Abstract
1. Na currents and fluctuations of Na currents were studied under voltage clamp in the same myelinated nerve fibres of Rana esculenta at 13 °C. The results were used to test several kinetic models for the gating process of Na channels.
2. Long voltage pulses, depolarizing the membrane by 16-48 mV from a hyperpolarizing holding level of — 28 mV, were applied in 4 sec intervals. The d.c. and a.c. components of the membrane current were recorded during the last 328 msec of the 473 msec pulses. For each depolarization, ninety-six trials were made with the node in Ringer solution and, again, after adding 300 nm-tetrodotoxin (TTX) in that solution.
3. The TTX-sensitive d.c. component declined during the 328 msec records by 14-51% of its time average. The a.c. component was corrected for this trend by subtracting the first from the second of each pair of subsequent records. The TTX-sensitive part of its variance declined, on the average, in parallel to the current, as if the open probability rather than the conductance of the individual Na channels was reduced by a slow process.
4. Single-channel conductances, γ, were calculated on the assumption that Na channels have only one non-zero conductance and were corrected for the limited band width (5 kHz) of the a.c. records. Values of γ increased slightly (< 30% from 16 to 40 mV), and averaged 8·85 ± 0·7 pS (s.e. of mean, seventeen measurements on ten fibres). This small degree of change in γ suggests that deviations from the all-or-none gating are very small.
5. Power spectral densities of the fluctuations between 3 Hz and 5 kHz were calculated from the trend-free a.c. records and corrected for the TTX-insensitive noise component. Control calculations showed that the only effect of the nonstationarity in the Na current was to enhance the low-frequency points of such spectra by less than 10%. The spectra revealed at least two Lorentzian components with cut-off frequencies in the range expected from the activation and inactivation kinetics. The low-frequency component became dominant as depolarization was increased.
