Abstract
Channel access resistance has been measured to estimate the characteristic size of a single ion channel. We compare channel conductance in the presence of nonpenetrating water-soluble polymers with that obtained for polymer-free electrolyte solution. The contribution of the access resistance to the total alamethicin channel resistance is approximately 10% for first three open channel levels. The open alamethicin channel radii inferred for these first three levels from the access resistance are 6.3, 10.3, and 11.4 A. The dependence of channel conductance on polymer molecular weight also allows evaluation of the channel dimensions from polymer exclusion. Despite varying conductance, it was shown that steric radii of the alamethicin channel at different conductance levels remain approximately unchanged. These results support a model of the alamethicin channel as an array of closely packed parallel pores of nearly uniform diameter.
Full text
PDF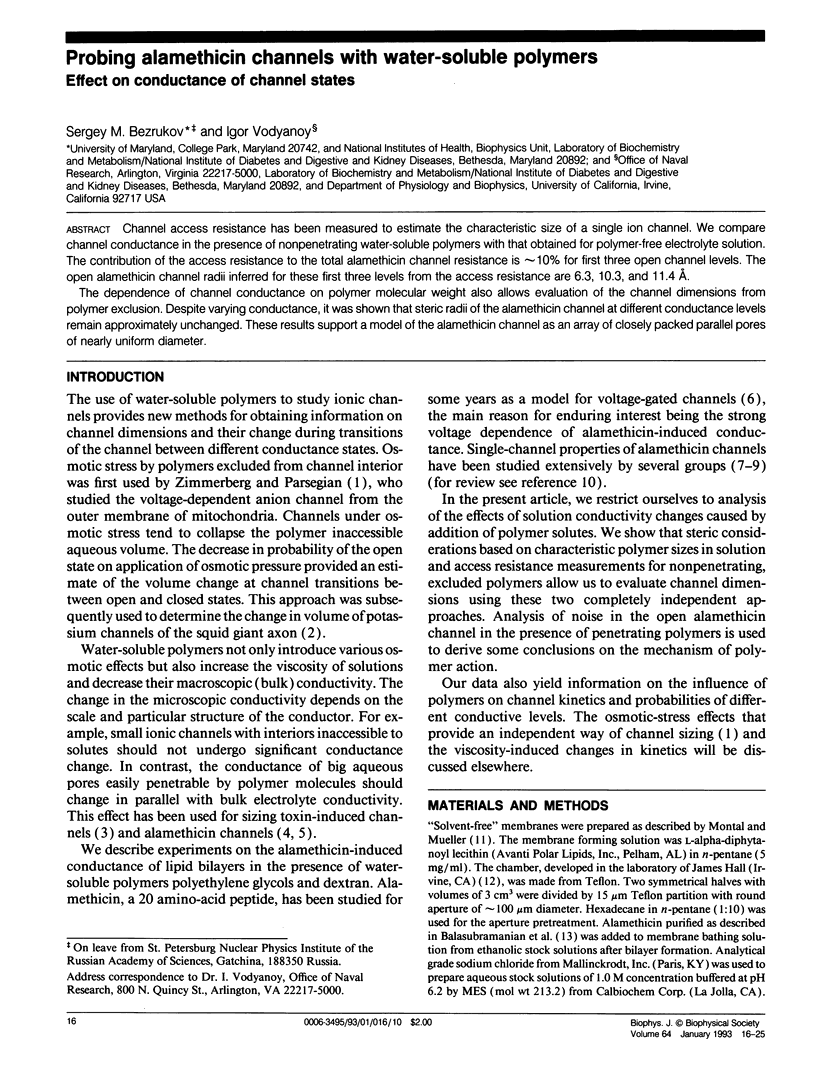
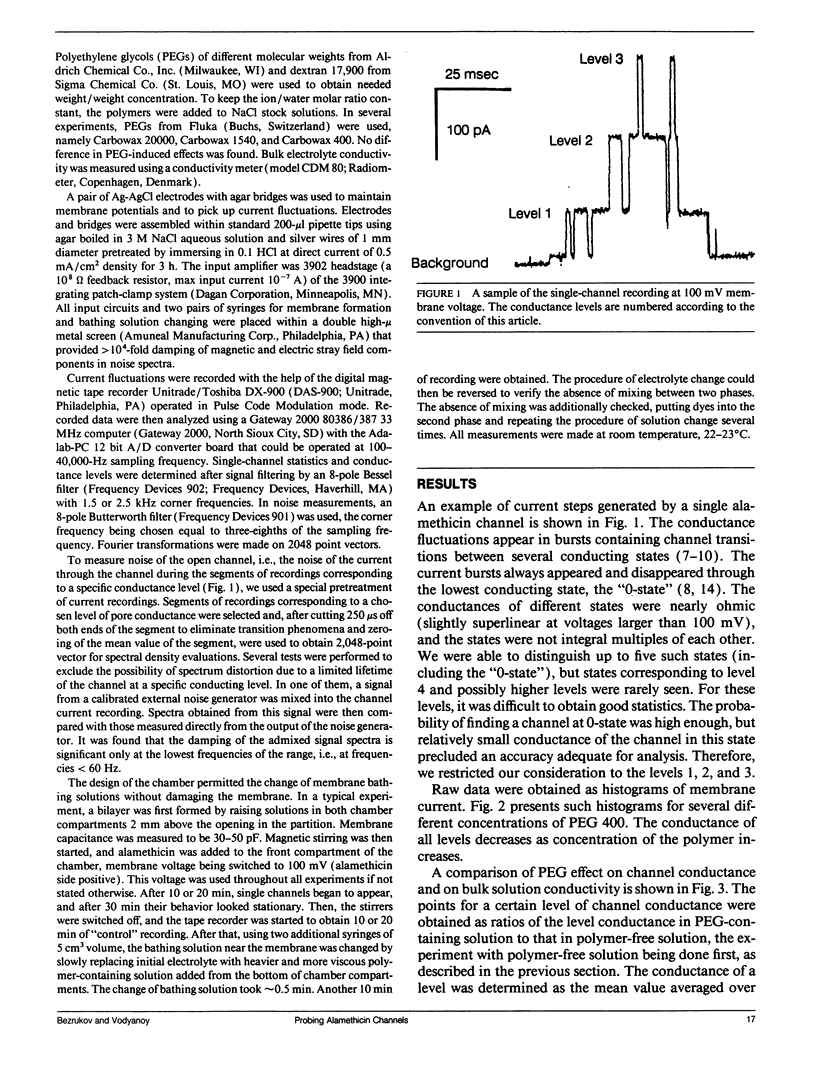
