Abstract
Small unilamellar vesicles (SUV) were prepared from the total lipid extract of Mycoplasma capricolum. The SUV were labeled with the fluorescent probe octadecylrhodamine B chloride (R18) to a level at which the R18 fluorescence was self-quenched. At pH 7.4 and 37 degrees C, and in the presence of 5% polyethylene glycol, an increase in the R18 fluorescence with time was observed when the R18-labeled SUV were introduced to a native M. capricolum cell suspension. The fluorescence dequenching resulting from dilution of the R18 into the unlabeled membranes of M. capricolum, was interpreted as a result of lipid mixing during fusion between the SUV and the mycoplasma cells. The presence of cholesterol in the SUV was found to be obligatory to allow SUV-mycoplasma fusion to occur. Adaptation of M. capricolum cells to grow in a medium containing low cholesterol concentration provided cells in which the unesterified cholesterol content was as low as 17 micrograms/mg cell protein. The fusion activity of the adapted cells was very low or nonexistent. Nonetheless, when an early exponential phase culture of the adapted cells was transferred to a cholesterol-rich medium, the cells accumulated cholesterol and regained their fusogenic activity. The cholesterol requirement for fusion in the target mycoplasma membrane was met by a variety of planar sterols having a free beta-hydroxyl group, but differing in the aliphatic side chain, e.g., beta-sitosterol or ergosterol, even though these