Abstract
A hypothesis of the nature of intracellular ice formation is proposed in which the osmotically driven water efflux that occurs in cells during freezing (caused by the increased osmotic pressure of the extracellular solution in the presence of ice) is viewed as the agent responsible for producing a rupture of the plasma membrane, thus allowing extracellular ice to propagate into the cytoplasm. This hypothesis is developed into a mathematical framework and the forces that are present during freezing are compared to the forces which are required to rupture membranes in circumstances unrelated to low temperatures. The theory is then applied to systems which have been previously studied to test implications of the theory on the nature of intracellular ice formation. The pressure that develops during freezing due to water flux is found to be sufficient to cause a rupture of the plasma membrane and the theory gives an accurate description of the phenomenology of intracellular ice formation.
Full text
PDF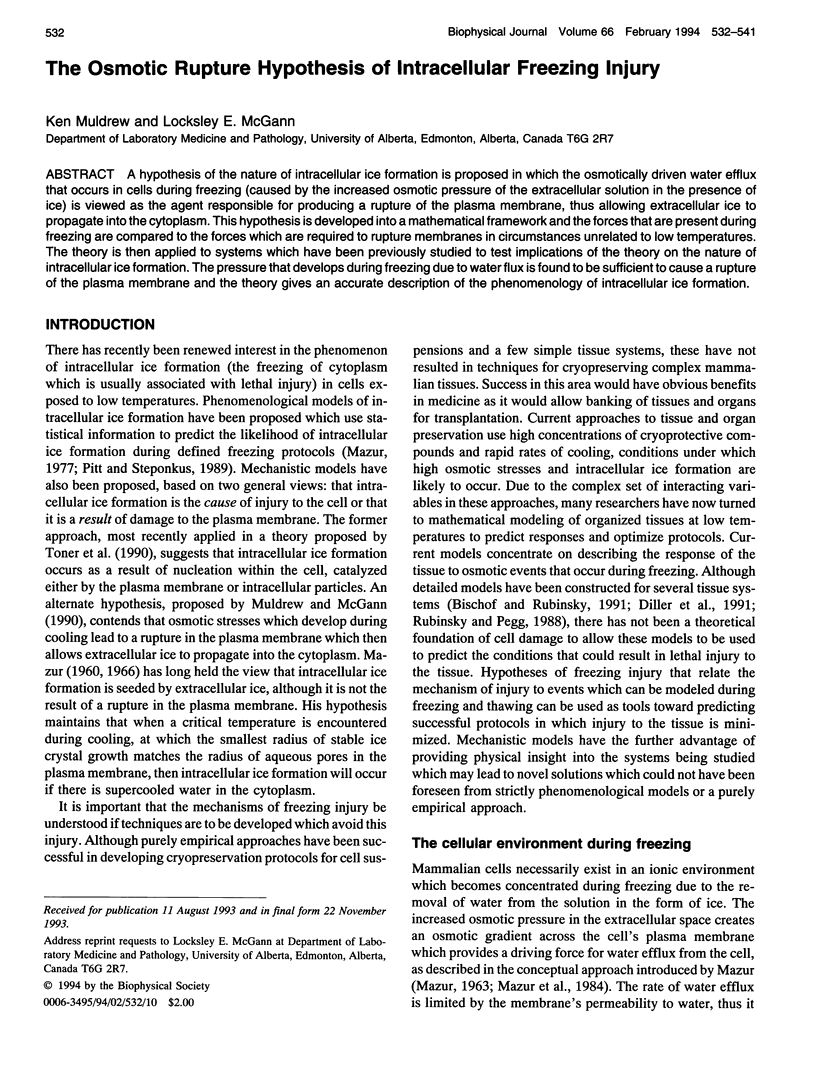
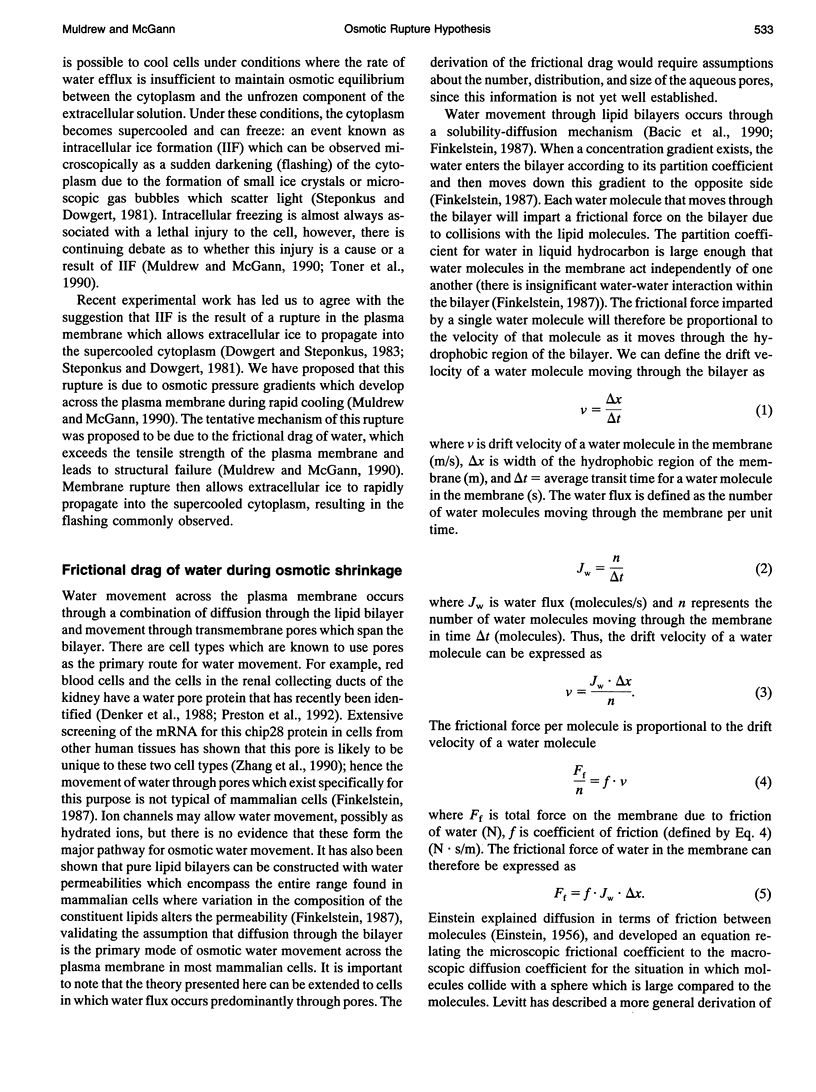
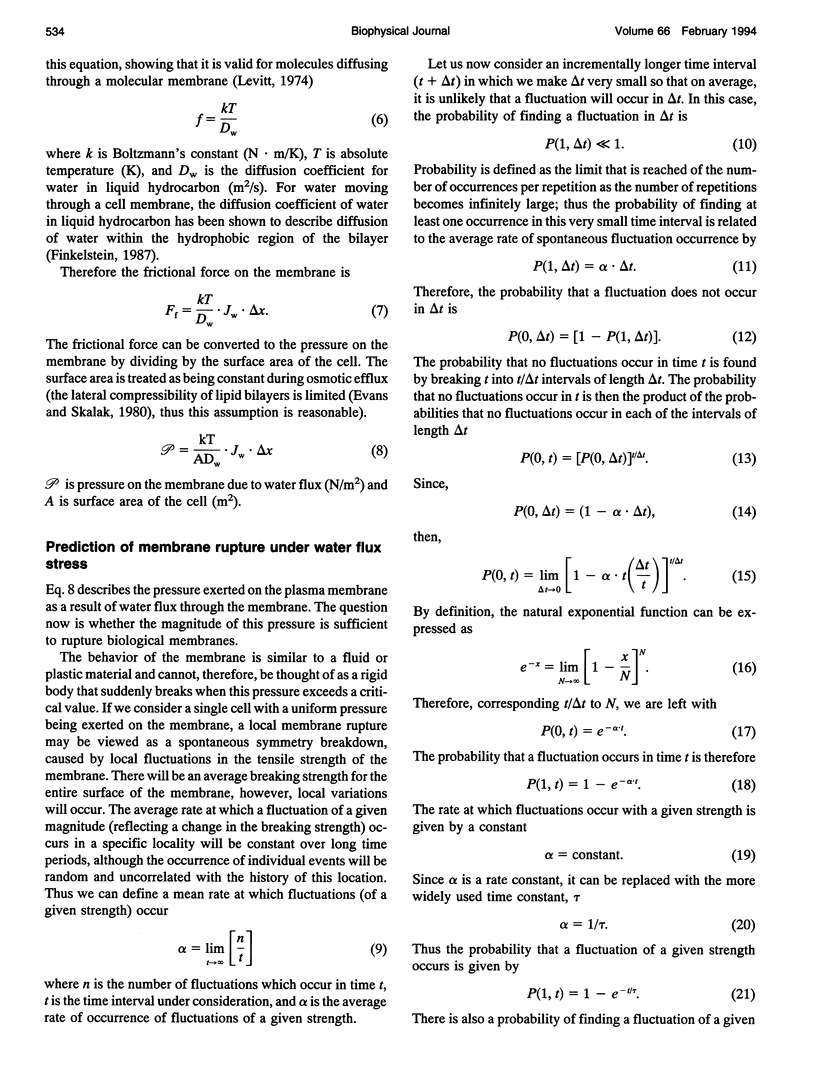
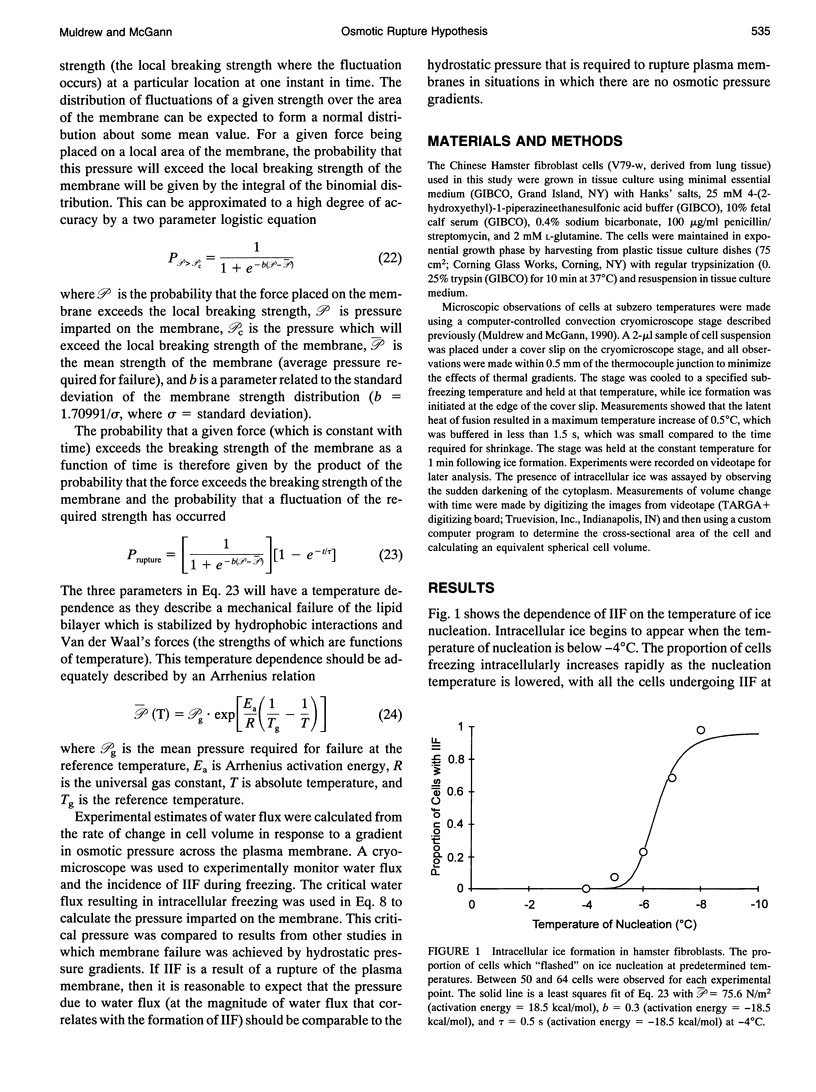
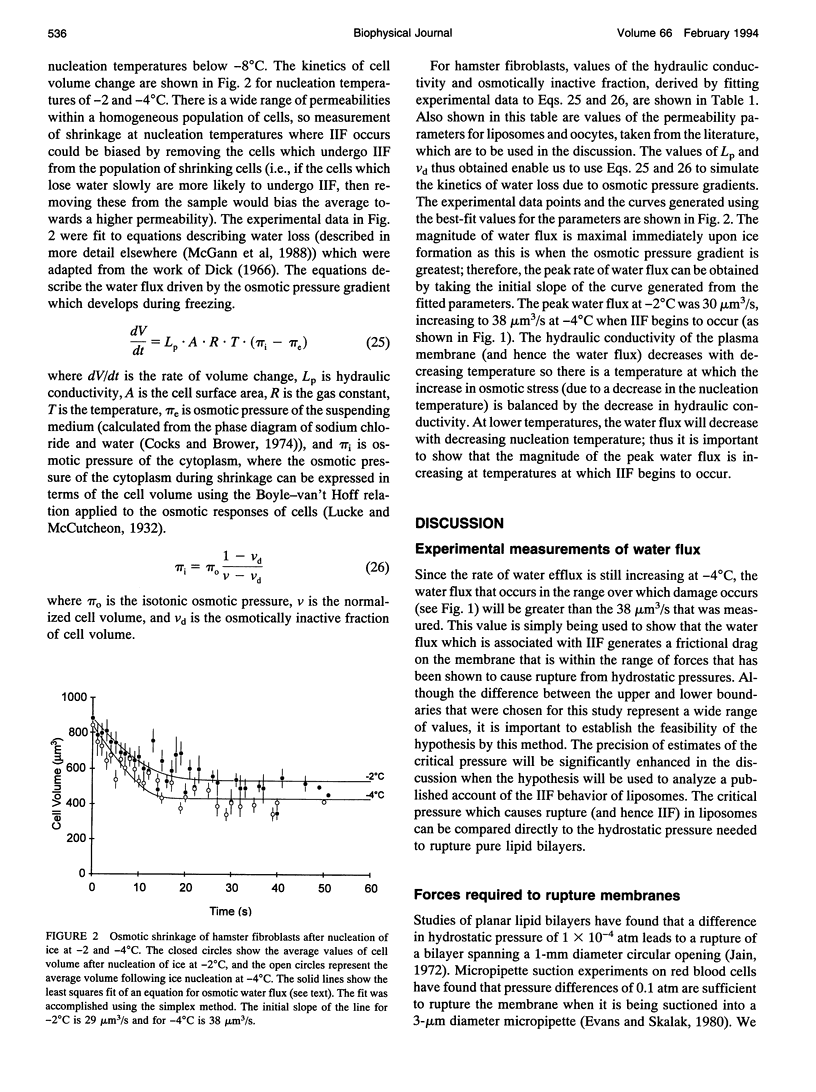
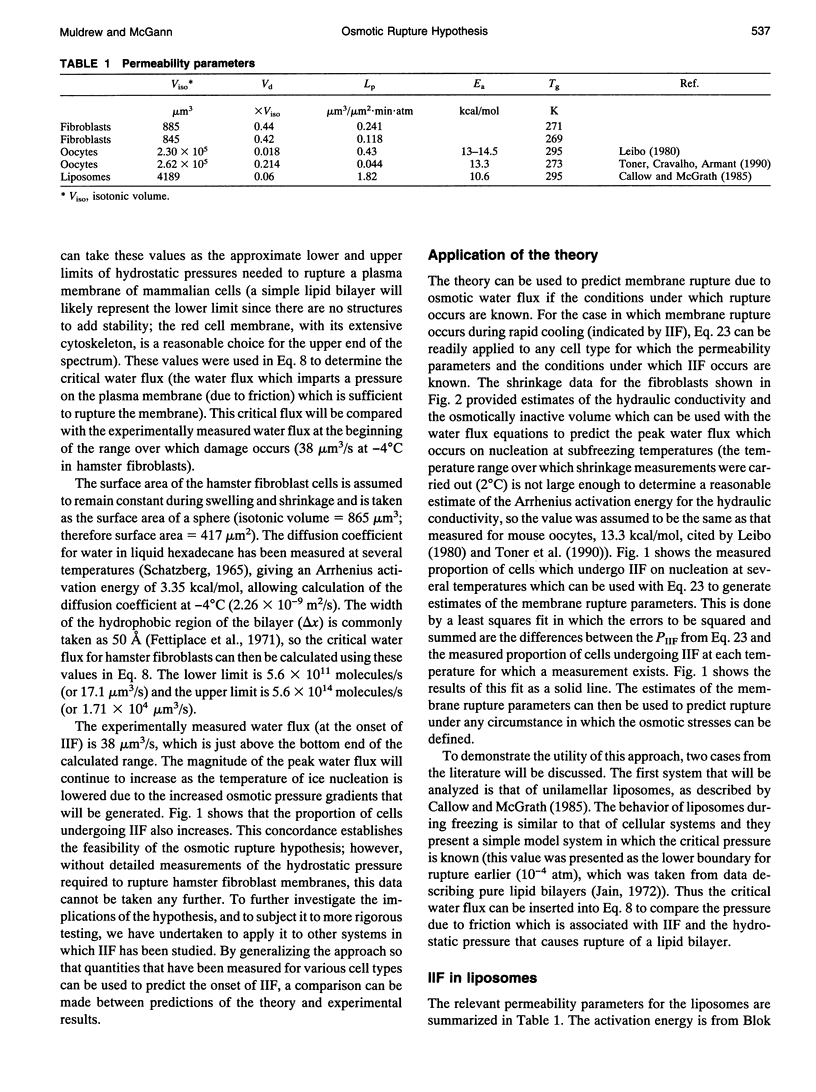
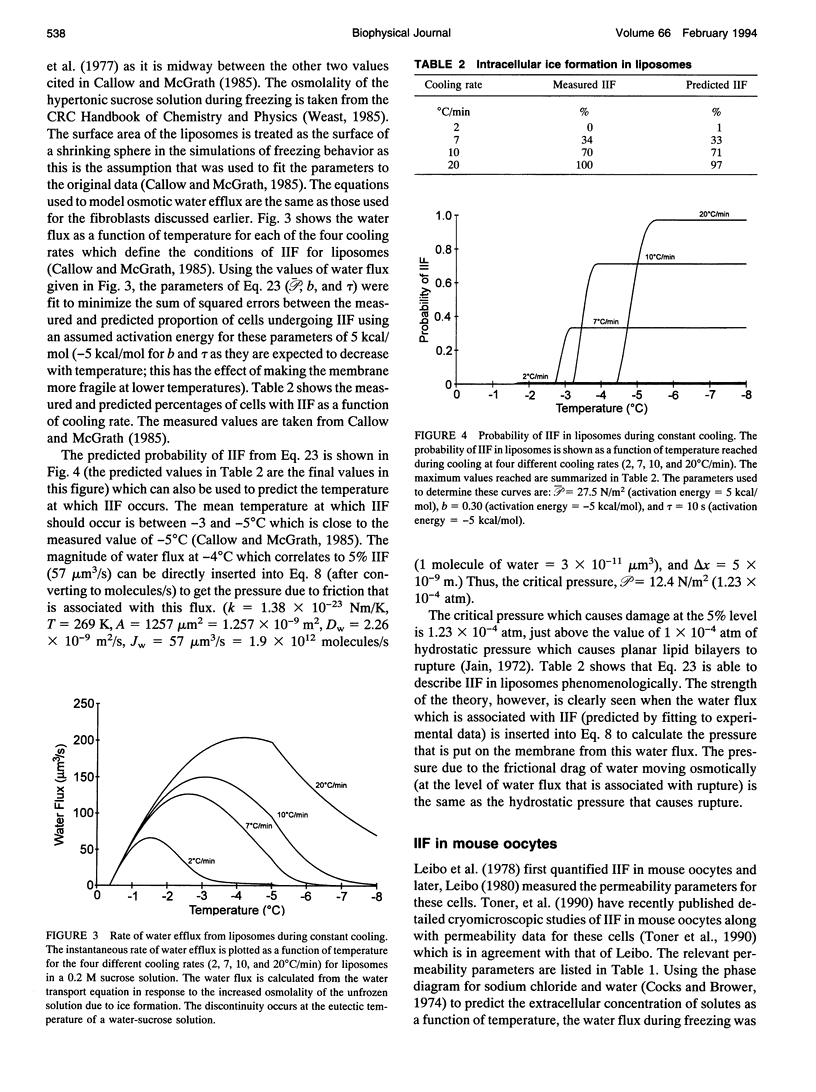
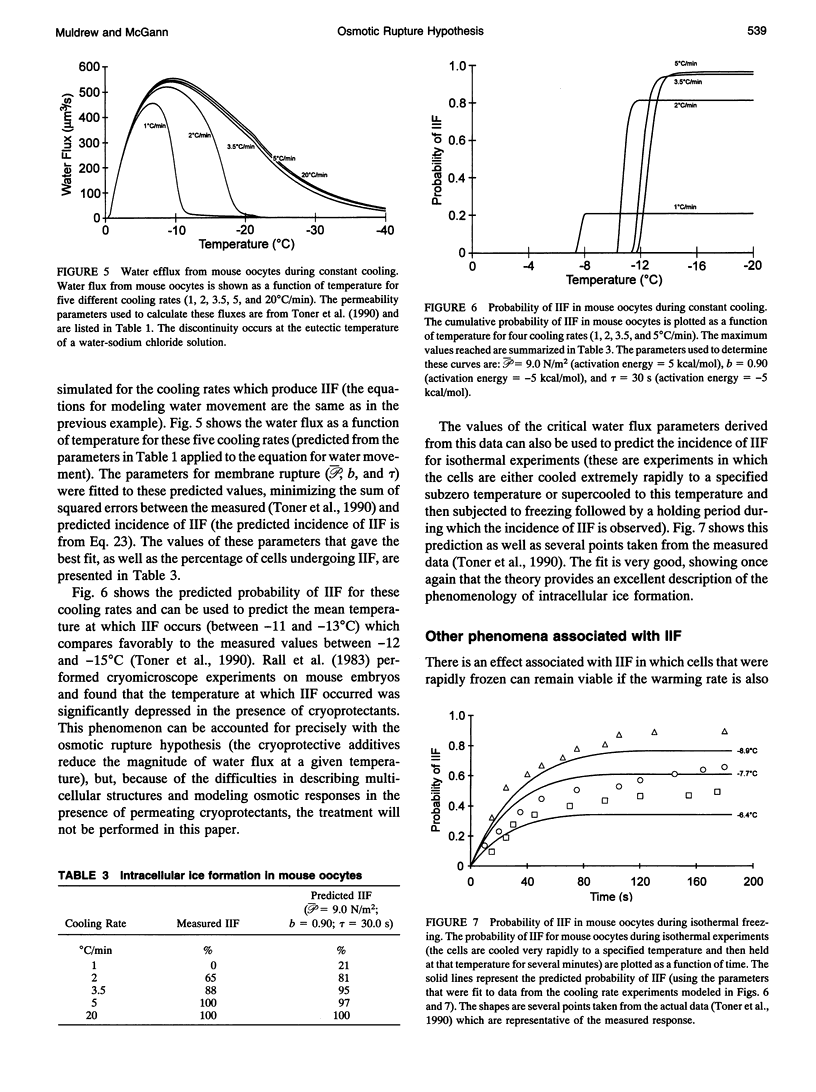
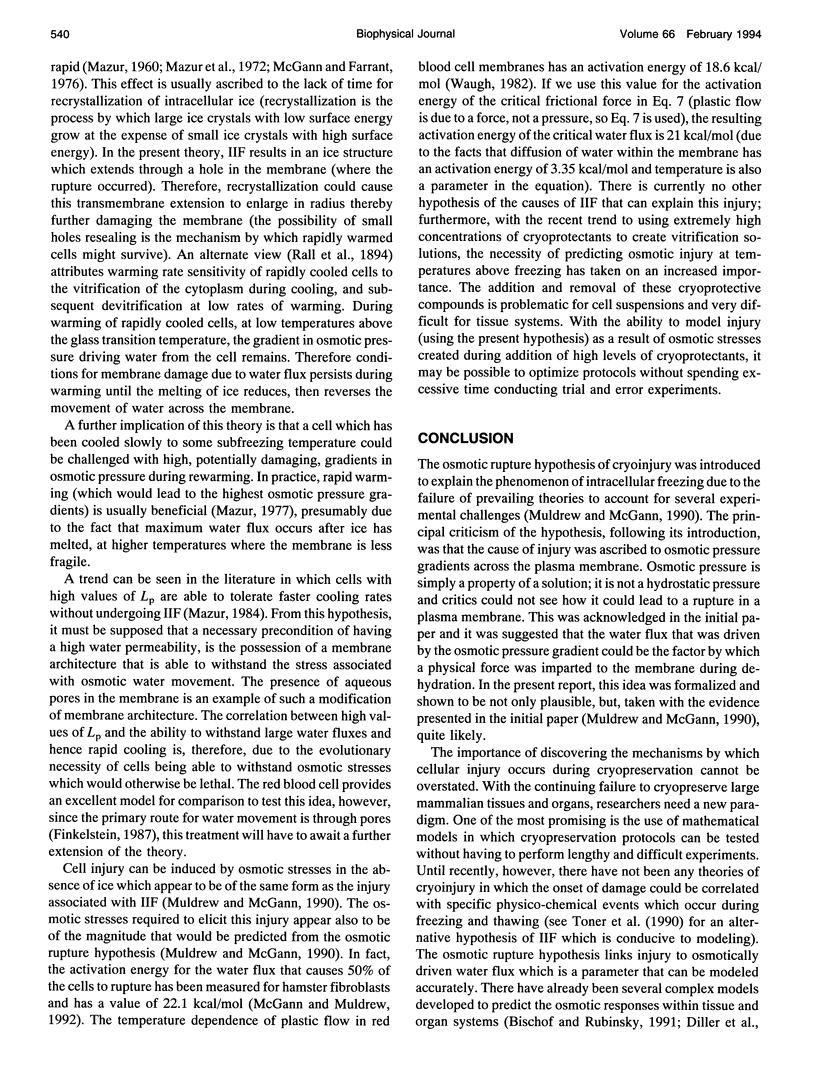
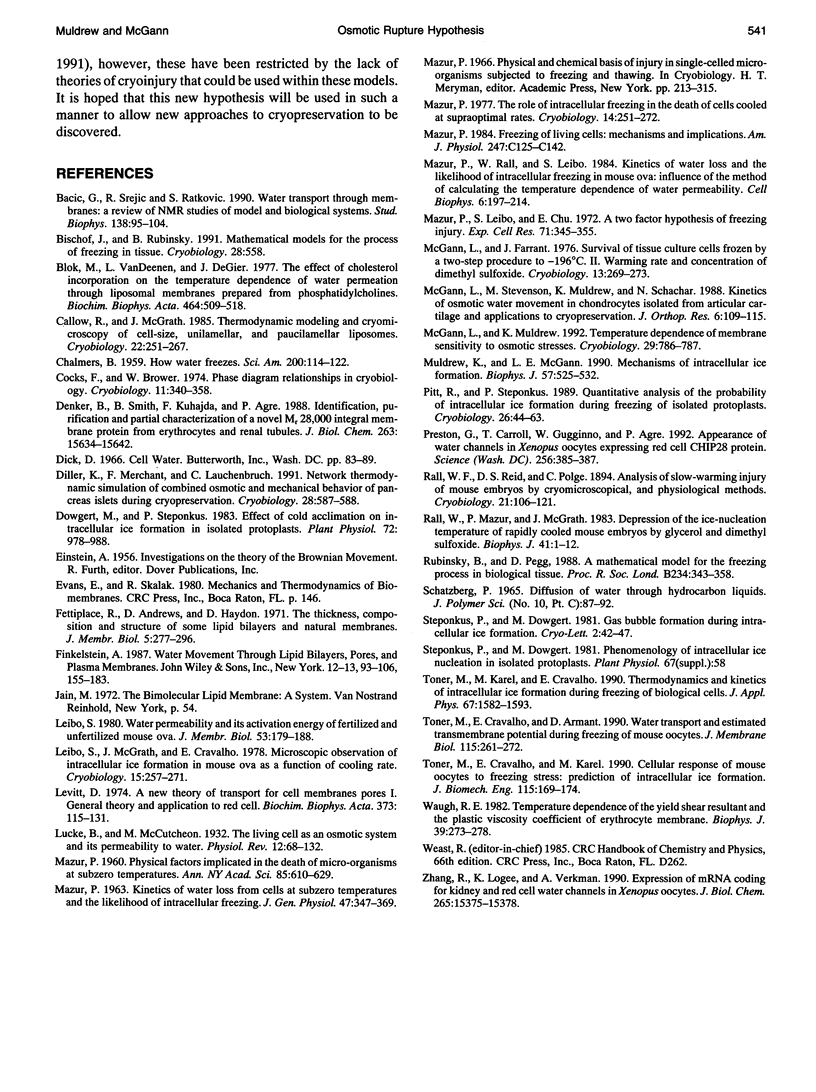
Selected References
These references are in PubMed. This may not be the complete list of references from this article.
- Blok M. C., Van Deenen L. L., De Gier J. The effect of cholesterol incorporation on the temperature dependence of water permeation through liposomal membranes prepared from phosphatidylcholines. Biochim Biophys Acta. 1977 Feb 4;464(3):509–518. doi: 10.1016/0005-2736(77)90026-8. [DOI] [PubMed] [Google Scholar]
