Abstract
1. The spread of electrical signals between rods in the salamander retina was examined by passing current into one rod and recording the voltage responses in nearby rods. Rod network behaviour, measured in this way, was simulated from data on rod membrane properties gathered in voltage-clamp experiments on single isolated rods.
2. The network voltage responses to square current pulses became smaller, more transient, and had a longer time-to-peak, for rods further away from the site of current injection. Depolarizing currents produced smaller responses than hyperpolarizing currents of the same magnitude.
3. Neighbouring rods and cones were coupled less strongly than neighbouring rods.
4. The response of the rod network to current injection was unaffected by 2 mm-aspartate-, which eliminates transmission from receptors to horizontal cells.
5. The input resistance of single isolated rods, measured at the resting potential, varied between 100 and 680 MΩ. The lower values were probably due to damage by the micro-electrodes. Electrical coupling was found to be very strong between the rod inner and outer segments.
6. A strong `instantaneous' outward rectification seen in isolated rods at potentials positive to -35 mV was reduced, but not abolished, by 15 mm-TEA.
7. In normal solution, isolated rods exhibited a voltage- and time-dependent current, IA, whose kinetics were approximated by a single first-order gating variable, and whose activation curve spanned the range between -40 and -80 mV. The time constant for the current varied with voltage and was 60-200 msec between -140 and -40 mV.
8. A reversal potential for IA could not be found between -140 and -40 mV in normal solution, and the fully activated current, ĪA, was approximately voltage-independent, with a magnitude of ∼ 0.1 nA over this potential range.