sterols, having a bulky side chain, are preferentially localized in the outer leaflet of the lipid bilayer. It is suggested that the role of cholesterol in mycoplasma-SUV fusion is not at the level of bulk bilayer viscosity but rather, affecting local lipid-lipid or lipid-protein interactions that are relevant to the fusion event.
Full text
PDF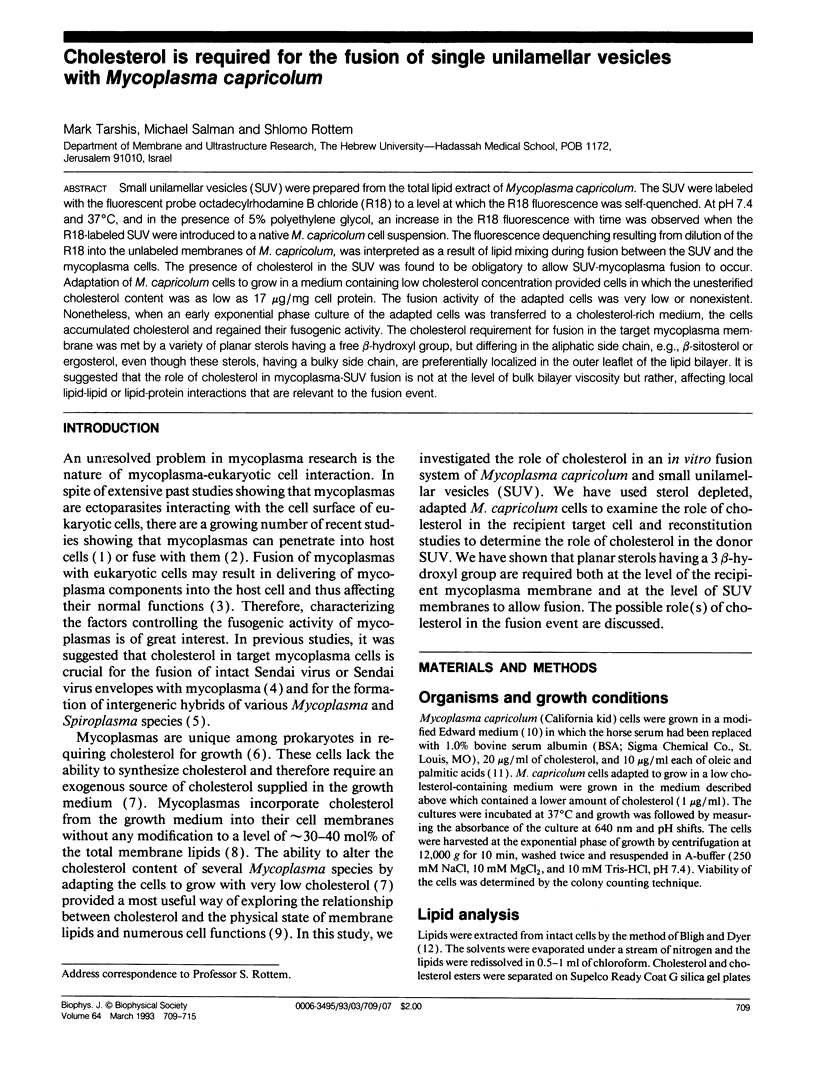