- Callow R. A., McGrath J. J. Thermodynamic modeling and cryomicroscopy of cell-size, unilamellar, and paucilamellar liposomes. Cryobiology. 1985 Jun;22(3):251–267. doi: 10.1016/0011-2240(85)90146-4. [DOI] [PubMed] [Google Scholar]
- Cocks F. H., Brower W. E. Phase diagram relationships in cryobiology. Cryobiology. 1974 Aug;11(4):340–358. doi: 10.1016/0011-2240(74)90011-x. [DOI] [PubMed] [Google Scholar]
- Denker B. M., Smith B. L., Kuhajda F. P., Agre P. Identification, purification, and partial characterization of a novel Mr 28,000 integral membrane protein from erythrocytes and renal tubules. J Biol Chem. 1988 Oct 25;263(30):15634–15642. [PubMed] [Google Scholar]
- Dowgert M. F., Steponkus P. L. Effect of cold acclimation on intracellular ice formation in isolated protoplasts. Plant Physiol. 1983 Aug;72(4):978–988. doi: 10.1104/pp.72.4.978. [DOI] [PMC free article] [PubMed] [Google Scholar]
- Leibo S. P., McGrath J. J., Cravalho E. G. Microscopic observation of intracellular ice formation in unfertilized mouse ova as a function of cooling rate. Cryobiology. 1978 Jun;15(3):257–271. doi: 10.1016/0011-2240(78)90036-6. [DOI] [PubMed] [Google Scholar]
- Leibo S. P. Water permeability and its activation energy of fertilized and unfertilized mouse ova. J Membr Biol. 1980;53(3):179–188. doi: 10.1007/BF01868823. [DOI] [PubMed] [Google Scholar]
- Levitt D. G. A new theory of transport for cell membrane pores. I. General theory and application to red cell. Biochim Biophys Acta. 1974 Nov 27;373(1):115–131. doi: 10.1016/0005-2736(74)90111-4. [DOI] [PubMed] [Google Scholar]
- MAZUR P. KINETICS OF WATER LOSS FROM CELLS AT SUBZERO TEMPERATURES AND THE LIKELIHOOD OF INTRACELLULAR FREEZING. J Gen Physiol. 1963 Nov;47:347–369. doi: 10.1085/jgp.47.2.347. [DOI] [PMC free article] [PubMed] [Google Scholar]
- MAZUR P. Physical factors implicated in the death of microorganisms at subzero temperatures. Ann N Y Acad Sci. 1960 Apr 13;85:610–629. doi: 10.1111/j.1749-6632.1960.tb49986.x. [DOI] [PubMed] [Google Scholar]