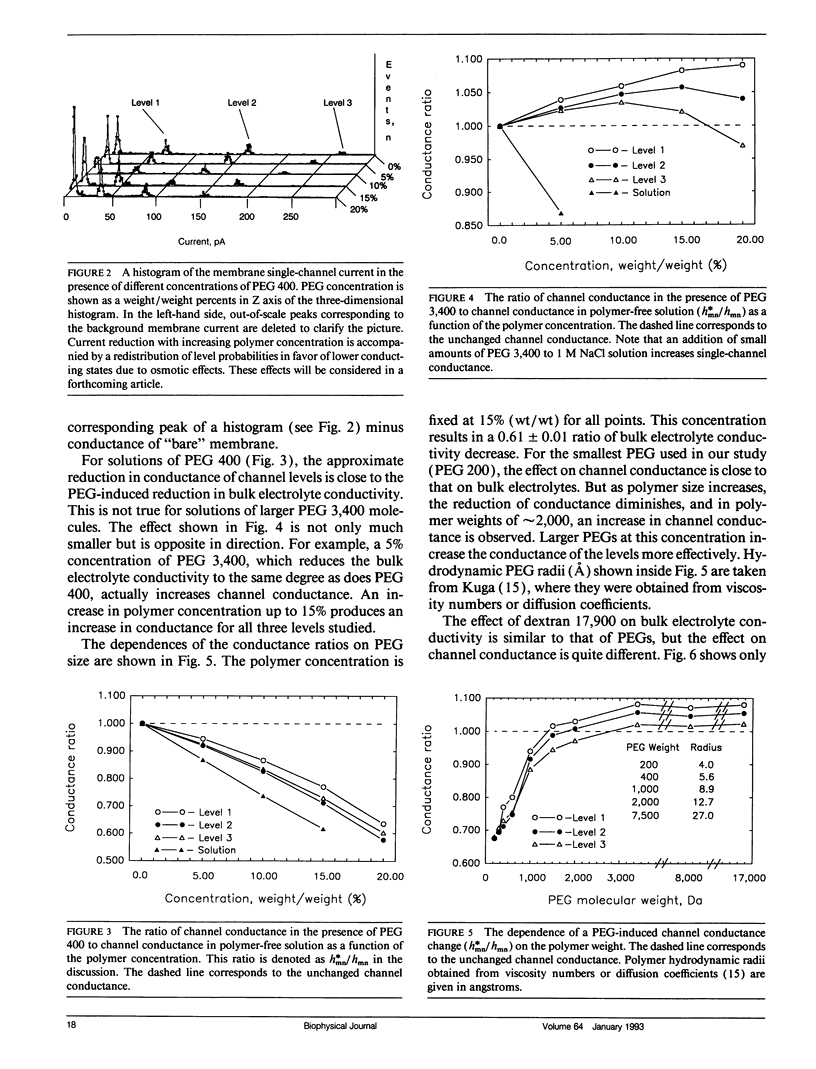
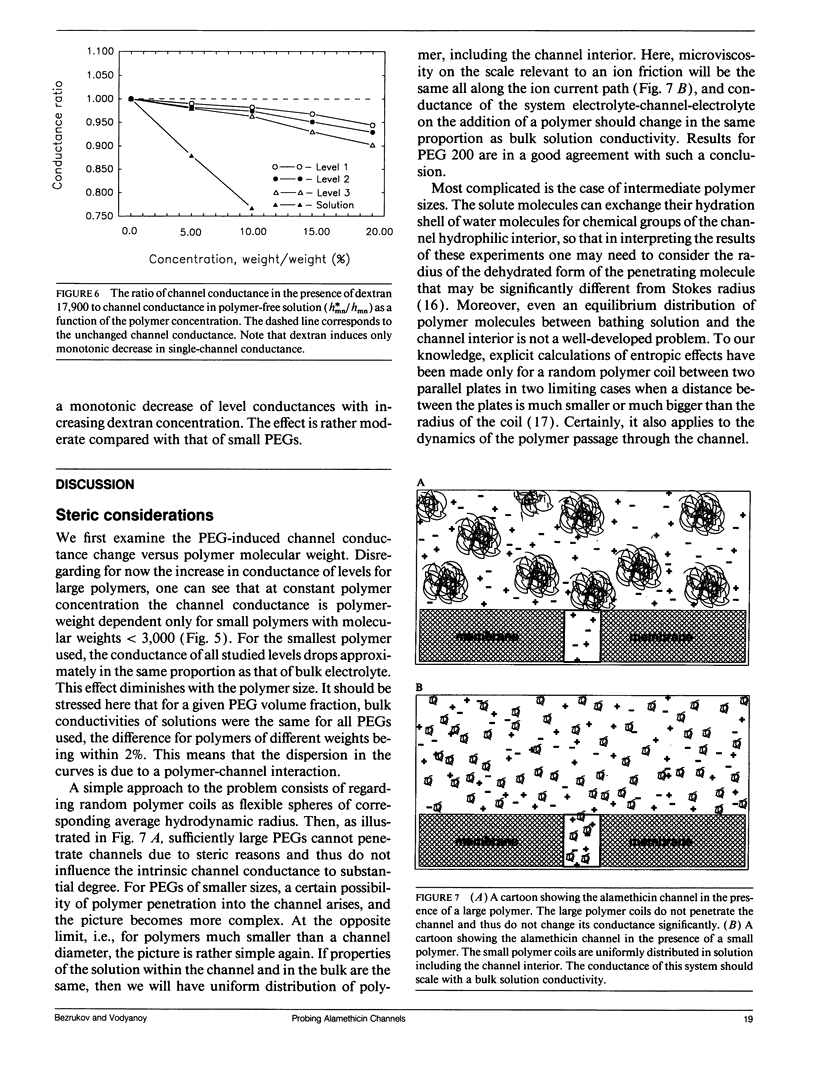
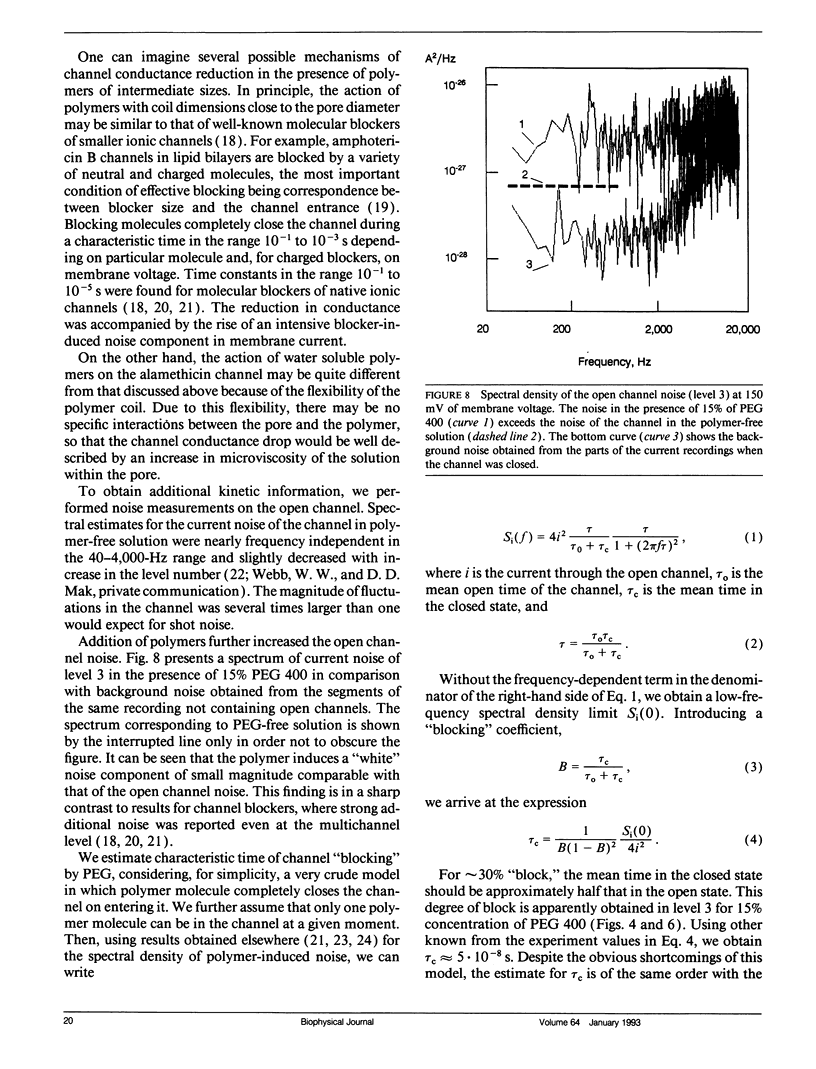
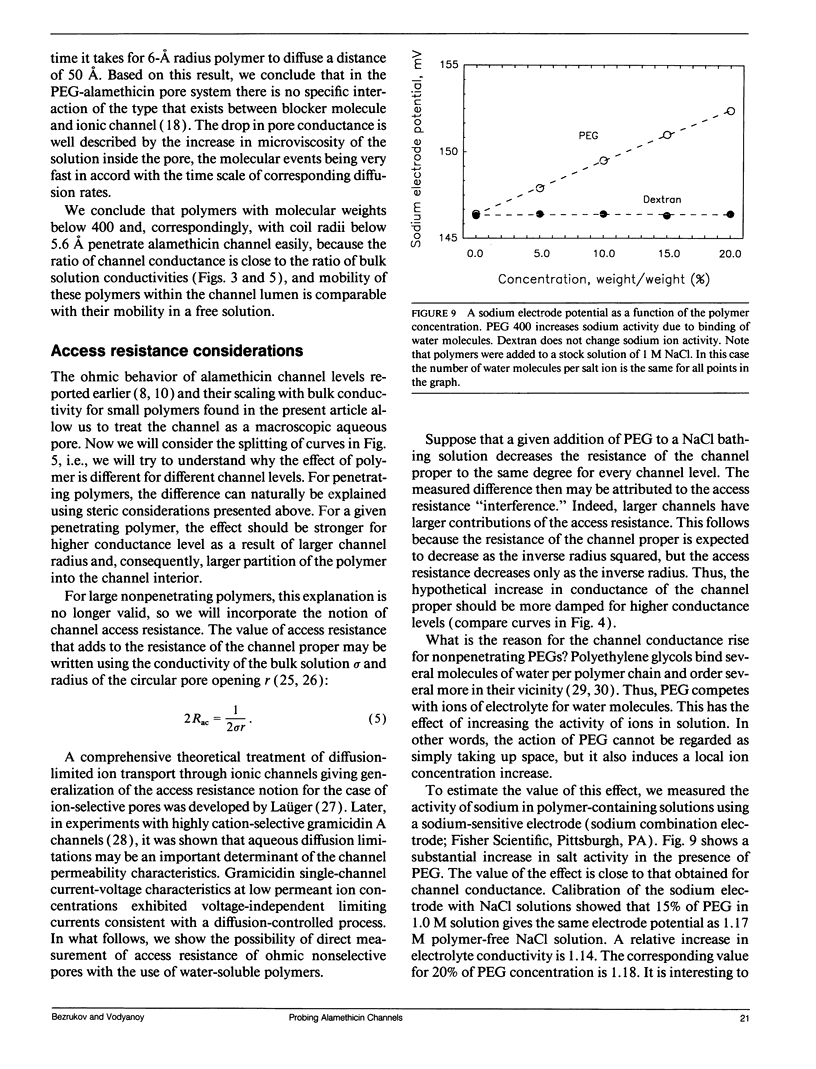
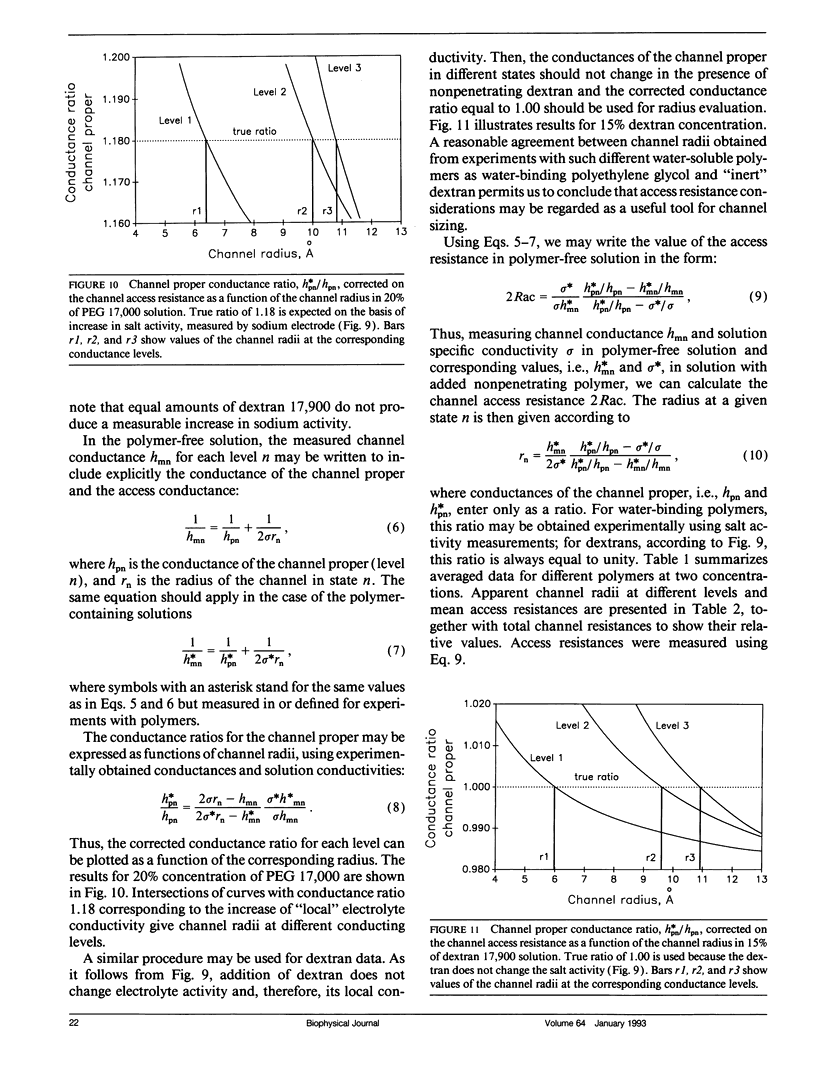
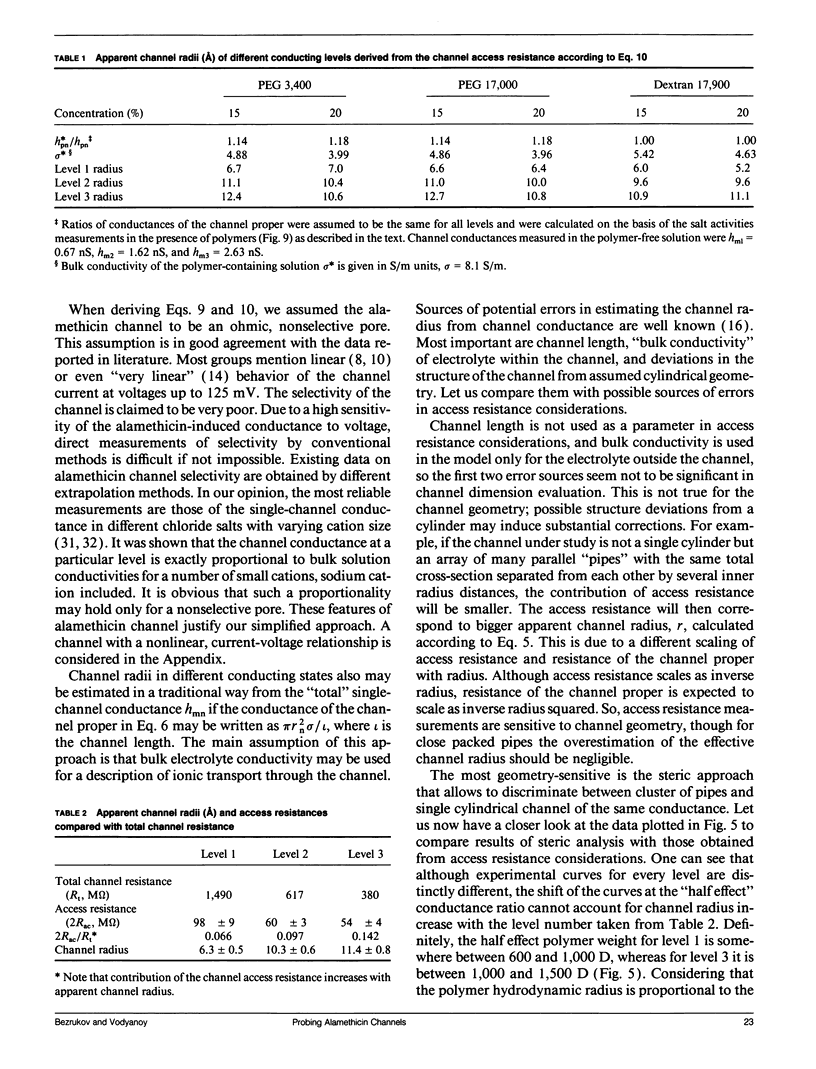
