Abstract
Chaen et al. (1986. J. Biol. Chem. 261:13632-13636) showed that treatment of relaxed single muscle fibers with para-phenylenedimaleimide (pPDM) results in inhibition of a fiber's ability to generate active force and a diminished ATPase activity. They postulated that the inhibition of force production was due to pPDM's ability to prevent crossbridges from participating in the normal ATP hydrolysis cycle. We find that the crossbridges produced by pPDM treatment of relaxed muscle cannot bind strongly to the actin filaments in rigor, but do bind weakly to the actin filaments in the presence and also absence of ATP. After pPDM treatment, fiber stiffness, as measured using ramp stretches of varying duration, is ATP-insensitive and identical to that of untreated relaxed fibers (both at high [165 mM] and low [40 mM] ionic strength). These results suggest that the pPDM-treated crossbridges, in both the presence and absence of ATP, are locked in a state that resembles the weakly-binding myosin ATP state of normal crossbridges. Their resemblance to the ATP-crossbridges of relaxed untreated fibers is quite strong; both bind to actin about equally tightly and have similar attachment and detachment rate constants. We also found that crossbridges are locked in a weakly-binding state after treatment with N-phenylmaleimide (NPM). In muscle fibers, this method of producing weakly-binding crossbridges appears preferable to pPDM treatment because, unlike treatment with pPDM, it does not increase the fiber's resting tension and stiffness and it does not disrupt the titin band seen on SDS-PAGE.
Full text
PDF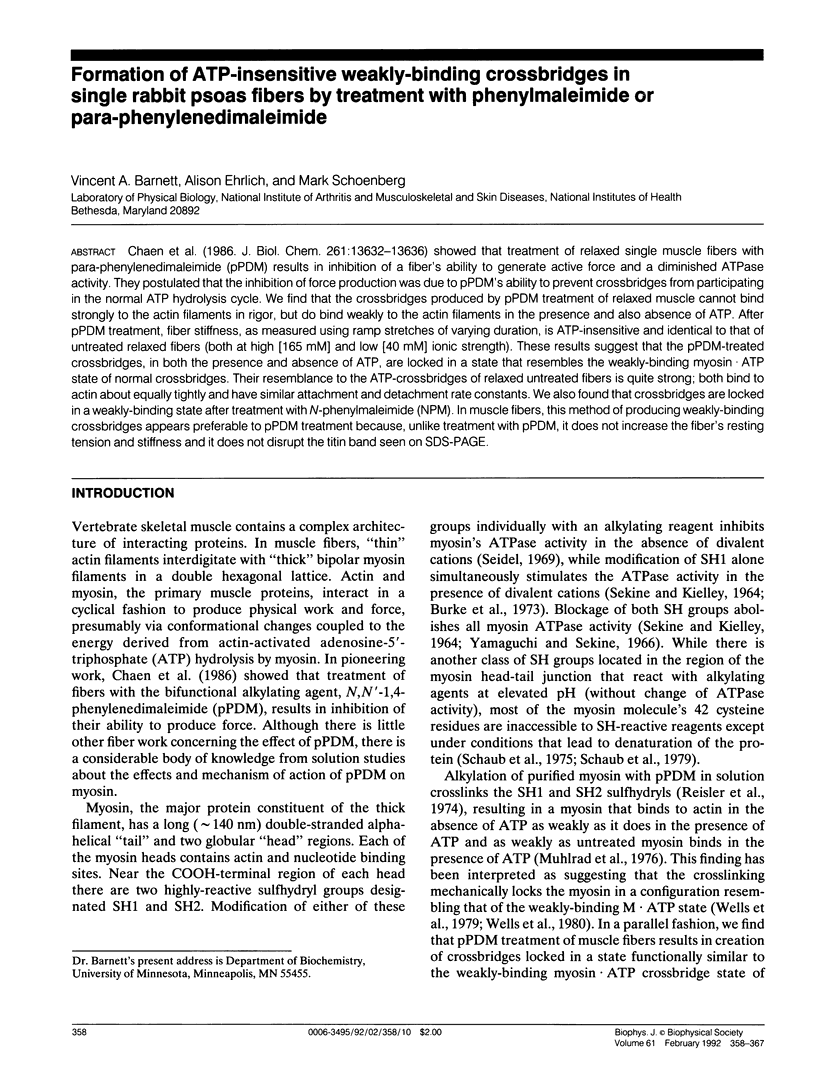
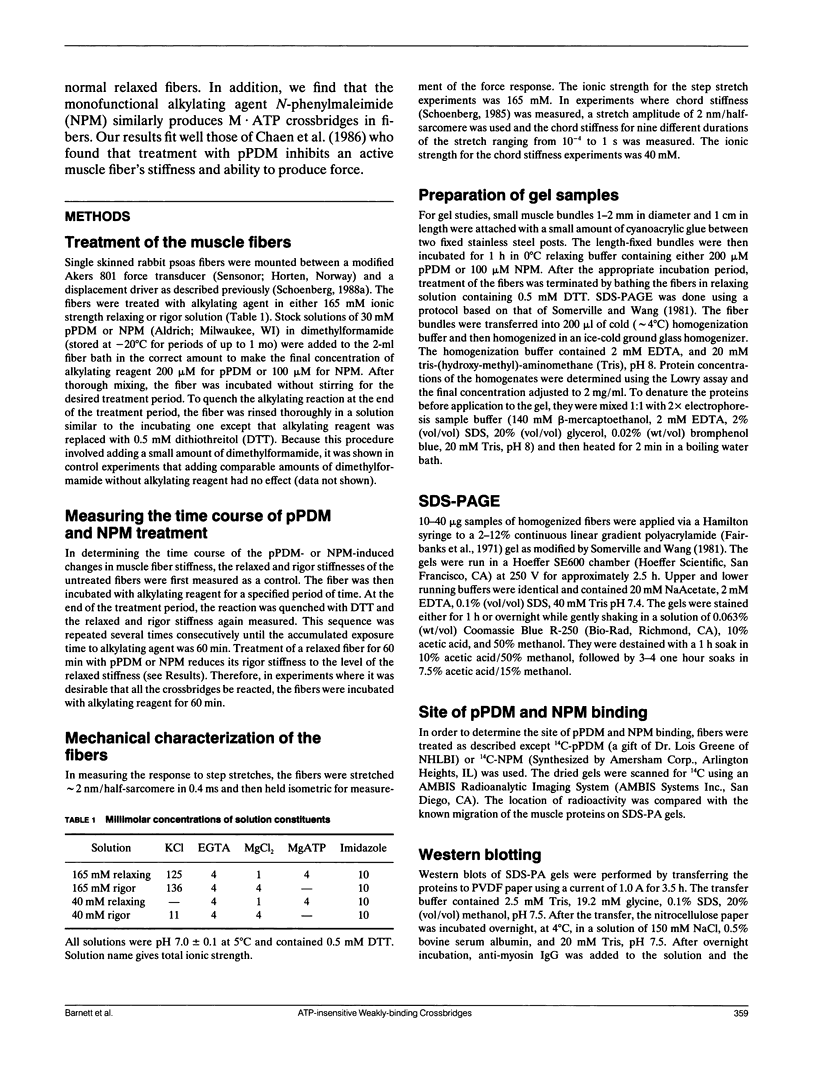
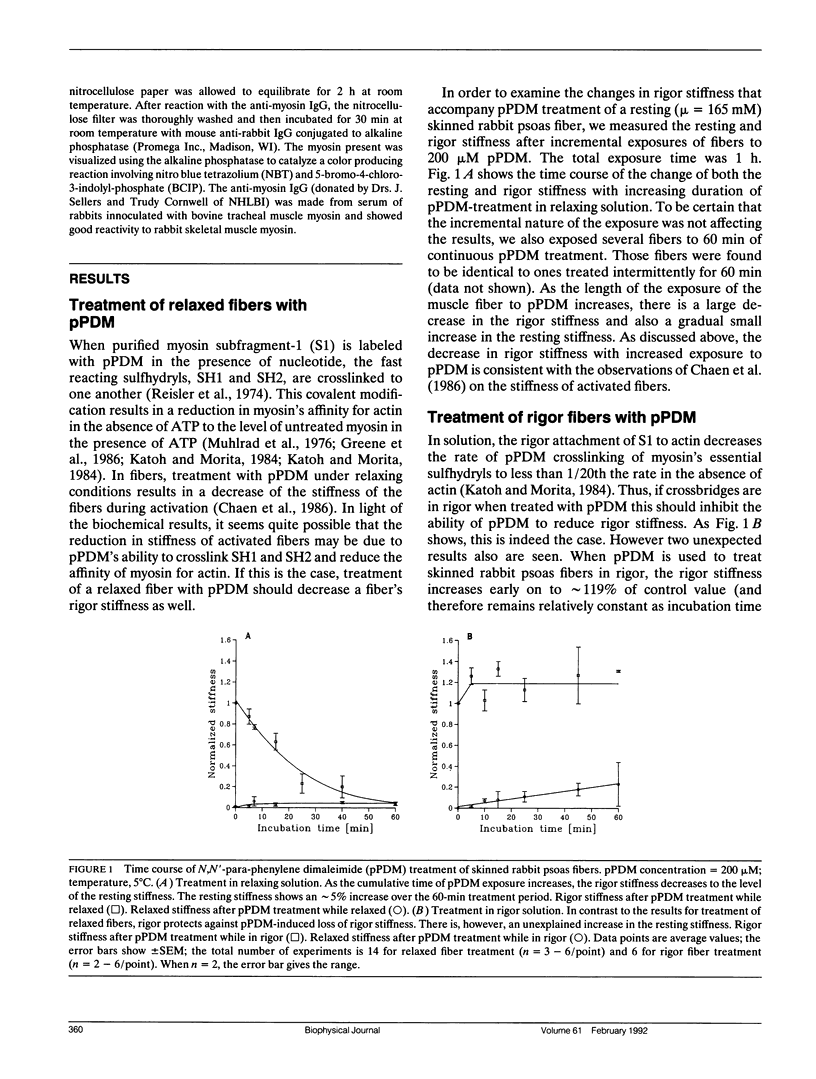
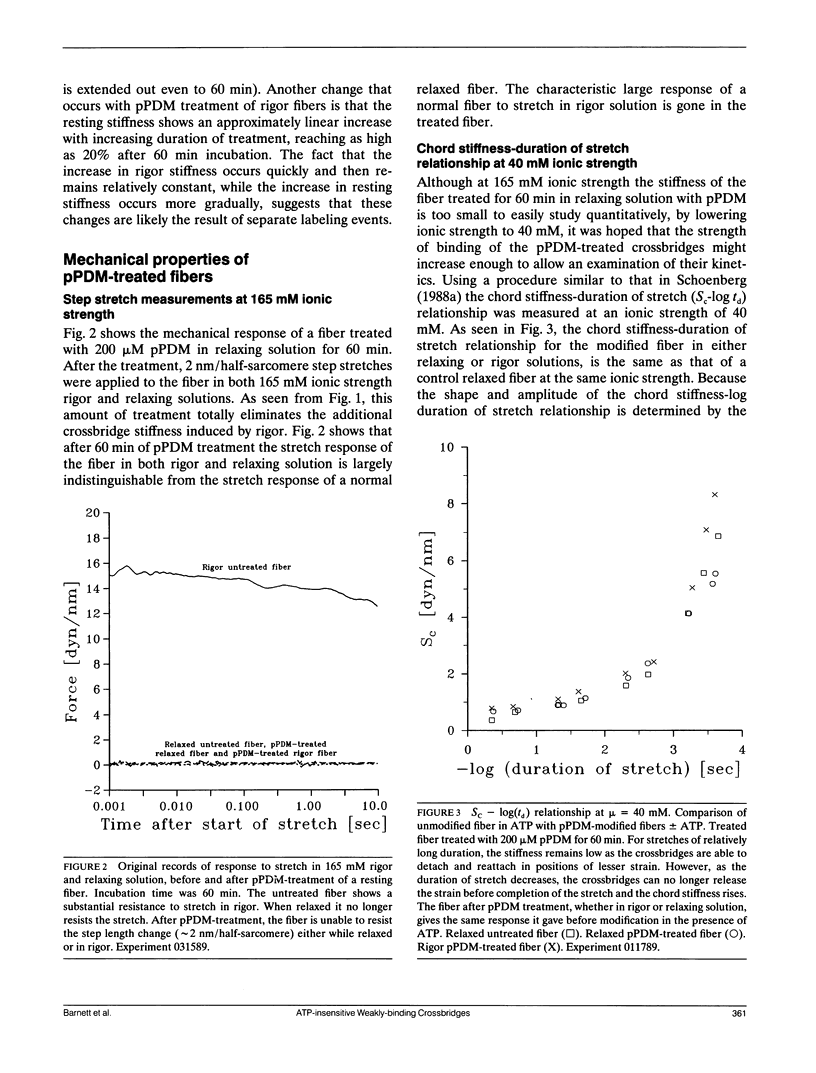
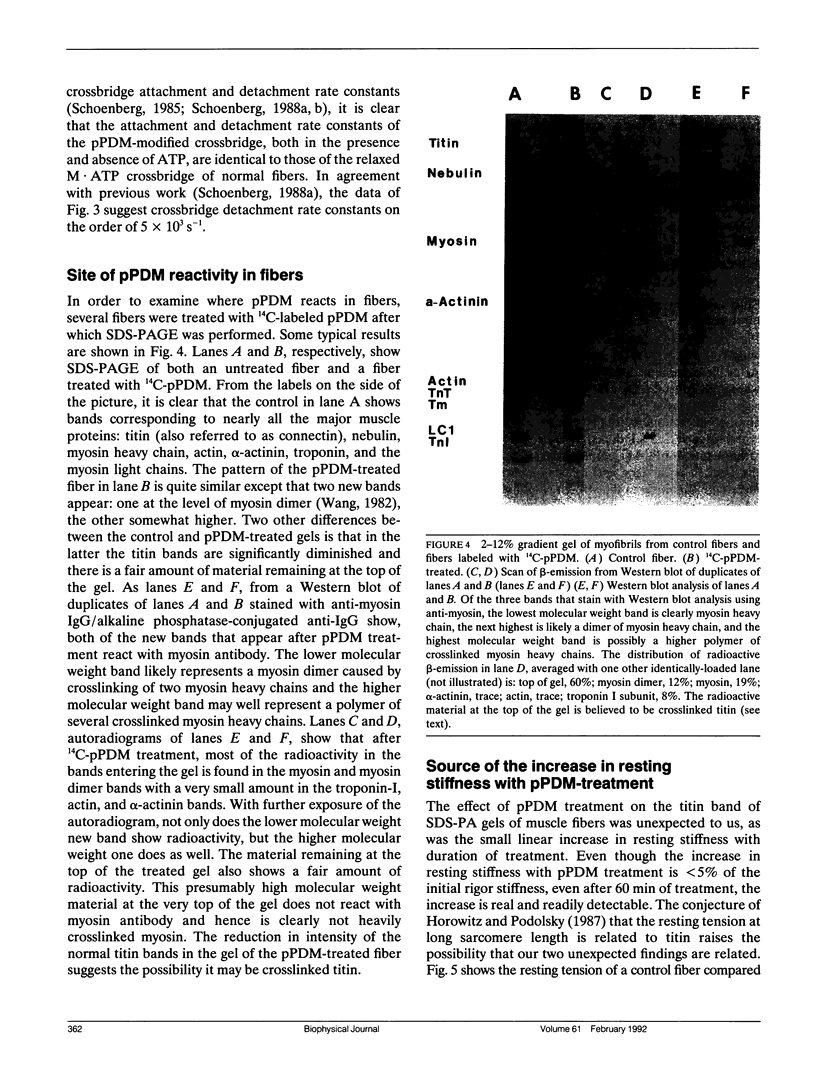
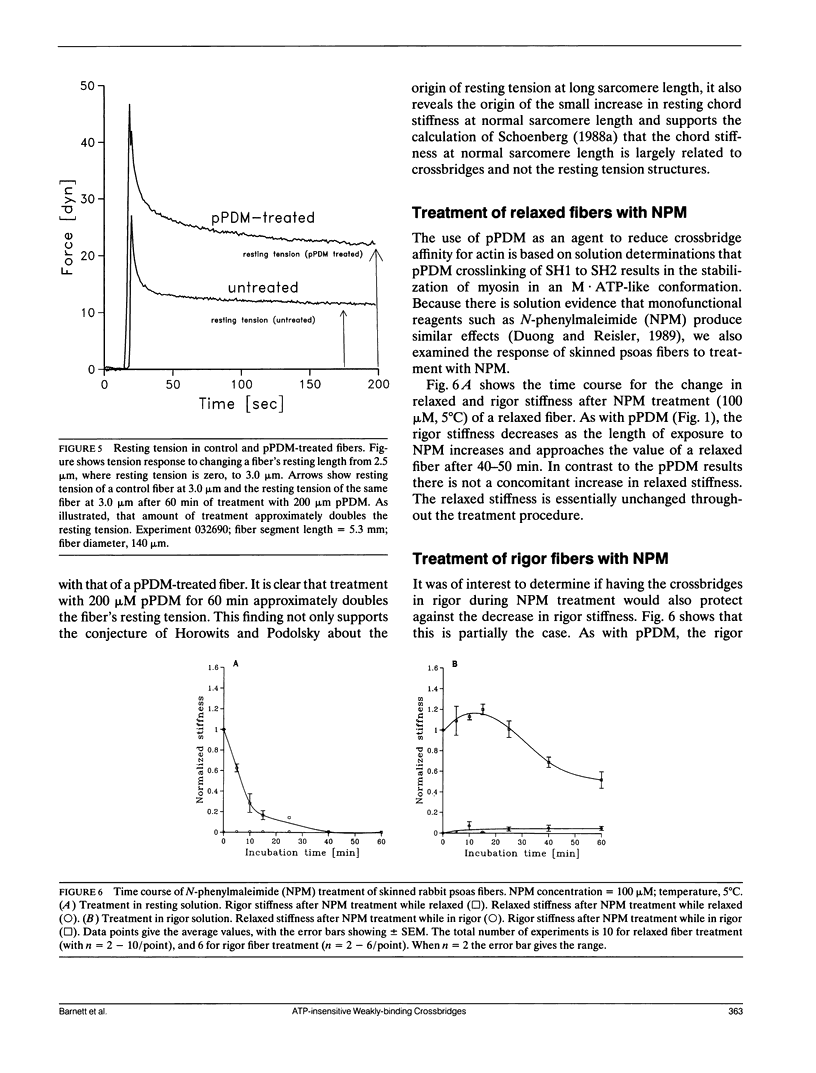
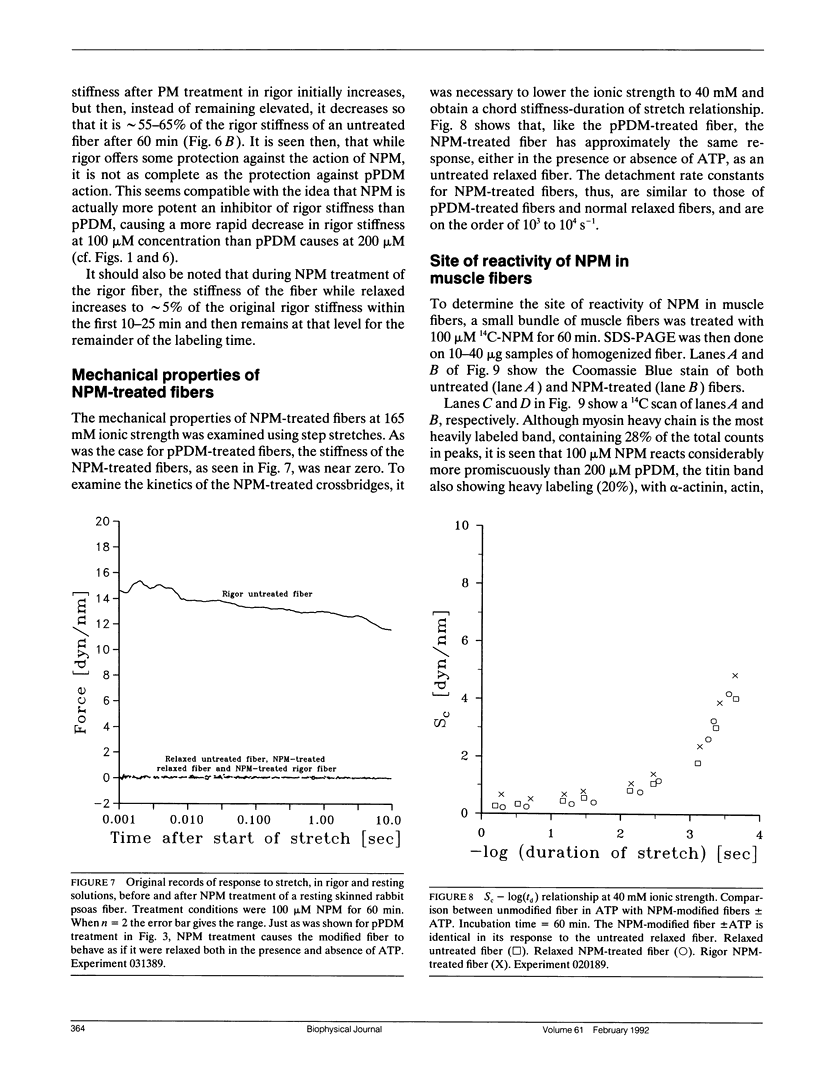
