Abstract
1. The kinetics of de-repression of alkaline phosphatase in Bacillus subtilis were studied after the removal of Pi. Enzyme activity appeared about 10min. after removal of Pi, whereas `enzyme-forming potential' appeared after 6min. 2. Protein synthesis is not impaired for at least 20min. on removal of Pi, but RNA synthesis is considerably diminished. 3. Adding chloramphenicol to cells without Pi, just at the time they start to make enzyme-forming potential, does not affect the differential rate of enzyme synthesis compared with total protein. Enzyme-forming potential accumulates to about normal levels in the presence of chloramphenicol, even though peptide-bond formation is inhibited by more than 95%. 4. Similar experiments performed with actinomycin C show more complex effects. Actinomycin initially prevents RNA synthesis and also the synthesis of enzyme-forming potential. After some minutes RNA synthesis resumes at a low rate, to be followed 4min. later by enzyme synthesis. Enzyme-forming potential can accumulate in the presence of actinomycin after the resumption of RNA synthesis. Protein synthesis, initially inhibited by actinomycin as a consequence of the effect on RNA synthesis, is later directly inhibited by actinomycin. 5. Adding actinomycin to de-repressed cells already making enzyme stops enzyme synthesis within 4–5min. Enzyme synthesis resumes, as before, 4min. after the resumption of RNA synthesis. 