6. Na currents recorded during brief (< 40 msec) depolarizations were analysed in terms of various all-or-none gating models, in which inactivation either was independent of activation (Hodgkin-Huxley (HH) model) or could occur only from the partly or fully activated states (coupled models). The transient Na currents were reproduced by all models.
7. With the parameters from such fits, the fluctuation spectra expected for each model were calculated. The predictions differed in the fraction, rh, of the variance contributed by the slow (inactivation) fluctuations; rh was larger in the coupled models than in the HH model.
8. The experimental spectra were divided into two spectral components to yield empirical values for rh. We used as templates the spectral curves derived for the fast and for the slow fluctuations of the HH model. The empirical rh values were one (48 mV) to four (16 mV) times larger than those expected for the HH model. They were also larger than the theoretical rh of the coupled models at the small depolarizations, but became equal or smaller than those at the largest depolarization. Direct comparison of the measured and theoretical spectra revealed the same discrepancies.
9. We conclude that all of the simple gating models considered in this paper are inconsistent with the fluctuation measurements, the coupled models giving slightly smaller deviations than the model with independent activation and inactivation.
Full text
PDF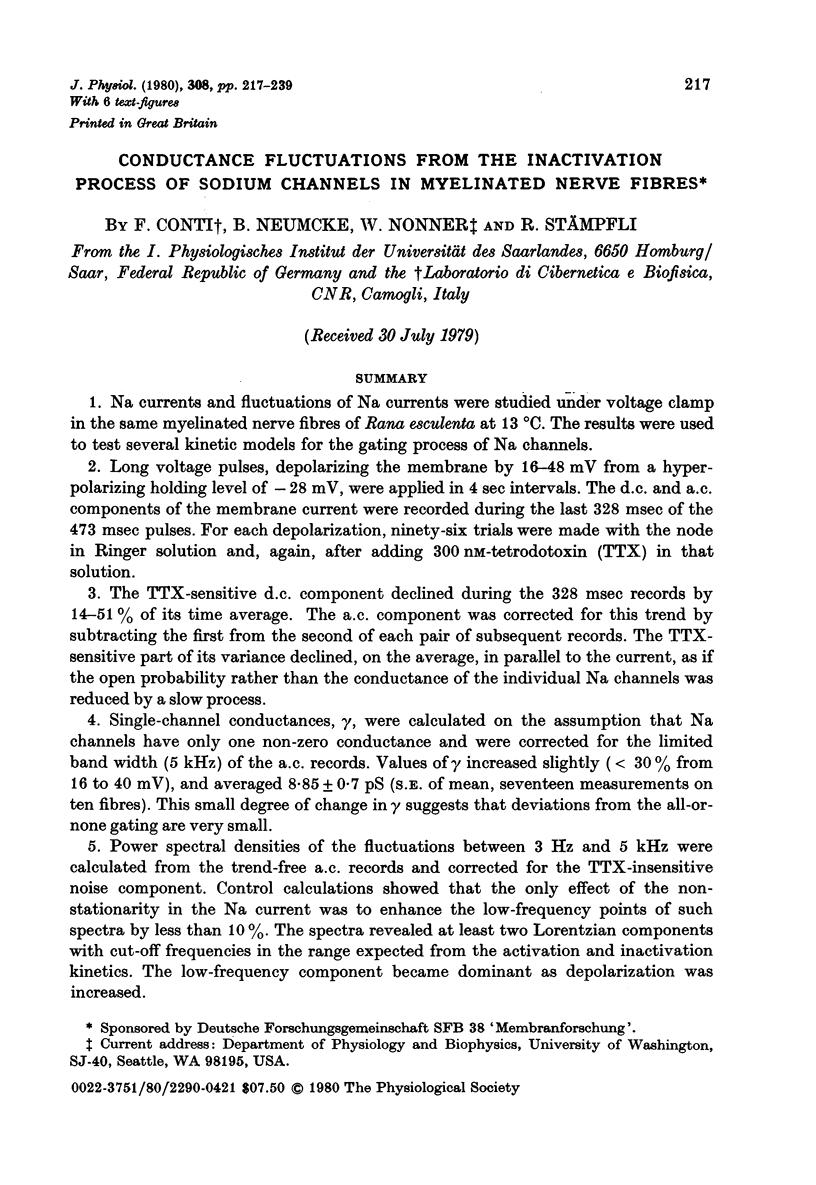
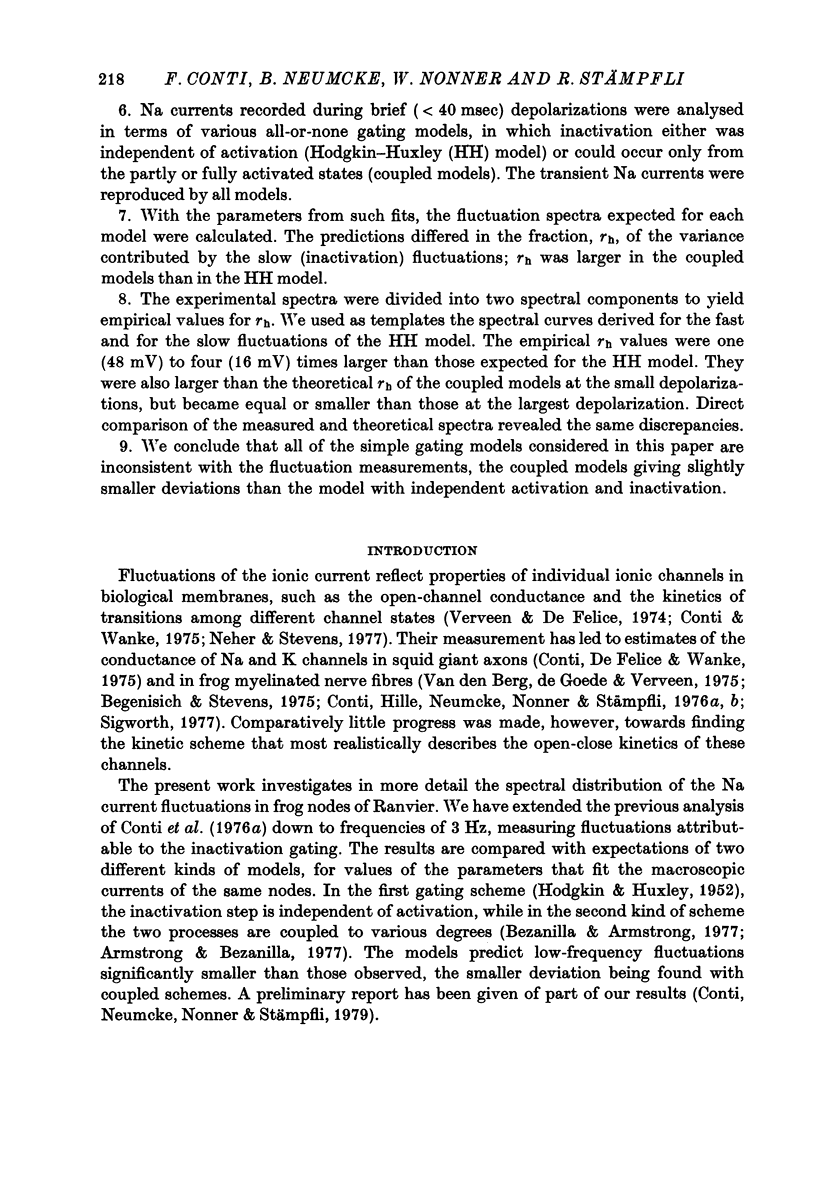
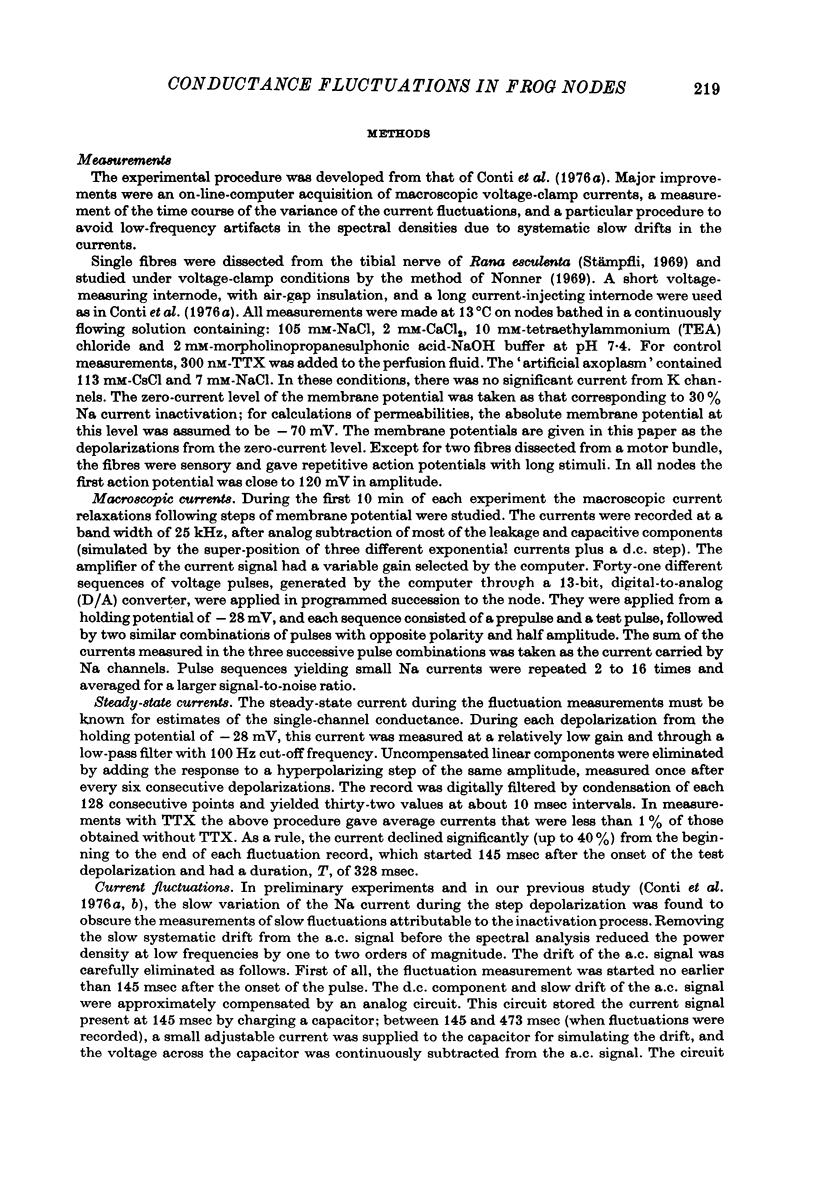
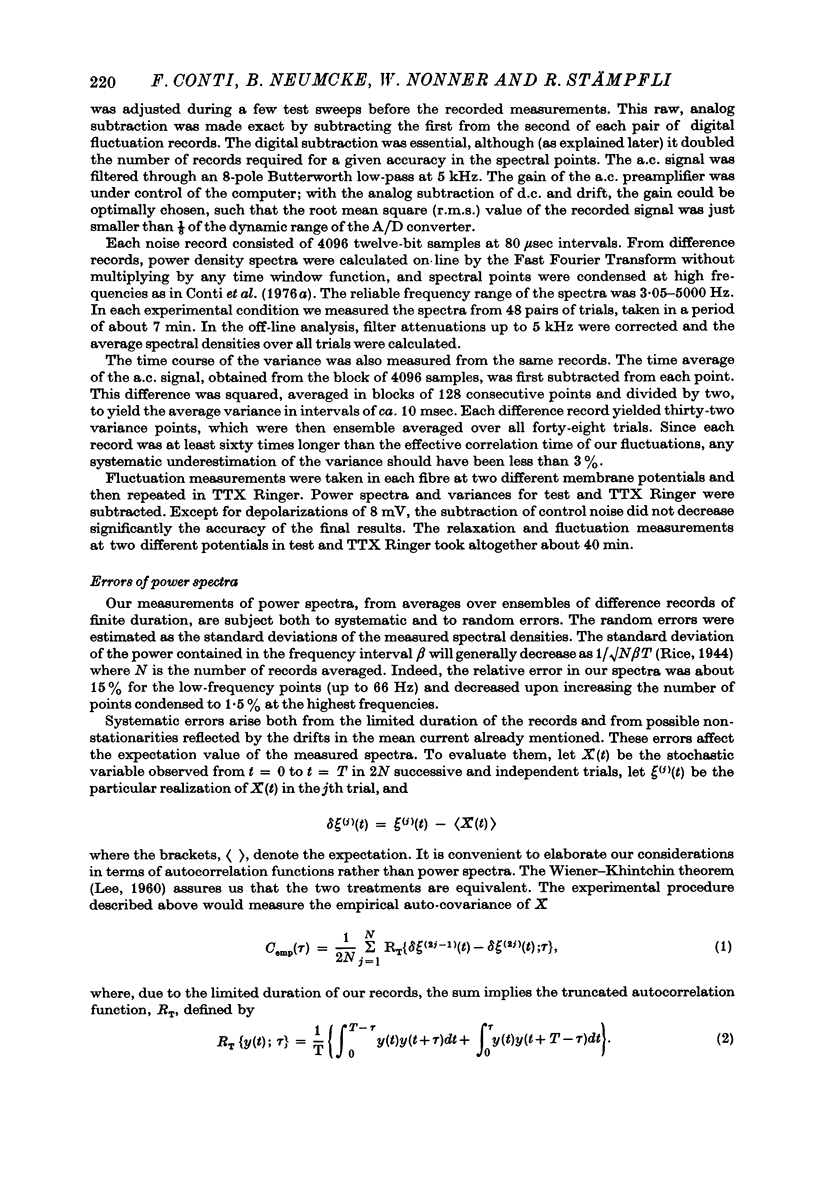
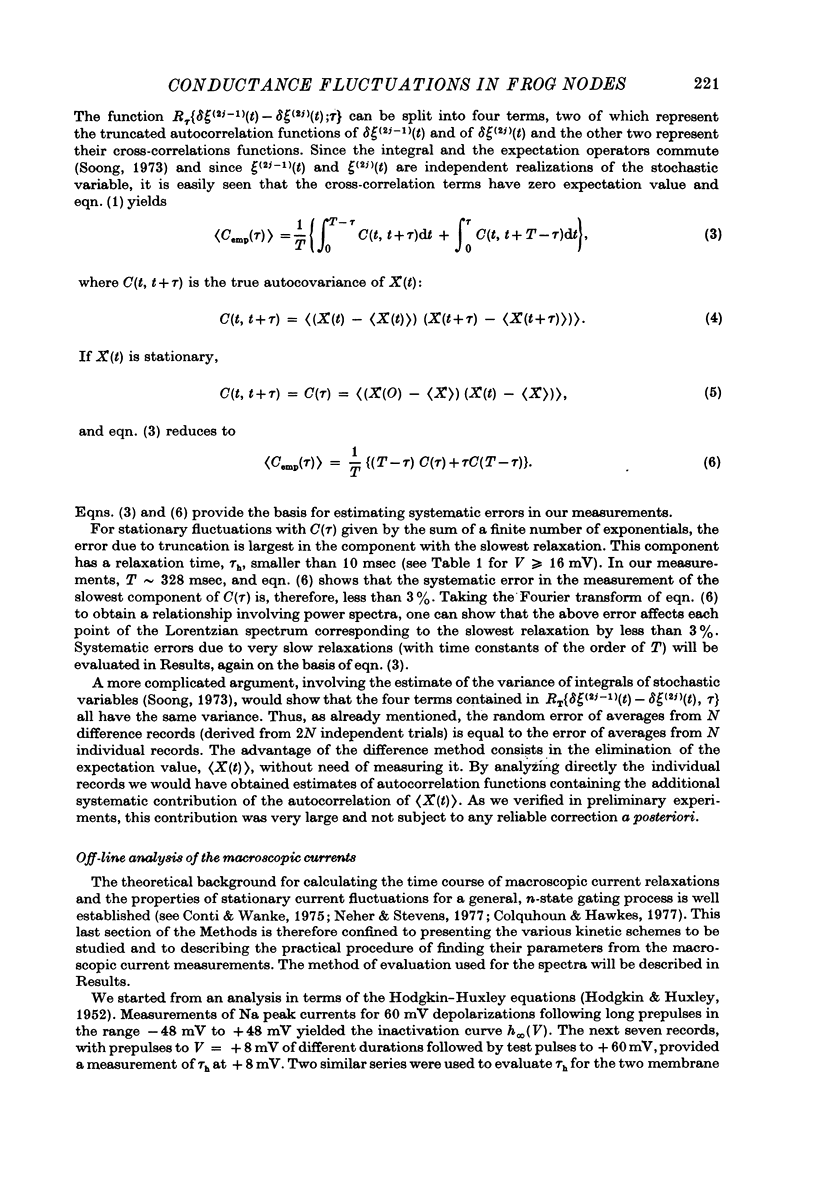
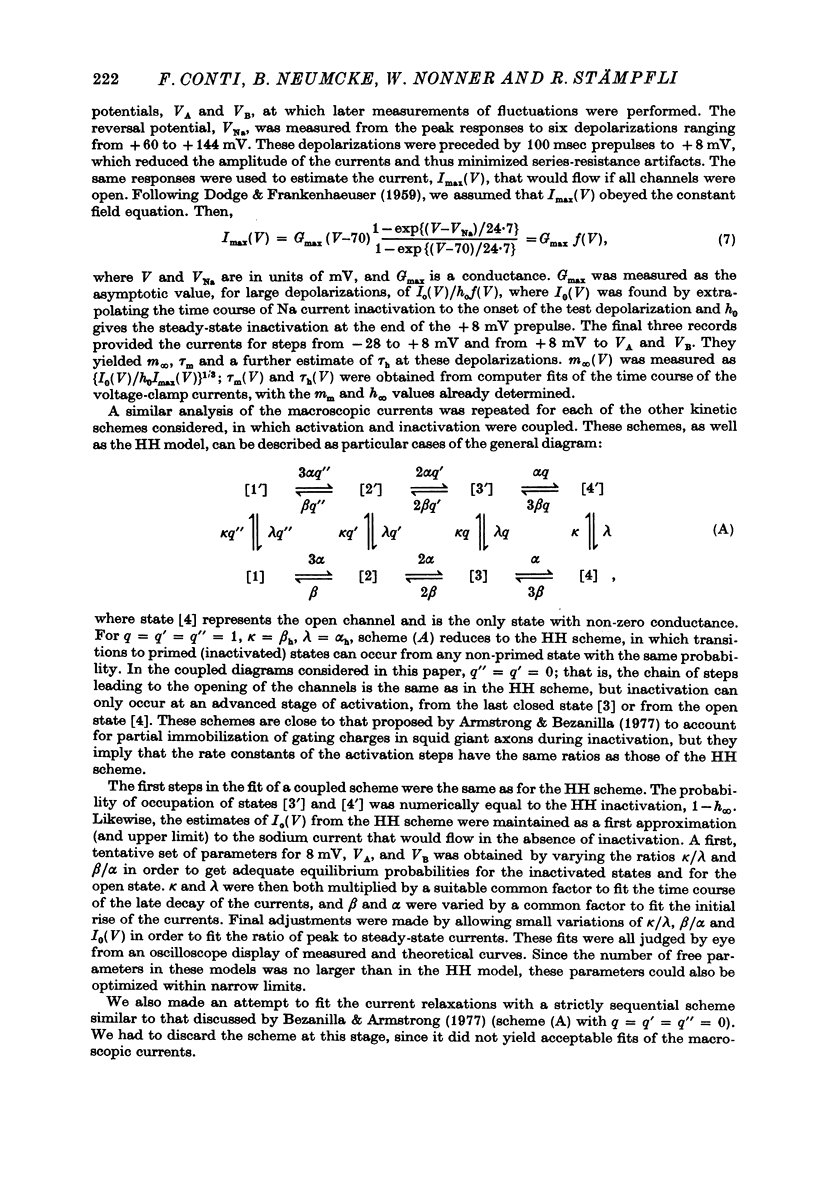
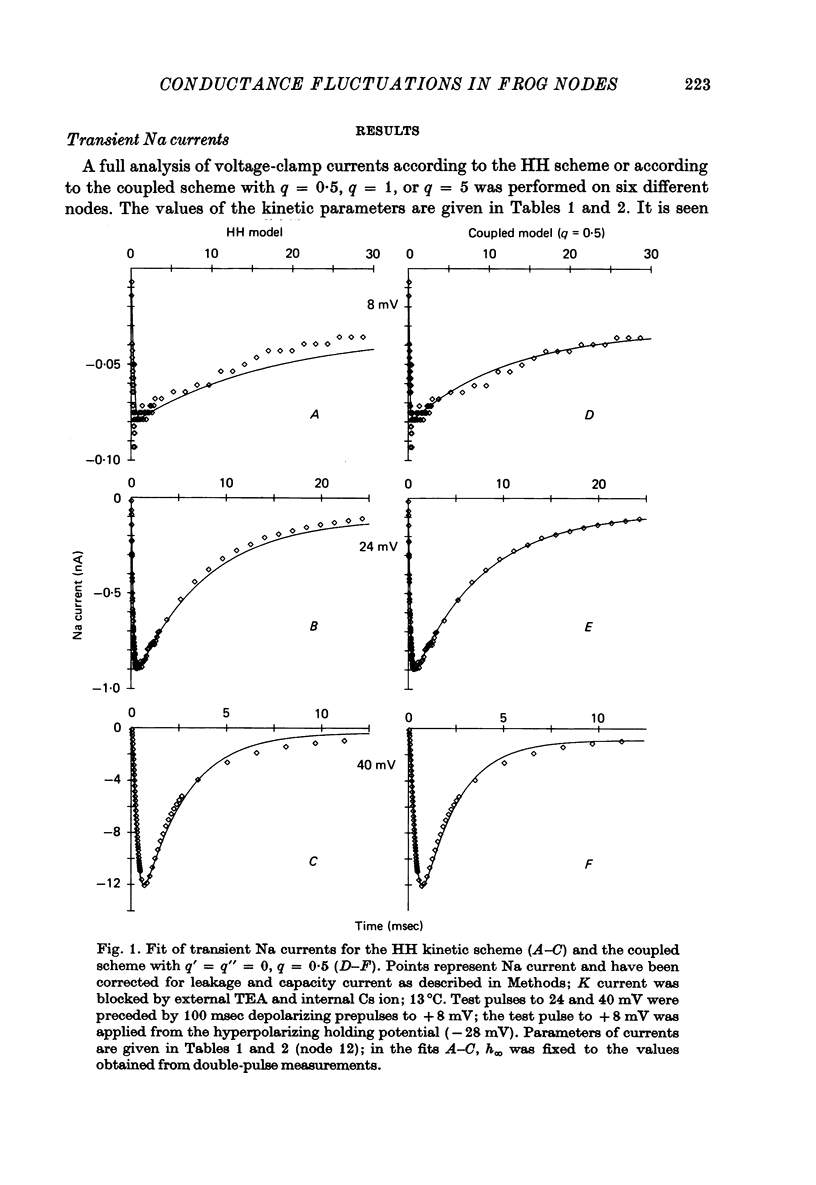
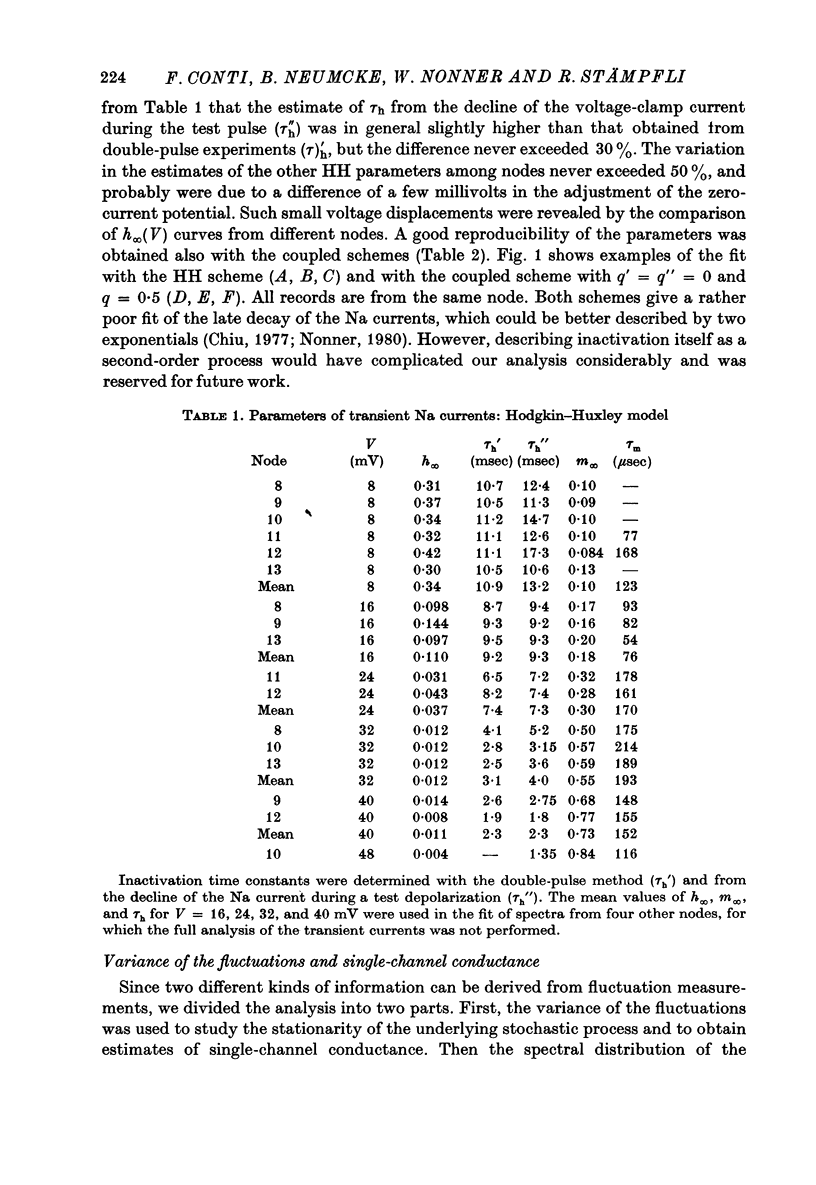
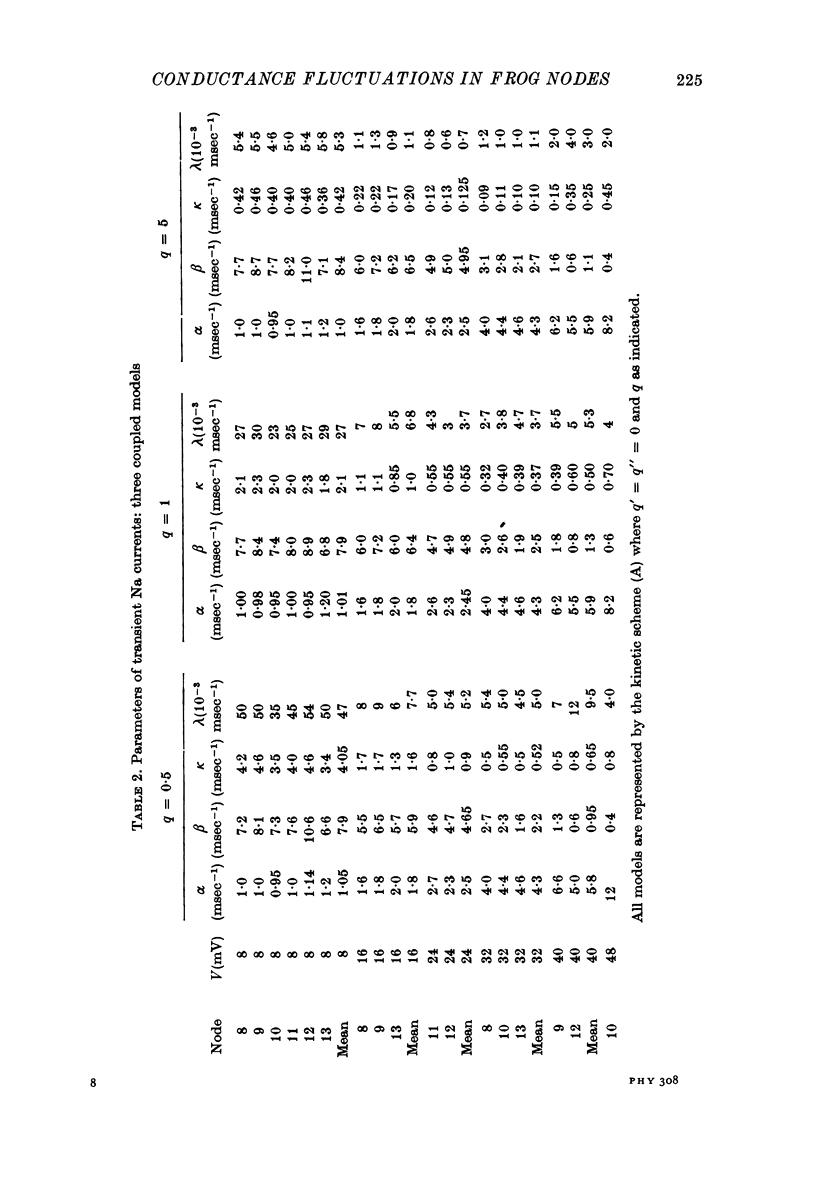
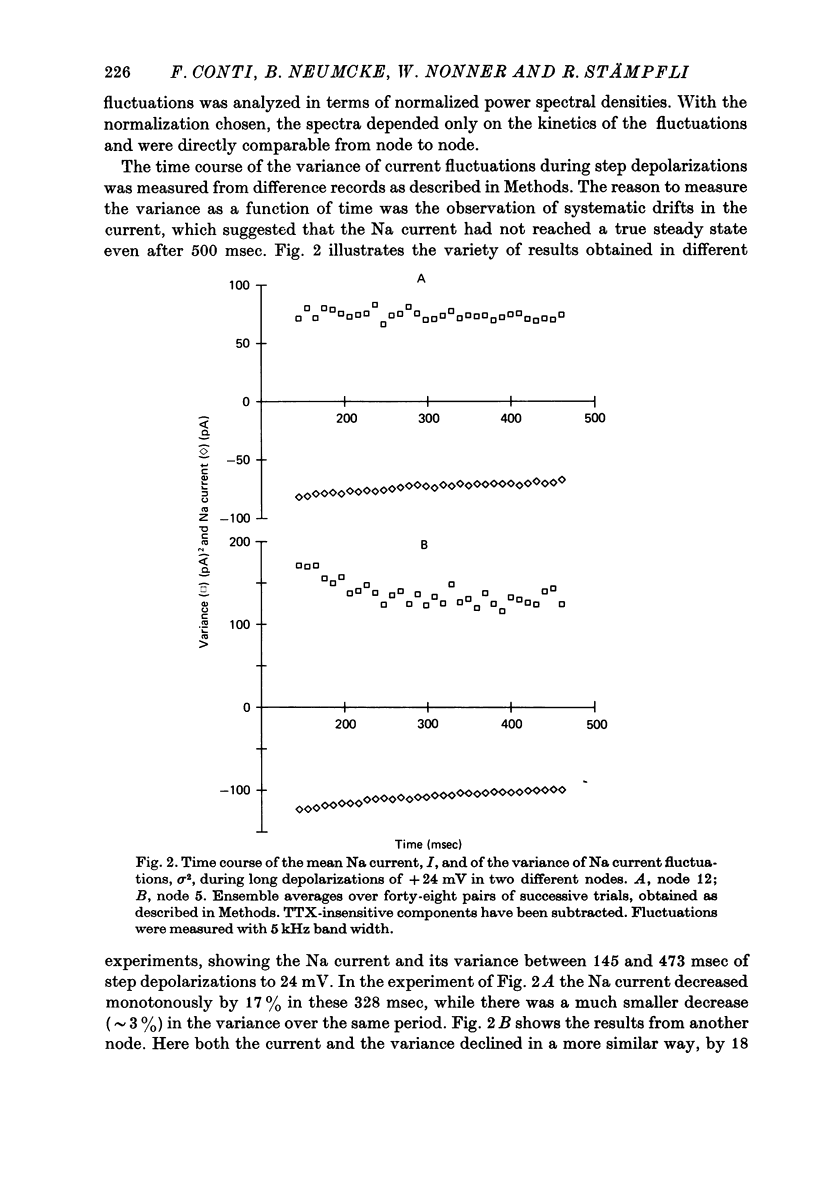
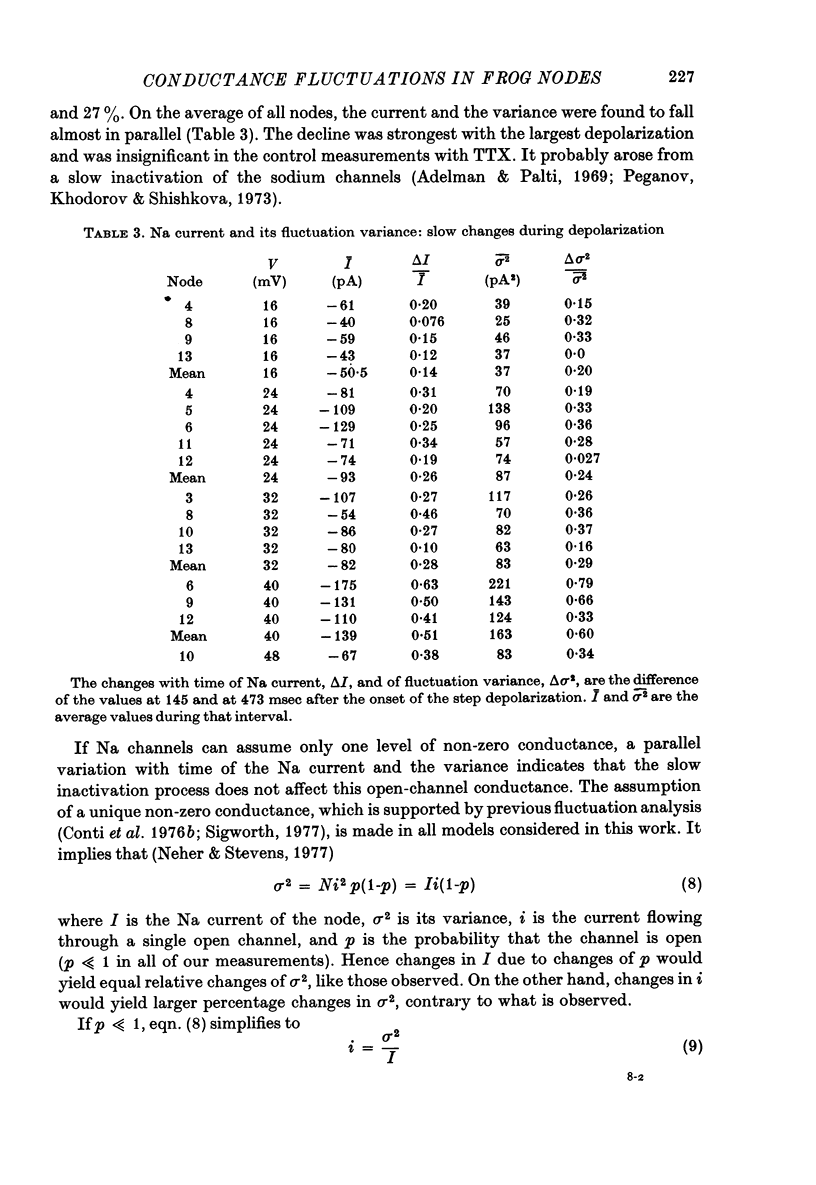
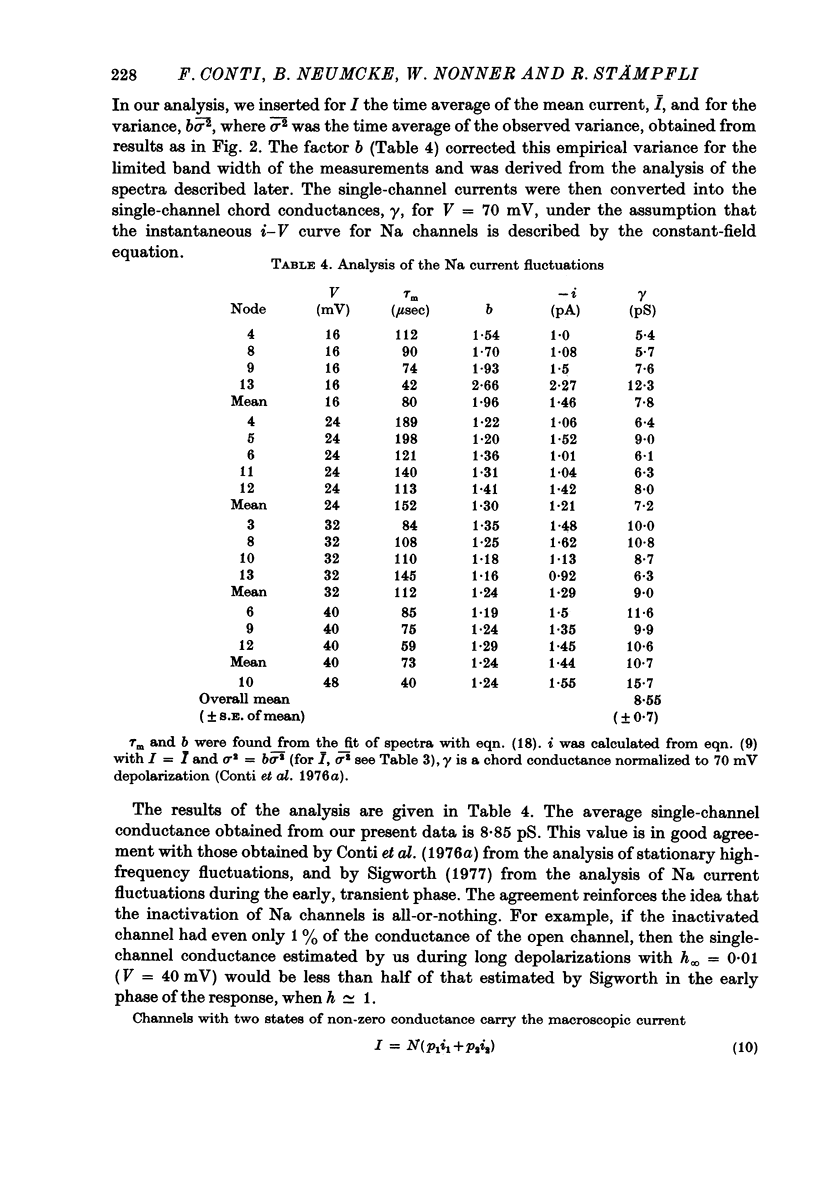
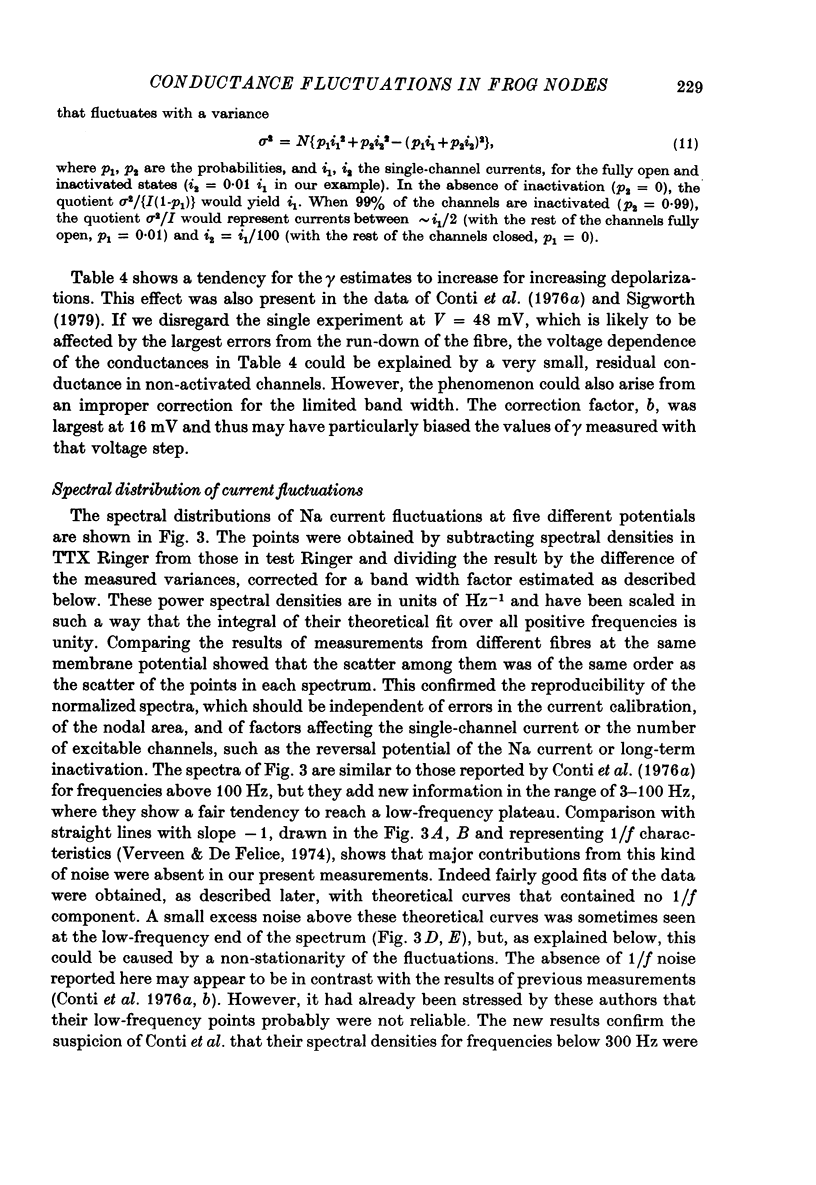
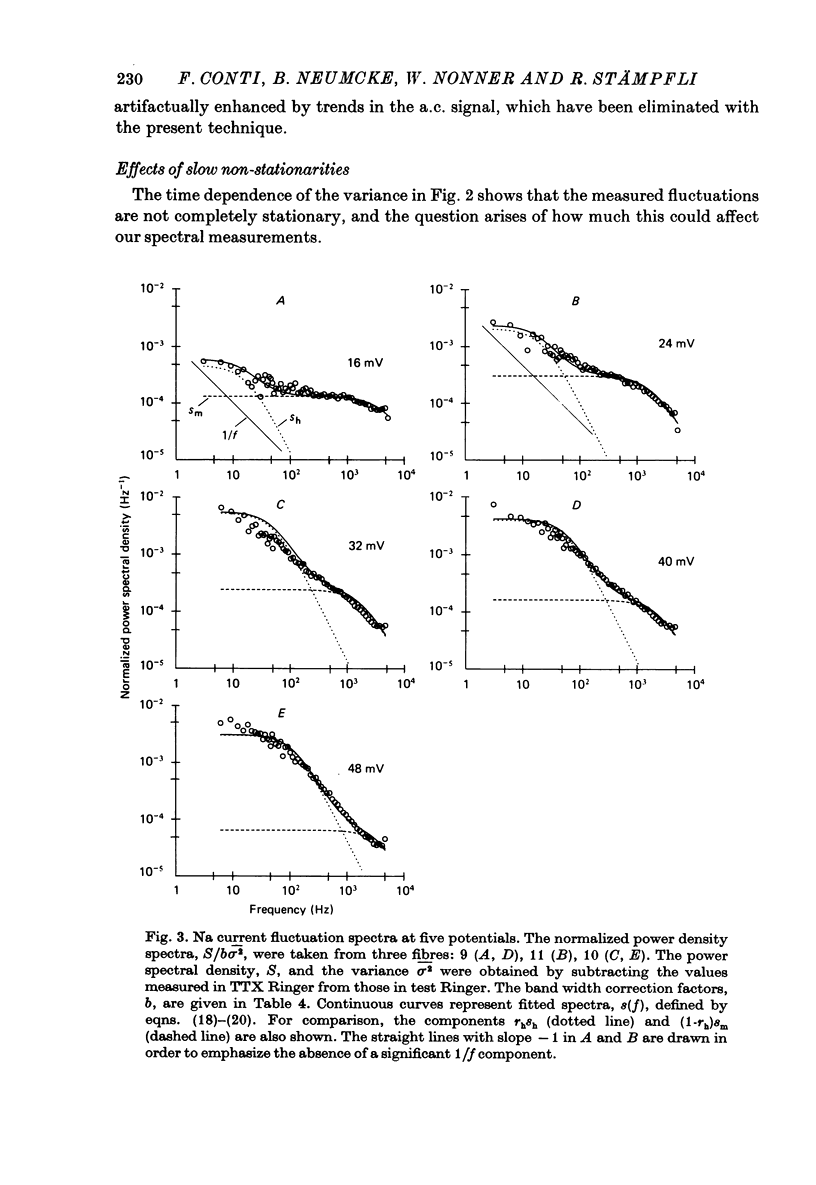
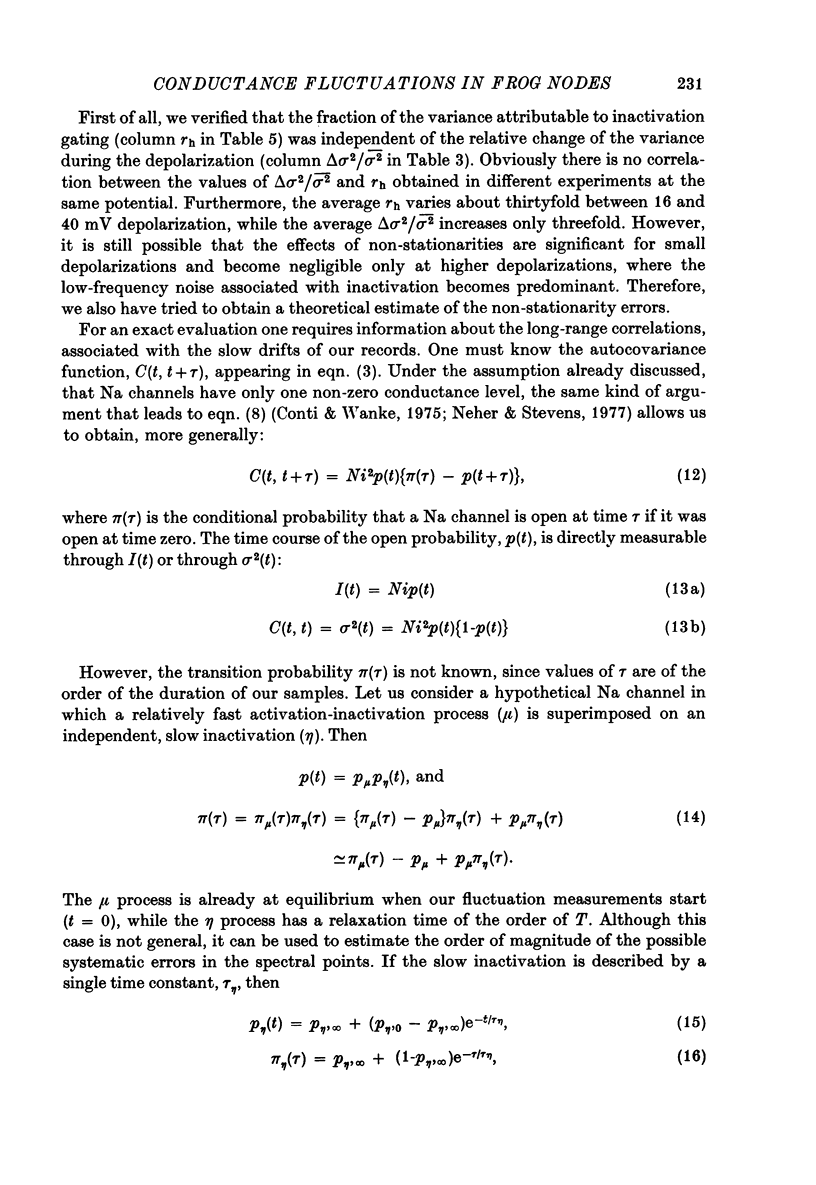
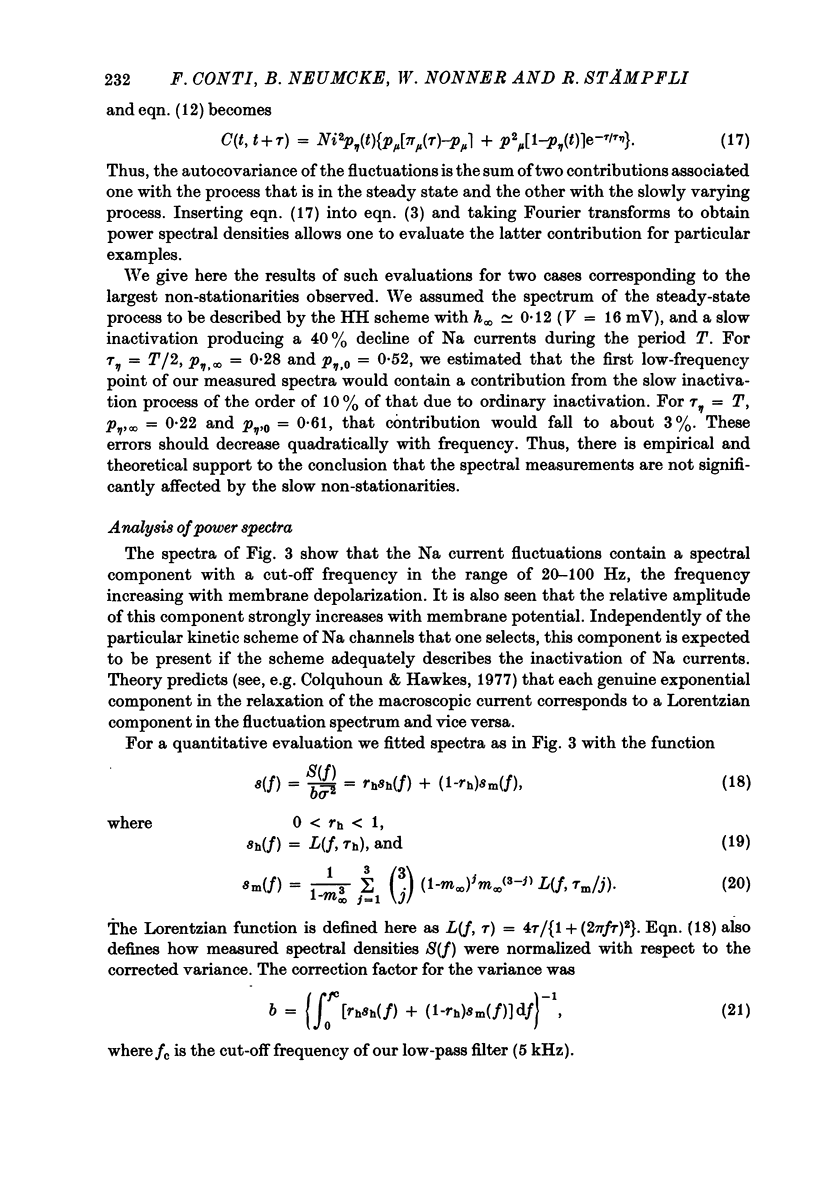
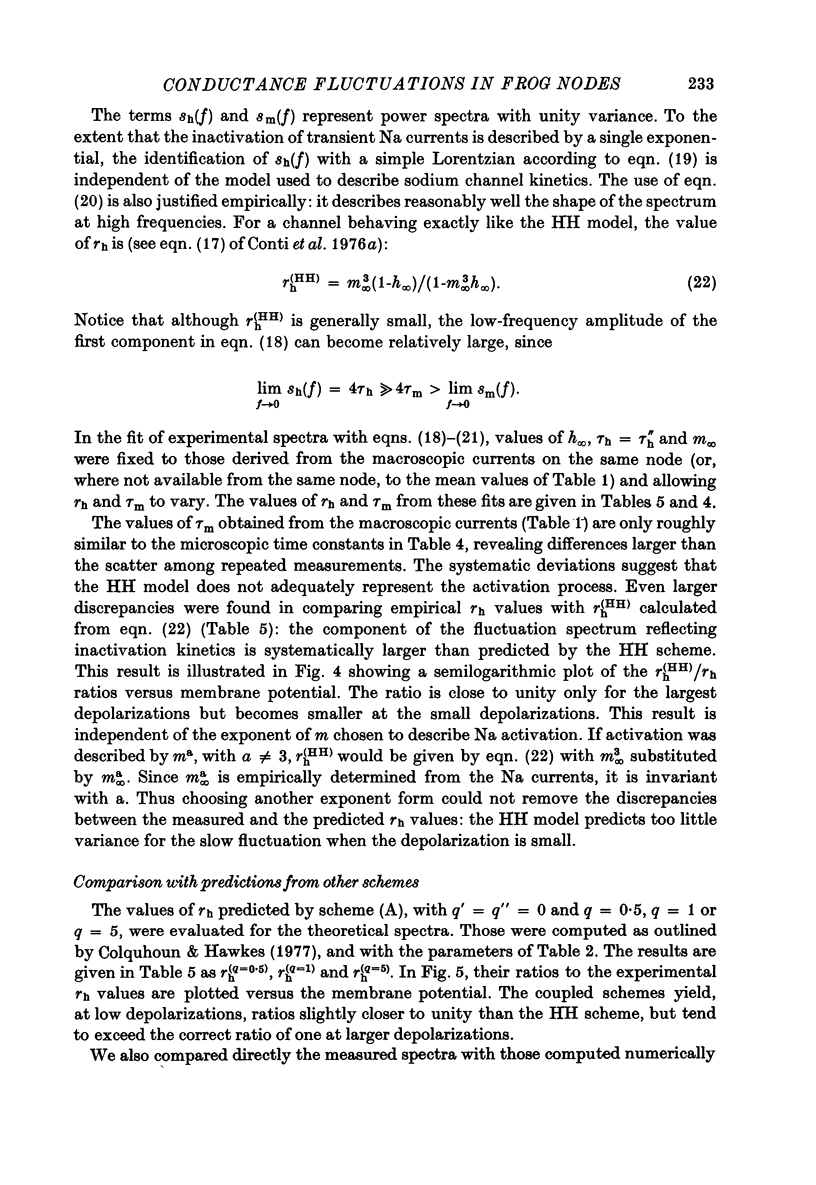