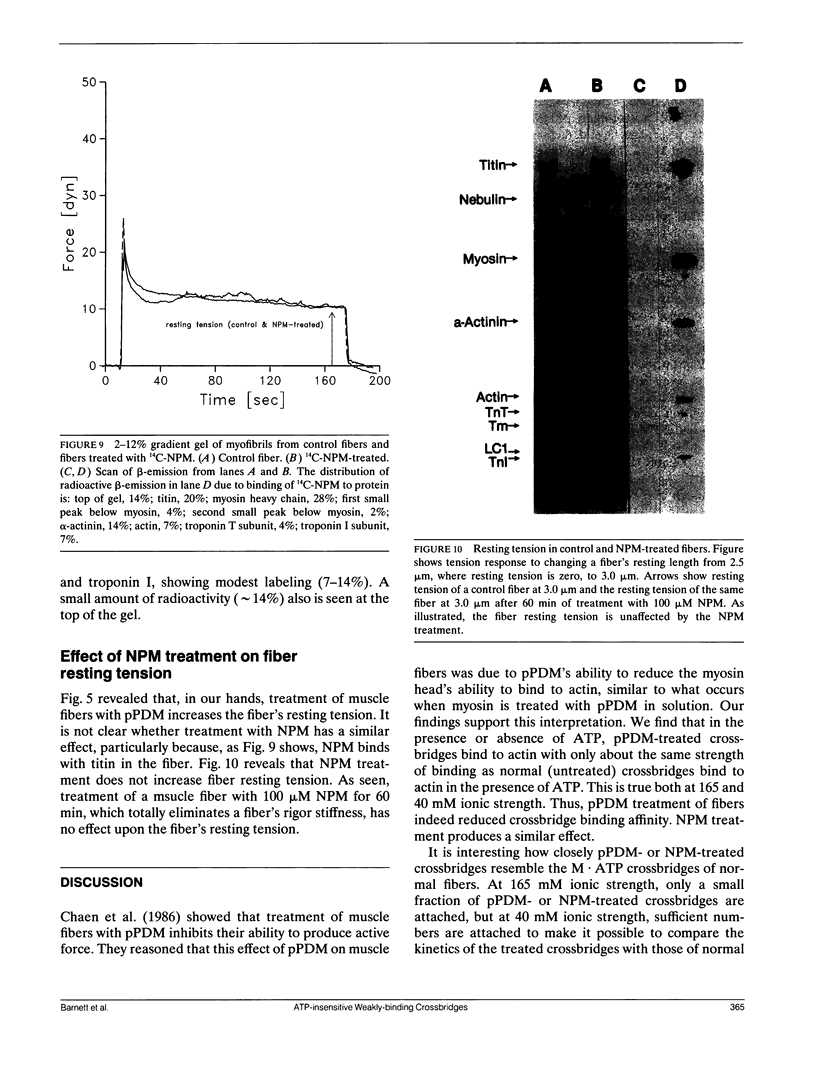
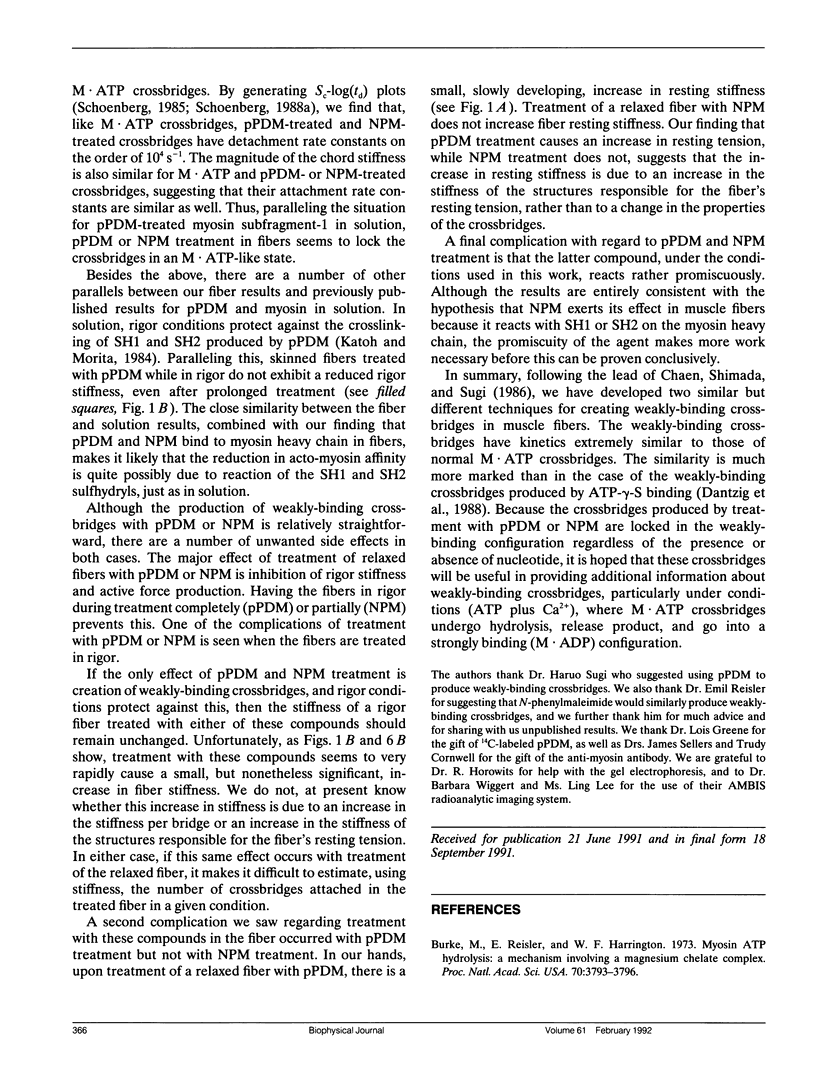
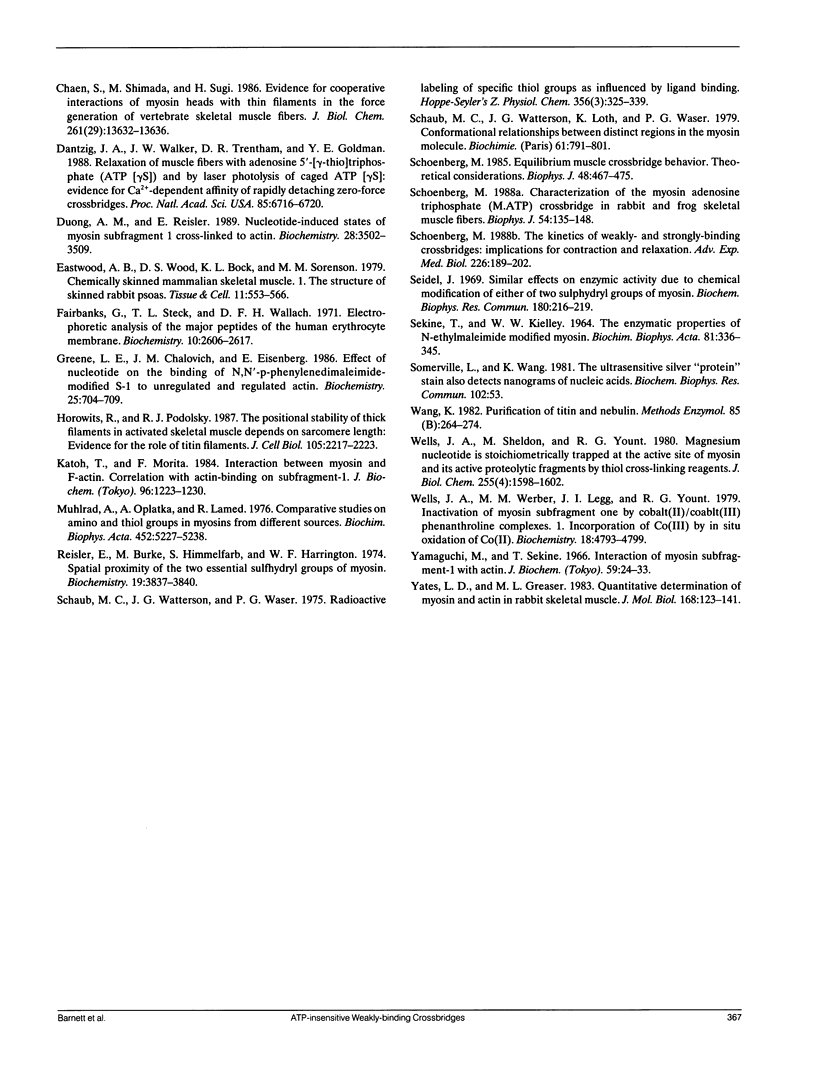
Images in this article
Selected References
These references are in PubMed. This may not be the complete list of references from this article.
- Burke M., Reisler E., Harrington W. F. Myosin ATP hydrolysis: a mechanism involving a magnesium chelate complex. Proc Natl Acad Sci U S A. 1973 Dec;70(12):3793–3796. doi: 10.1073/pnas.70.12.3793. [DOI] [PMC free article] [PubMed] [Google Scholar]
- Chaen S., Shimada M., Sugi H. Evidence for cooperative interactions of myosin heads with thin filament in the force generation of vertebrate skeletal muscle fibers. J Biol Chem. 1986 Oct 15;261(29):13632–13636. [PubMed] [Google Scholar]
- Dantzig J. A., Walker J. W., Trentham D. R., Goldman Y. E. Relaxation of muscle fibers with adenosine 5'-[gamma-thio]triphosphate (ATP[gamma S]) and by laser photolysis of caged ATP[gamma S]: evidence for Ca2+-dependent