9. By several criteria, IA behaved as a single inward current activated by hyperpolarization. Pharmacological studies suggest, however, that it is the sum of at least two currents with very similar kinetics.
10. Most isolated rods exhibited a very slow (τ ∼ 3 sec) increase in net outward current on depolarizing beyond -35 mV. The magnitude of this current varied considerably between cells.
11. Assuming that the rod network can be approximated by a square lattice of individual rods, resistively coupled together, the voltage-clamp data on isolated rods were used to predict the response of the network to current injection at one cell. The theoretical and observed network behaviour were in good agreement. The resistance coupling neighbouring rods was estimated to be ∼ 300 MΩ. The current IA plays a major role in determining the behaviour of the rod network.
12. The time-dependent current, IA, is responsible for the peak—plateau wave form of the response to a bright flash. A current similar to IA could also account for the negative propagation velocity of the peak of the dim flash response, through the rod network of the turtle, observed by Detwiler, Hodgkin & McNaughton (1978).
Full text
PDF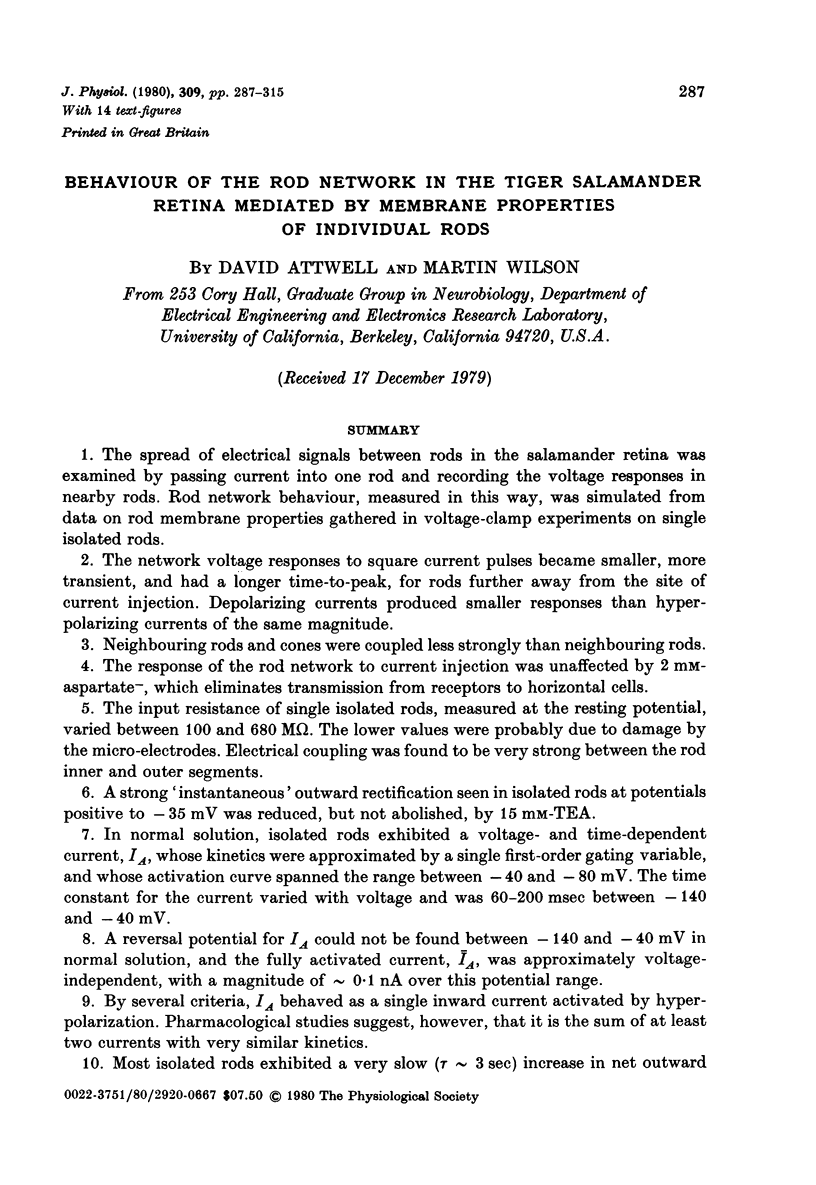
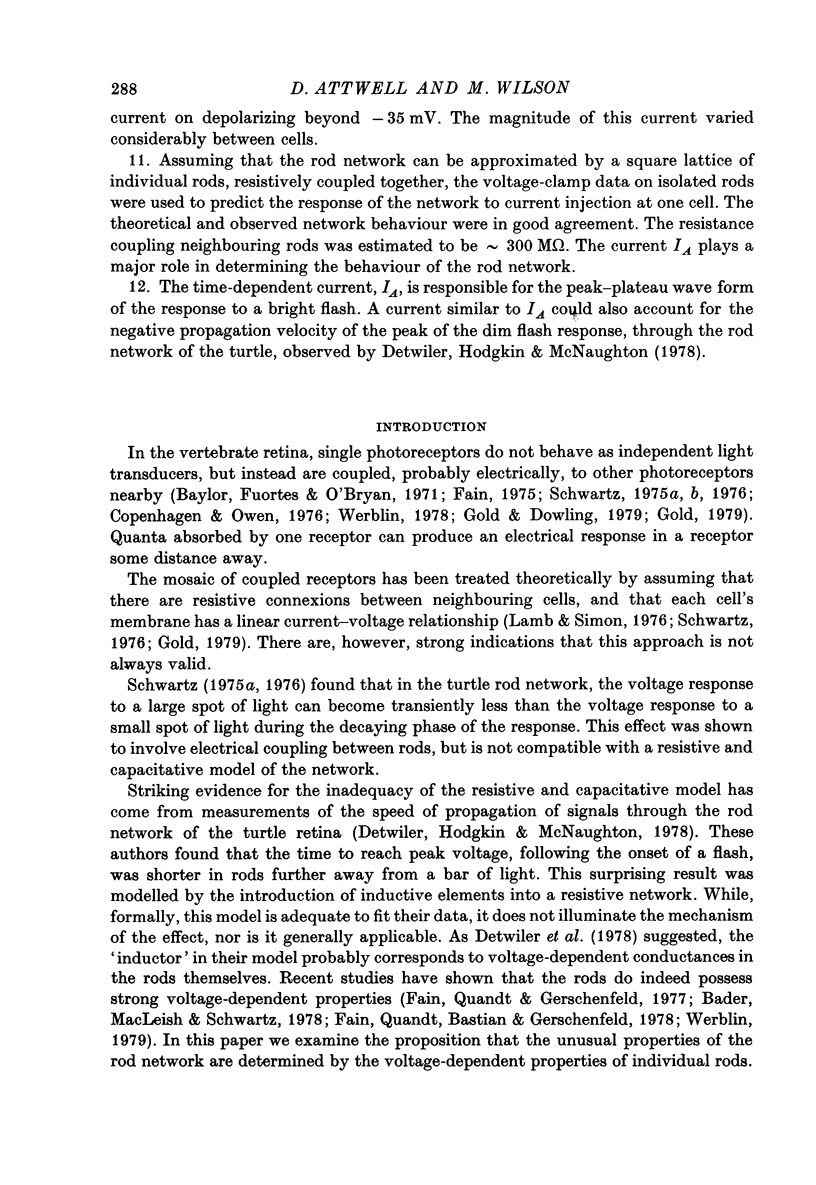
