Abstract
We have examined the morphology and osmotic properties of large unilamellar vesicles (LUVs) prepared by extrusion. Contrary to expectations, we observe by cryo-electron microscopy that such vesicles, under isoosmotic conditions, are non-spherical. This morphology appears to be a consequence of vesicle passage through the filter pores during preparation. As a result when such LUVs are placed in a hypoosmotic medium they are able to compensate, at least partially, for the resulting influx of water by "rounding up" and thereby increasing their volume with no change in surface area. The increase in vesicle trapped volume associated with these morphological changes was determined using the slowly membrane-permeable solute [3H]-glucose. This allowed calculation of the actual osmotic gradient experienced by the vesicle membrane for a given applied differential. When LUVs were exposed to osmotic differentials of sufficient magnitude lysis occurred with the extent of solute release being dependent on the size of the osmotic gradient. Surprisingly, lysis was not an all-or-nothing event, but instead a residual osmotic differential remained after lysis. This differential value was comparable in magnitude to the minimum osmotic differential required to trigger lysis. Further, by comparing the release of solutes of differing molecular weights (glucose and dextran) a lower limit of about 12 nm diameter can be set for the bilayer defect created during lysis. Finally, the maximum residual osmotic differentials were compared for LUVs varying in mean diameter from 90 to 340 nm. This comparison confirmed that these systems obey Laplace's Law relating vesicle diameter and lysis pressure. This analysis also yielded a value for the membrane tension at lysis of 40 dyn cm-1 at 23 degrees C, which is in reasonable agreement with previously published values for giant unilamellar vesicles.
Full text
PDF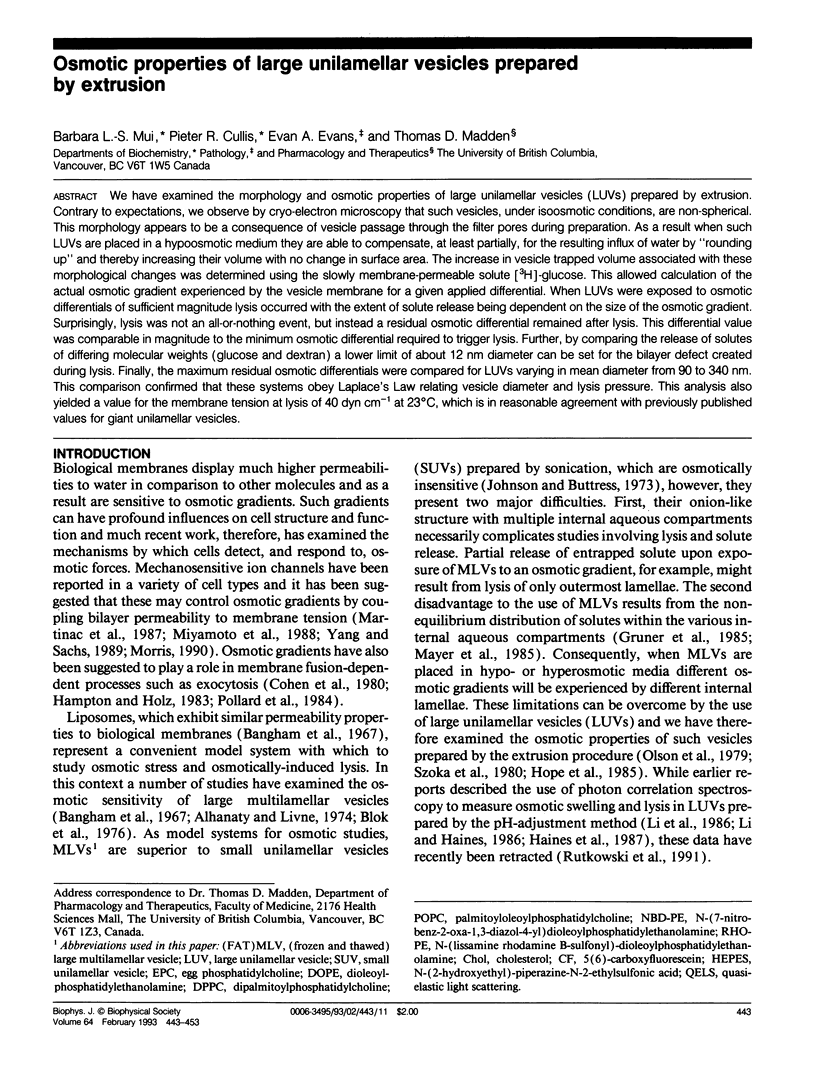
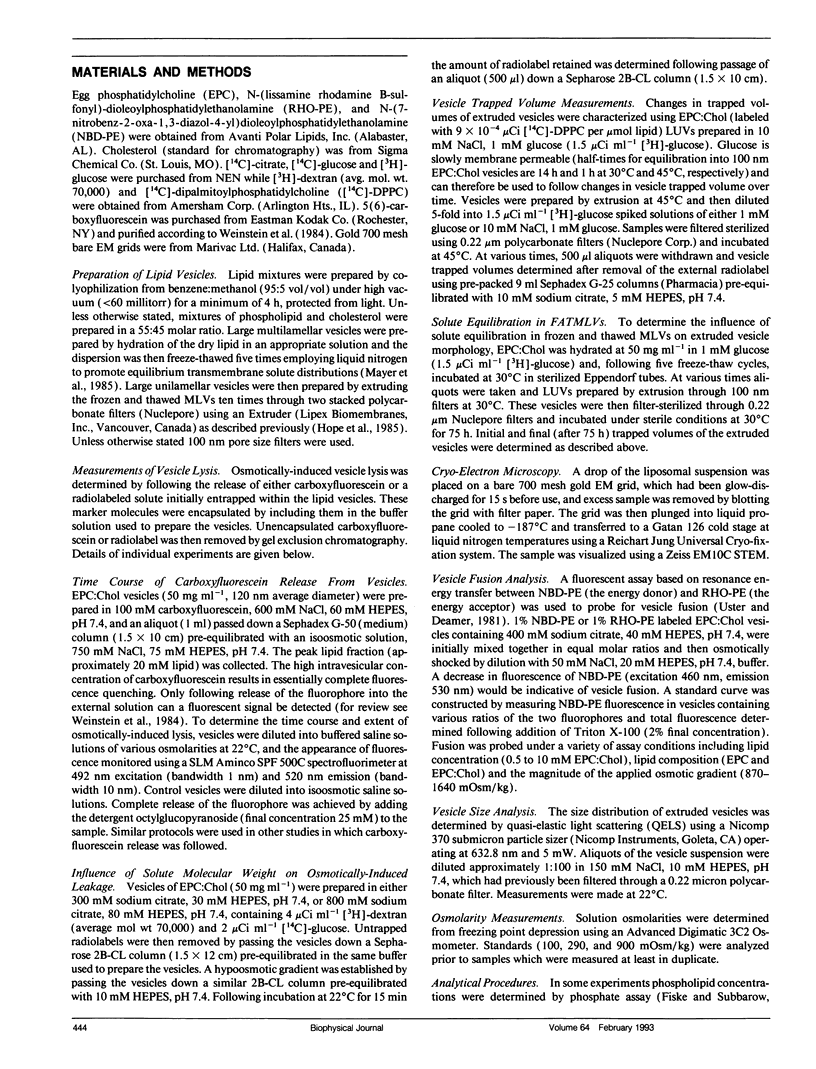
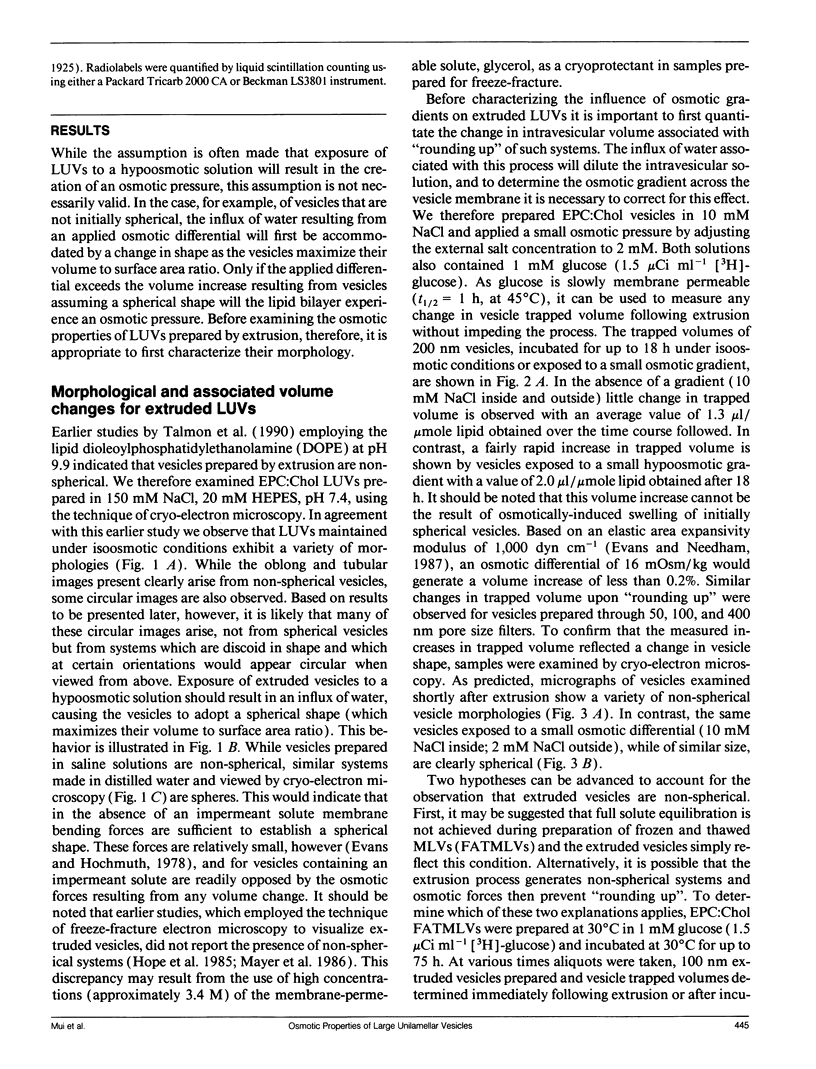
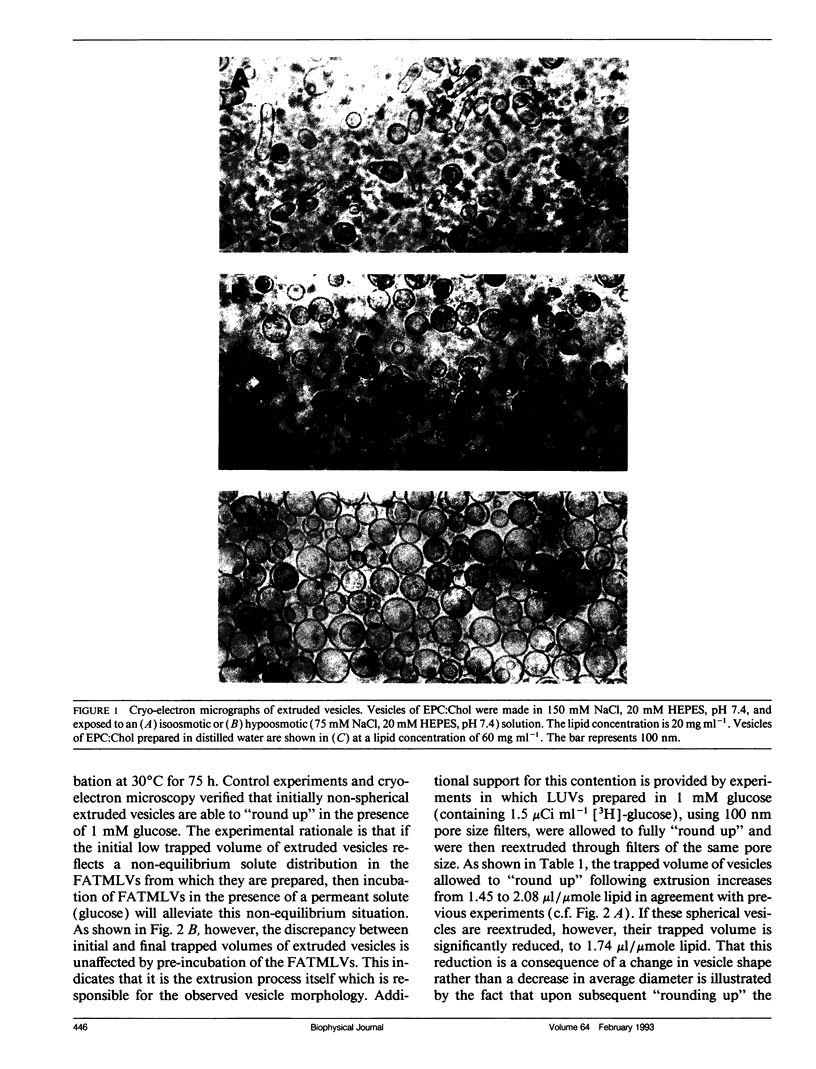
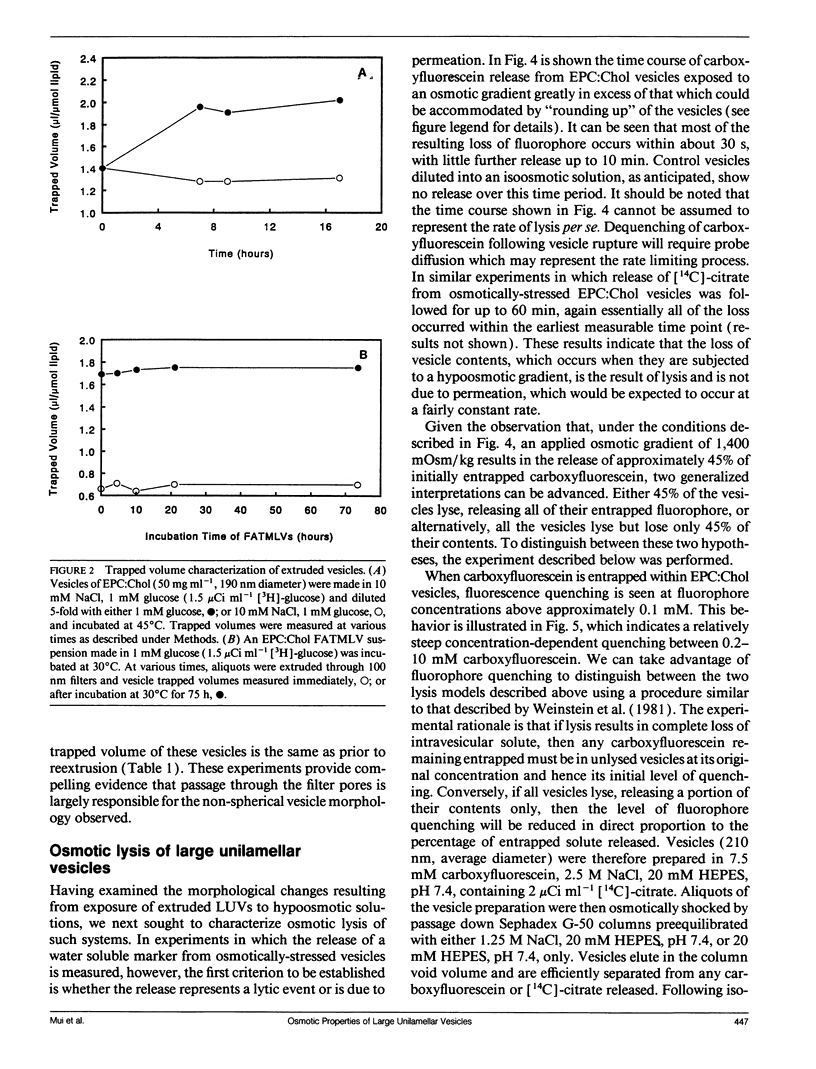
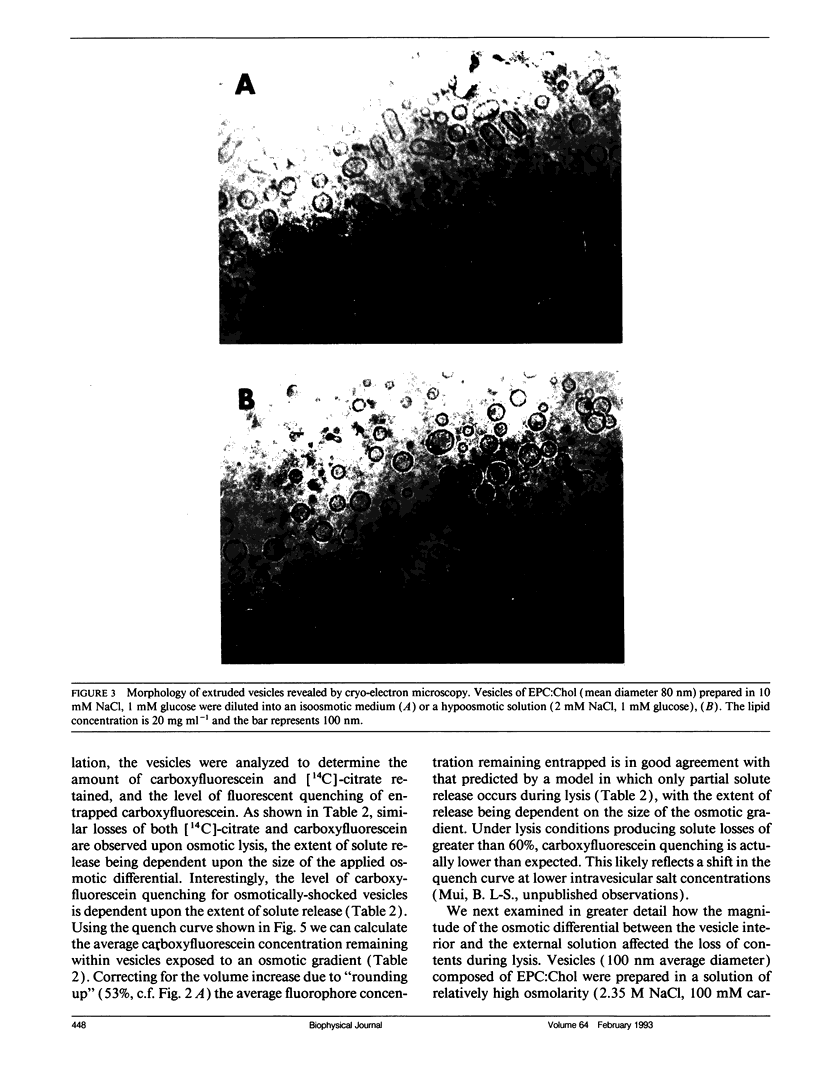
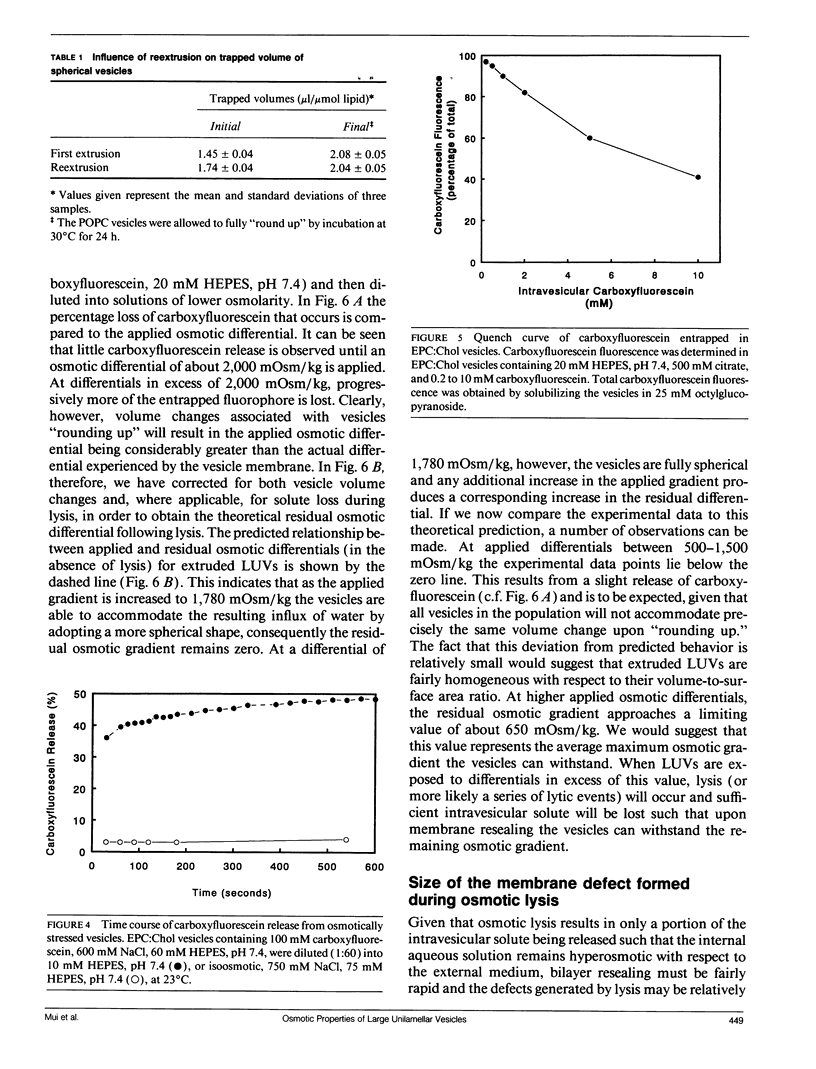
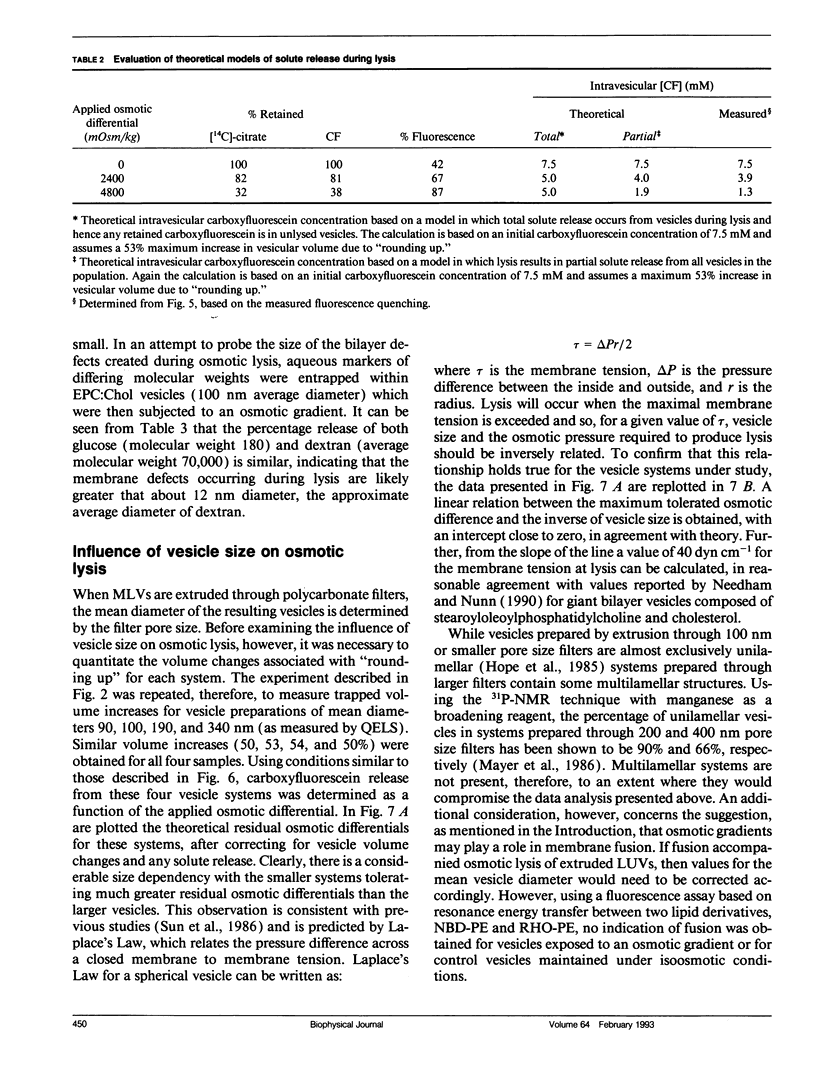
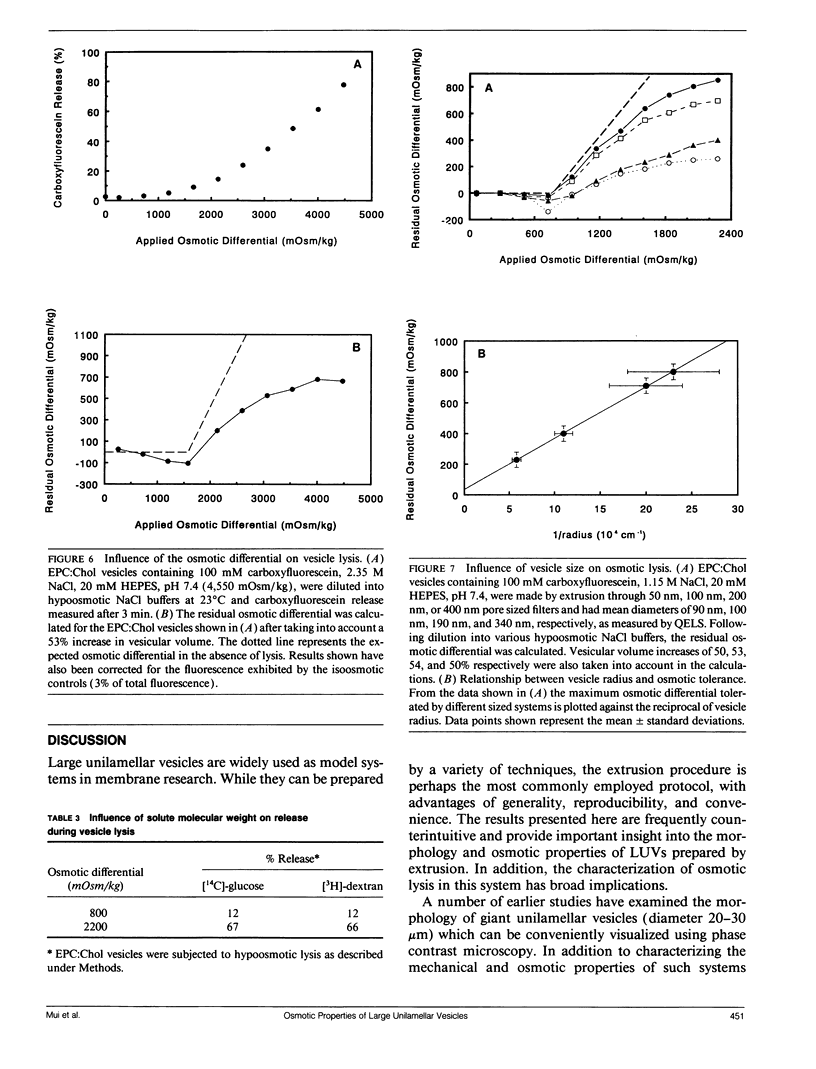
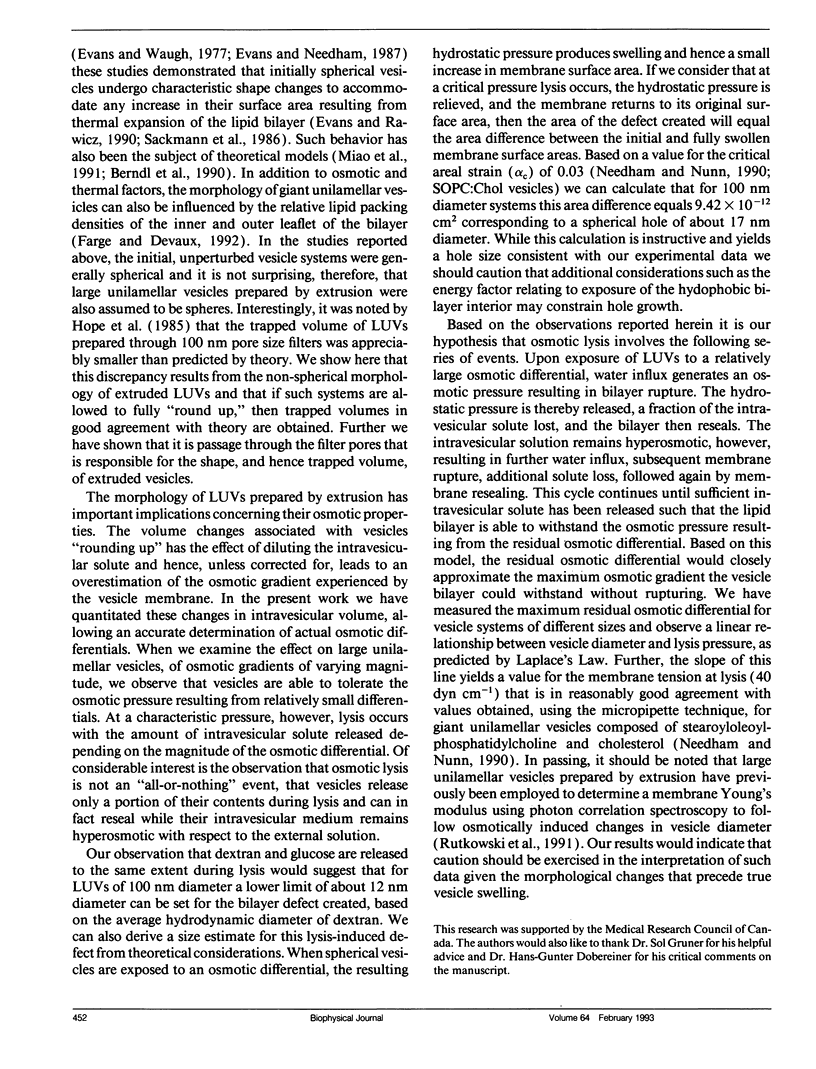
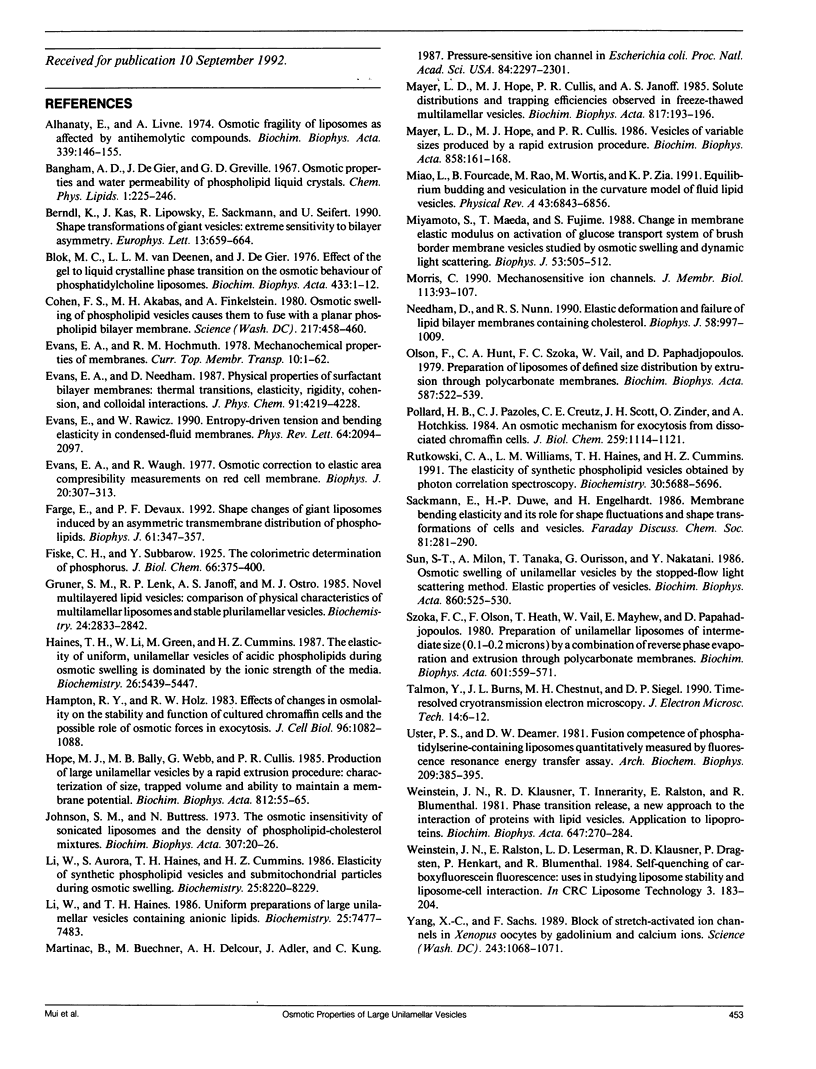
Images in this article