affinity of rapidly detaching zero-force cross-bridges. Proc Natl Acad Sci U S A. 1988 Sep;85(18):6716–6720. doi: 10.1073/pnas.85.18.6716. [DOI] [PMC free article] [PubMed] [Google Scholar]
- Duong A. M., Reisler E. Nucleotide-induced states of myosin subfragment 1 cross-linked to actin. Biochemistry. 1989 Apr 18;28(8):3502–3509. doi: 10.1021/bi00434a053. [DOI] [PubMed] [Google Scholar]
- Eastwood A. B., Wood D. S., Bock K. L., Sorenson M. M. Chemically skinned mammalian skeletal muscle. I. The structure of skinned rabbit psoas. Tissue Cell. 1979;11(3):553–566. doi: 10.1016/0040-8166(79)90062-4. [DOI] [PubMed] [Google Scholar]
- Fairbanks G., Steck T. L., Wallach D. F. Electrophoretic analysis of the major polypeptides of the human erythrocyte membrane. Biochemistry. 1971 Jun 22;10(13):2606–2617. doi: 10.1021/bi00789a030. [DOI] [PubMed] [Google Scholar]
- Greene L. E., Chalovich J. M., Eisenberg E. Effect of nucleotide on the binding of N,N'-p-phenylenedimaleimide-modified S-1 to unregulated and regulated actin. Biochemistry. 1986 Feb 11;25(3):704–709. doi: 10.1021/bi00351a030. [DOI] [PubMed] [Google Scholar]
- Horowits R., Podolsky R. J. The positional stability of thick filaments in activated skeletal muscle depends on sarcomere length: evidence for the role of titin filaments. J Cell Biol. 1987 Nov;105(5):2217–2223. doi: 10.1083/jcb.105.5.2217. [DOI] [PMC free article] [PubMed] [Google Scholar]
- Katoh T., Morita F. Interaction between myosin and F-actin. Correlation with actin-binding sites on subfragment-1. J Biochem. 1984 Oct;96(4):1223–1230. doi: 10.1093/oxfordjournals.jbchem.a134940. [DOI] [PubMed] [Google Scholar]
- Reisler E., Burke M., Himmelfarb S., Harrington W. F. Spatial proximity of the two essential sulfhydryl groups of myosin. Biochemistry. 1974 Sep 10;13(19):3837–3840. doi: 10.1021/bi00716a001. [DOI] [PubMed] [Google Scholar]
