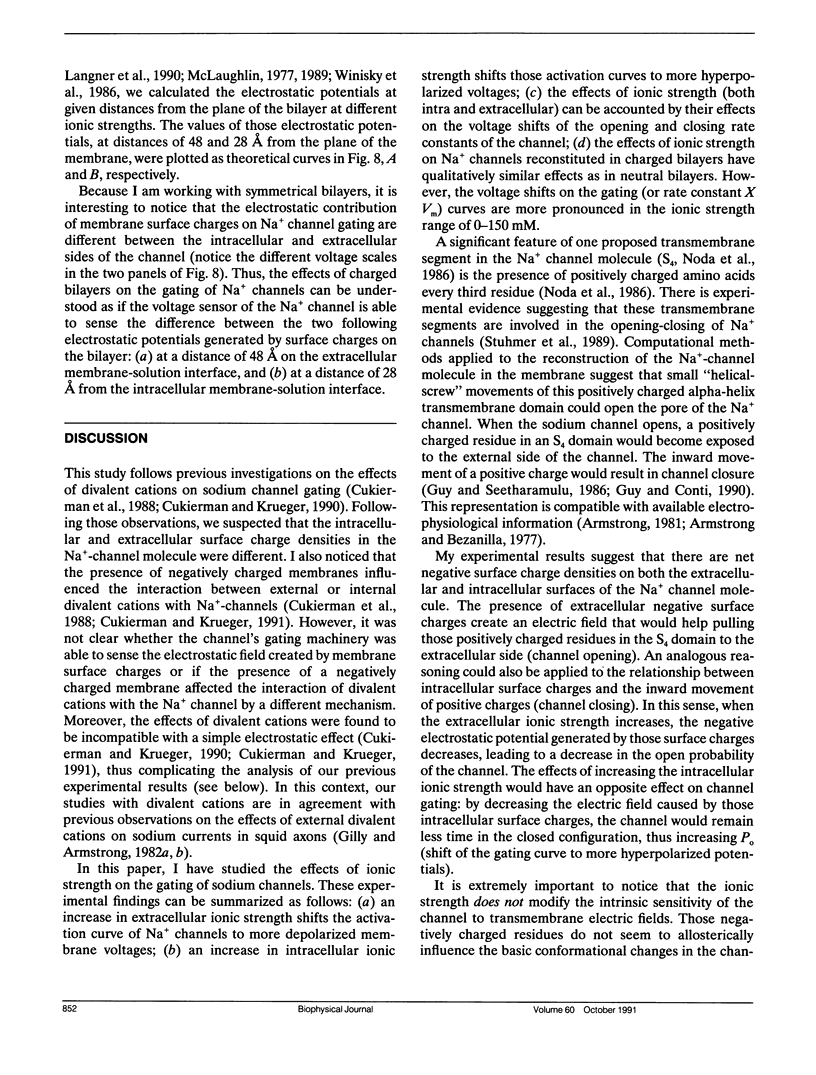
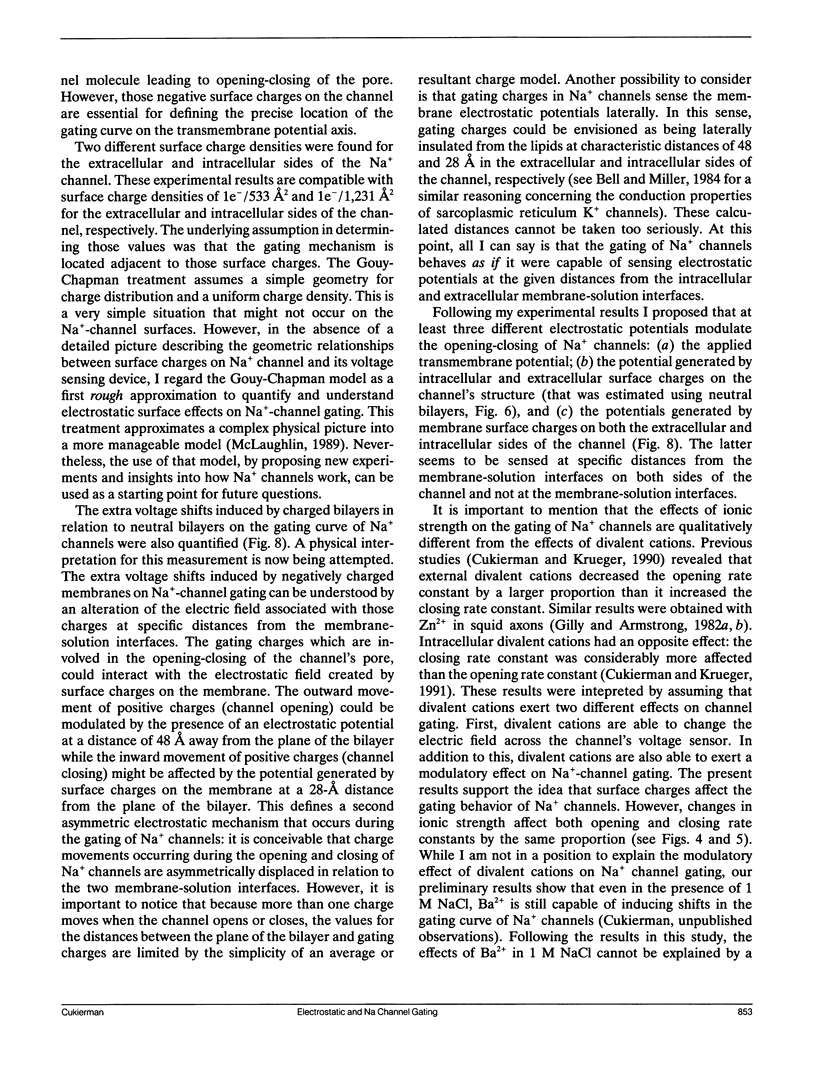