Selected References
These references are in PubMed. This may not be the complete list of references from this article.
- Alhanaty E., Livne A. Osmotic fragility of liposomes as affected by antihelmolytic compounds. Biochim Biophys Acta. 1974 Feb 26;339(1):146–155. doi: 10.1016/0005-2736(74)90340-x. [DOI] [PubMed] [Google Scholar]
- Blok M. C., van Deenen L. L., De Gier J. Effect of the gel to liquid crystalline phase transition on the osmotic behaviour of phosphatidylcholine liposomes. Biochim Biophys Acta. 1976 Apr 16;433(1):1–12. doi: 10.1016/0005-2736(76)90172-3. [DOI] [PubMed] [Google Scholar]
- Cohen F. S., Akabas M. H., Finkelstein A. Osmotic swelling of phospholipid vesicles causes them to fuse with a planar phospholipid bilayer membrane. Science. 1982 Jul 30;217(4558):458–460. doi: 10.1126/science.6283637. [DOI] [PubMed] [Google Scholar]
- Evans E. A., Waugh R. Osmotic correction to elastic area compressibility measurements on red cell membrane. Biophys J. 1977 Dec;20(3):307–313. doi: 10.1016/S0006-3495(77)85551-3. [DOI] [PMC free article] [PubMed] [Google Scholar]
- Evans E, Rawicz W. Entropy-driven tension and bending elasticity in condensed-fluid membranes. Phys Rev Lett. 1990 Apr 23;64(17):2094–2097. doi: 10.1103/PhysRevLett.64.2094. [DOI] [PubMed] [Google Scholar]