6. Adding Pi together with actinomycin to de-repressed cells synthesizing enzyme does not result in a lower yield of enzyme compared with actinomycin alone. 7. Actinomycin is less effective an inhibitor of RNA and protein synthesis in Pi-starved cells if Pi is also added. 8. These results are discussed in view of the three main models for the regulation of enzyme induction: regulation at the level of transcription only, at translation only, or a coupled model in which transcription requires concomitant translation. It is concluded that the present evidence most powerfully supports the model of transcriptional regulation.
Full text
PDF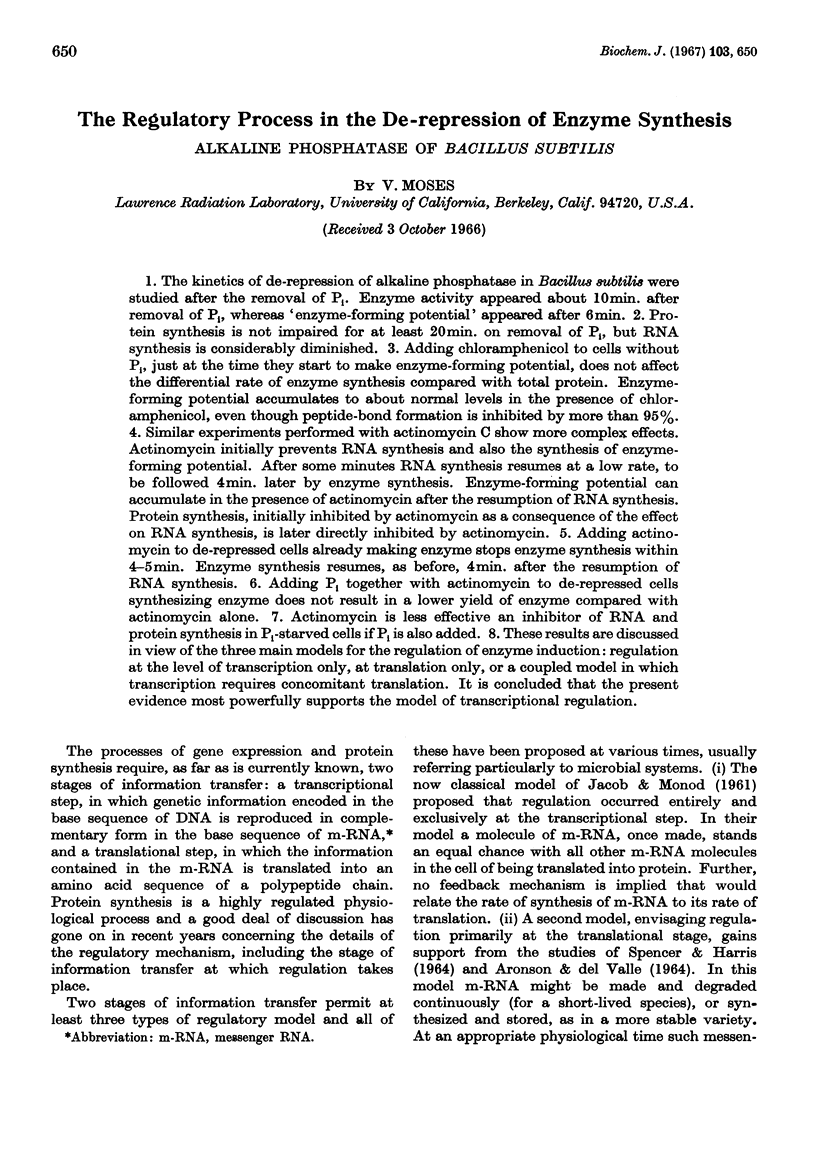
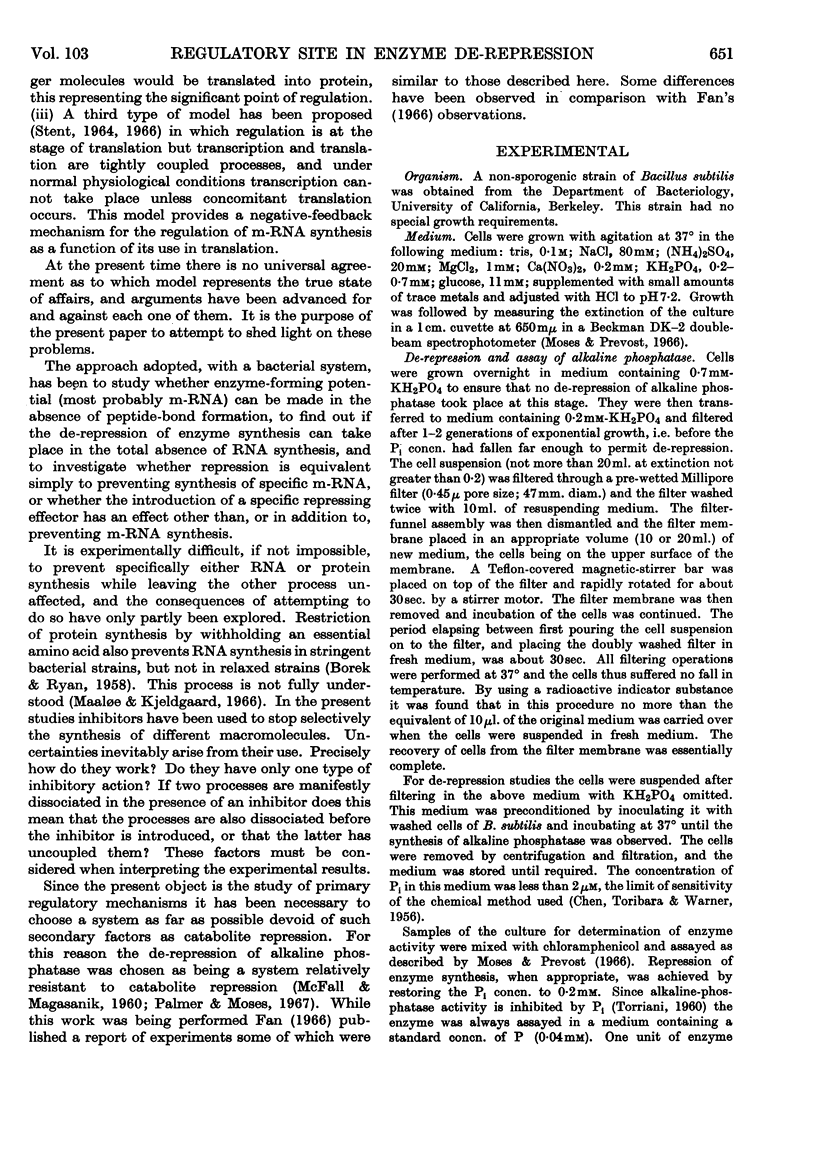
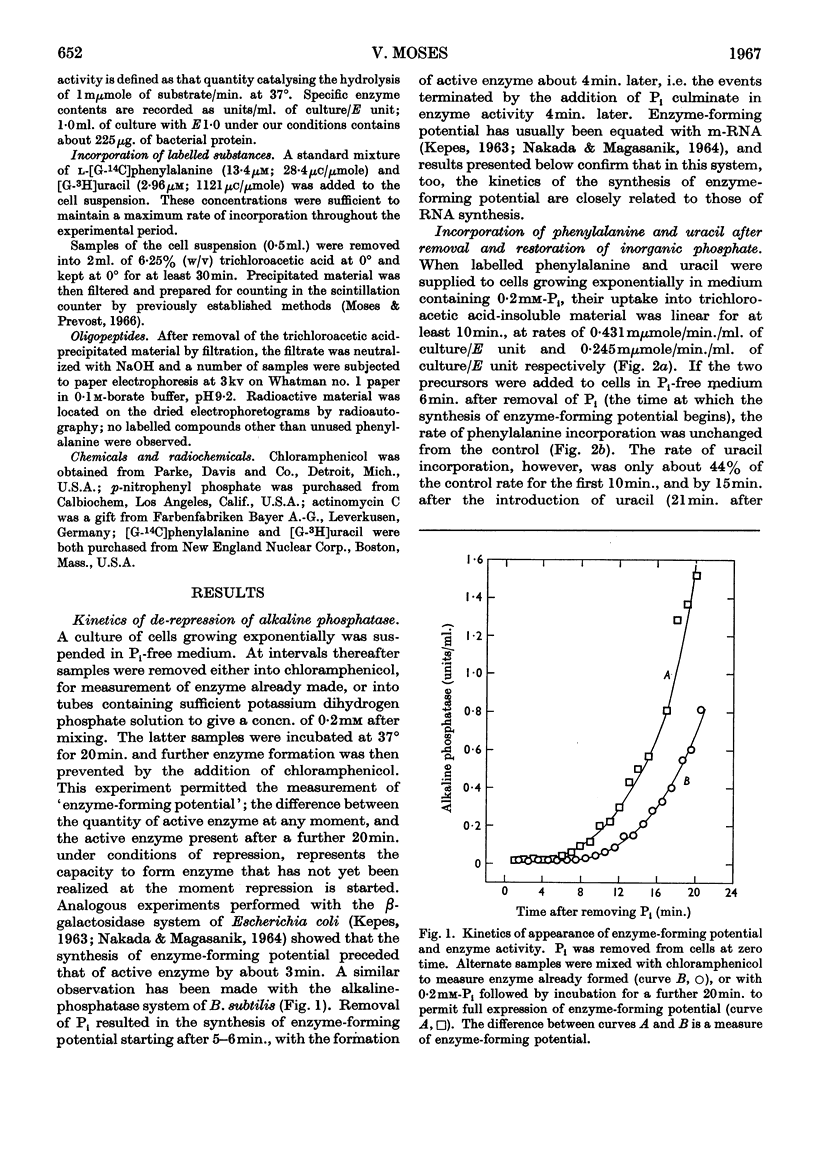
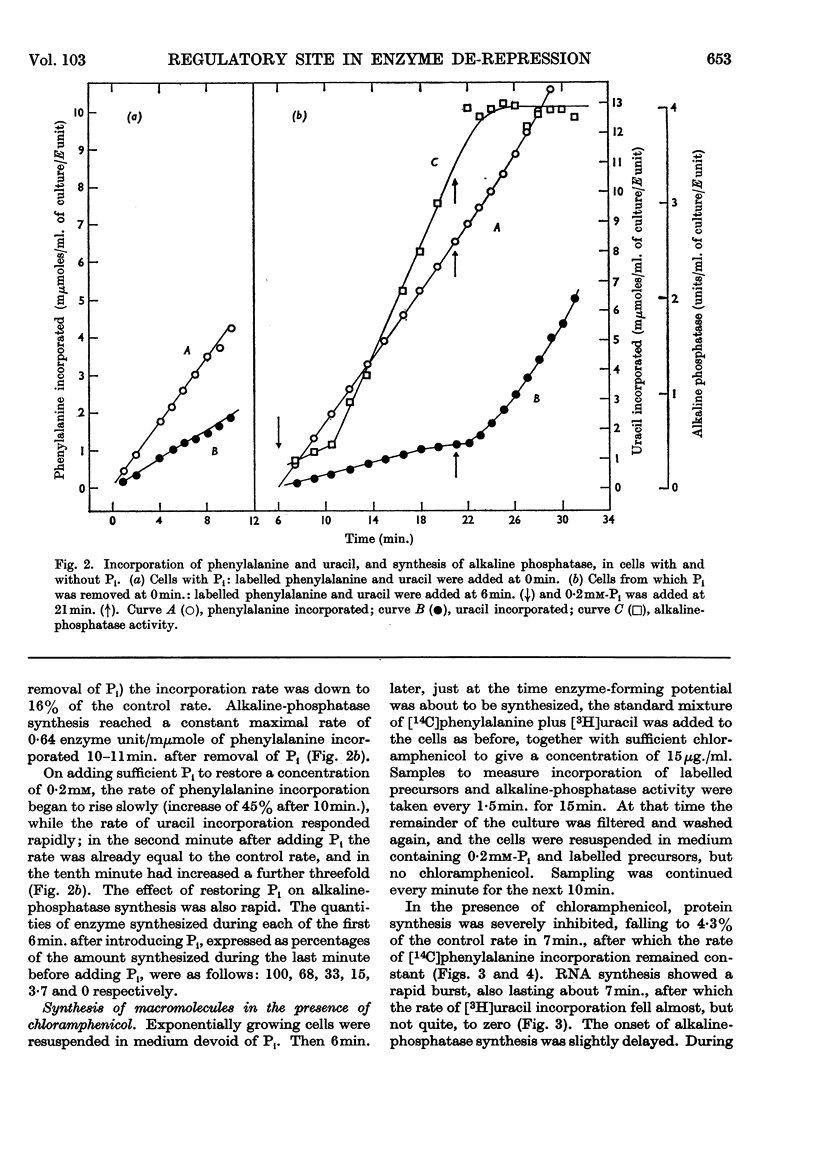