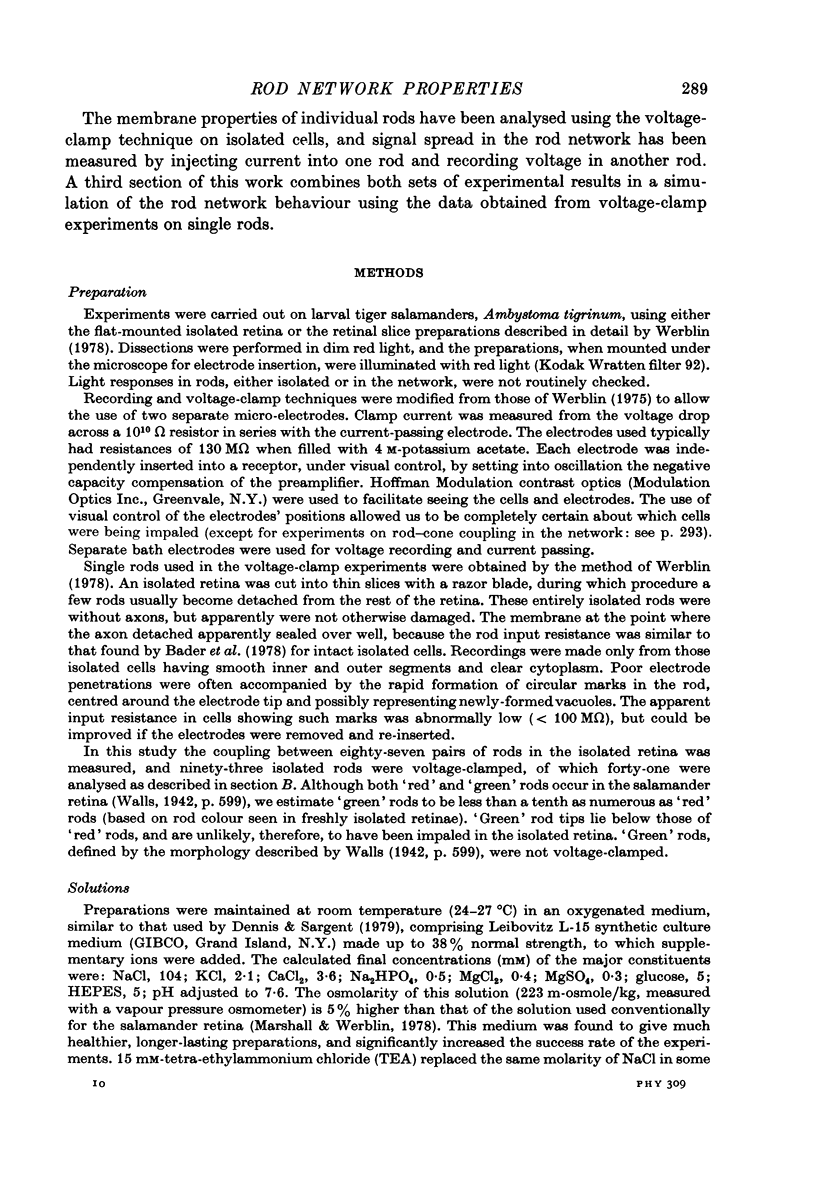
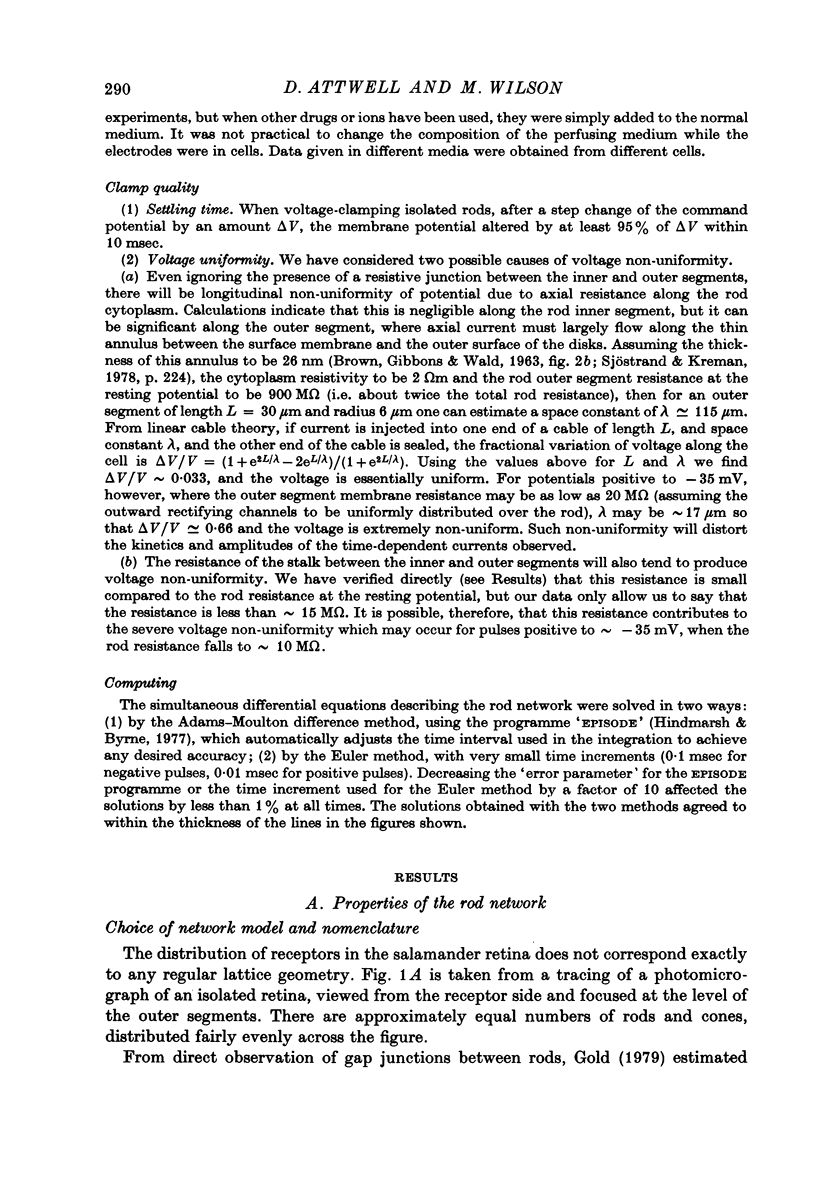
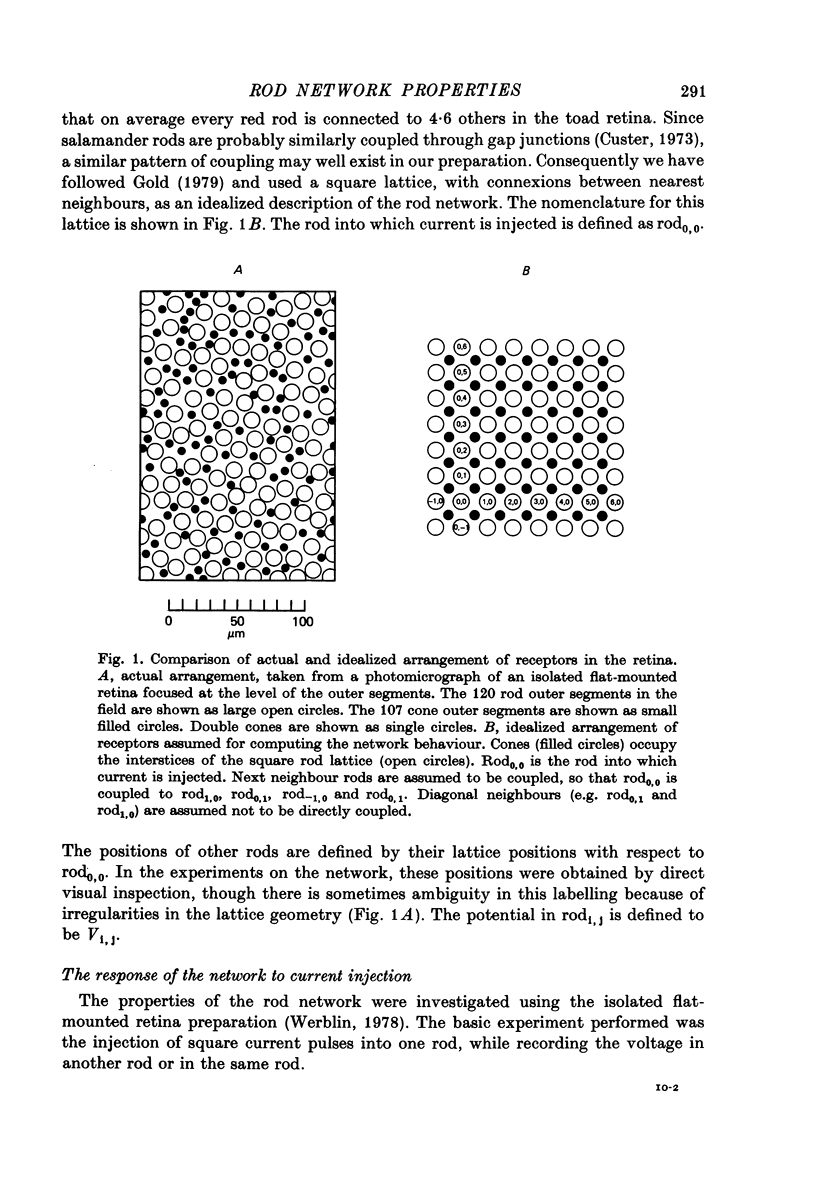
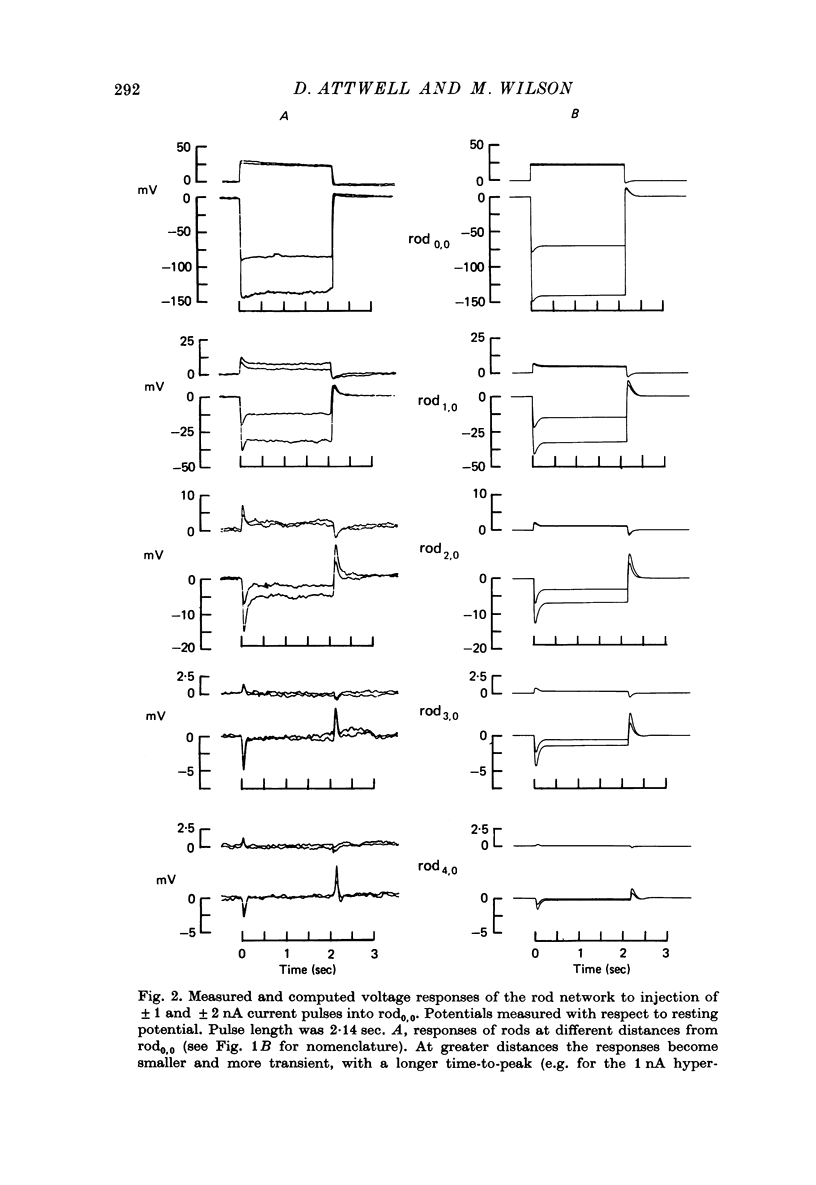
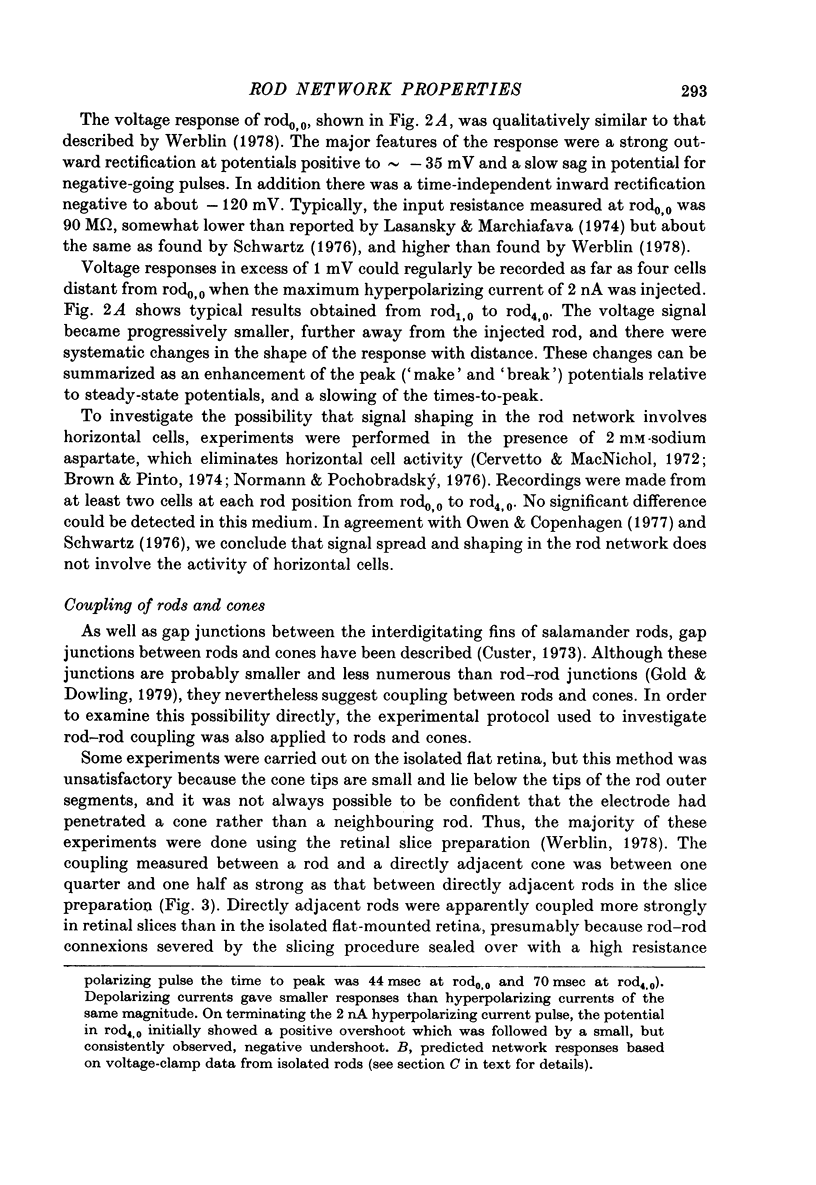
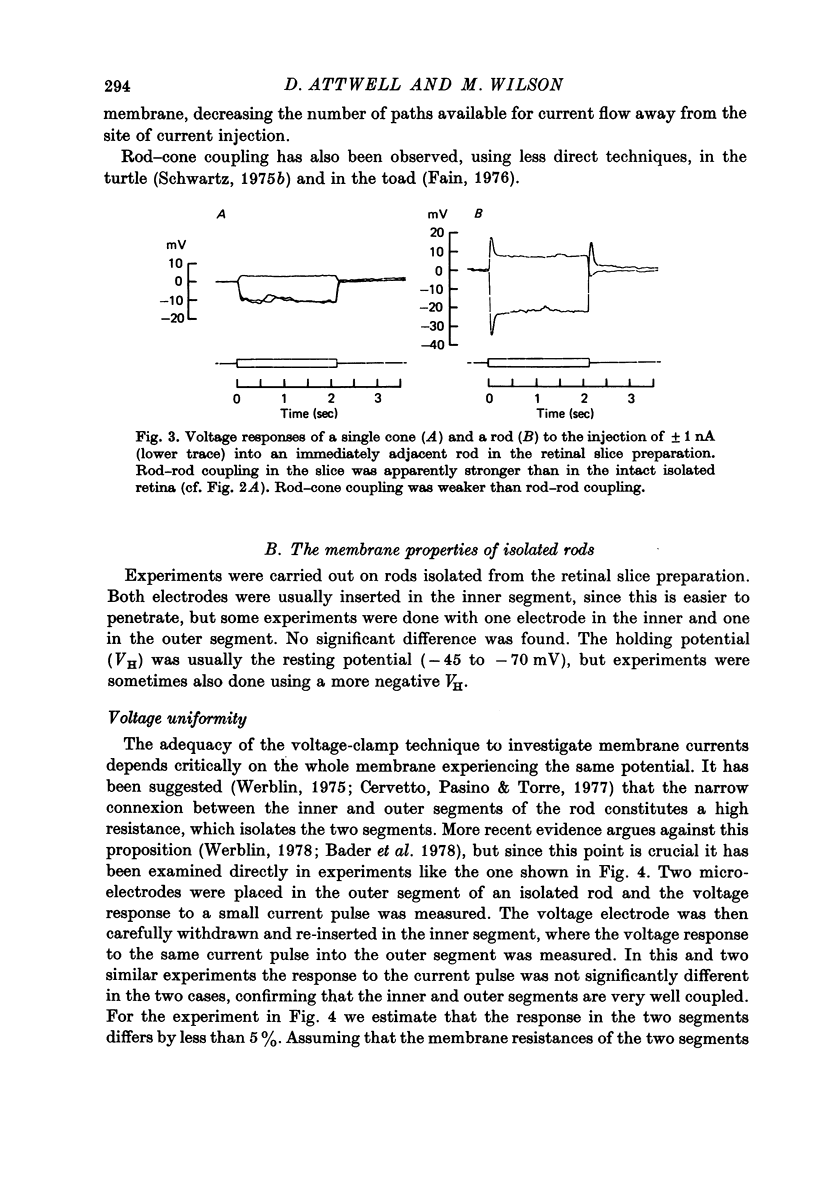
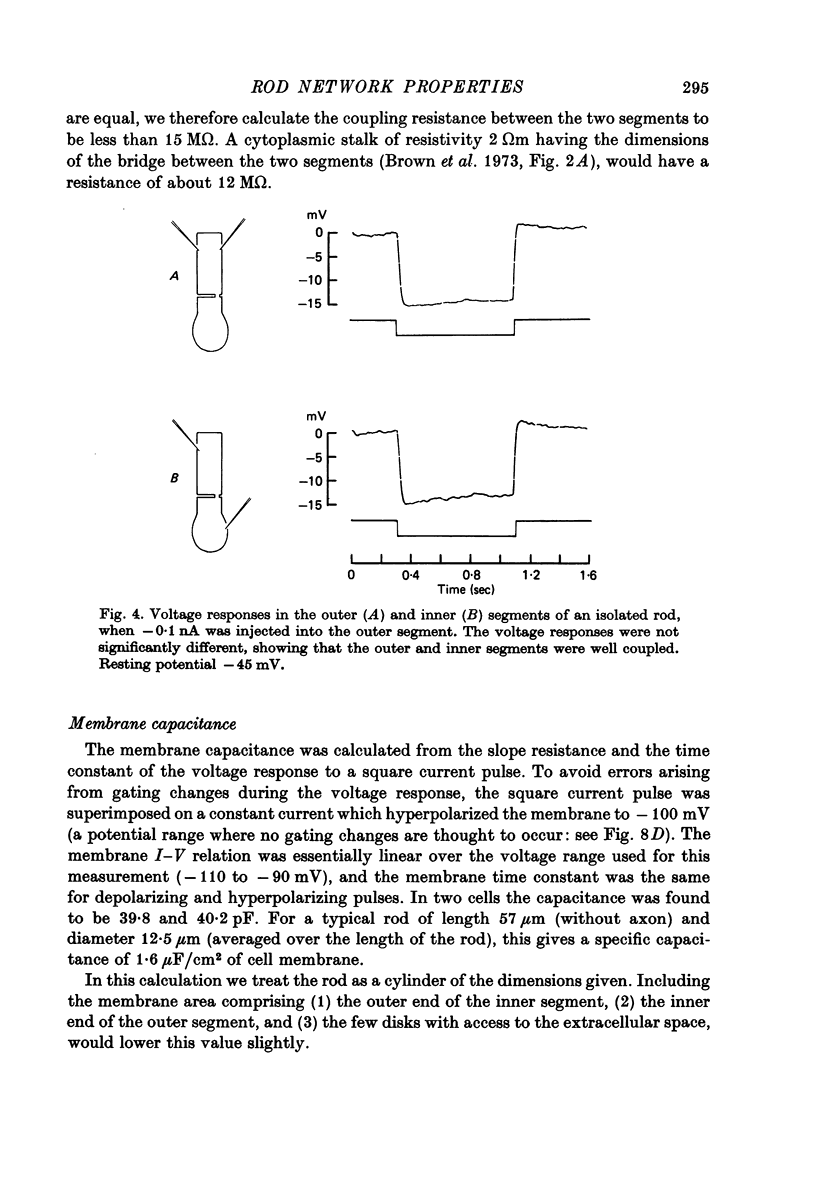
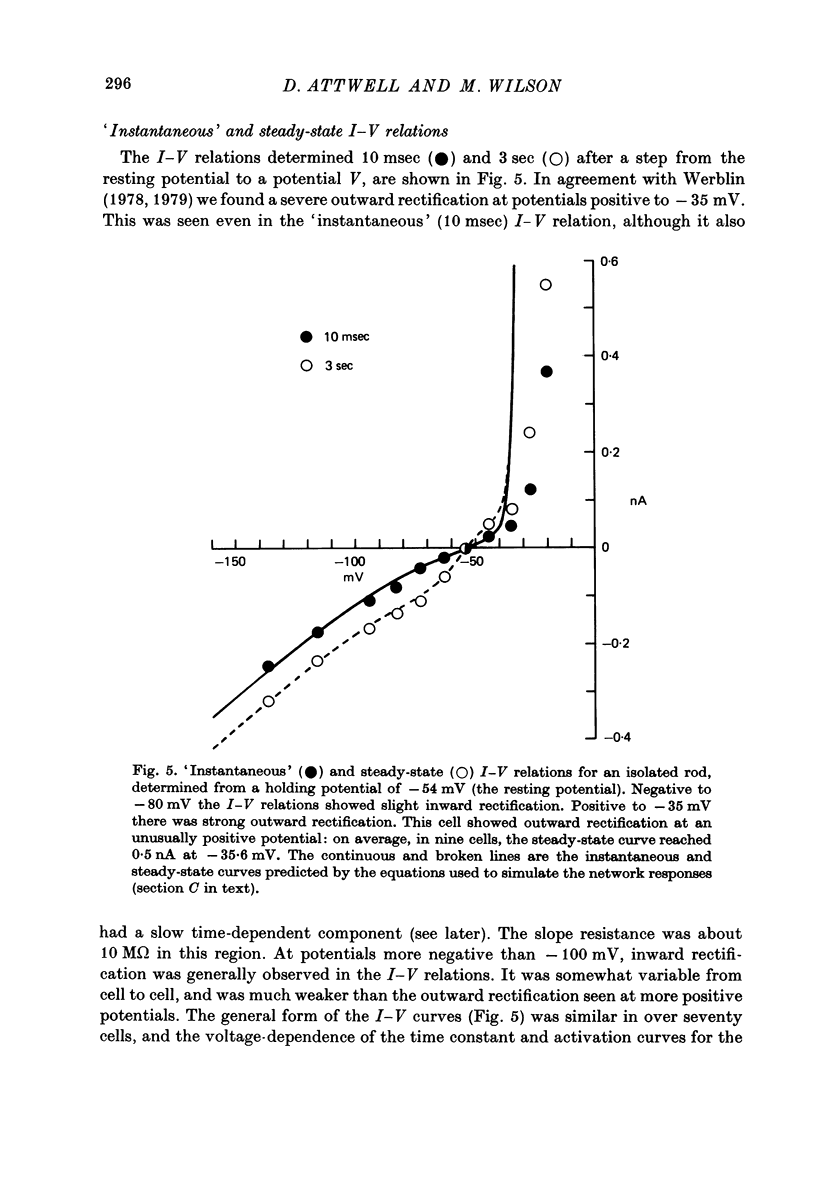
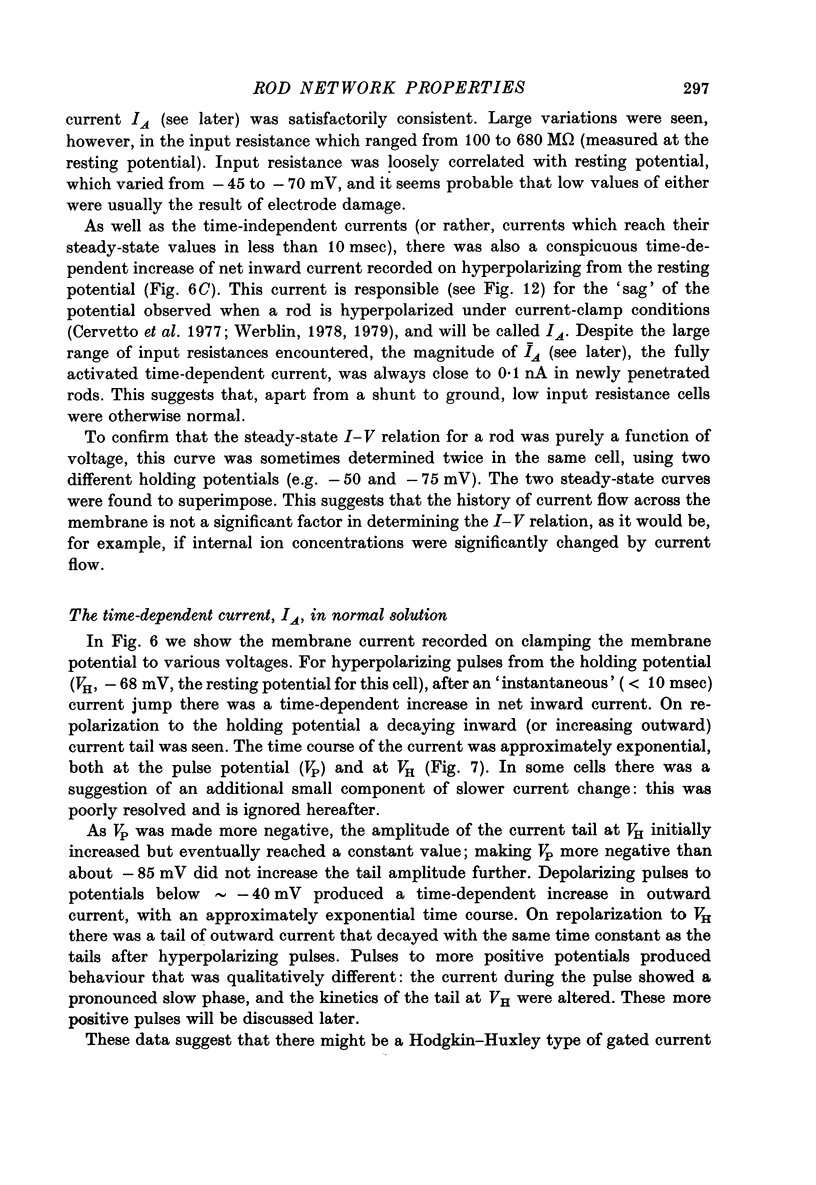
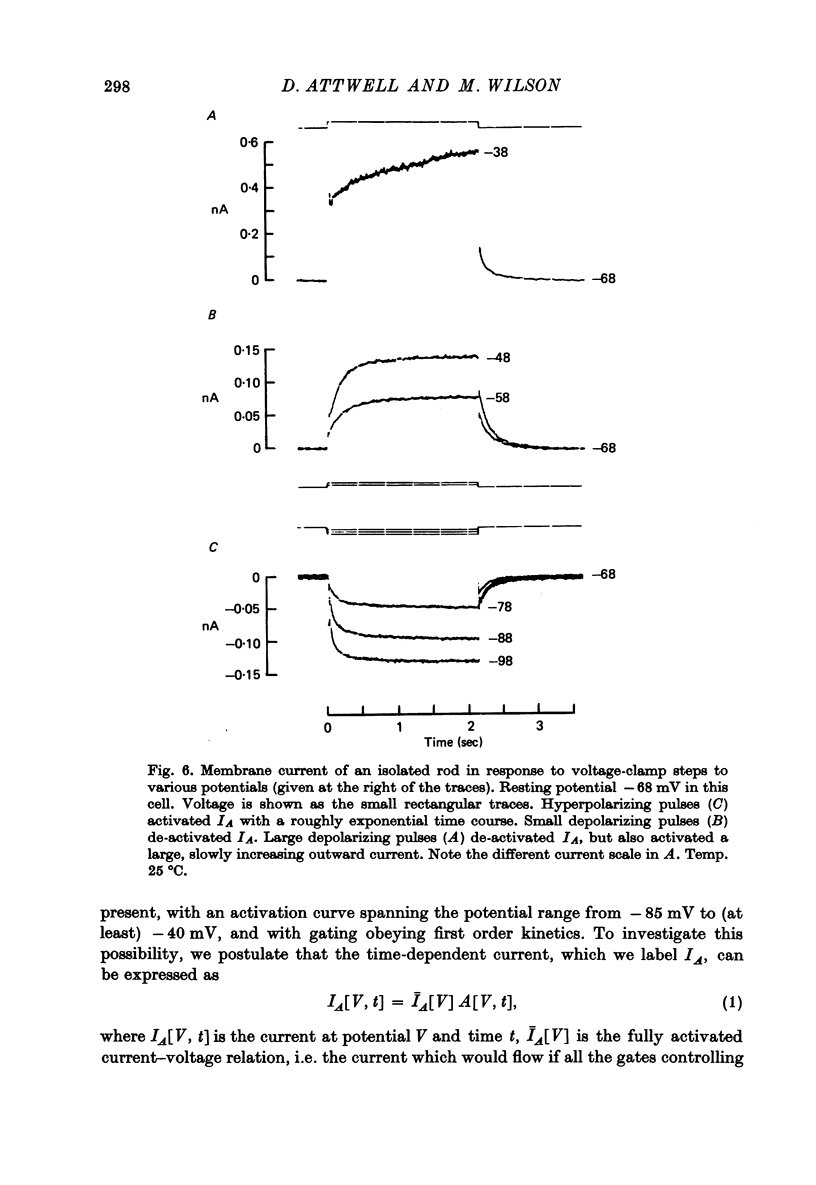
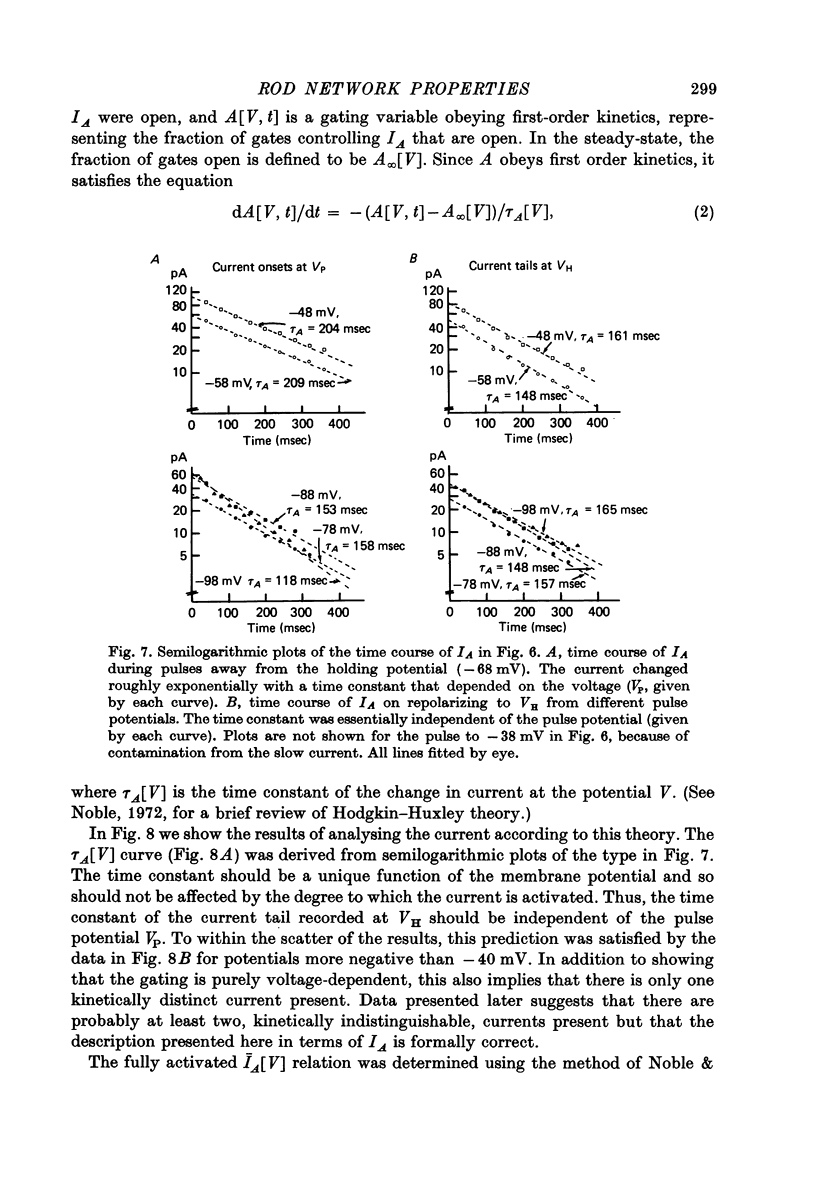
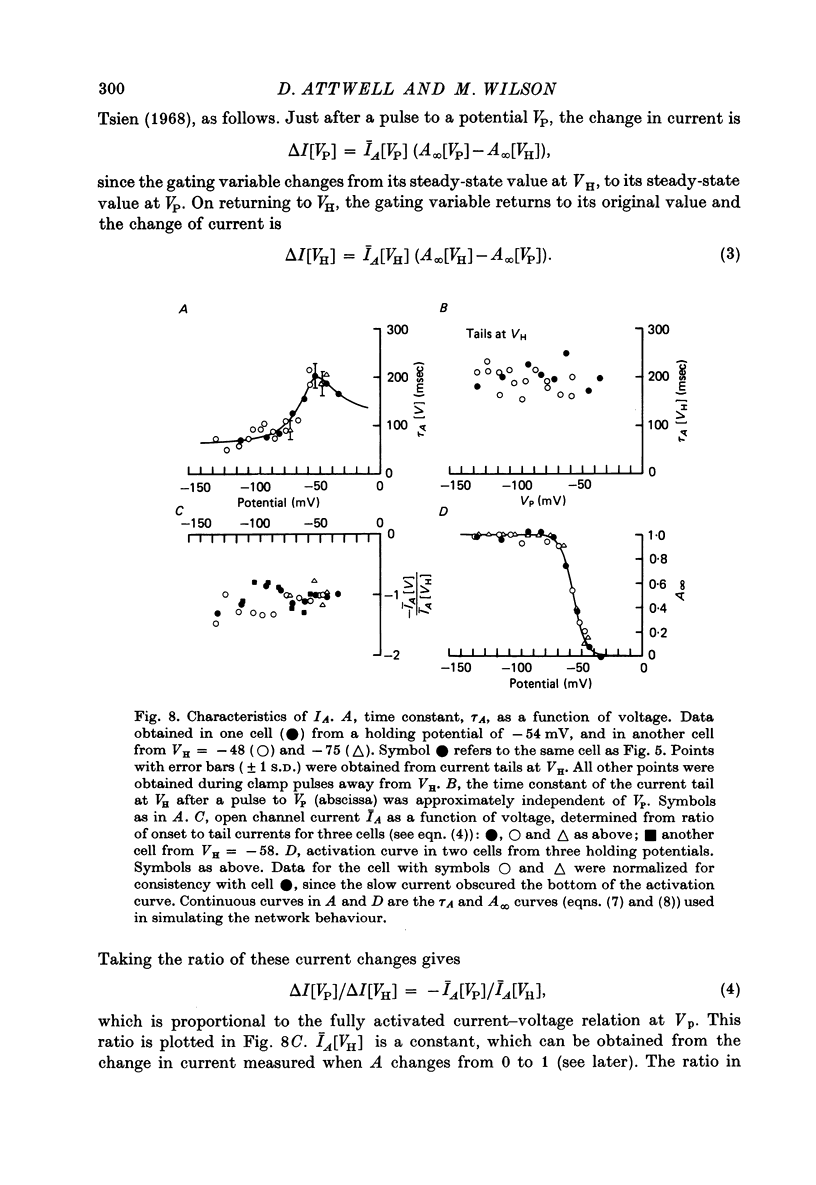
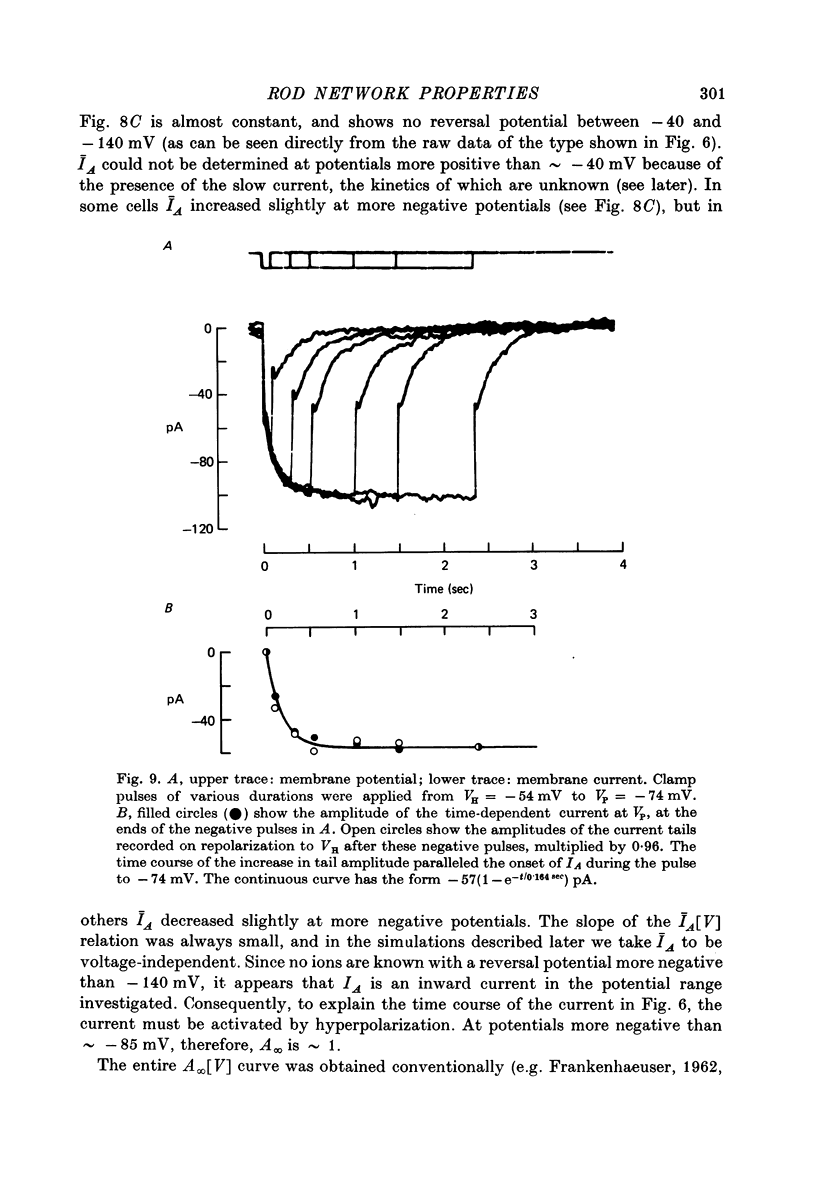
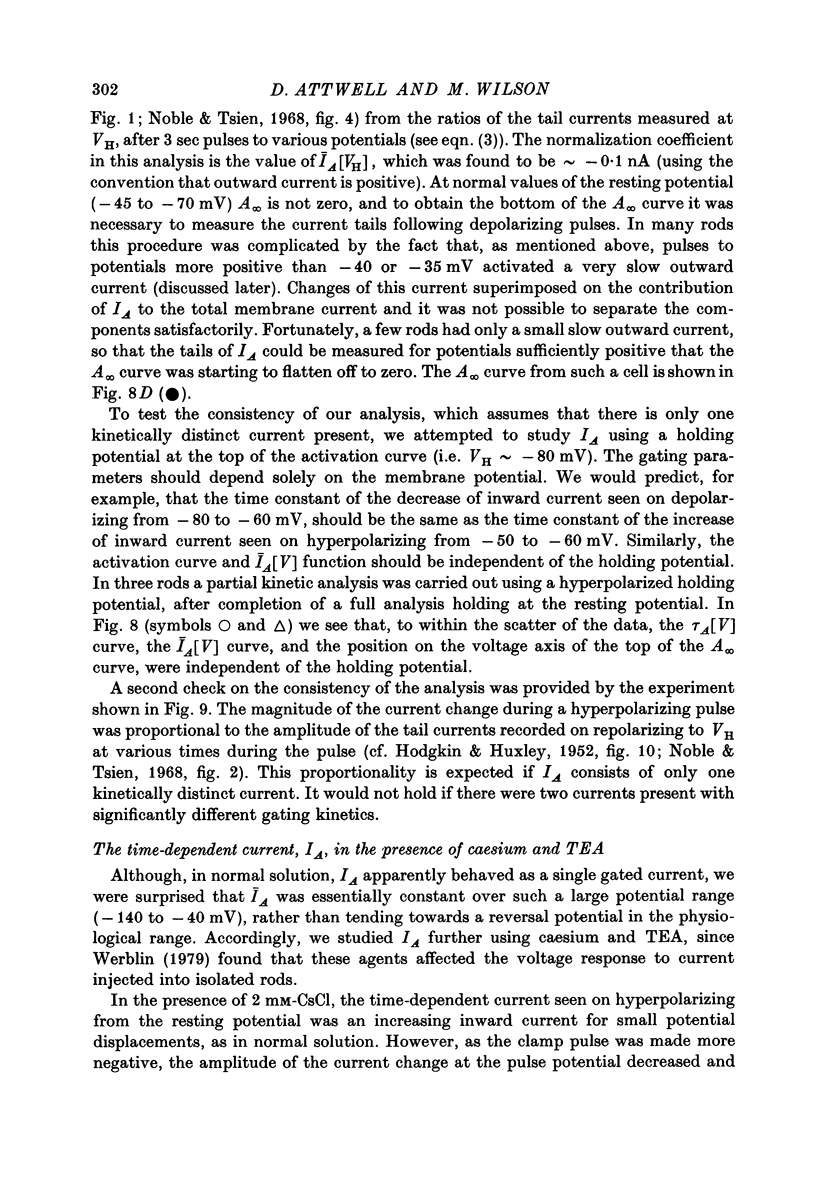
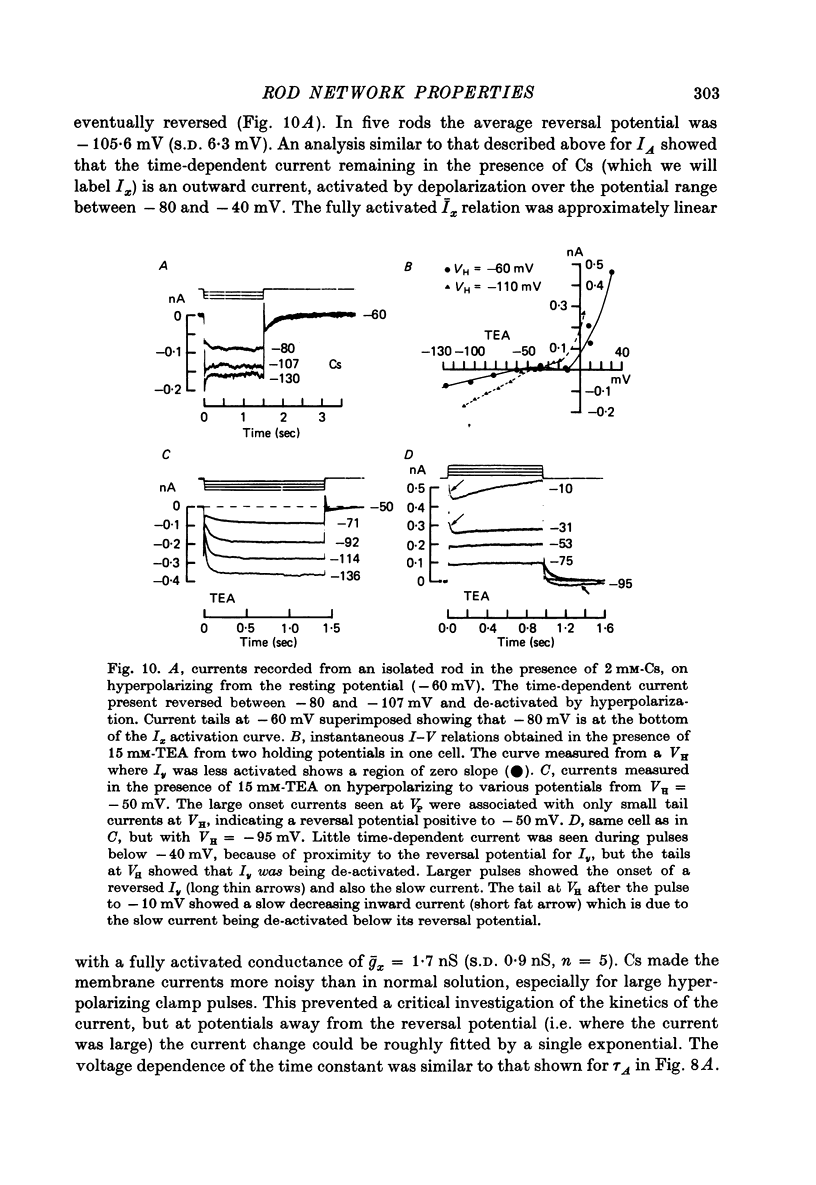