- Farge E., Devaux P. F. Shape changes of giant liposomes induced by an asymmetric transmembrane distribution of phospholipids. Biophys J. 1992 Feb;61(2):347–357. doi: 10.1016/S0006-3495(92)81841-6. [DOI] [PMC free article] [PubMed] [Google Scholar]
- Gruner S. M., Lenk R. P., Janoff A. S., Ostro M. J. Novel multilayered lipid vesicles: comparison of physical characteristics of multilamellar liposomes and stable plurilamellar vesicles. Biochemistry. 1985 Jun 4;24(12):2833–2842. doi: 10.1021/bi00333a004. [DOI] [PubMed] [Google Scholar]
- Haines T. H., Li W., Green M., Cummins H. Z. The elasticity of uniform, unilamellar vesicles of acidic phospholipids during osmotic swelling is dominated by the ionic strength of the media. Biochemistry. 1987 Aug 25;26(17):5439–5447. doi: 10.1021/bi00391a034. [DOI] [PubMed] [Google Scholar]
- Hampton R. Y., Holz R. W. Effects of changes in osmolality on the stability and function of cultured chromaffin cells and the possible role of osmotic forces in exocytosis. J Cell Biol. 1983 Apr;96(4):1082–1088. doi: 10.1083/jcb.96.4.1082. [DOI] [PMC free article] [PubMed] [Google Scholar]