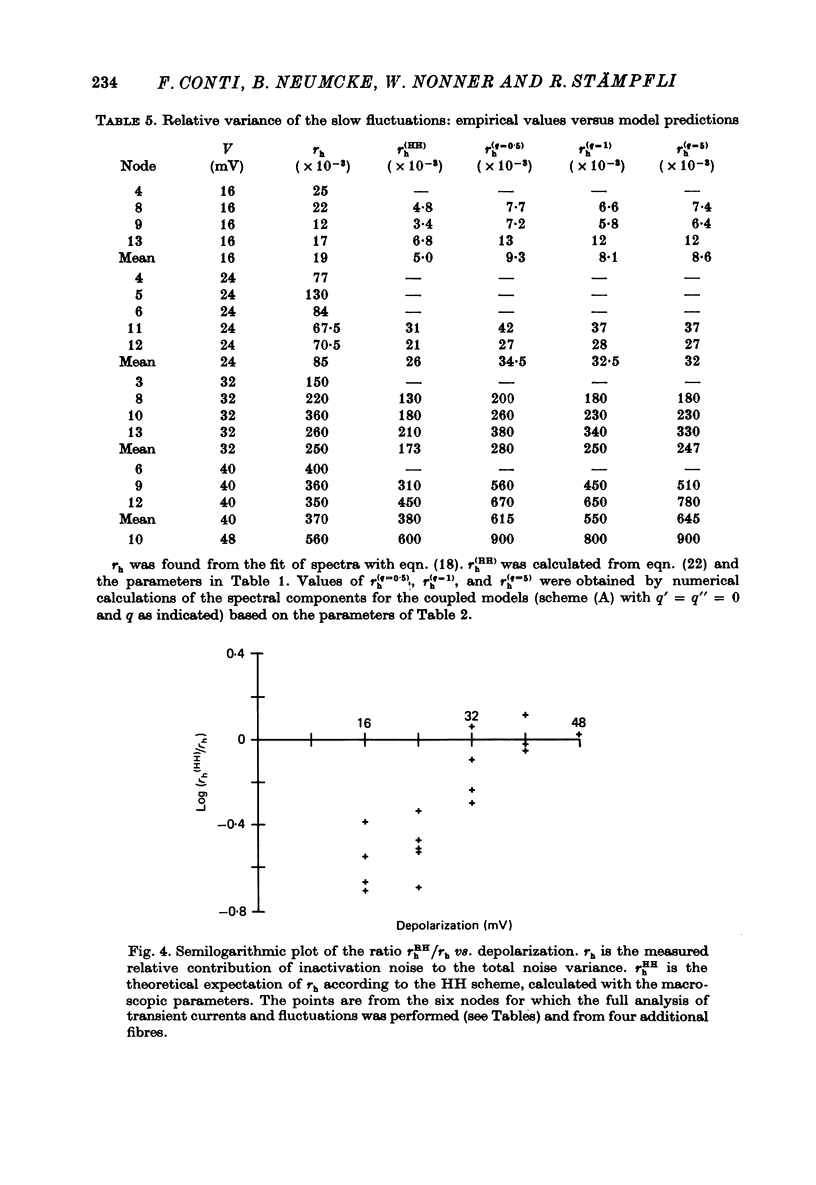
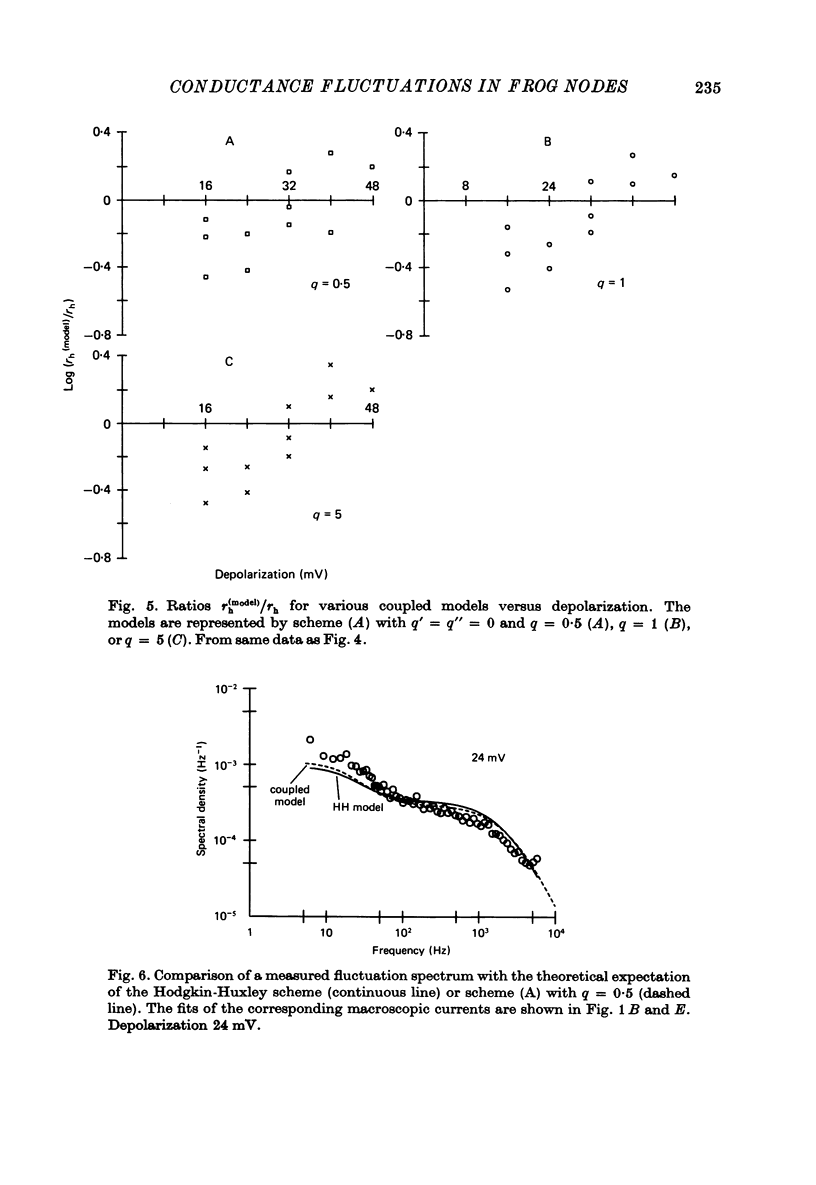
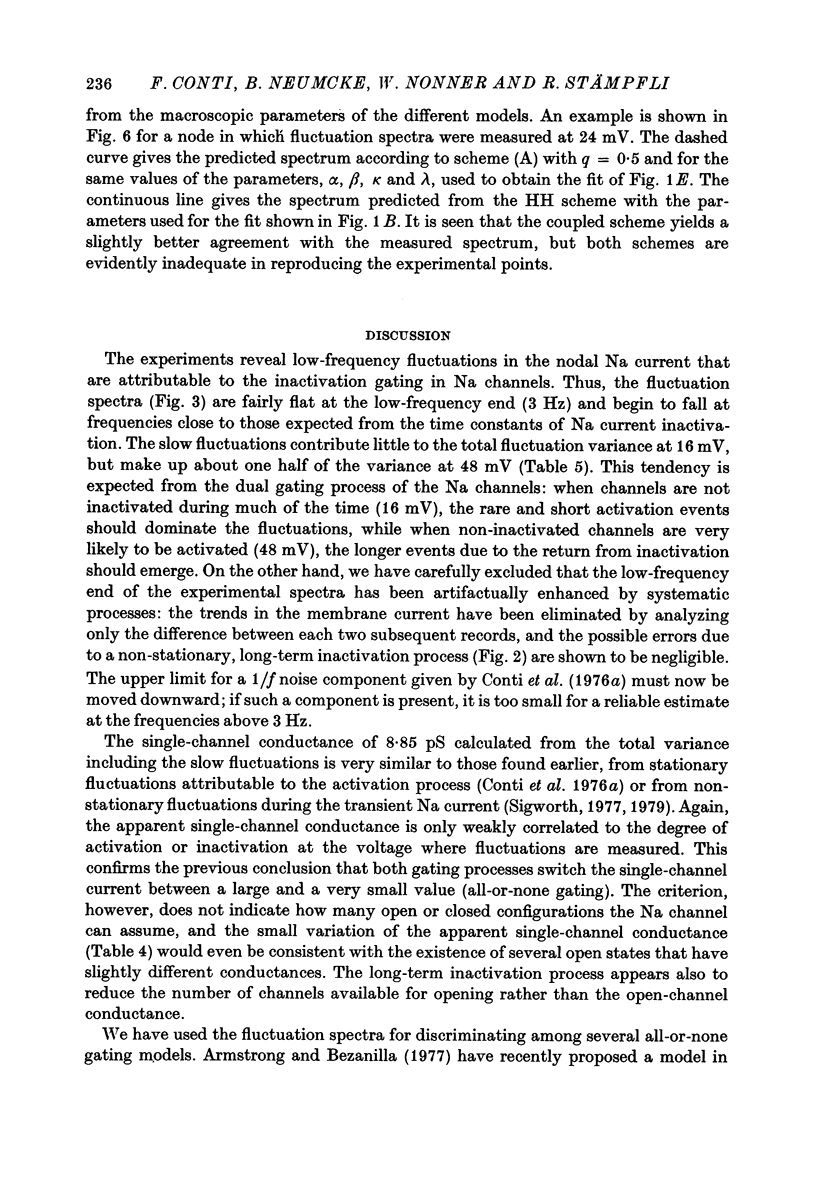
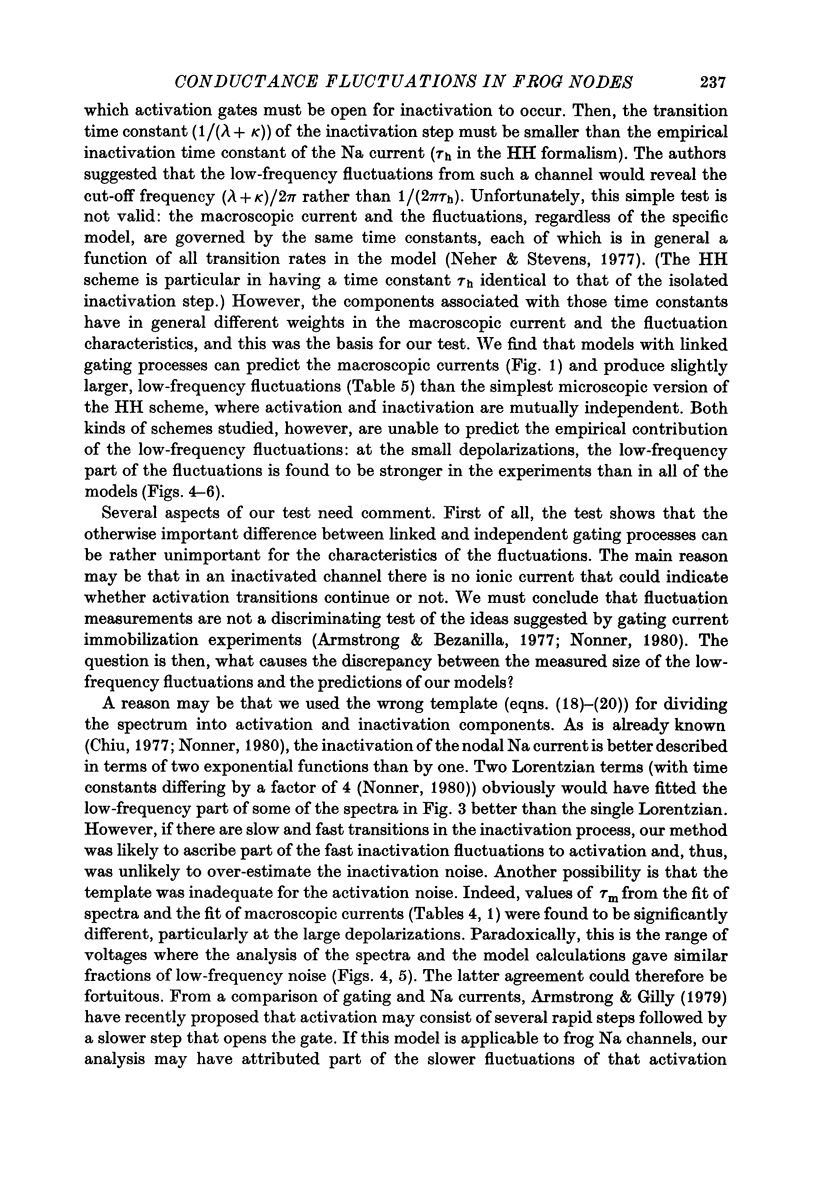
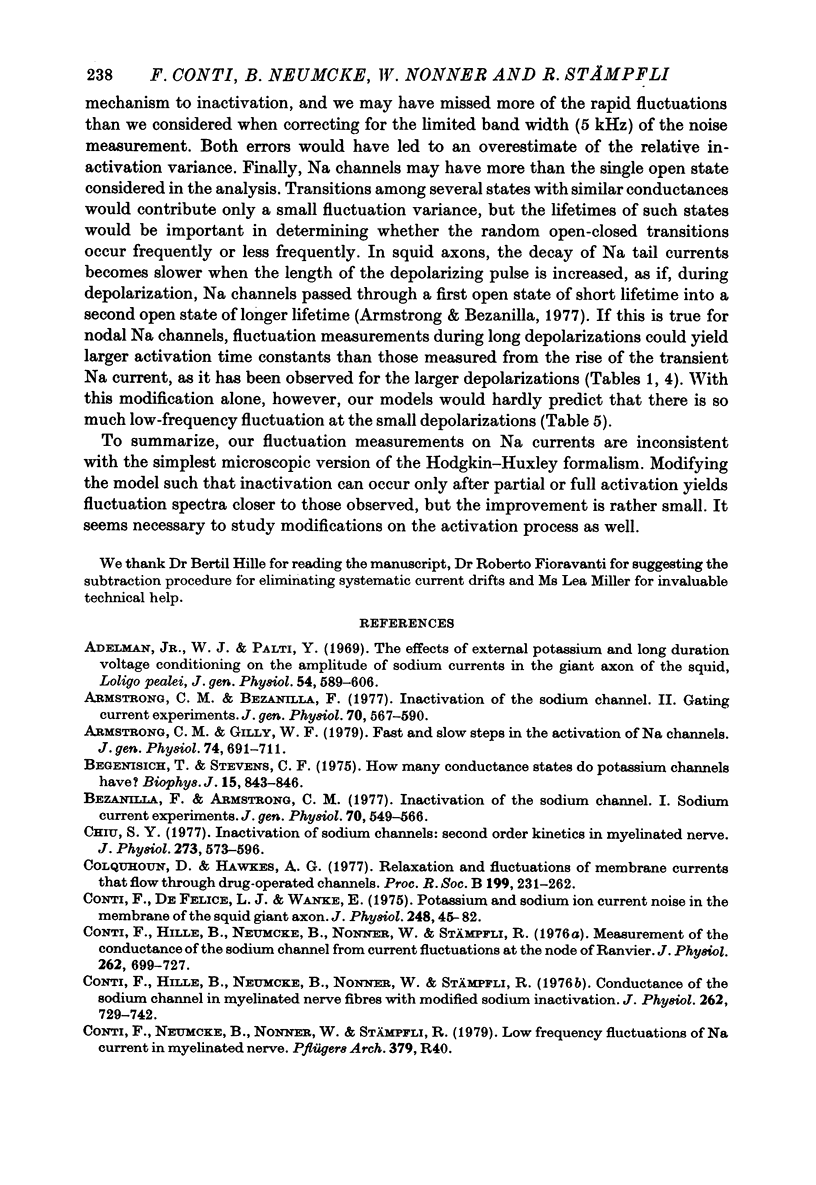
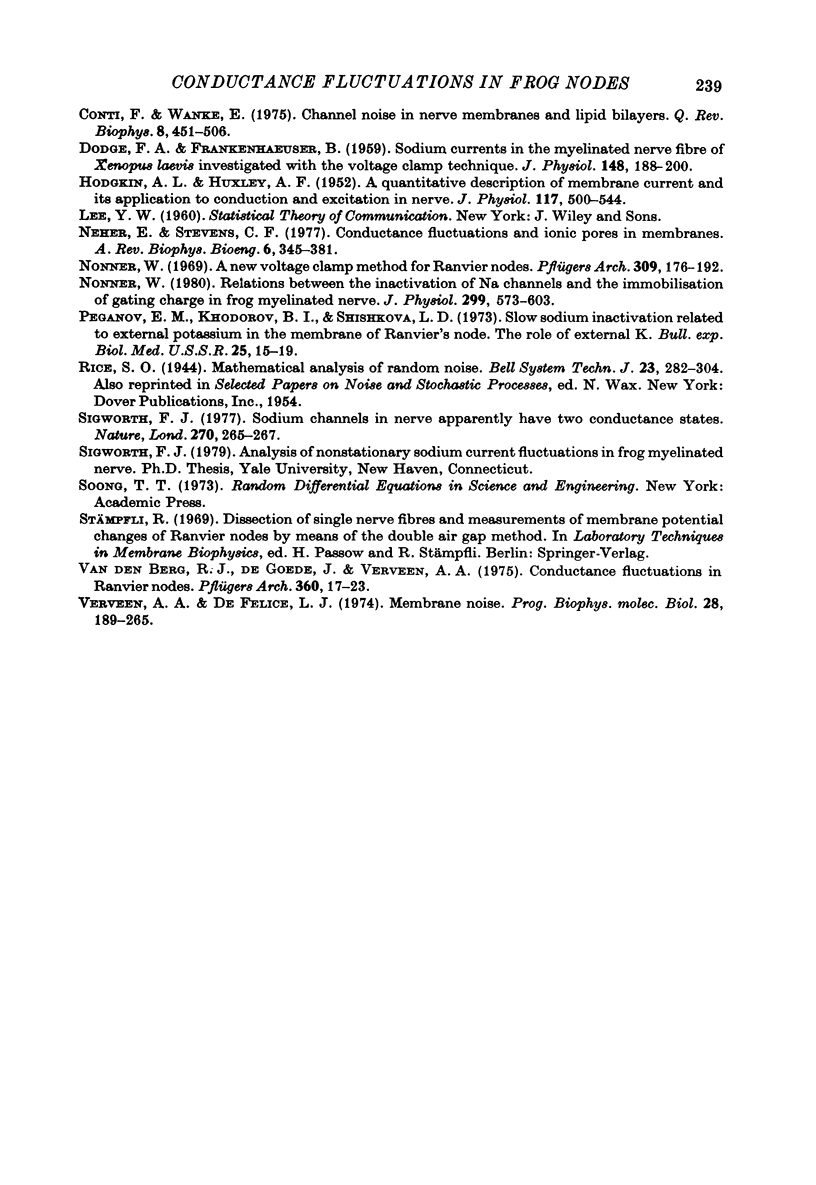
Selected References
These references are in PubMed. This may not be the complete list of references from this article.
- Adelman W. J., Jr, Palti Y. The effects of external potassium and long duration voltage conditioning on the amplitude of sodium currents in the giant axon of the squid, Loligo pealei. J Gen Physiol. 1969 Nov;54(5):589–606. doi: 10.1085/jgp.54.5.589. [DOI] [PMC free article] [PubMed] [Google Scholar]
- Armstrong C. M., Bezanilla F. Inactivation of the sodium channel. II. Gating current experiments. J Gen Physiol. 1977 Nov;70(5):567–590. doi: 10.1085/jgp.70.5.567. [DOI] [PMC free article] [PubMed] [Google Scholar]
- Armstrong C. M., Gilly W. F. Fast and slow steps in the activation of sodium channels. J Gen Physiol. 1979 Dec;74(6):691–711. doi: 10.1085/jgp.74.6.691. [DOI] [PMC free article] [PubMed] [Google Scholar]