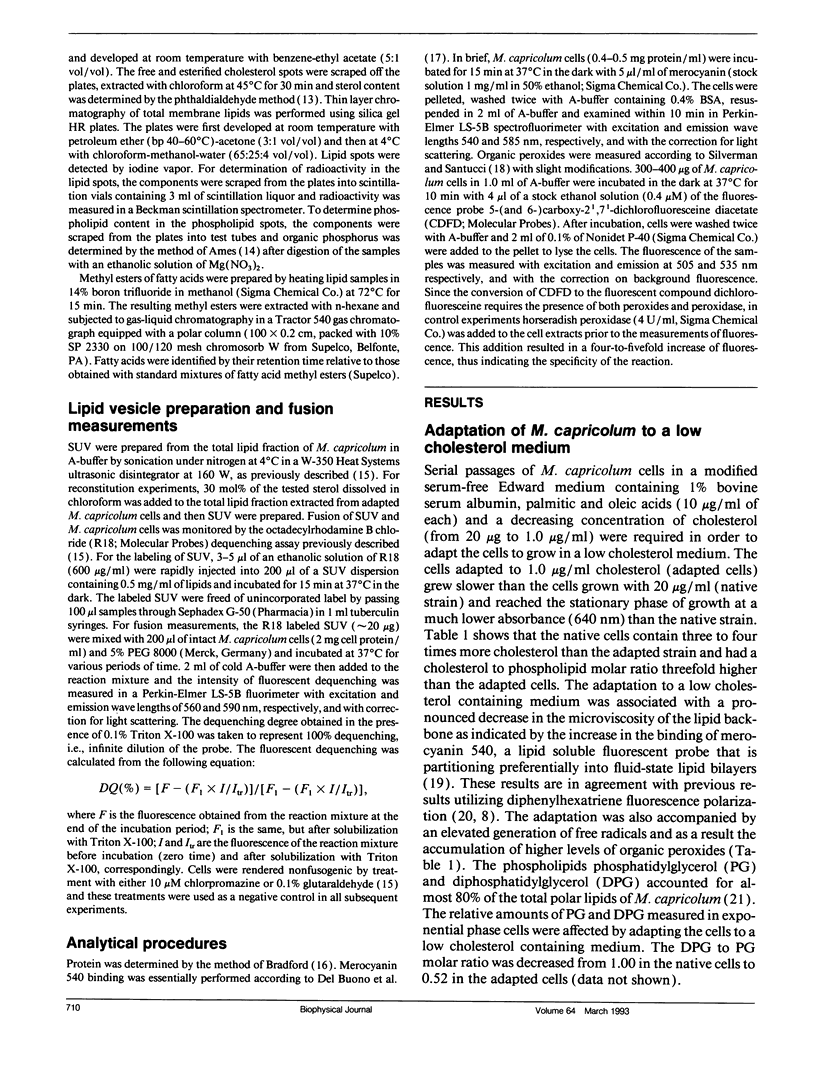
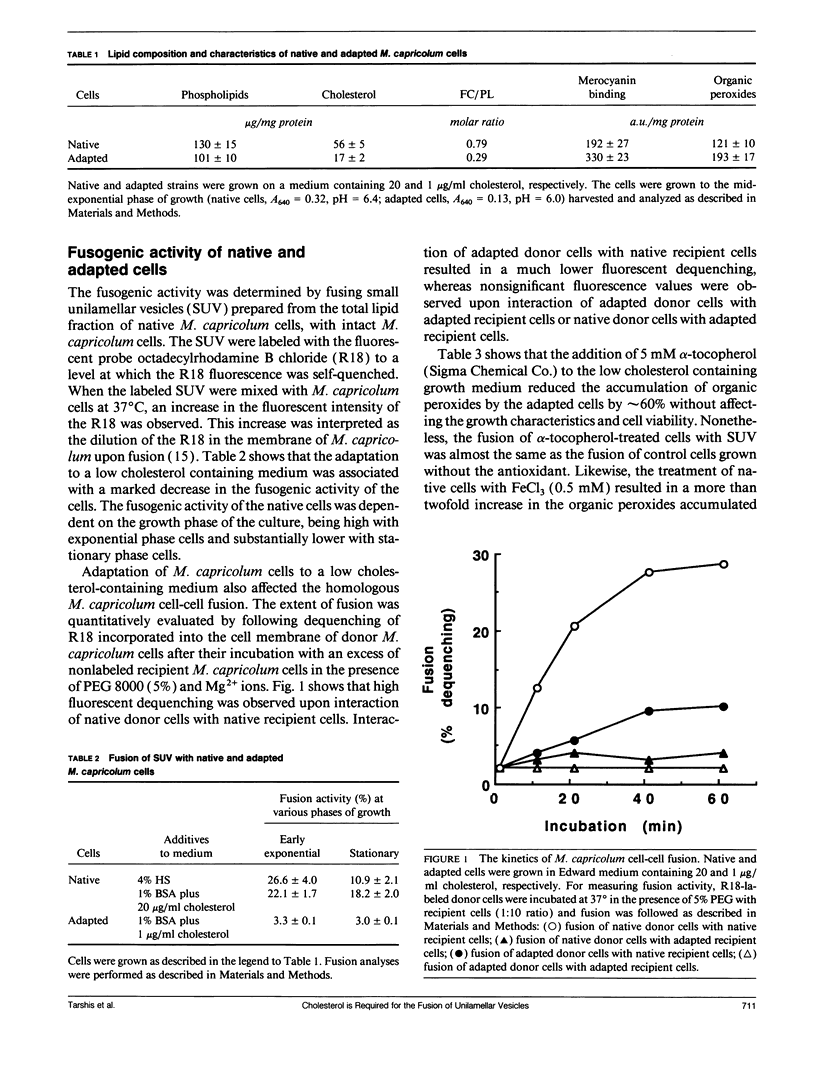
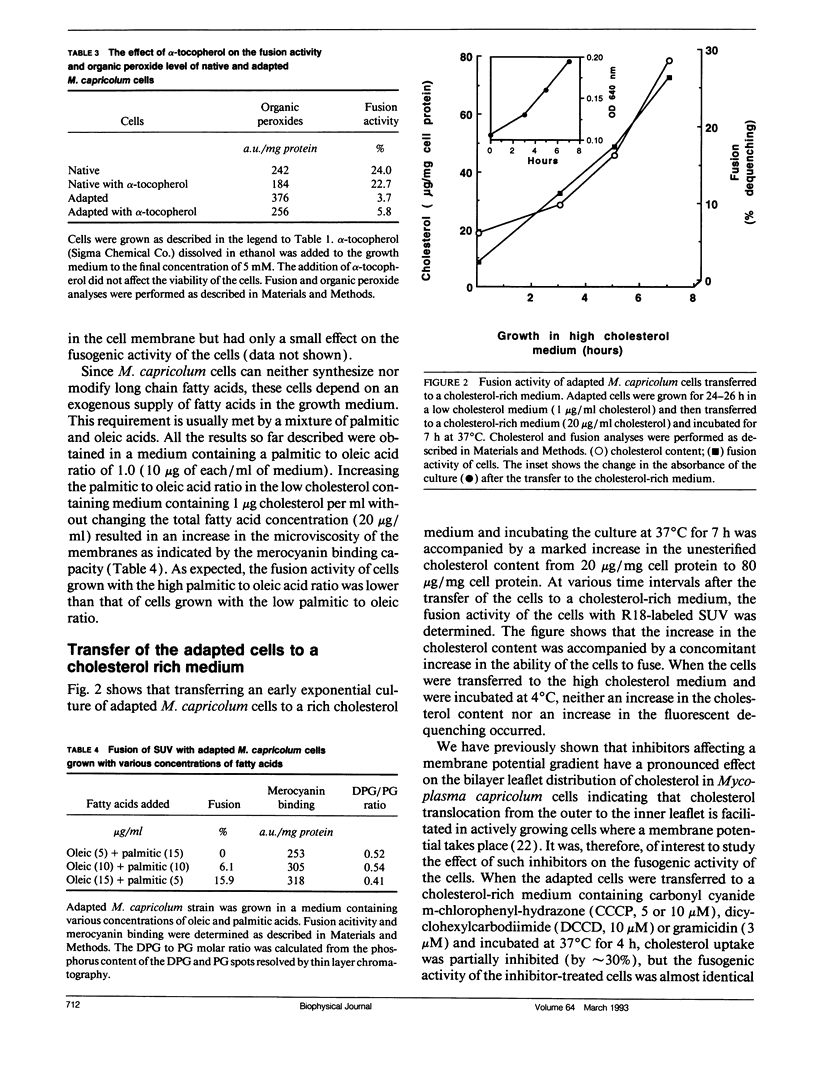
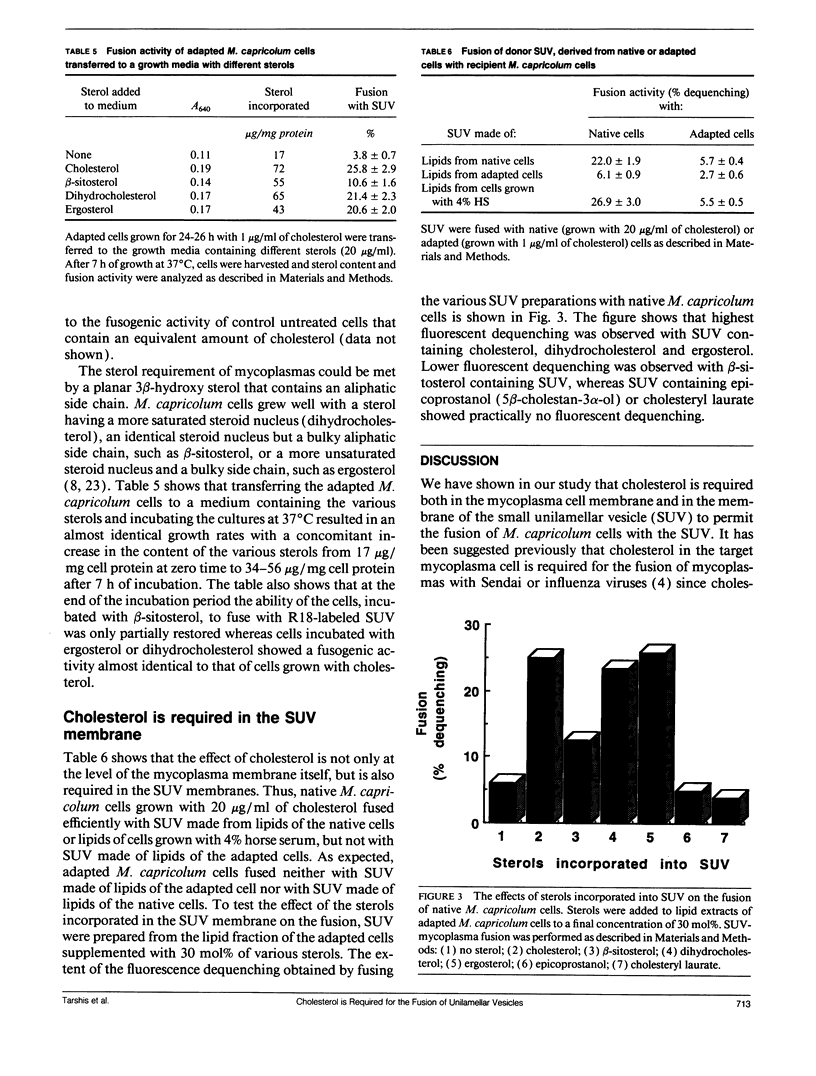
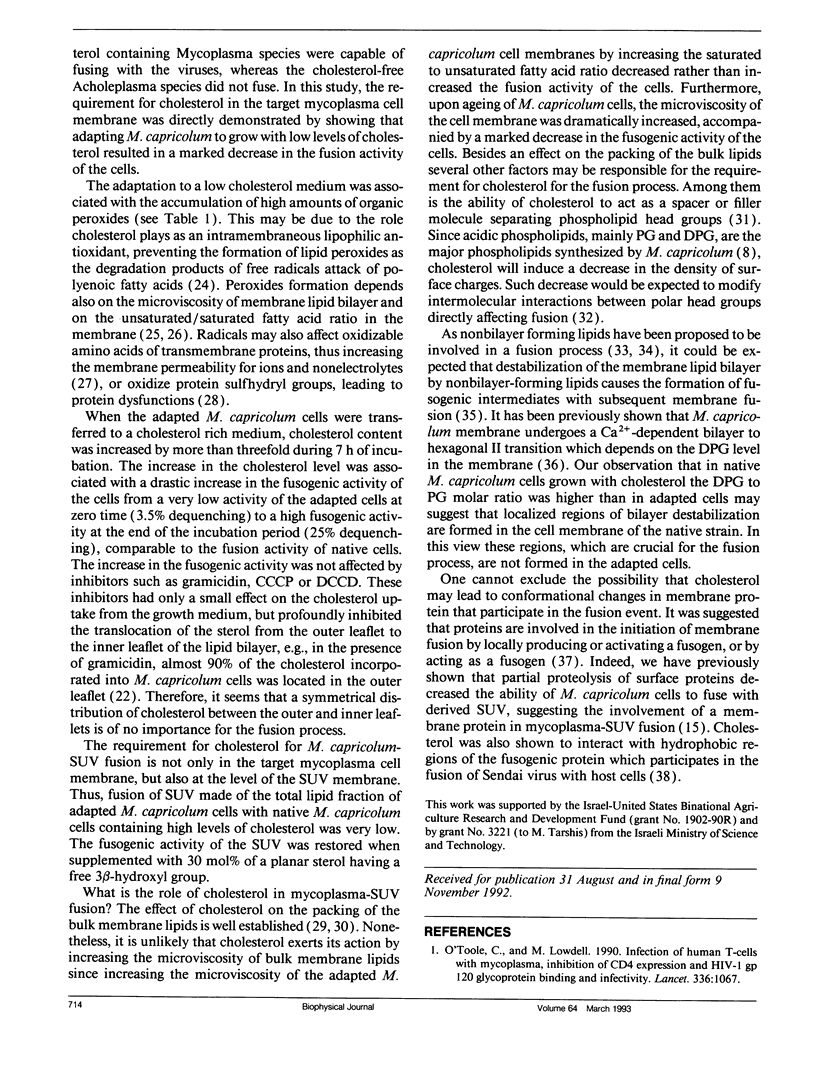
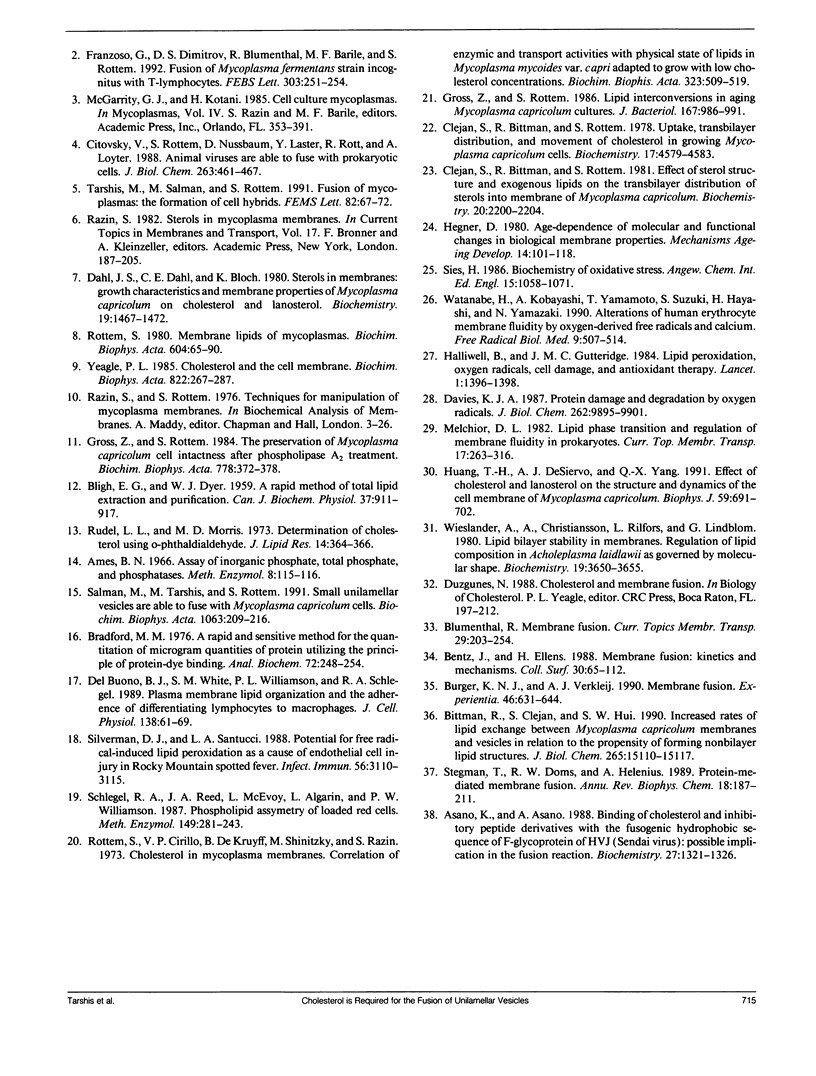
Selected References
These references are in PubMed. This may not be the complete list of references from this article.