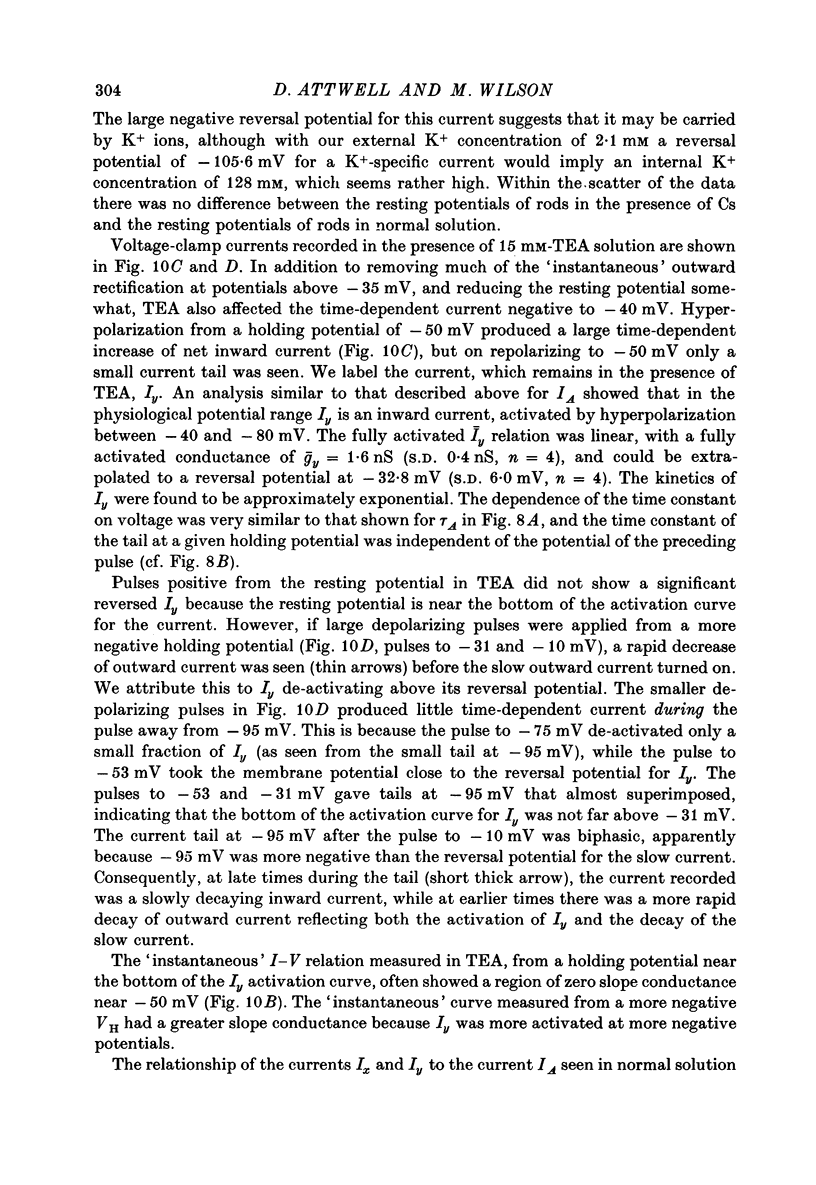
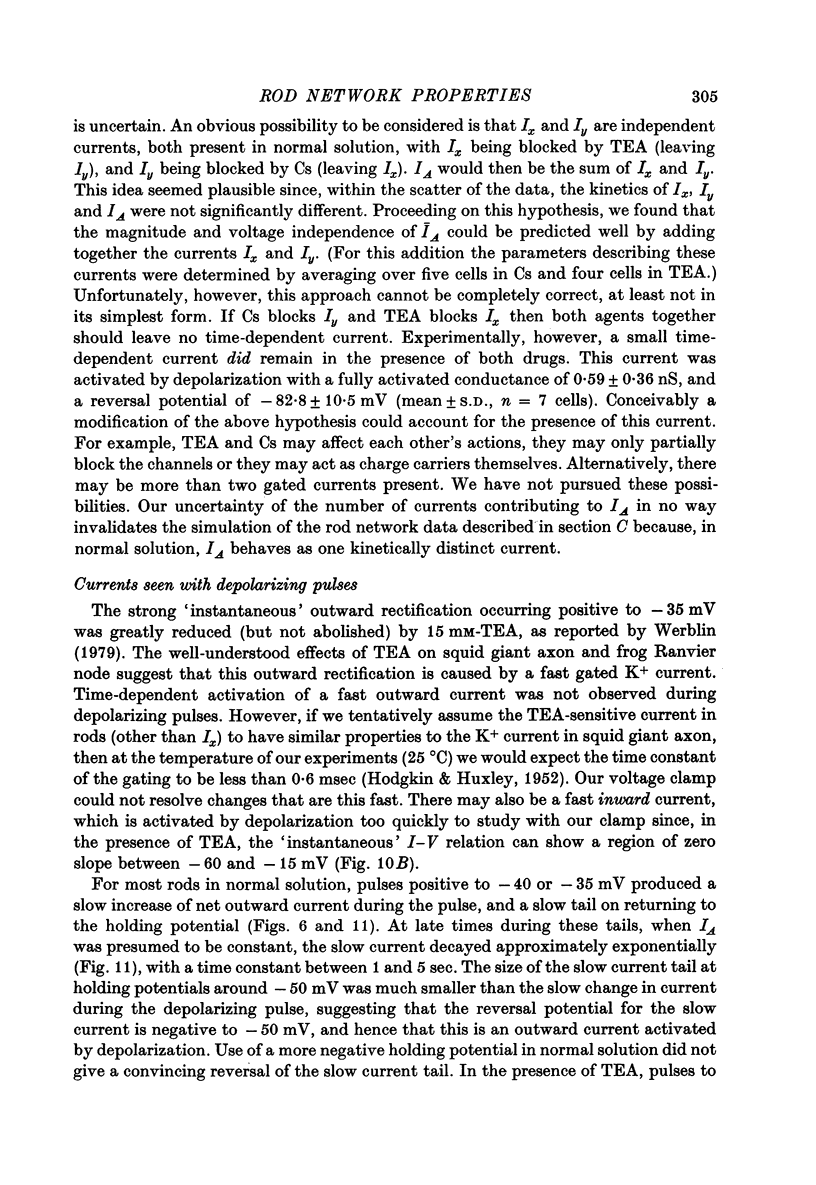
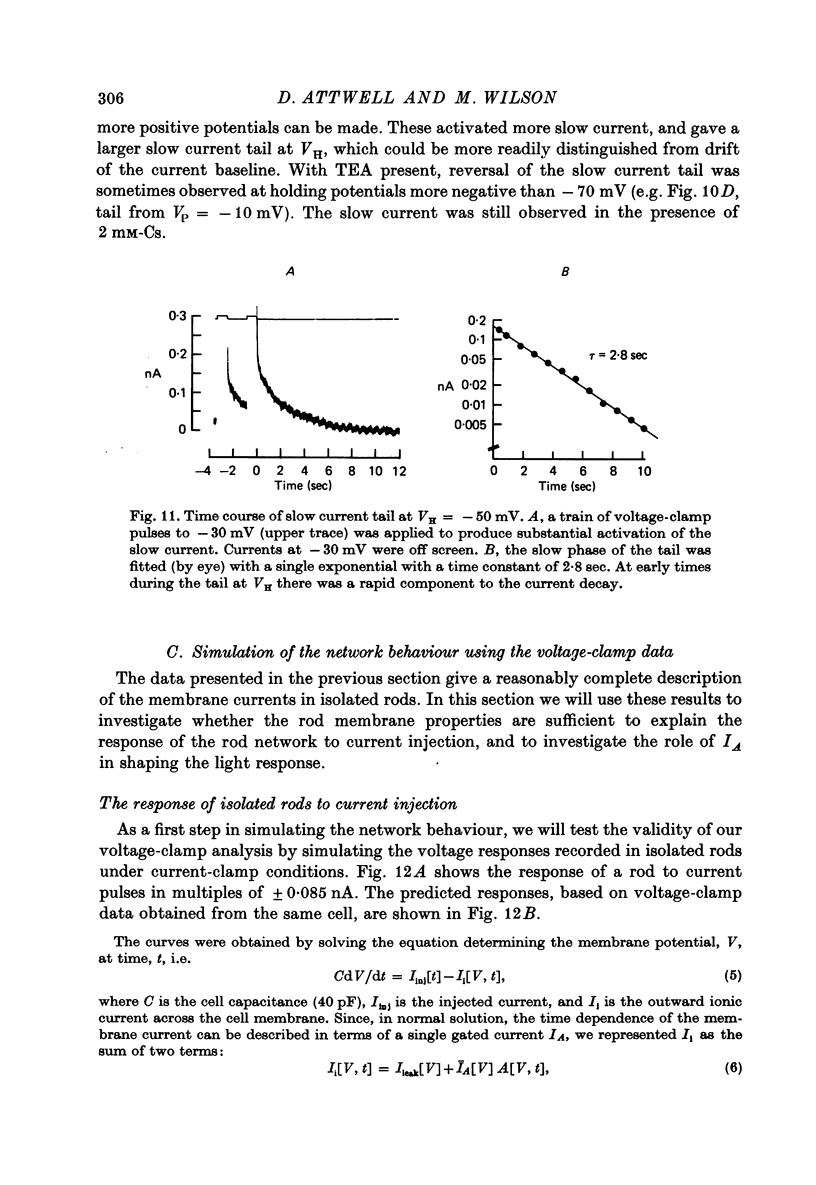
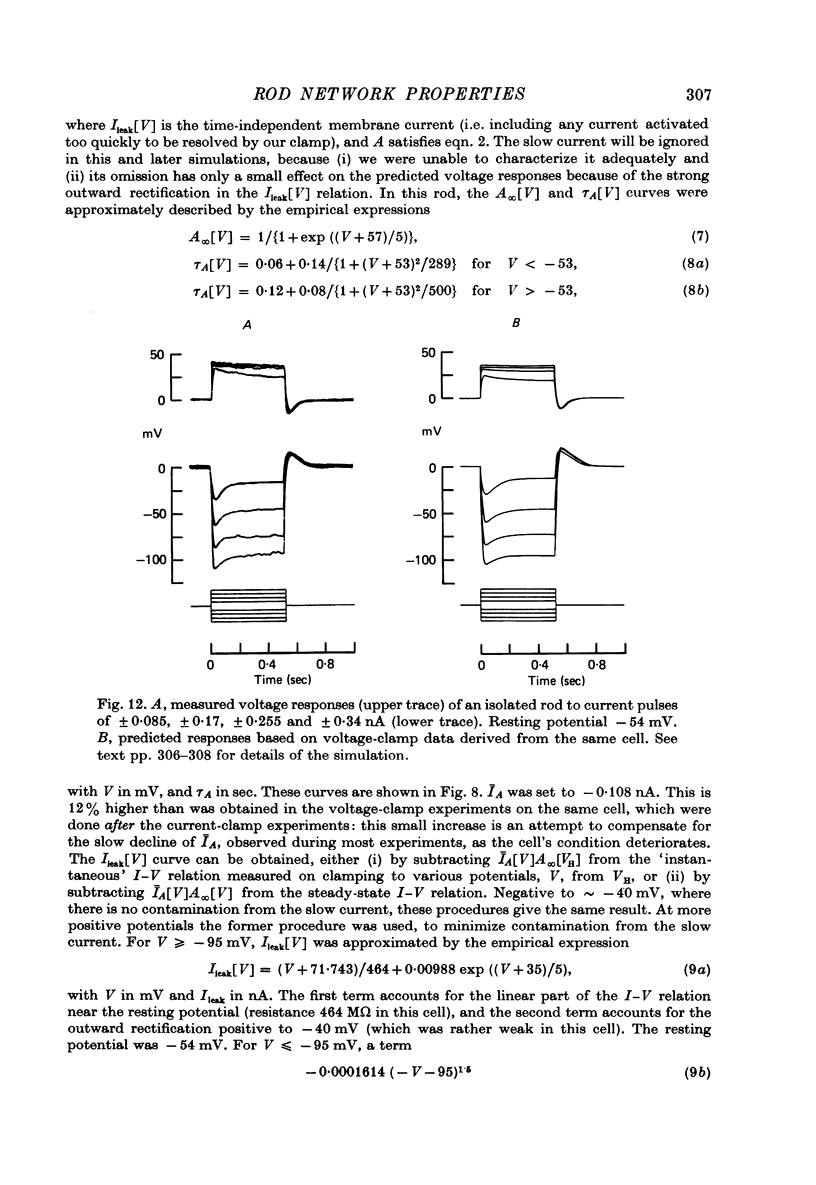
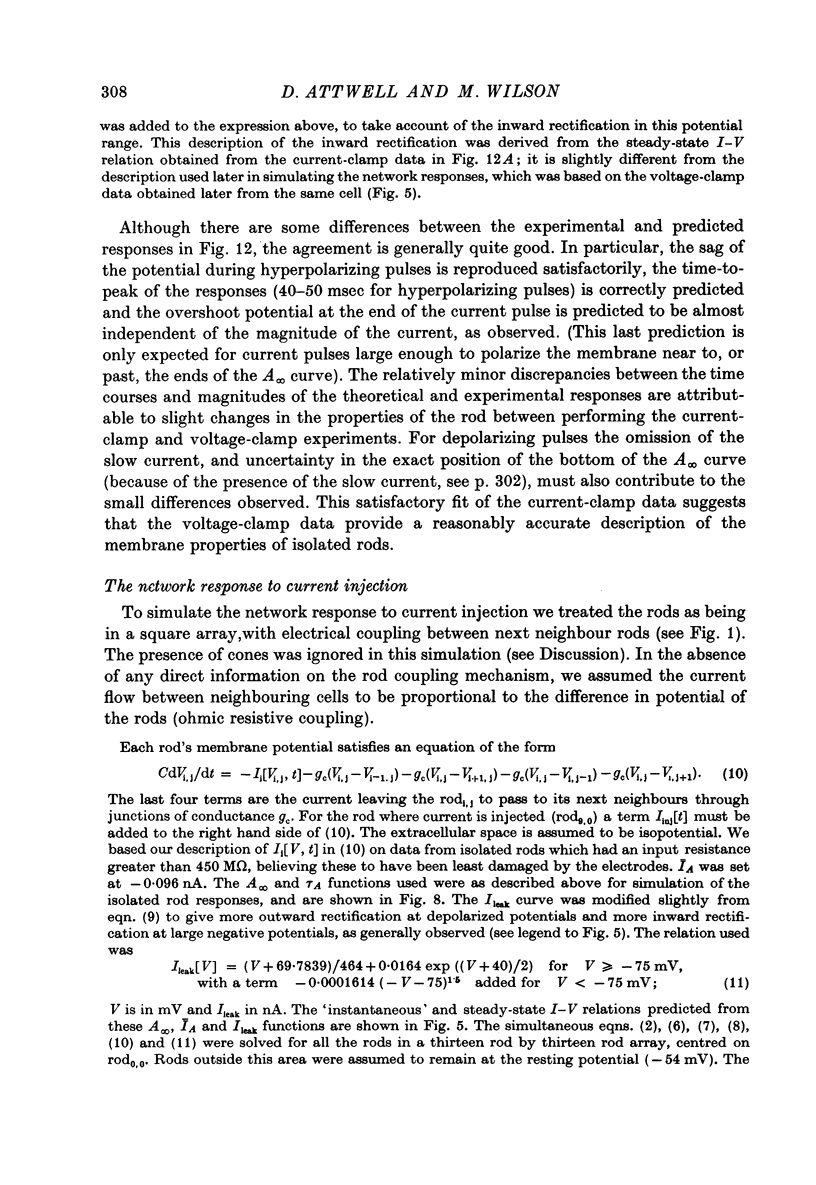
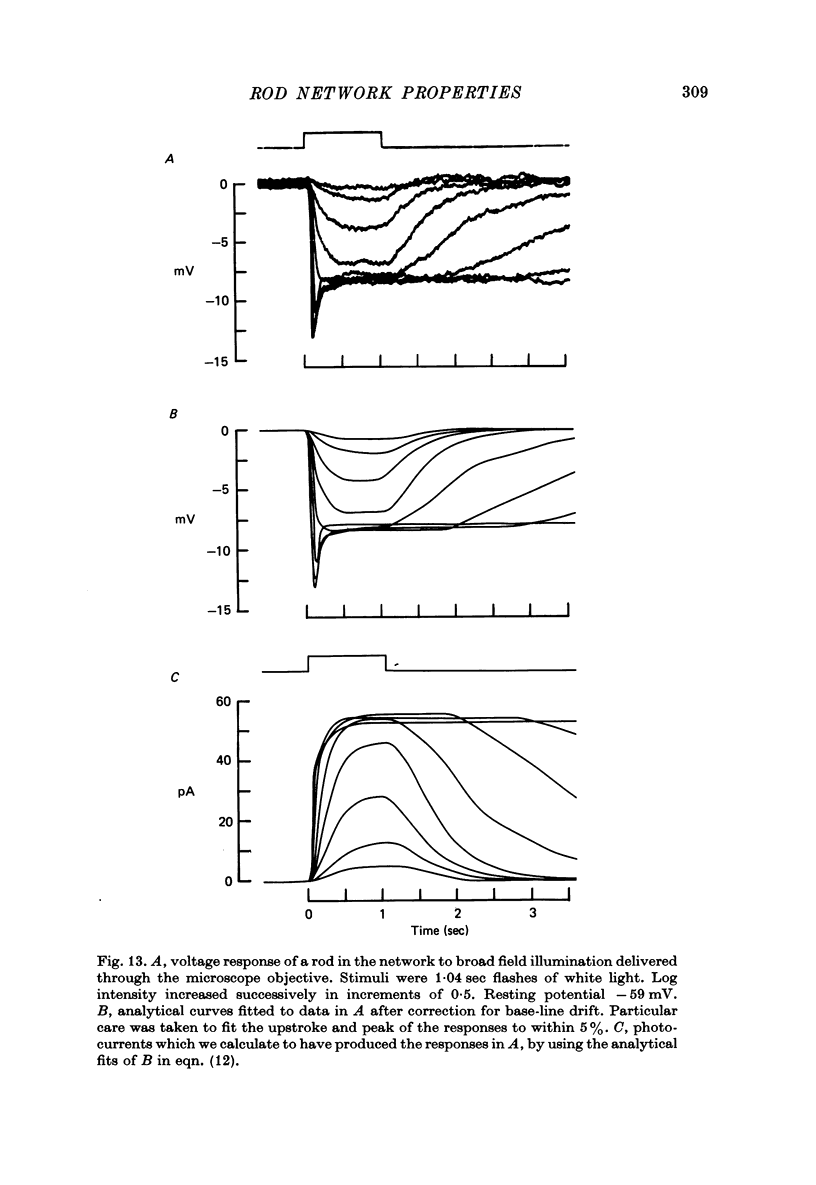
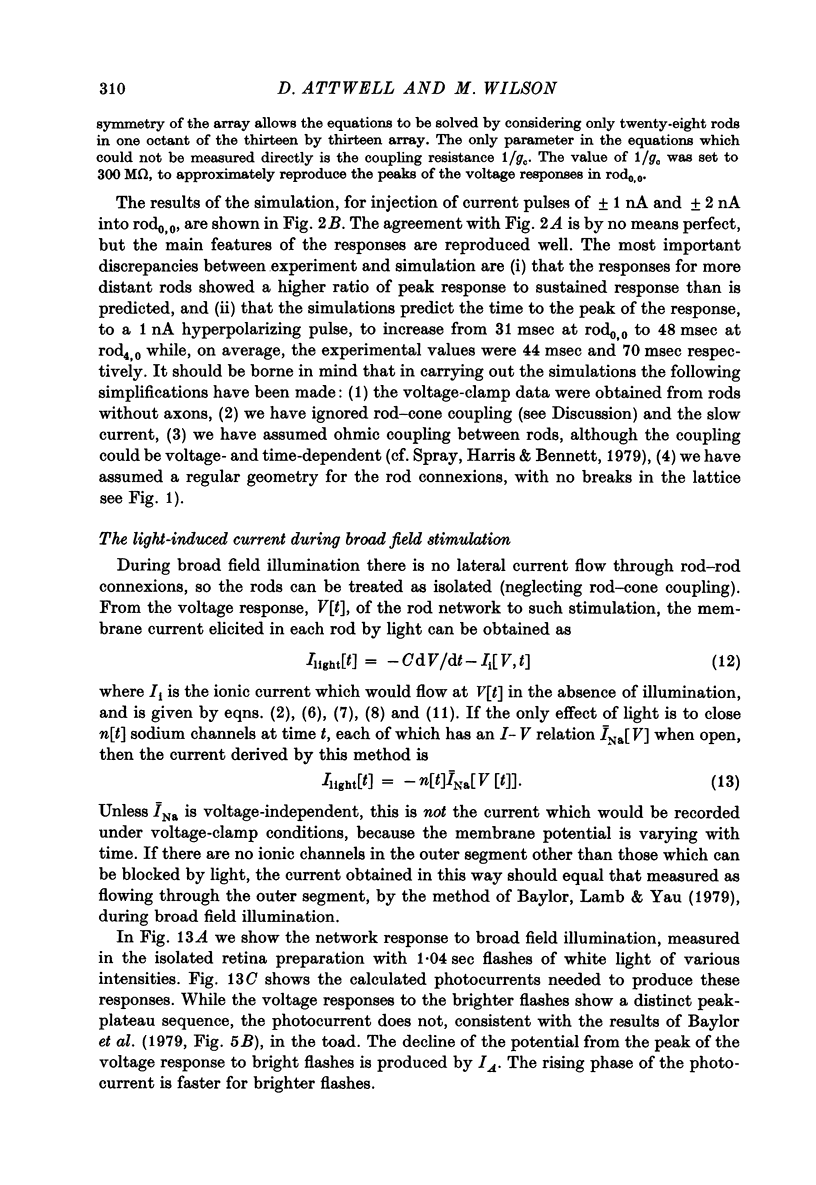
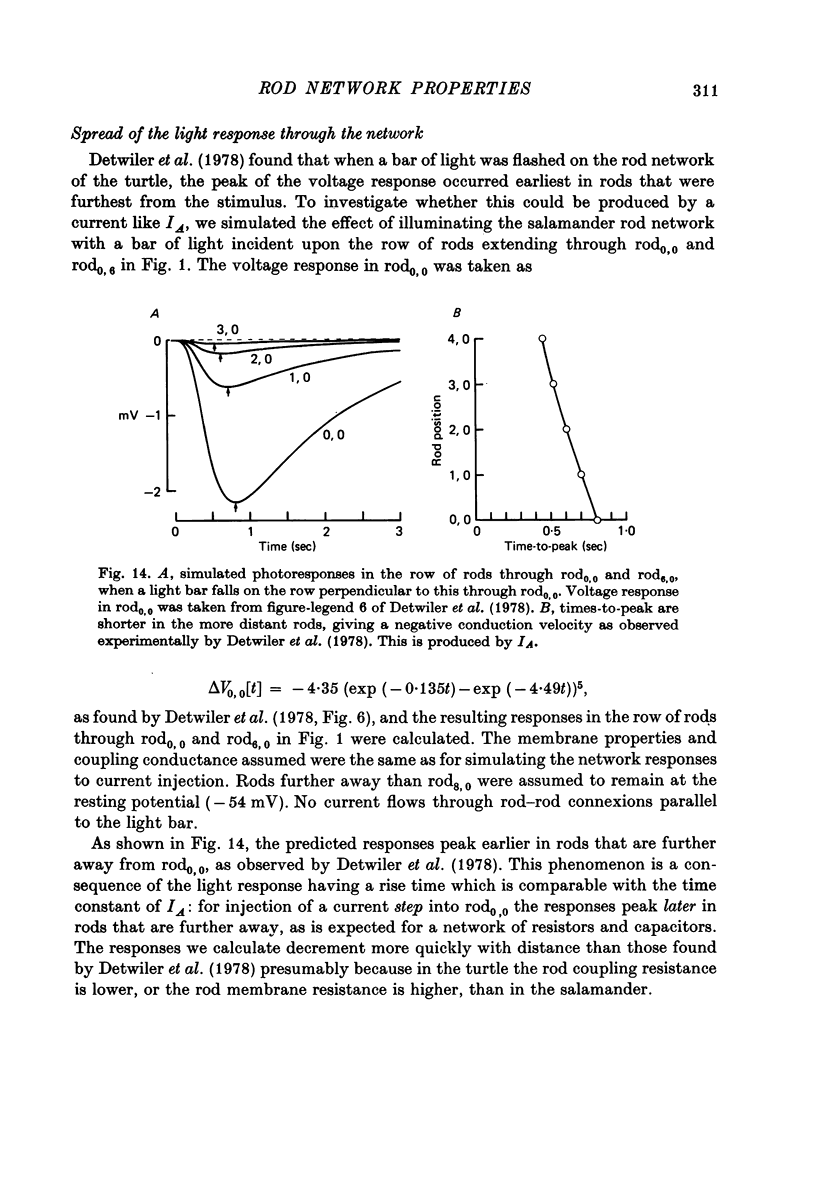
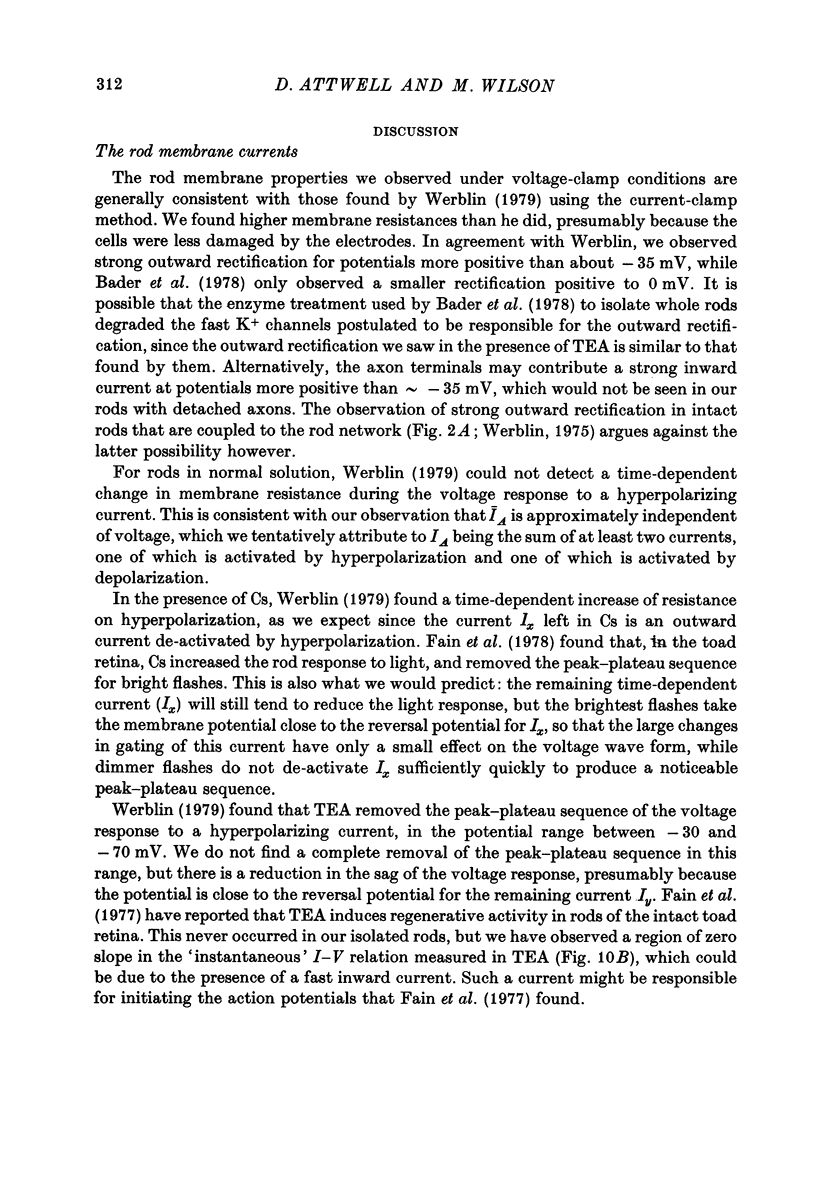
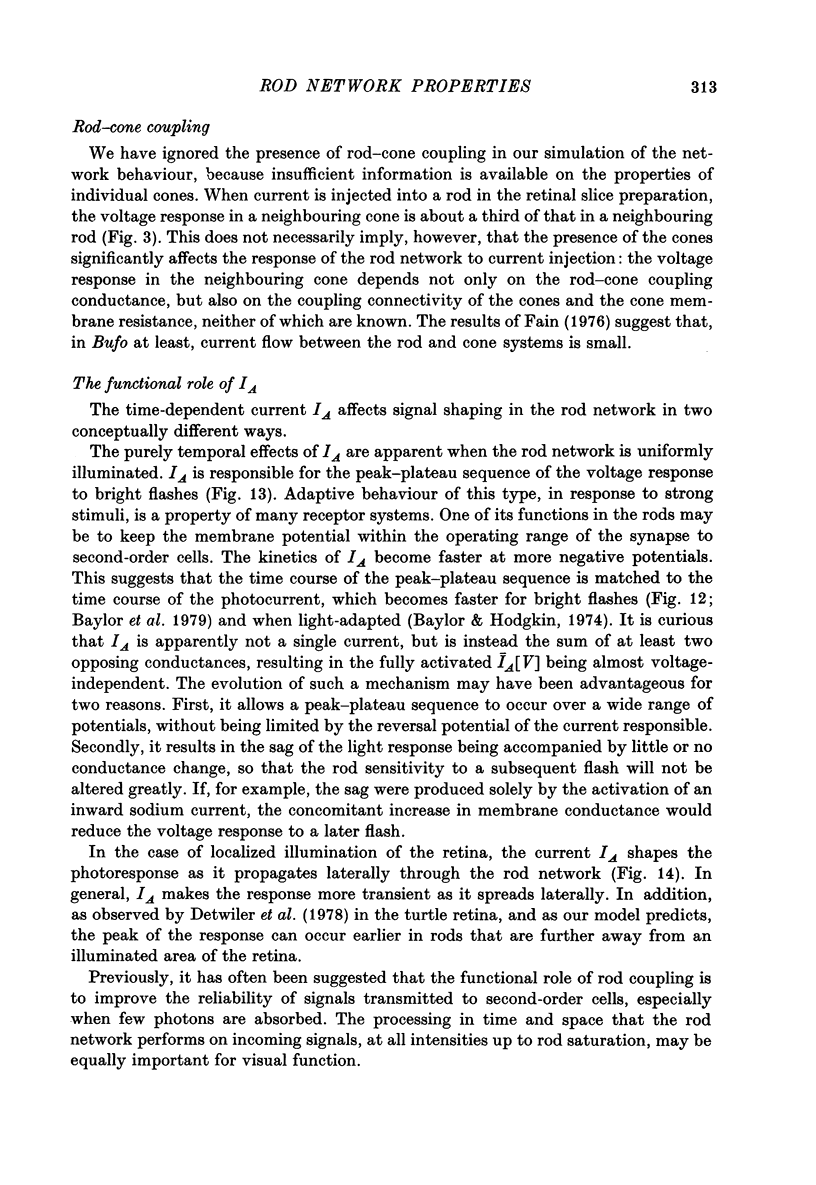
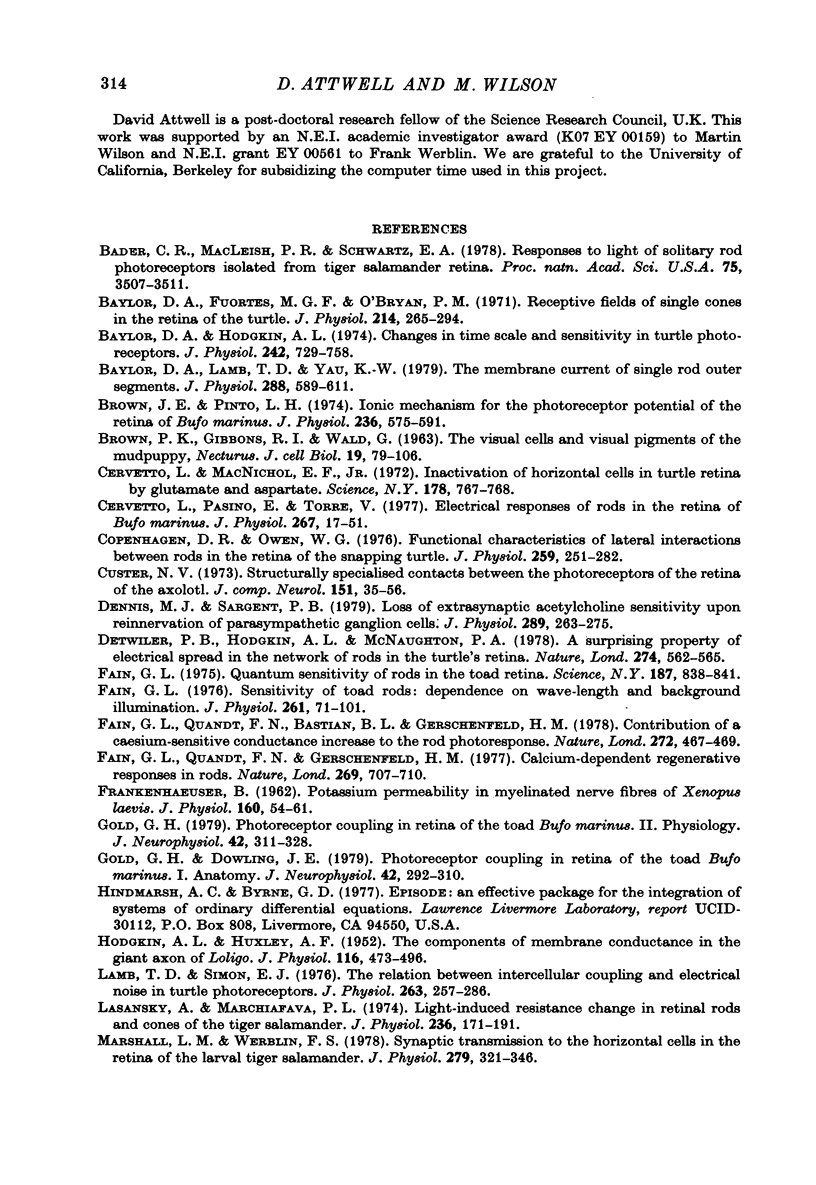
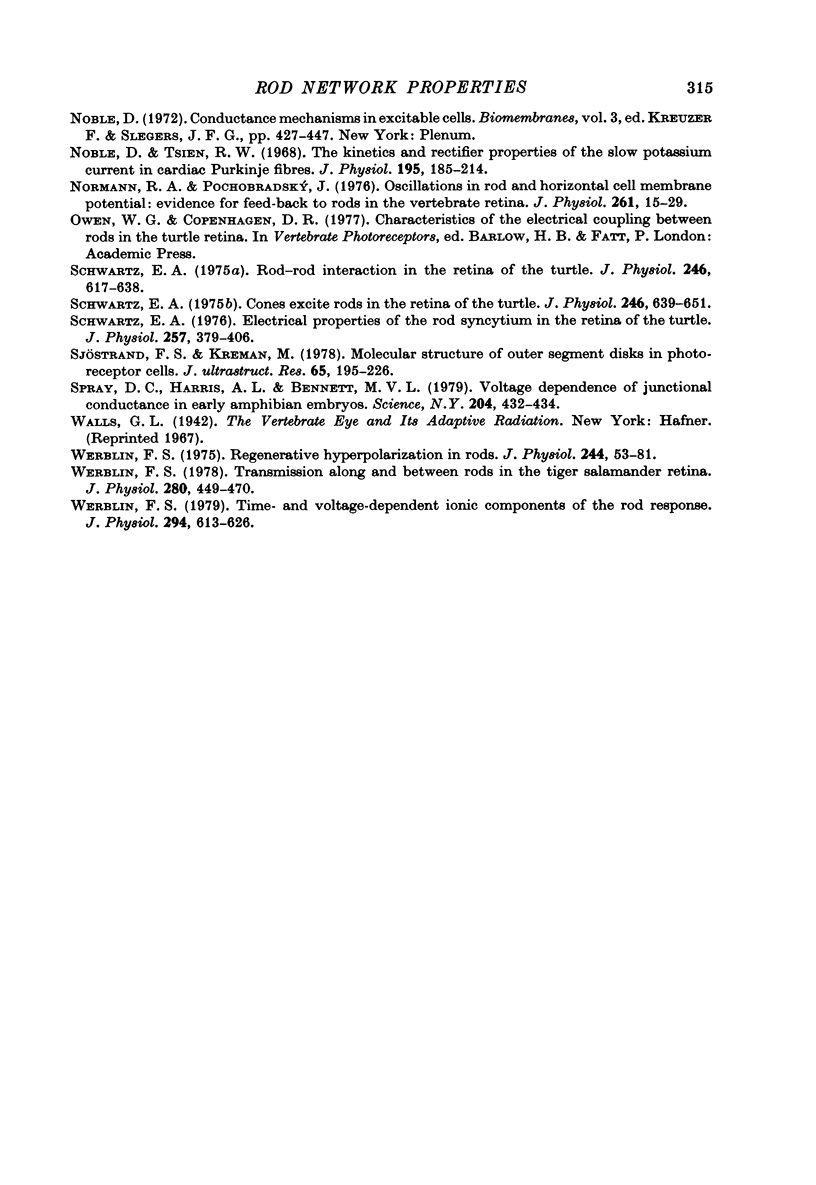
Selected References
These references are in PubMed. This may not be the complete list of references from this article.