- Mazur P. Freezing of living cells: mechanisms and implications. Am J Physiol. 1984 Sep;247(3 Pt 1):C125–C142. doi: 10.1152/ajpcell.1984.247.3.C125. [DOI] [PubMed] [Google Scholar]
- Mazur P., Leibo S. P., Chu E. H. A two-factor hypothesis of freezing injury. Evidence from Chinese hamster tissue-culture cells. Exp Cell Res. 1972;71(2):345–355. doi: 10.1016/0014-4827(72)90303-5. [DOI] [PubMed] [Google Scholar]
- Mazur P., Rall W. F., Leibo S. P. Kinetics of water loss and the likelihood of intracellular freezing in mouse ova. Influence of the method of calculating the temperature dependence of water permeability. Cell Biophys. 1984 Sep;6(3):197–213. doi: 10.1007/BF02788619. [DOI] [PubMed] [Google Scholar]
- Mazur P. The role of intracellular freezing in the death of cells cooled at supraoptimal rates. Cryobiology. 1977 Jun;14(3):251–272. doi: 10.1016/0011-2240(77)90175-4. [DOI] [PubMed] [Google Scholar]
- McGann L. E., Farrant J. Survival of tissue culture cells frozen by a two-step procedure to -196 degrees C. II. Warming rate and concentration of dimethyl sulphoxide. Cryobiology. 1976 Jun;13(3):269–273. doi: 10.1016/0011-2240(76)90107-3. [DOI] [PubMed] [Google Scholar]
- McGann L. E., Stevenson M., Muldrew K., Schachar N. Kinetics of osmotic water movement in chondrocytes isolated from articular cartilage and applications to cryopreservation. J Orthop Res. 1988;6(1):109–115. doi: 10.1002/jor.1100060114. [DOI] [PubMed] [Google Scholar]
- Muldrew K., McGann L. E. Mechanisms of intracellular ice formation. Biophys J. 1990 Mar;57(3):525–532. doi: 10.1016/S0006-3495(90)82568-6. [DOI] [PMC free article] [PubMed] [Google Scholar]
- Pitt R. E., Steponkus P. L. Quantitative analysis of the probability of intracellular ice formation during freezing of isolated protoplasts. Cryobiology. 1989 Feb;26(1):44–63. doi: 10.1016/0011-2240(89)90032-1. [DOI] [PubMed] [Google Scholar]