- Johnson S. M., Buttress N. The osmotic insensitivity of sonicated liposomes and the density of phospholipid-cholesterol mixtures. Biochim Biophys Acta. 1973 Apr 25;307(1):20–26. doi: 10.1016/0005-2736(73)90021-7. [DOI] [PubMed] [Google Scholar]
- Li W., Aurora T. S., Haines T. H., Cummins H. Z. Elasticity of synthetic phospholipid vesicles and submitochondrial particles during osmotic swelling. Biochemistry. 1986 Dec 16;25(25):8220–8229. doi: 10.1021/bi00373a015. [DOI] [PubMed] [Google Scholar]
- Li W., Haines T. H. Uniform preparations of large unilamellar vesicles containing anionic lipids. Biochemistry. 1986 Nov 18;25(23):7477–7483. doi: 10.1021/bi00371a033. [DOI] [PubMed] [Google Scholar]
- Martinac B., Buechner M., Delcour A. H., Adler J., Kung C. Pressure-sensitive ion channel in Escherichia coli. Proc Natl Acad Sci U S A. 1987 Apr;84(8):2297–2301. doi: 10.1073/pnas.84.8.2297. [DOI] [PMC free article] [PubMed] [Google Scholar]
- Mayer L. D., Hope M. J., Cullis P. R., Janoff A. S. Solute distributions and trapping efficiencies observed in freeze-thawed multilamellar vesicles. Biochim Biophys Acta. 1985 Jul 11;817(1):193–196. doi: 10.1016/0005-2736(85)90084-7. [DOI] [PubMed] [Google Scholar]