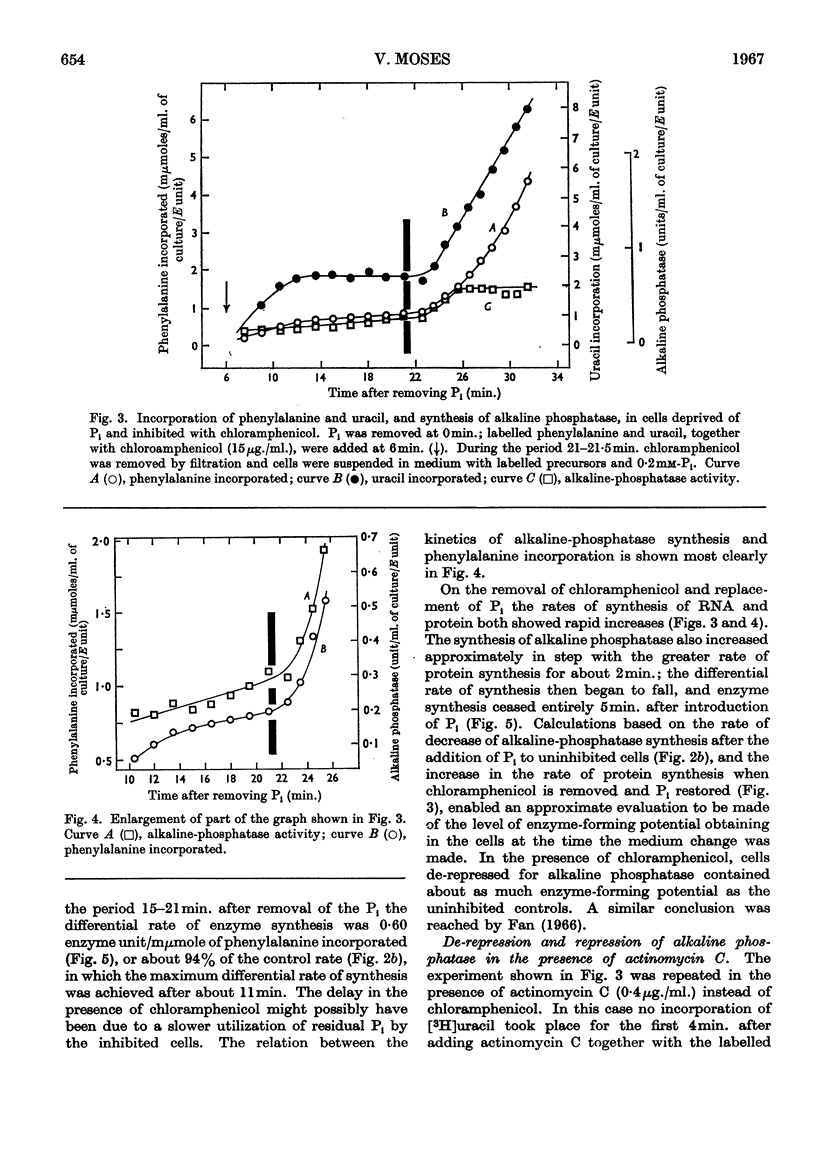
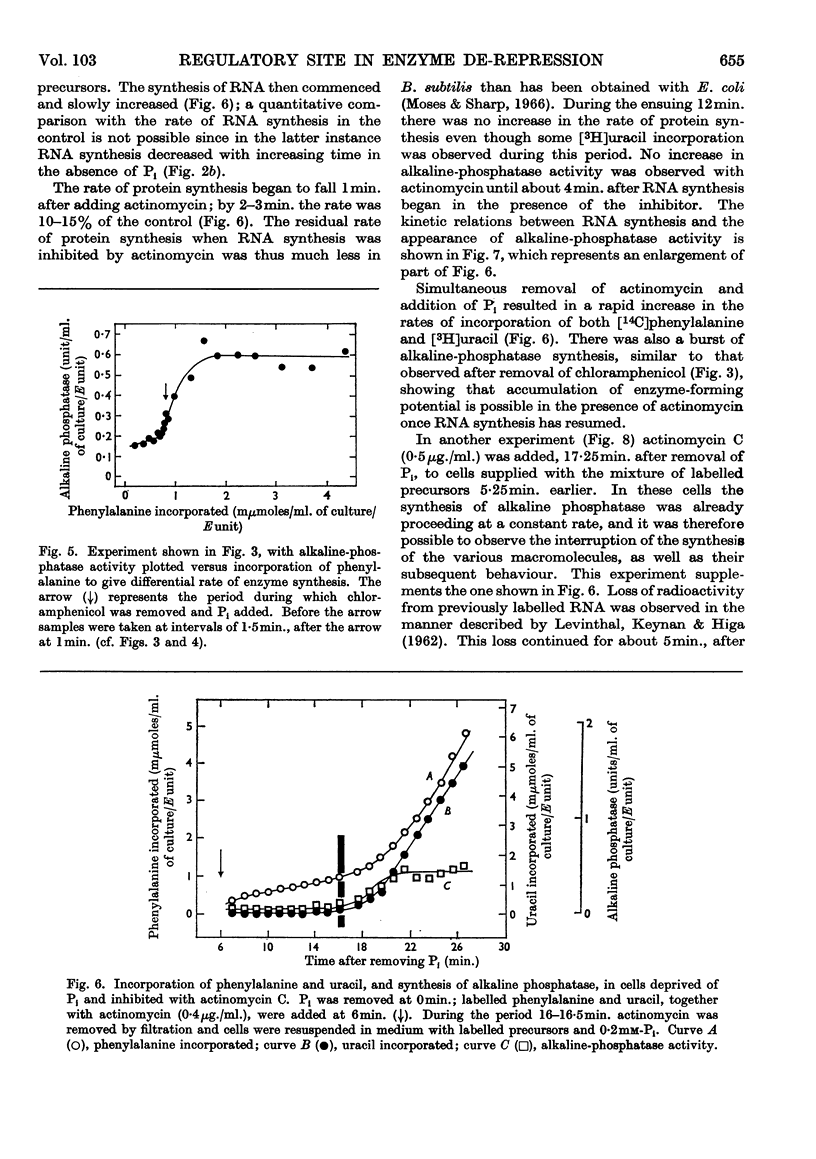
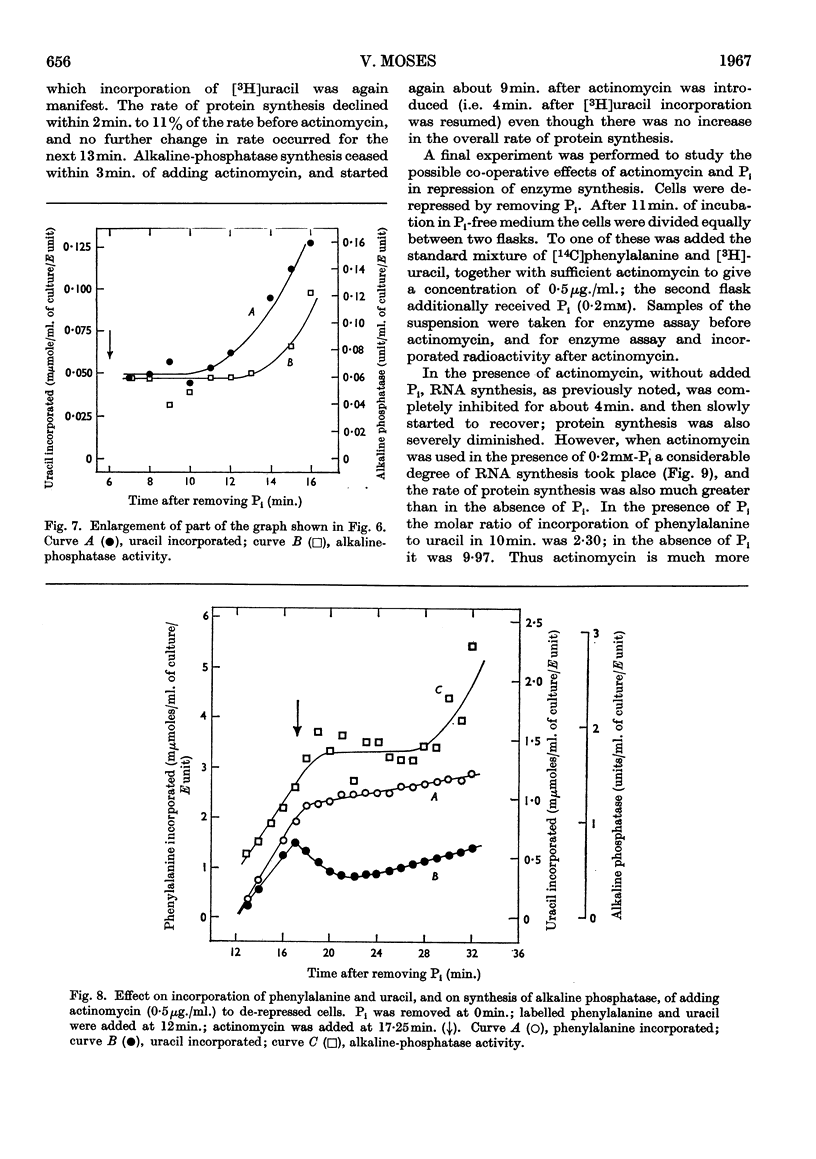
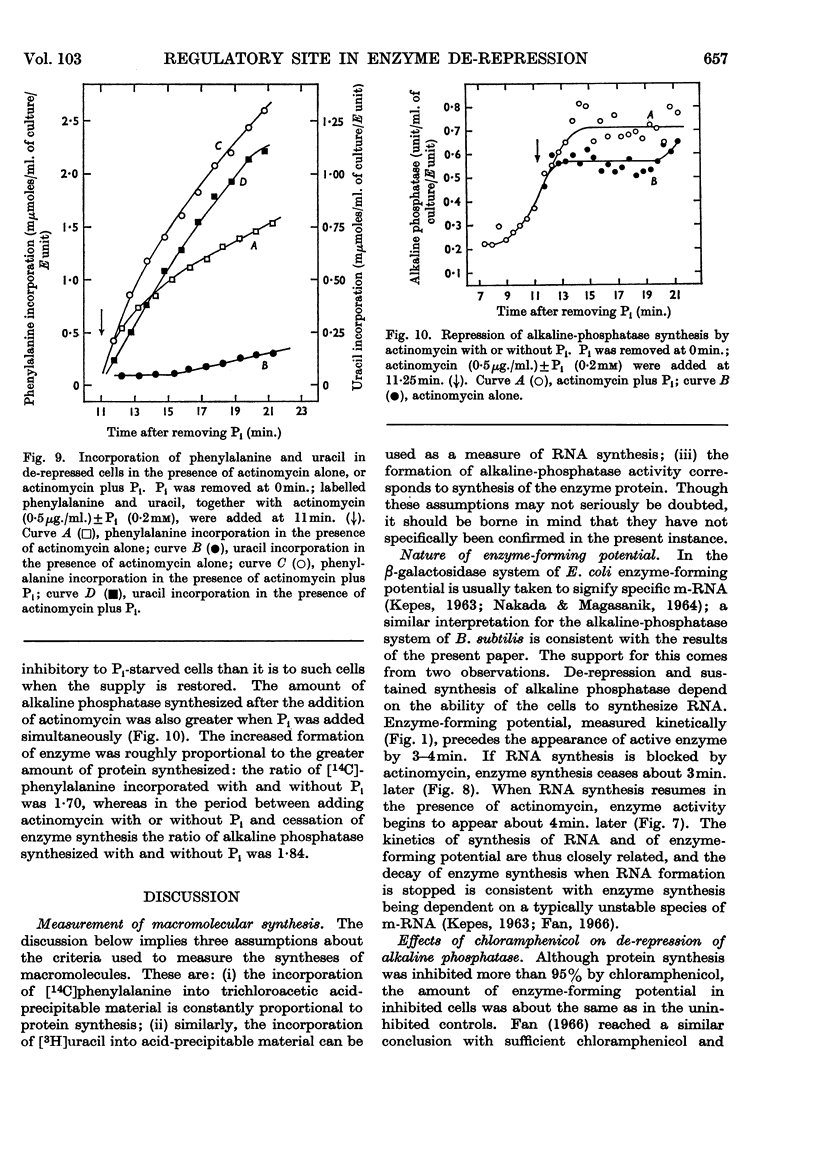
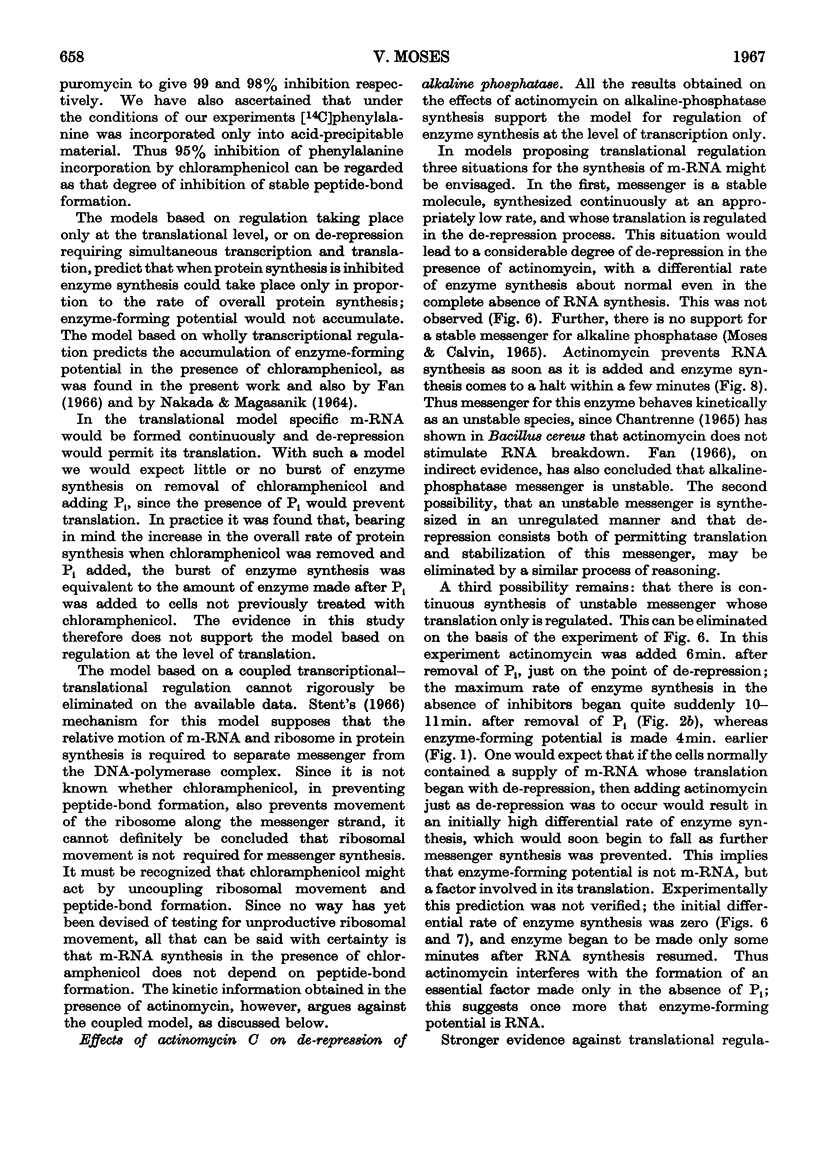
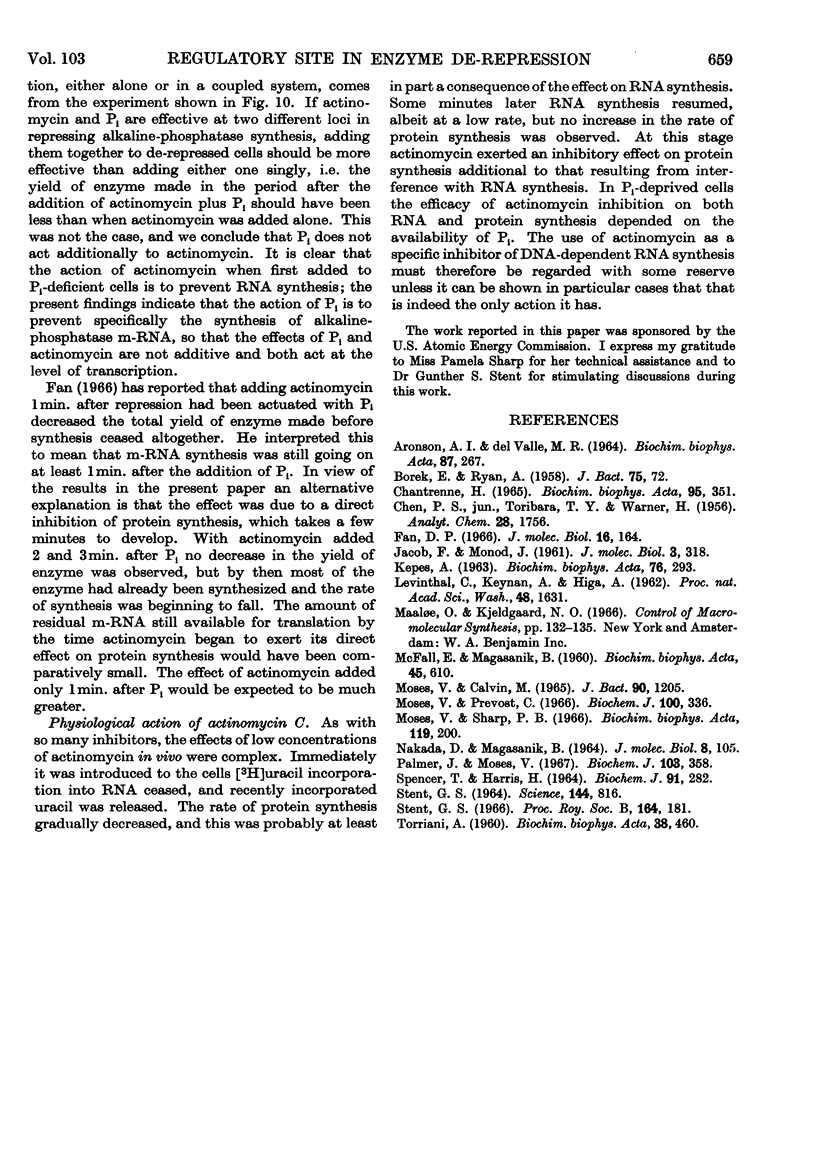
Selected References
These references are in PubMed. This may not be the complete list of references from this article.