- Begenisich T., Stevens C. F. How many conductance states do potassium channels have? Biophys J. 1975 Aug;15(8):843–846. doi: 10.1016/S0006-3495(75)85858-9. [DOI] [PMC free article] [PubMed] [Google Scholar]
- Bezanilla F., Armstrong C. M. Inactivation of the sodium channel. I. Sodium current experiments. J Gen Physiol. 1977 Nov;70(5):549–566. doi: 10.1085/jgp.70.5.549. [DOI] [PMC free article] [PubMed] [Google Scholar]
- Colquhoun D., Hawkes A. G. Relaxation and fluctuations of membrane currents that flow through drug-operated channels. Proc R Soc Lond B Biol Sci. 1977 Nov 14;199(1135):231–262. doi: 10.1098/rspb.1977.0137. [DOI] [PubMed] [Google Scholar]
- Conti F., De Felice L. J., Wanke E. Potassium and sodium ion current noise in the membrane of the squid giant axon. J Physiol. 1975 Jun;248(1):45–82. doi: 10.1113/jphysiol.1975.sp010962. [DOI] [PMC free article] [PubMed] [Google Scholar]
- Conti F., Hille B., Neumcke B., Nonner W., Stämpfli R. Conductance of the sodium channel in myelinated nerve fibres with modified sodium inactivation. J Physiol. 1976 Nov;262(3):729–742. doi: 10.1113/jphysiol.1976.sp011617. [DOI] [PMC free article] [PubMed] [Google Scholar]
- Conti F., Hille B., Neumcke B., Nonner W., Stämpfli R. Measurement of the conductance of the sodium channel from current fluctuations at the node of Ranvier. J Physiol. 1976 Nov;262(3):699–727. doi: 10.1113/jphysiol.1976.sp011616. [DOI] [PMC free article] [PubMed] [Google Scholar]
- DODGE F. A., FRANKENHAEUSER B. Sodium currents in the myelinated nerve fibre of Xenopus laevis investigated with the voltage clamp technique. J Physiol. 1959 Oct;148:188–200. doi: 10.1113/jphysiol.1959.sp006281. [DOI] [PMC free article] [PubMed] [Google Scholar]
- HODGKIN A. L., HUXLEY A. F. A quantitative description of membrane current and its application to conduction and excitation in nerve. J Physiol. 1952 Aug;117(4):500–544. doi: 10.1113/jphysiol.1952.sp004764. [DOI] [PMC free article] [PubMed] [Google Scholar]
- Neher E., Stevens C. F. Conductance fluctuations and ionic pores in membranes. Annu Rev Biophys Bioeng. 1977;6:345–381. doi: 10.1146/annurev.bb.06.060177.002021. [DOI] [PubMed] [Google Scholar]