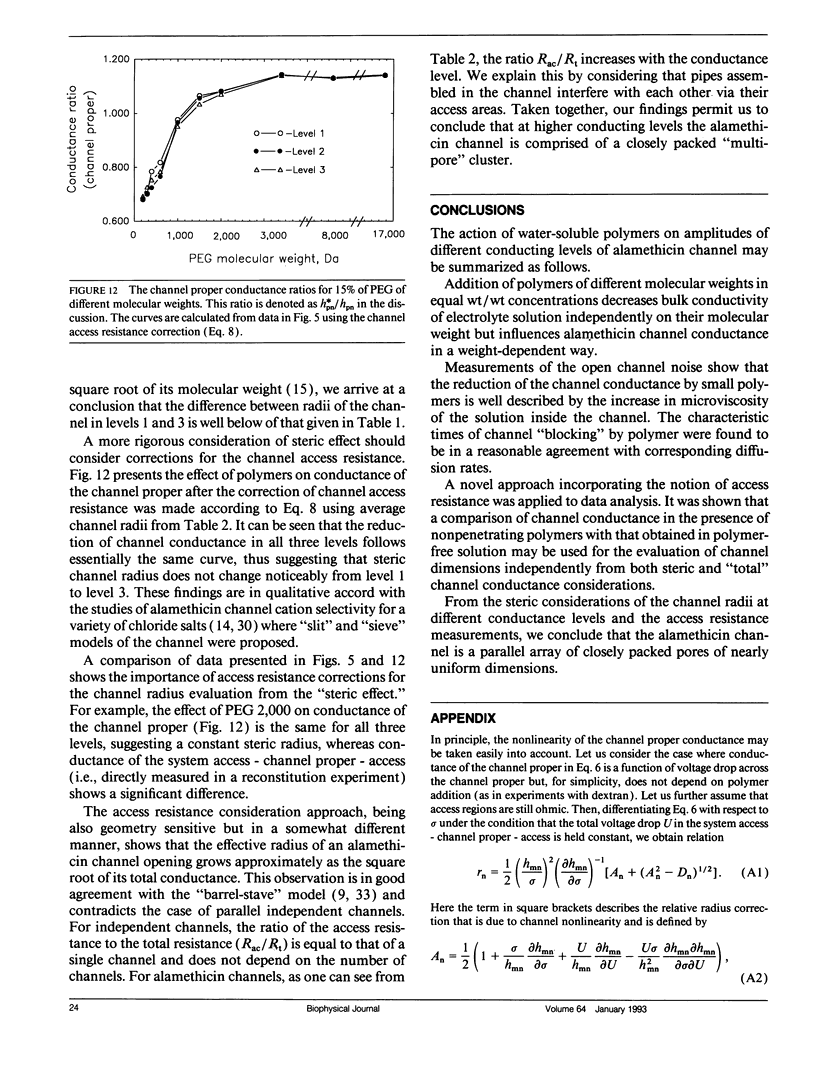
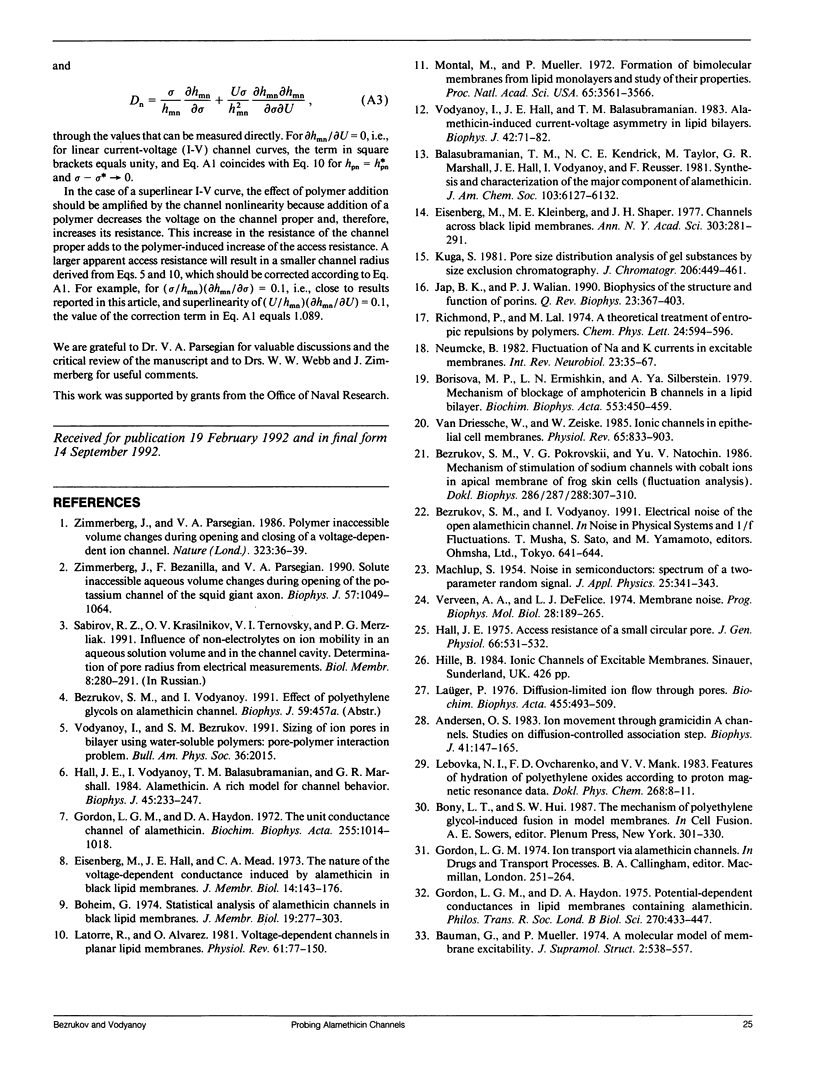
Selected References
These references are in PubMed. This may not be the complete list of references from this article.