- BROWN P. K., GIBBONS I. R., WALD G. THE VISUAL CELLS AND VISUAL PIGMENT OF THE MUDPUPPY, NECTURUS. J Cell Biol. 1963 Oct;19:79–106. doi: 10.1083/jcb.19.1.79. [DOI] [PMC free article] [PubMed] [Google Scholar]
- Bader C. R., MacLeish P. R., Schwartz E. A. Responses to light of solitary rod photoreceptors isolated from tiger salamander retina. Proc Natl Acad Sci U S A. 1978 Jul;75(7):3507–3511. doi: 10.1073/pnas.75.7.3507. [DOI] [PMC free article] [PubMed] [Google Scholar]
- Baylor D. A., Fuortes M. G., O'Bryan P. M. Receptive fields of cones in the retina of the turtle. J Physiol. 1971 Apr;214(2):265–294. doi: 10.1113/jphysiol.1971.sp009432. [DOI] [PMC free article] [PubMed] [Google Scholar]
- Baylor D. A., Hodgkin A. L. Changes in time scale and sensitivity in turtle photoreceptors. J Physiol. 1974 Nov;242(3):729–758. doi: 10.1113/jphysiol.1974.sp010732. [DOI] [PMC free article] [PubMed] [Google Scholar]
- Baylor D. A., Lamb T. D., Yau K. W. The membrane current of single rod outer segments. J Physiol. 1979 Mar;288:589–611. [PMC free article] [PubMed] [Google Scholar]
- Brown J. E., Pinto L. H. Ionic mechanism for the photoreceptor potential of the retina of Bufo marinus. J Physiol. 1974 Feb;236(3):575–591. doi: 10.1113/jphysiol.1974.sp010453. [DOI] [PMC free article] [PubMed] [Google Scholar]
- Cervetto L., MacNichol E. F., Jr Inactivation of horizontal cells in turtle retina by glutamate and aspartate. Science. 1972 Nov 17;178(4062):767–768. doi: 10.1126/science.178.4062.767. [DOI] [PubMed] [Google Scholar]
- Cervetto L., Pasino E., Torre V. Electrical responses of rods in the retina of Bufo marinus. J Physiol. 1977 May;267(1):17–51. doi: 10.1113/jphysiol.1977.sp011799. [DOI] [PMC free article] [PubMed] [Google Scholar]
- Copenhagen D. R., Owen W. G. Functional characteristics of lateral interactions between rods in the retina of the snapping turtle. J Physiol. 1976 Jul;259(2):251–282. doi: 10.1113/jphysiol.1976.sp011465. [DOI] [PMC free article] [PubMed] [Google Scholar]
- Custer N. V. Structurally specialized contacts between the photoreceptors of the retina of the axolotl. J Comp Neurol. 1973 Sep 1;151(1):35–56. doi: 10.1002/cne.901510104. [DOI] [PubMed] [Google Scholar]
- Dennis M. J., Sargent P. B. Loss of extrasynaptic acetylcholine sensitivity upon reinnervation of parasympathetic ganglion cells. J Physiol. 1979 Apr;289:263–275. doi: 10.1113/jphysiol.1979.sp012736. [DOI] [PMC free article] [PubMed] [Google Scholar]
- Detwiler P. B., Hodgkin A. L., McNaughton P. A. A surprising property of electrical spread in the network of rods in the turtle's retina. Nature. 1978 Aug 10;274(5671):562–565. doi: 10.1038/274562a0. [DOI] [PubMed] [Google Scholar]
- FRANKENHAEUSER B. Potassium permeability in myelinated nerve fibres of Xenopus laevis. J Physiol. 1962 Jan;160:54–61. doi: 10.1113/jphysiol.1962.sp006834. [DOI] [PMC free article] [PubMed] [Google Scholar]
- Fain G. L., Quandt F. N., Bastian B. L., Gerschenfeld H. M. Contribution of a caesium-sensitive conductance increase to the rod photoresponse. Nature. 1978 Mar 30;272(5652):466–469. doi: 10.1038/272467a0. [DOI] [PubMed] [Google Scholar]
- Fain G. L., Quandt F. N., Gerschenfeld H. M. Calcium-dependent regenerative responses in rods. Nature. 1977 Oct 20;269(5630):707–710. doi: 10.1038/269707a0. [DOI] [PubMed] [Google Scholar]
- Fain G. L. Quantum sensitivity of rods in the toad retina. Science. 1975 Mar 7;187(4179):838–841. doi: 10.1126/science.1114328. [DOI] [PubMed] [Google Scholar]
- Fain G. L. Sensitivity of toad rods: Dependence on wave-length and background illumination. J Physiol. 1976 Sep;261(1):71–101. doi: 10.1113/jphysiol.1976.sp011549. [DOI] [PMC free article] [PubMed] [Google Scholar]
- Gold G. H., Dowling J. E. Photoreceptor coupling in retina of the toad, Bufo marinus. I. Anatomy. J Neurophysiol. 1979 Jan;42(1 Pt 1):292–310. doi: 10.1152/jn.1979.42.1.292. [DOI] [PubMed] [Google Scholar]
- Gold G. H. Photoreceptor coupling in retina of the toad, Bufo marinus. II. Physiology. J Neurophysiol. 1979 Jan;42(1 Pt 1):311–328. doi: 10.1152/jn.1979.42.1.311. [DOI] [PubMed] [Google Scholar]
- HODGKIN A. L., HUXLEY A. F. The components of membrane conductance in the giant axon of Loligo. J Physiol. 1952 Apr;116(4):473–496. doi: 10.1113/jphysiol.1952.sp004718. [DOI] [PMC free article] [PubMed] [Google Scholar]
- Lamb T. D., Simon E. J. The relation between intercellular coupling and electrical noise in turtle photoreceptors. J Physiol. 1976 Dec;263(2):257–286. doi: 10.1113/jphysiol.1976.sp011631. [DOI] [PMC free article] [PubMed] [Google Scholar]
- Lasansky A., Marchiafava P. L. Light-induced resistance changes in retinal rods and cones of the tiger salamander. J Physiol. 1974 Jan;236(1):171–191. doi: 10.1113/jphysiol.1974.sp010429. [DOI] [PMC free article] [PubMed] [Google Scholar]
- Marshall L. M., Werblin F. S. Synaptic transmission to the horizontal cells in the retina of the larval tiger salamander. J Physiol. 1978 Jun;279:321–346. doi: 10.1113/jphysiol.1978.sp012347. [DOI] [PMC free article] [PubMed] [Google Scholar]
- Noble D. Conductance mechanisms in excitable cells. Biomembranes. 1972;3:427–447. doi: 10.1007/978-1-4684-0961-1_30. [DOI] [PubMed] [Google Scholar]
- Noble D., Tsien R. W. The kinetics and rectifier properties of the slow potassium current in cardiac Purkinje fibres. J Physiol. 1968 Mar;195(1):185–214. doi: 10.1113/jphysiol.1968.sp008454. [DOI] [PMC free article] [PubMed] [Google Scholar]
- Normann R. A., Pochobradský J. Oscillations in rod and horizontal cell membrane potential: evidence for feed-back to rods in the vertebrate retina. J Physiol. 1976 Sep;261(1):15–29. doi: 10.1113/jphysiol.1976.sp011546. [DOI] [PMC free article] [PubMed] [Google Scholar]
- Schwartz E. A. Cones excite rods in the retina of the turtle. J Physiol. 1975 Apr;246(3):639–651. doi: 10.1113/jphysiol.1975.sp010908. [DOI] [PMC free article] [PubMed] [Google Scholar]
- Schwartz E. A. Electrical properties of the rod syncytium in the retina of the turtle. J Physiol. 1976 May;257(2):379–406. doi: 10.1113/jphysiol.1976.sp011374. [DOI] [PMC free article] [PubMed] [Google Scholar]
- Schwartz E. A. Rod-rod interaction in the retina of the turtle. J Physiol. 1975 Apr;246(3):617–638. doi: 10.1113/jphysiol.1975.sp010907. [DOI] [PMC free article] [PubMed] [Google Scholar]
- Sjöstrand F. S., Kreman M. Molecular structure of outer segment disks in photoreceptor cells. J Ultrastruct Res. 1978 Dec;65(3):195–226. doi: 10.1016/s0022-5320(78)80059-8. [DOI] [PubMed] [Google Scholar]
- Spray D. C., Harris A. L., Bennett M. V. Voltage dependence of junctional conductance in early amphibian embryos. Science. 1979 Apr 27;204(4391):432–434. doi: 10.1126/science.312530. [DOI] [PubMed] [Google Scholar]
- Werblin F. S. Regenerative hyperpolarization in rods. J Physiol. 1975 Jan;244(1):53–81. doi: 10.1113/jphysiol.1975.sp010784. [DOI] [PMC free article] [PubMed] [Google Scholar]
- Werblin F. S. Time- and voltage-dependent ionic components of the rod response. J Physiol. 1979 Sep;294:613–626. doi: 10.1113/jphysiol.1979.sp012949. [DOI] [PMC free article] [PubMed] [Google Scholar]
- Werblin F. S. Transmission along and between rods in the tiger salamander retina. J Physiol. 1978 Jul;280:449–470. doi: 10.1113/jphysiol.1978.sp012394. [DOI] [PMC free article] [PubMed] [Google Scholar]