- Asano K., Asano A. Binding of cholesterol and inhibitory peptide derivatives with the fusogenic hydrophobic sequence of F-glycoprotein of HVJ (Sendai virus): possible implication in the fusion reaction. Biochemistry. 1988 Feb 23;27(4):1321–1329. doi: 10.1021/bi00404a035. [DOI] [PubMed] [Google Scholar]
- BLIGH E. G., DYER W. J. A rapid method of total lipid extraction and purification. Can J Biochem Physiol. 1959 Aug;37(8):911–917. doi: 10.1139/o59-099. [DOI] [PubMed] [Google Scholar]
- Bittman R., Clejan S., Hui S. W. Increased rates of lipid exchange between Mycoplasma capricolum membranes and vesicles in relation to the propensity of forming nonbilayer lipid structures. J Biol Chem. 1990 Sep 5;265(25):15110–15117. [PubMed] [Google Scholar]
- Bradford M. M. A rapid and sensitive method for the quantitation of microgram quantities of protein utilizing the principle of protein-dye binding. Anal Biochem. 1976 May 7;72:248–254. doi: 10.1006/abio.1976.9999. [DOI] [PubMed] [Google Scholar]
- Burger K. N., Verkleij A. J. Membrane fusion. Experientia. 1990 Jun 15;46(6):631–644. doi: 10.1007/BF01939702. [DOI] [PubMed] [Google Scholar]
- Citovsky V., Rottem S., Nussbaum O., Laster Y., Rott R., Loyter A. Animal viruses are able to fuse with prokaryotic cells. Fusion between Sendai or influenza virions and Mycoplasma. J Biol Chem. 1988 Jan 5;263(1):461–467. [PubMed] [Google Scholar]
- Clejan S., Bittman R., Rottem S. Effects of sterol structure and exogenous lipids on the transbilayer distribution of sterols in the membrane of Mycoplasma capricolum. Biochemistry. 1981 Apr 14;20(8):2200–2204. doi: 10.1021/bi00511a019. [DOI] [PubMed] [Google Scholar]
- Clejan S., Bittman R., Rottem S. Uptake, transbilayer distribution, and movement of cholesterol in growing Mycoplasma capricolum cells. Biochemistry. 1978 Oct 31;17(22):4579–4583. doi: 10.1021/bi00615a001. [DOI] [PubMed] [Google Scholar]
- Dahl J. S., Dahl C. E., Bloch K. Sterols in membranes: growth characteristics and membrane properties of Mycoplasma capricolum cultured on cholesterol and lanosterol. Biochemistry. 1980 Apr 1;19(7):1467–1472. doi: 10.1021/bi00548a032. [DOI] [PubMed] [Google Scholar]
- Davies K. J. Protein damage and degradation by oxygen radicals. I. general aspects. J Biol Chem. 1987 Jul 15;262(20):9895–9901. [PubMed] [Google Scholar]
- Del Buono B. J., White S. M., Williamson P. L., Schlegel R. A. Plasma membrane lipid organization and the adherence of differentiating lymphocytes to macrophages. J Cell Physiol. 1989 Jan;138(1):61–69. doi: 10.1002/jcp.1041380110. [DOI] [PubMed] [Google Scholar]
- Franzoso G., Dimitrov D. S., Blumenthal R., Barile M. F., Rottem S. Fusion of Mycoplasma fermentans strain incognitus with T-lymphocytes. FEBS Lett. 1992 Jun 1;303(2-3):251–254. doi: 10.1016/0014-5793(92)80531-k. [DOI] [PubMed] [Google Scholar]
- Gross Z., Rottem S. Lipid interconversions in aging Mycoplasma capricolum cultures. J Bacteriol. 1986 Sep;167(3):986–991. doi: 10.1128/jb.167.3.986-991.1986. [DOI] [PMC free article] [PubMed] [Google Scholar]
- Halliwell B., Gutteridge J. M. Lipid peroxidation, oxygen radicals, cell damage, and antioxidant therapy. Lancet. 1984 Jun 23;1(8391):1396–1397. doi: 10.1016/s0140-6736(84)91886-5. [DOI] [PubMed] [Google Scholar]