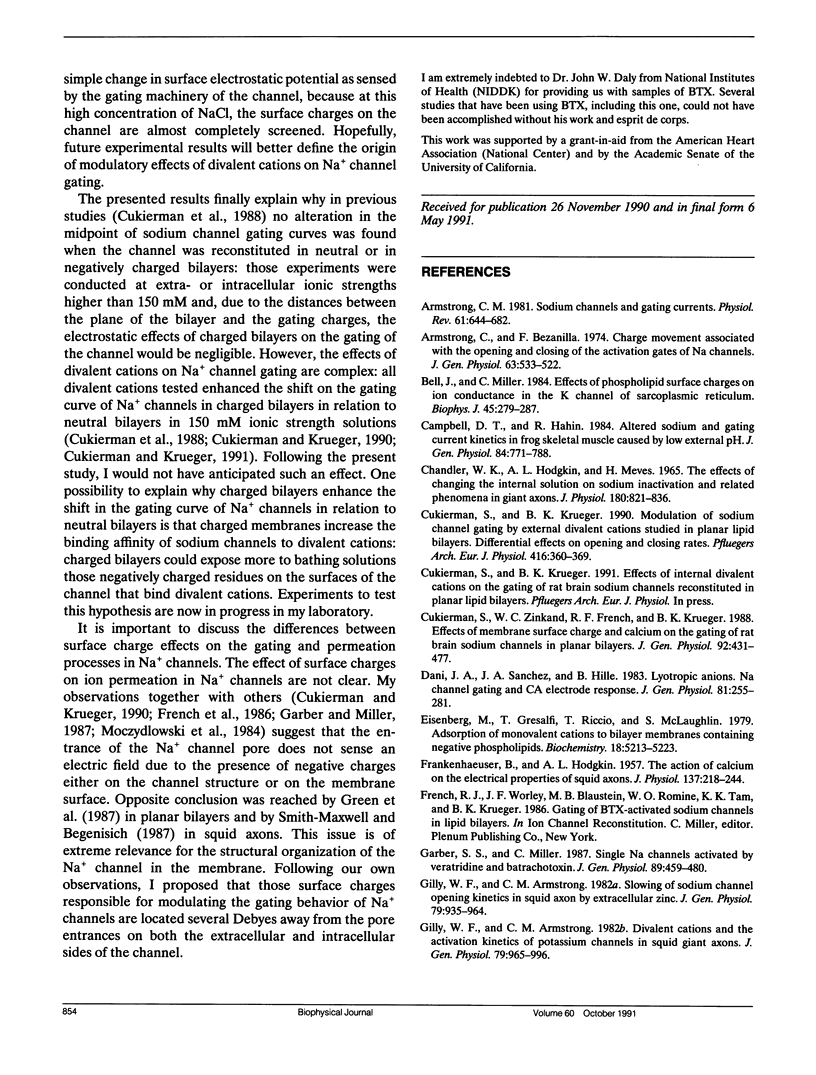
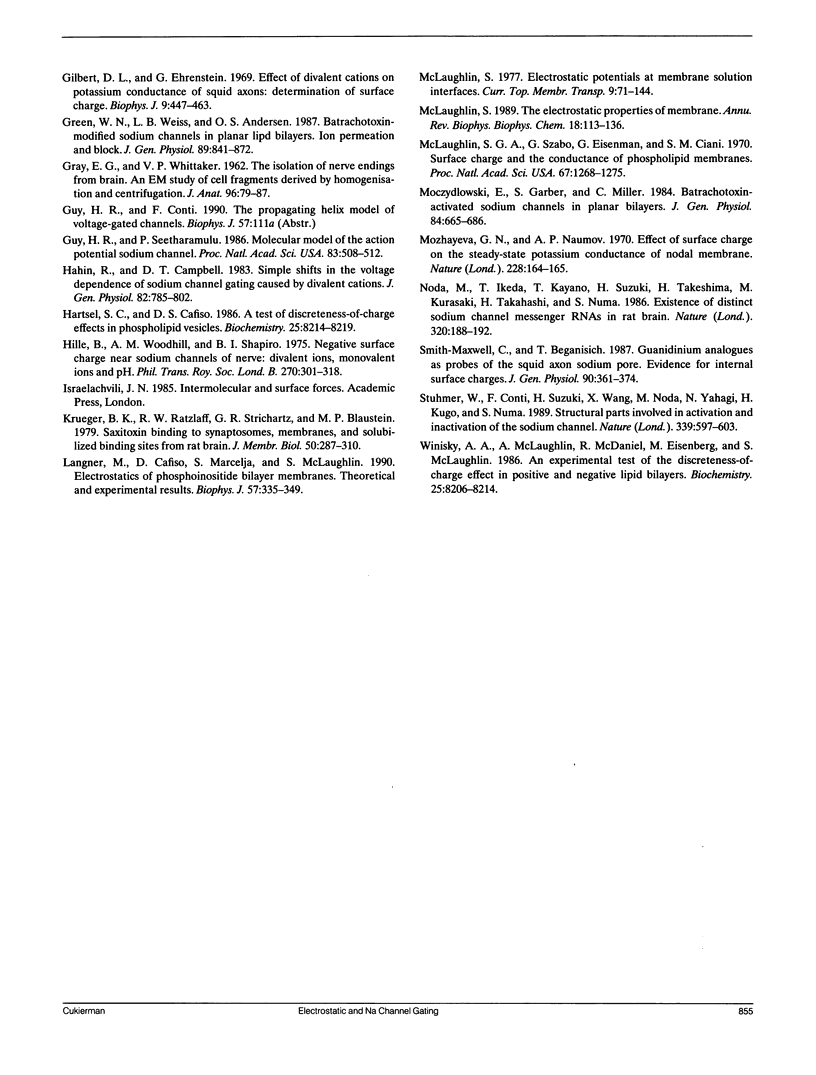
Selected References
These references are in PubMed. This may not be the complete list of references from this article.