- Andersen O. S. Ion movement through gramicidin A channels. Studies on the diffusion-controlled association step. Biophys J. 1983 Feb;41(2):147–165. doi: 10.1016/S0006-3495(83)84416-6. [DOI] [PMC free article] [PubMed] [Google Scholar]
- Baumann G., Mueller P. A molecular model of membrane excitability. J Supramol Struct. 1974;2(5-6):538–557. doi: 10.1002/jss.400020504. [DOI] [PubMed] [Google Scholar]
- Boheim G. Statistical analysis of alamethicin channels in black lipid membranes. J Membr Biol. 1974;19(3):277–303. doi: 10.1007/BF01869983. [DOI] [PubMed] [Google Scholar]
- Borisova M. P., Ermishkin L. N., Silberstein A. Y. Mechanism of blockage of amphotericin B channels in a lipid bilayer. Biochim Biophys Acta. 1979 Jun 2;553(3):450–459. doi: 10.1016/0005-2736(79)90300-6. [DOI] [PubMed] [Google Scholar]
- Eisenberg M., Hall J. E., Mead C. A. The nature of the voltage-dependent conductance induced by alamethicin in black lipid membranes. J Membr Biol. 1973 Dec 31;14(2):143–176. doi: 10.1007/BF01868075. [DOI] [PubMed] [Google Scholar]
- Eisenberg M., Kleinberg M. E., Shaper J. H. Channels across black lipid membranes. Ann N Y Acad Sci. 1977 Dec 30;303:281–294. [PubMed] [Google Scholar]
- Gordon L. G., Haydon D. A. Potential-dependent conductances in lipid membranes containing alamethicin. Philos Trans R Soc Lond B Biol Sci. 1975 Jun 10;270(908):433–447. doi: 10.1098/rstb.1975.0021. [DOI] [PubMed] [Google Scholar]
- Gordon L. G., Haydon D. A. The unit conductance channel of alamethicin. Biochim Biophys Acta. 1972 Mar 17;255(3):1014–1018. doi: 10.1016/0005-2736(72)90415-4. [DOI] [PubMed] [Google Scholar]
- Hall J. E. Access resistance of a small circular pore. J Gen Physiol. 1975 Oct;66(4):531–532. doi: 10.1085/jgp.66.4.531. [DOI] [PMC free article] [PubMed] [Google Scholar]
- Hall J. E., Vodyanoy I., Balasubramanian T. M., Marshall G. R. Alamethicin. A rich model for channel behavior. Biophys J. 1984 Jan;45(1):233–247. doi: 10.1016/S0006-3495(84)84151-X. [DOI] [PMC free article] [PubMed] [Google Scholar]
- Jap B. K., Walian P. J. Biophysics of the structure and function of porins. Q Rev Biophys. 1990 Nov;23(4):367–403. doi: 10.1017/s003358350000559x. [DOI] [PubMed] [Google Scholar]
- Latorre R., Alvarez O. Voltage-dependent channels in planar lipid bilayer membranes. Physiol Rev. 1981 Jan;61(1):77–150. doi: 10.1152/physrev.1981.61.1.77. [DOI] [PubMed] [Google Scholar]
- Läuger P. Diffusion-limited ion flow through pores. Biochim Biophys Acta. 1976 Dec 2;455(2):493–509. doi: 10.1016/0005-2736(76)90320-5. [DOI] [PubMed] [Google Scholar]
- Montal M., Mueller P. Formation of bimolecular membranes from lipid monolayers and a study of their electrical properties. Proc Natl Acad Sci U S A. 1972 Dec;69(12):3561–3566. doi: 10.1073/pnas.69.12.3561. [DOI] [PMC free article] [PubMed] [Google Scholar]
- Neumcke B. Fluctuation of Na and K currents in excitable membranes. Int Rev Neurobiol. 1982;23:35–67. doi: 10.1016/s0074-7742(08)60621-2. [DOI] [PubMed] [Google Scholar]
- Van Driessche W., Zeiske W. Ionic channels in epithelial cell membranes. Physiol Rev. 1985 Oct;65(4):833–903. doi: 10.1152/physrev.1985.65.4.833. [DOI] [PubMed] [Google Scholar]
- Verveen A. A., DeFelice L. J. Membrane noise. Prog Biophys Mol Biol. 1974;28:189–265. doi: 10.1016/0079-6107(74)90019-4. [DOI] [PubMed] [Google Scholar]
- Vodyanoy I., Hall J. E., Balasubramanian T. M. Alamethicin-induced current-voltage curve asymmetry in lipid bilayers. Biophys J. 1983 Apr;42(1):71–82. doi: 10.1016/S0006-3495(83)84370-7. [DOI] [PMC free article] [PubMed] [Google Scholar]
- Zimmerberg J., Bezanilla F., Parsegian V. A. Solute inaccessible aqueous volume changes during opening of the potassium channel of the squid giant axon. Biophys J. 1990 May;57(5):1049–1064. doi: 10.1016/S0006-3495(90)82623-0. [DOI] [PMC free article] [PubMed] [Google Scholar]
- Zimmerberg J., Parsegian V. A. Polymer inaccessible volume changes during opening and closing of a voltage-dependent ionic channel. Nature. 1986 Sep 4;323(6083):36–39. doi: 10.1038/323036a0. [DOI] [PubMed] [Google Scholar]