- Hegner D. Age-dependence of molecular and functional changes in biological membrane properties. Mech Ageing Dev. 1980 Sep-Oct;14(1-2):101–118. doi: 10.1016/0047-6374(80)90109-8. [DOI] [PubMed] [Google Scholar]
- Huang T. H., DeSiervo A. J., Yang Q. X. Effect of cholesterol and lanosterol on the structure and dynamics of the cell membrane of Mycoplasma capricolum. Deuterium nuclear magnetic resonance study. Biophys J. 1991 Mar;59(3):691–702. doi: 10.1016/S0006-3495(91)82283-4. [DOI] [PMC free article] [PubMed] [Google Scholar]
- O'Toole C., Lowdell M. Infection of human T cells with mycoplasma, inhibition of CD4 expression and HIV-1 gp120 glycoprotein binding, and infectivity. Lancet. 1990 Oct 27;336(8722):1067–1067. doi: 10.1016/0140-6736(90)92535-p. [DOI] [PubMed] [Google Scholar]
- Rottem S., Cirillo V. P., de Kruyff B., Shinitzky M., Razin S. Cholesterol in mycoplasma membranes. Correlation of enzymic and transport activities with physical state of lipids in membranes of Mycoplasma mycoides var. capri adapted to grow with low cholesterol concentrations. Biochim Biophys Acta. 1973 Nov 16;323(4):509–519. doi: 10.1016/0005-2736(73)90159-4. [DOI] [PubMed] [Google Scholar]
- Rottem S. Membrane lipids of mycoplasmas. Biochim Biophys Acta. 1980 May 27;604(1):65–90. doi: 10.1016/0005-2736(80)90585-4. [DOI] [PubMed] [Google Scholar]
- Rudel L. L., Morris M. D. Determination of cholesterol using o-phthalaldehyde. J Lipid Res. 1973 May;14(3):364–366. [PubMed] [Google Scholar]
- Salman M., Tarshis M., Rottem S. Small unilamellar vesicles are able to fuse with Mycoplasma capricolum cells. Biochim Biophys Acta. 1991 Apr 2;1063(2):209–216. doi: 10.1016/0005-2736(91)90373-g. [DOI] [PubMed] [Google Scholar]
- Schlegel R. A., Reed J. A., McEvoy L., Algarin L., Williamson P. Phospholipid asymmetry of loaded red cells. Methods Enzymol. 1987;149:281–293. doi: 10.1016/0076-6879(87)49066-6. [DOI] [PubMed] [Google Scholar]
- Silverman D. J., Santucci L. A. Potential for free radical-induced lipid peroxidation as a cause of endothelial cell injury in Rocky Mountain spotted fever. Infect Immun. 1988 Dec;56(12):3110–3115. doi: 10.1128/iai.56.12.3110-3115.1988. [DOI] [PMC free article] [PubMed] [Google Scholar]
- Stegmann T., Doms R. W., Helenius A. Protein-mediated membrane fusion. Annu Rev Biophys Biophys Chem. 1989;18:187–211. doi: 10.1146/annurev.bb.18.060189.001155. [DOI] [PubMed] [Google Scholar]
- Tarshis M., Salman M., Rottem S. Fusion of mycoplasmas: the formation of cell hybrids. FEMS Microbiol Lett. 1991 Jul 15;66(1):67–71. doi: 10.1016/0378-1097(91)90422-7. [DOI] [PubMed] [Google Scholar]
- Watanabe H., Kobayashi A., Yamamoto T., Suzuki S., Hayashi H., Yamazaki N. Alterations of human erythrocyte membrane fluidity by oxygen-derived free radicals and calcium. Free Radic Biol Med. 1990;8(6):507–514. doi: 10.1016/0891-5849(90)90150-h. [DOI] [PubMed] [Google Scholar]
- Wieslander A., Christiansson A., Rilfors L., Lindblom G. Lipid bilayer stability in membranes. Regulation of lipid composition in Acholeplasma laidlawii as governed by molecular shape. Biochemistry. 1980 Aug 5;19(16):3650–3655. doi: 10.1021/bi00557a002. [DOI] [PubMed] [Google Scholar]
- Yeagle P. L. Cholesterol and the cell membrane. Biochim Biophys Acta. 1985 Dec 9;822(3-4):267–287. doi: 10.1016/0304-4157(85)90011-5. [DOI] [PubMed] [Google Scholar]