- Armstrong C. M., Bezanilla F. Charge movement associated with the opening and closing of the activation gates of the Na channels. J Gen Physiol. 1974 May;63(5):533–552. doi: 10.1085/jgp.63.5.533. [DOI] [PMC free article] [PubMed] [Google Scholar]
- Armstrong C. M. Sodium channels and gating currents. Physiol Rev. 1981 Jul;61(3):644–683. doi: 10.1152/physrev.1981.61.3.644. [DOI] [PubMed] [Google Scholar]
- Bell J. E., Miller C. Effects of phospholipid surface charge on ion conduction in the K+ channel of sarcoplasmic reticulum. Biophys J. 1984 Jan;45(1):279–287. doi: 10.1016/S0006-3495(84)84154-5. [DOI] [PMC free article] [PubMed] [Google Scholar]
- Campbell D. T., Hahin R. Altered sodium and gating current kinetics in frog skeletal muscle caused by low external pH. J Gen Physiol. 1984 Nov;84(5):771–788. doi: 10.1085/jgp.84.5.771. [DOI] [PMC free article] [PubMed] [Google Scholar]
- Chandler W. K., Hodgkin A. L., Meves H. The effect of changing the internal solution on sodium inactivation and related phenomena in giant axons. J Physiol. 1965 Oct;180(4):821–836. doi: 10.1113/jphysiol.1965.sp007733. [DOI] [PMC free article] [PubMed] [Google Scholar]
- Cukierman S., Krueger B. K. Modulation of sodium channel gating by external divalent cations: differential effects on opening and closing rates. Pflugers Arch. 1990 Jun;416(4):360–367. doi: 10.1007/BF00370741. [DOI] [PubMed] [Google Scholar]
- Cukierman S., Zinkand W. C., French R. J., Krueger B. K. Effects of membrane surface charge and calcium on the gating of rat brain sodium channels in planar bilayers. J Gen Physiol. 1988 Oct;92(4):431–447. doi: 10.1085/jgp.92.4.431. [DOI] [PMC free article] [PubMed] [Google Scholar]
- Dani J. A., Sanchez J. A., Hille B. Lyotropic anions. Na channel gating and Ca electrode response. J Gen Physiol. 1983 Feb;81(2):255–281. doi: 10.1085/jgp.81.2.255. [DOI] [PMC free article] [PubMed] [Google Scholar]
- Eisenberg M., Gresalfi T., Riccio T., McLaughlin S. Adsorption of monovalent cations to bilayer membranes containing negative phospholipids. Biochemistry. 1979 Nov 13;18(23):5213–5223. doi: 10.1021/bi00590a028. [DOI] [PubMed] [Google Scholar]
- FRANKENHAEUSER B., HODGKIN A. L. The action of calcium on the electrical properties of squid axons. J Physiol. 1957 Jul 11;137(2):218–244. doi: 10.1113/jphysiol.1957.sp005808. [DOI] [PMC free article] [PubMed] [Google Scholar]
- GRAY E. G., WHITTAKER V. P. The isolation of nerve endings from brain: an electron-microscopic study of cell fragments derived by homogenization and centrifugation. J Anat. 1962 Jan;96:79–88. [PMC free article] [PubMed] [Google Scholar]
- Garber S. S., Miller C. Single Na+ channels activated by veratridine and batrachotoxin. J Gen Physiol. 1987 Mar;89(3):459–480. doi: 10.1085/jgp.89.3.459. [DOI] [PMC free article] [PubMed] [Google Scholar]
- Gilbert D. L., Ehrenstein G. Effect of divalent cations on potassium conductance of squid axons: determination of surface charge. Biophys J. 1969 Mar;9(3):447–463. doi: 10.1016/S0006-3495(69)86396-4. [DOI] [PMC free article] [PubMed] [Google Scholar]
- Gilly W. F., Armstrong C. M. Divalent cations and the activation kinetics of potassium channels in squid giant axons. J Gen Physiol. 1982 Jun;79(6):965–996. doi: 10.1085/jgp.79.6.965. [DOI] [PMC free article] [PubMed] [Google Scholar]
- Gilly W. F., Armstrong C. M. Slowing of sodium channel opening kinetics in squid axon by extracellular zinc. J Gen Physiol. 1982 Jun;79(6):935–964. doi: 10.1085/jgp.79.6.935. [DOI] [PMC free article] [PubMed] [Google Scholar]