- Schaub M. C., Watterson J. G., Loth K., Waser P. G. Conformational relationships between distinct regions in the myosin molecule. Biochimie. 1979;61(7):791–801. doi: 10.1016/s0300-9084(79)80273-4. [DOI] [PubMed] [Google Scholar]
- Schaub M. C., Watterson J. G., Waser P. G. Conformational differences in myosin, IV.[1-3] Radioactive labeling of specific thiol groups as influenced by ligand binding. Hoppe Seylers Z Physiol Chem. 1975 Mar;356(3):325–339. doi: 10.1515/bchm2.1975.356.1.325. [DOI] [PubMed] [Google Scholar]
- Schoenberg M. Characterization of the myosin adenosine triphosphate (M.ATP) crossbridge in rabbit and frog skeletal muscle fibers. Biophys J. 1988 Jul;54(1):135–148. doi: 10.1016/S0006-3495(88)82938-2. [DOI] [PMC free article] [PubMed] [Google Scholar]
- Schoenberg M. Equilibrium muscle cross-bridge behavior. Theoretical considerations. Biophys J. 1985 Sep;48(3):467–475. doi: 10.1016/S0006-3495(85)83802-9. [DOI] [PMC free article] [PubMed] [Google Scholar]
- Schoenberg M. The kinetics of weakly- and strongly-binding crossbridges: implications for contraction and relaxation. Adv Exp Med Biol. 1988;226:189–202. [PubMed] [Google Scholar]
- Seidel J. C. Similar effects on enzymic activity due to chemical modification of either of two sulfhydryl groups of myosin. Biochim Biophys Acta. 1969 May;180(1):216–219. doi: 10.1016/0005-2728(69)90215-1. [DOI] [PubMed] [Google Scholar]
- Somerville L. L., Wang K. The ultrasensitive silver "protein" stain also detects nanograms of nucleic acids. Biochem Biophys Res Commun. 1981 Sep 16;102(1):53–58. doi: 10.1016/0006-291x(81)91487-x. [DOI] [PubMed] [Google Scholar]
- Wang K. Purification of titin and nebulin. Methods Enzymol. 1982;85(Pt B):264–274. doi: 10.1016/0076-6879(82)85025-8. [DOI] [PubMed] [Google Scholar]
- Wells J. A., Sheldon M., Yount R. G. Magnesium nucleotide is stoichiometrically trapped at the active site of myosin and its active proteolytic fragments by thiol cross-linking reagents. J Biol Chem. 1980 Feb 25;255(4):1598–1602. [PubMed] [Google Scholar]
- Wells J. A., Werber M. M., Legg J. I., Yount R. G. Inactivation of myosin subfragment one by cobalt(II)/cobalt(III) phenanthroline complexes. I. Incorporation of Co(III) by in situ oxidation of Co(II). Biochemistry. 1979 Oct 30;18(22):4793–4799. doi: 10.1021/bi00589a005. [DOI] [PubMed] [Google Scholar]
- Yamaguchi M., Sekine T. Sulfhydryl groups involved in the active site of myosin A adenosine triphosphatase. I. Specific blocking of the SH group responsible for the inhibitory phase in "B phasic response" of the catalytic activity. J Biochem. 1966 Jan;59(1):24–33. doi: 10.1093/oxfordjournals.jbchem.a128254. [DOI] [PubMed] [Google Scholar]
- Yates L. D., Greaser M. L. Quantitative determination of myosin and actin in rabbit skeletal muscle. J Mol Biol. 1983 Jul 25;168(1):123–141. doi: 10.1016/s0022-2836(83)80326-x. [DOI] [PubMed] [Google Scholar]