- Mayer L. D., Hope M. J., Cullis P. R. Vesicles of variable sizes produced by a rapid extrusion procedure. Biochim Biophys Acta. 1986 Jun 13;858(1):161–168. doi: 10.1016/0005-2736(86)90302-0. [DOI] [PubMed] [Google Scholar]
- Miao L, Fourcade B, Rao M, Wortis M, Zia RK. Equilibrium budding and vesiculation in the curvature model of fluid lipid vesicles. Phys Rev A. 1991 Jun 15;43(12):6843–6856. doi: 10.1103/physreva.43.6843. [DOI] [PubMed] [Google Scholar]
- Miyamoto S., Maeda T., Fujime S. Change in membrane elastic modulus on activation of glucose transport system of brush border membrane vesicles studied by osmotic swelling and dynamic light scattering. Biophys J. 1988 Apr;53(4):505–512. doi: 10.1016/S0006-3495(88)83130-8. [DOI] [PMC free article] [PubMed] [Google Scholar]
- Morris C. E. Mechanosensitive ion channels. J Membr Biol. 1990 Feb;113(2):93–107. doi: 10.1007/BF01872883. [DOI] [PubMed] [Google Scholar]
- Near J. A., Szoka F. C., Jr, Olsen R., Ettinger M. J. Photoaffinity labeling of three renal cyclic 3',5'-adenosine monophosphate-binding proteins. Biochim Biophys Acta. 1979 Nov 1;587(4):522–539. doi: 10.1016/0304-4165(79)90006-0. [DOI] [PubMed] [Google Scholar]