- Green W. N., Weiss L. B., Andersen O. S. Batrachotoxin-modified sodium channels in planar lipid bilayers. Ion permeation and block. J Gen Physiol. 1987 Jun;89(6):841–872. doi: 10.1085/jgp.89.6.841. [DOI] [PMC free article] [PubMed] [Google Scholar]
- Guy H. R., Seetharamulu P. Molecular model of the action potential sodium channel. Proc Natl Acad Sci U S A. 1986 Jan;83(2):508–512. doi: 10.1073/pnas.83.2.508. [DOI] [PMC free article] [PubMed] [Google Scholar]
- Hahin R., Campbell D. T. Simple shifts in the voltage dependence of sodium channel gating caused by divalent cations. J Gen Physiol. 1983 Dec;82(6):785–805. doi: 10.1085/jgp.82.6.785. [DOI] [PMC free article] [PubMed] [Google Scholar]
- Hartsel S. C., Cafiso D. S. A test of discreteness-of-charge effects in phospholipid vesicles: measurements using paramagnetic amphiphiles. Biochemistry. 1986 Dec 16;25(25):8214–8219. doi: 10.1021/bi00373a014. [DOI] [PubMed] [Google Scholar]
- Hille B., Woodhull A. M., Shapiro B. I. Negative surface charge near sodium channels of nerve: divalent ions, monovalent ions, and pH. Philos Trans R Soc Lond B Biol Sci. 1975 Jun 10;270(908):301–318. doi: 10.1098/rstb.1975.0011. [DOI] [PubMed] [Google Scholar]
- Krueger B. K., Ratzlaff R. W., Strichartz G. R., Blaustein M. P. Saxitoxin binding to synaptosomes, membranes, and solubilized binding sites from rat brain. J Membr Biol. 1979 Nov 30;50(3-4):287–310. doi: 10.1007/BF01868894. [DOI] [PubMed] [Google Scholar]
- Langner M., Cafiso D., Marcelja S., McLaughlin S. Electrostatics of phosphoinositide bilayer membranes. Theoretical and experimental results. Biophys J. 1990 Feb;57(2):335–349. doi: 10.1016/S0006-3495(90)82535-2. [DOI] [PMC free article] [PubMed] [Google Scholar]
- McLaughlin S. G., Szabo G., Eisenman G., Ciani S. M. Surface charge and the conductance of phospholipid membranes. Proc Natl Acad Sci U S A. 1970 Nov;67(3):1268–1275. doi: 10.1073/pnas.67.3.1268. [DOI] [PMC free article] [PubMed] [Google Scholar]
- McLaughlin S. The electrostatic properties of membranes. Annu Rev Biophys Biophys Chem. 1989;18:113–136. doi: 10.1146/annurev.bb.18.060189.000553. [DOI] [PubMed] [Google Scholar]
- Moczydlowski E., Garber S. S., Miller C. Batrachotoxin-activated Na+ channels in planar lipid bilayers. Competition of tetrodotoxin block by Na+. J Gen Physiol. 1984 Nov;84(5):665–686. doi: 10.1085/jgp.84.5.665. [DOI] [PMC free article] [PubMed] [Google Scholar]
- Mozhayeva G. N., Naumov A. P. Effect of surface charge on the steady-state potassium conductance of nodal membrane. Nature. 1970 Oct 10;228(5267):164–165. doi: 10.1038/228164a0. [DOI] [PubMed] [Google Scholar]
- Noda M., Ikeda T., Kayano T., Suzuki H., Takeshima H., Kurasaki M., Takahashi H., Numa S. Existence of distinct sodium channel messenger RNAs in rat brain. Nature. 1986 Mar 13;320(6058):188–192. doi: 10.1038/320188a0. [DOI] [PubMed] [Google Scholar]
- Smith-Maxwell C., Begenisich T. Guanidinium analogues as probes of the squid axon sodium pore. Evidence for internal surface charges. J Gen Physiol. 1987 Sep;90(3):361–374. doi: 10.1085/jgp.90.3.361. [DOI] [PMC free article] [PubMed] [Google Scholar]
- Stühmer W., Conti F., Suzuki H., Wang X. D., Noda M., Yahagi N., Kubo H., Numa S. Structural parts involved in activation and inactivation of the sodium channel. Nature. 1989 Jun 22;339(6226):597–603. doi: 10.1038/339597a0. [DOI] [PubMed] [Google Scholar]
- Winiski A. P., McLaughlin A. C., McDaniel R. V., Eisenberg M., McLaughlin S. An experimental test of the discreteness-of-charge effect in positive and negative lipid bilayers. Biochemistry. 1986 Dec 16;25(25):8206–8214. doi: 10.1021/bi00373a013. [DOI] [PubMed] [Google Scholar]