- ARONSON A. I., ROSASDELVALLE M. RNA AND PROTEIN SYNTHESIS REQUIRED FOR BACTERIAL SPORE FORMATION. Biochim Biophys Acta. 1964 Jun 22;87:267–276. doi: 10.1016/0926-6550(64)90222-1. [DOI] [PubMed] [Google Scholar]
- BOREK E., RYAN A. Studies on a mutant of Escherichia coli with unbalanced ribonucleic acid synthesis. II. The concomitance of ribonucleic acid synthesis with resumed protein synthesis. J Bacteriol. 1958 Jan;75(1):72–76. doi: 10.1128/jb.75.1.72-76.1958. [DOI] [PMC free article] [PubMed] [Google Scholar]
- CHANTRENNE H. ON THE USE OF ACTINOMYCIN FOR OBSERVING THE TURNOVER OF RIBONUCLEIC ACID. Biochim Biophys Acta. 1965 Feb 8;95:351–353. doi: 10.1016/0005-2787(65)90499-5. [DOI] [PubMed] [Google Scholar]
- JACOB F., MONOD J. Genetic regulatory mechanisms in the synthesis of proteins. J Mol Biol. 1961 Jun;3:318–356. doi: 10.1016/s0022-2836(61)80072-7. [DOI] [PubMed] [Google Scholar]
- KEPES A. KINETICS OF INDUCED ENZYME SYNTHESIS. DETERMINATION OF THE MEAN LIFE OF GALACTOSIDASE-SPECIFIC MESSENGER RNA. Biochim Biophys Acta. 1963 Oct 15;76:293–309. [PubMed] [Google Scholar]
- LEVINTHAL C., KEYNAN A., HIGA A. Messenger RNA turnover and protein synthesis in B. subtilis inhibited by actinomycin D. Proc Natl Acad Sci U S A. 1962 Sep 15;48:1631–1638. doi: 10.1073/pnas.48.9.1631. [DOI] [PMC free article] [PubMed] [Google Scholar]
- MCFALL E., MAGASANIK B. Thymine starvation and enzyme synthesis. Biochim Biophys Acta. 1960 Dec 18;45:610–612. doi: 10.1016/0006-3002(60)91505-5. [DOI] [PubMed] [Google Scholar]
- Moses V., Calvin M. Lifetime of bacterial messenger ribonucleic acid. J Bacteriol. 1965 Nov;90(5):1205–1217. doi: 10.1128/jb.90.5.1205-1217.1965. [DOI] [PMC free article] [PubMed] [Google Scholar]
- Moses V., Prevost C. Catabolite repression of beta-galactosidase synthesis in Escherichia coli. Biochem J. 1966 Aug;100(2):336–353. doi: 10.1042/bj1000336. [DOI] [PMC free article] [PubMed] [Google Scholar]
- Moses V., Sharp P. B. Effect of actinomycin on the synthesis of macromolecules in Escherichia coli. Biochim Biophys Acta. 1966 Apr 18;119(1):200–203. doi: 10.1016/0005-2787(66)90053-0. [DOI] [PubMed] [Google Scholar]
- NAKADA D., MAGASANIK B. THE ROLES OF INDUCER AND CATABOLITE REPRESSOR IN THE SYNTHESIS OF BETA-GALACTOSIDASE BY ESCHERICHIA COLI. J Mol Biol. 1964 Jan;8:105–127. doi: 10.1016/s0022-2836(64)80153-4. [DOI] [PubMed] [Google Scholar]
- Palmer J., Moses V. Involvement of the lac regulatory genes in catabolite repression in Escherichia coli. Biochem J. 1967 May;103(2):358–366. doi: 10.1042/bj1030358. [DOI] [PMC free article] [PubMed] [Google Scholar]
- STENT G. S. THE OPERON: ON ITS THIRD ANNIVERSARY. MODULATION OF TRANSFER RNA SPECIES CAN PROVIDE A WORKABLE MODEL OF AN OPERATOR-LESS OPERON. Science. 1964 May 15;144(3620):816–820. doi: 10.1126/science.144.3620.816. [DOI] [PubMed] [Google Scholar]
- Spencer T., Harris H. Regulation of enzyme synthesis in an enucleate cell. Biochem J. 1964 May;91(2):282–286. doi: 10.1042/bj0910282. [DOI] [PMC free article] [PubMed] [Google Scholar]
- Stent G. S. Genetic transcription. Proc R Soc Lond B Biol Sci. 1966 Mar 22;164(995):181–197. doi: 10.1098/rspb.1966.0022. [DOI] [PubMed] [Google Scholar]
- TORRIANI A. Influence of inorganic phosphate in the formation of phosphatases by Escherichia coli. Biochim Biophys Acta. 1960 Mar 11;38:460–469. doi: 10.1016/0006-3002(60)91281-6. [DOI] [PubMed] [Google Scholar]