- Nonner W. Relations between the inactivation of sodium channels and the immobilization of gating charge in frog myelinated nerve. J Physiol. 1980 Feb;299:573–603. doi: 10.1113/jphysiol.1980.sp013143. [DOI] [PMC free article] [PubMed] [Google Scholar]
- Peganov E. M., Khodorov B. I., Shishkova L. D. Medlennaia natrievaia inaktivatsiia v membrane perekhvata Ranve. Rol' naruzhnogo kaliia. Biull Eksp Biol Med. 1973 Sep;76(9):15–19. [PubMed] [Google Scholar]
- Sigworth F. J. Sodium channels in nerve apparently have two conductance states. Nature. 1977 Nov 17;270(5634):265–267. doi: 10.1038/270265a0. [DOI] [PubMed] [Google Scholar]
- Verveen A. A., DeFelice L. J. Membrane noise. Prog Biophys Mol Biol. 1974;28:189–265. doi: 10.1016/0079-6107(74)90019-4. [DOI] [PubMed] [Google Scholar]
- van den Berg R. J., de Goede J., Verveen A. A. Conductance fluctuations in Ranvier nodes. Pflugers Arch. 1975 Oct 16;360(1):17–23. doi: 10.1007/BF00584323. [DOI] [PubMed] [Google Scholar]