- Preston G. M., Carroll T. P., Guggino W. B., Agre P. Appearance of water channels in Xenopus oocytes expressing red cell CHIP28 protein. Science. 1992 Apr 17;256(5055):385–387. doi: 10.1126/science.256.5055.385. [DOI] [PubMed] [Google Scholar]
- Rall W. F., Mazur P., McGrath J. J. Depression of the ice-nucleation temperature of rapidly cooled mouse embryos by glycerol and dimethyl sulfoxide. Biophys J. 1983 Jan;41(1):1–12. doi: 10.1016/S0006-3495(83)84399-9. [DOI] [PMC free article] [PubMed] [Google Scholar]
- Rall W. F., Reid D. S., Polge C. Analysis of slow-warming injury of mouse embryos by cryomicroscopical and physiochemical methods. Cryobiology. 1984 Feb;21(1):106–121. doi: 10.1016/0011-2240(84)90027-0. [DOI] [PubMed] [Google Scholar]
- Rubinsky B., Pegg D. E. A mathematical model for the freezing process in biological tissue. Proc R Soc Lond B Biol Sci. 1988 Aug 23;234(1276):343–358. doi: 10.1098/rspb.1988.0053. [DOI] [PubMed] [Google Scholar]
- Toner M., Cravalho E. G., Armant D. R. Water transport and estimated transmembrane potential during freezing of mouse oocytes. J Membr Biol. 1990 May;115(3):261–272. doi: 10.1007/BF01868641. [DOI] [PubMed] [Google Scholar]
- Toner M., Cravalho E. G., Karel M. Cellular response of mouse oocytes to freezing stress: prediction of intracellular ice formation. J Biomech Eng. 1993 May;115(2):169–174. doi: 10.1115/1.2894117. [DOI] [PubMed] [Google Scholar]
- Waugh R. E. Temperature dependence of the yield shear resultant and the plastic viscosity coefficient of erythrocyte membrane. Implications about molecular events during membrane failure. Biophys J. 1982 Sep;39(3):273–278. doi: 10.1016/S0006-3495(82)84517-7. [DOI] [PMC free article] [PubMed] [Google Scholar]
- Zhang R. B., Logee K. A., Verkman A. S. Expression of mRNA coding for kidney and red cell water channels in Xenopus oocytes. J Biol Chem. 1990 Sep 15;265(26):15375–15378. [PubMed] [Google Scholar]