- Needham D., Nunn R. S. Elastic deformation and failure of lipid bilayer membranes containing cholesterol. Biophys J. 1990 Oct;58(4):997–1009. doi: 10.1016/S0006-3495(90)82444-9. [DOI] [PMC free article] [PubMed] [Google Scholar]
- Pollard H. B., Pazoles C. J., Creutz C. E., Scott J. H., Zinder O., Hotchkiss A. An osmotic mechanism for exocytosis from dissociated chromaffin cells. J Biol Chem. 1984 Jan 25;259(2):1114–1121. [PubMed] [Google Scholar]
- Rutkowski C. A., Williams L. M., Haines T. H., Cummins H. Z. The elasticity of synthetic phospholipid vesicles obtained by photon correlation spectroscopy. Biochemistry. 1991 Jun 11;30(23):5688–5696. doi: 10.1021/bi00237a008. [DOI] [PubMed] [Google Scholar]
- Sackmann E., Duwe H. P., Engelhardt H. Membrane bending elasticity and its role for shape fluctuations and shape transformations of cells and vesicles. Faraday Discuss Chem Soc. 1986;(81):281–290. doi: 10.1039/dc9868100281. [DOI] [PubMed] [Google Scholar]
- Szoka F., Olson F., Heath T., Vail W., Mayhew E., Papahadjopoulos D. Preparation of unilamellar liposomes of intermediate size (0.1-0.2 mumol) by a combination of reverse phase evaporation and extrusion through polycarbonate membranes. Biochim Biophys Acta. 1980 Oct 2;601(3):559–571. doi: 10.1016/0005-2736(80)90558-1. [DOI] [PubMed] [Google Scholar]
- Talmon Y., Burns J. L., Chestnut M. H., Siegel D. P. Time-resolved cryotransmission electron microscopy. J Electron Microsc Tech. 1990 Jan;14(1):6–12. doi: 10.1002/jemt.1060140103. [DOI] [PubMed] [Google Scholar]
- Uster P. S., Deamer D. W. Fusion competence of phosphatidylserine-containing liposomes quantitatively measured by a fluorescence resonance energy transfer assay. Arch Biochem Biophys. 1981 Jul;209(2):385–395. doi: 10.1016/0003-9861(81)90296-4. [DOI] [PubMed] [Google Scholar]
- Weinstein J. N., Klausner R. D., Innerarity T., Ralston E., Blumenthal R. Phase transition release, a new approach to the interaction of proteins with lipid vesicles. Application to lipoproteins. Biochim Biophys Acta. 1981 Oct 2;647(2):270–284. doi: 10.1016/0005-2736(81)90255-8. [DOI] [PubMed] [Google Scholar]
- Yang X. C., Sachs F. Block of stretch-activated ion channels in Xenopus oocytes by gadolinium and calcium ions. Science. 1989 Feb 24;243(4894 Pt 1):1068–1071. doi: 10.1126/science.2466333. [DOI] [PubMed] [Google Scholar]