Abstract
The behavior of a multiply-occupied cation-selective channel has been computed by Brownian dynamics. The length, cross-section, ion-ion repulsion force, and ionic mobility within the channel are all estimated from data and physical reasoning. The only free parameter is a partition energy at the mouth of the channel, defining the free energy of an ion in the channel compared to the bath. It is presumed that this partition energy is associated with the energetics of exchanging a bulk hydration environment for a channel hydration environment. Varying the partition energy alone, keeping all other parameters fixed, gives approximately the full range of magnitudes of single channel conductances seen experimentally for K channels. Setting the partition energy at -11 kT makes the computed channel look similar to a squid axon K channel with respect to magnitude of conductance, shape of the I-V curve, non-unity of Ussing flux ratio exponents, decrease of current and increase of conductance with extracellular ion accumulation, and saturation at high ion concentration in the bathing solution. The model includes no preferred binding sites (local free energy minima) for ions in the channel. Therefore it follows that none of the above-mentioned properties of K channels are strong evidence for the existence of such sites. The model does not show supersaturation of current at very high bathing concentrations nor any pronounced voltage-dependence of the Ussing flux ratio exponent, suggesting that these features would require additional details not included in the model presented herein.
Full text
PDF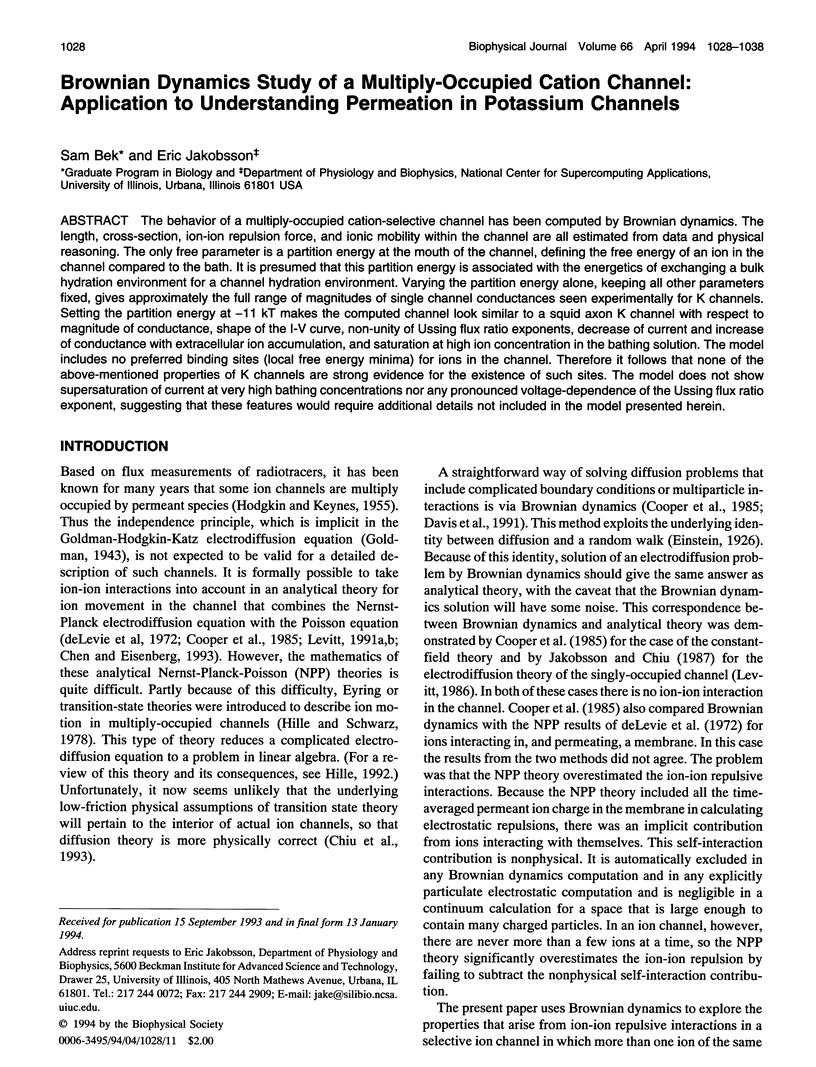
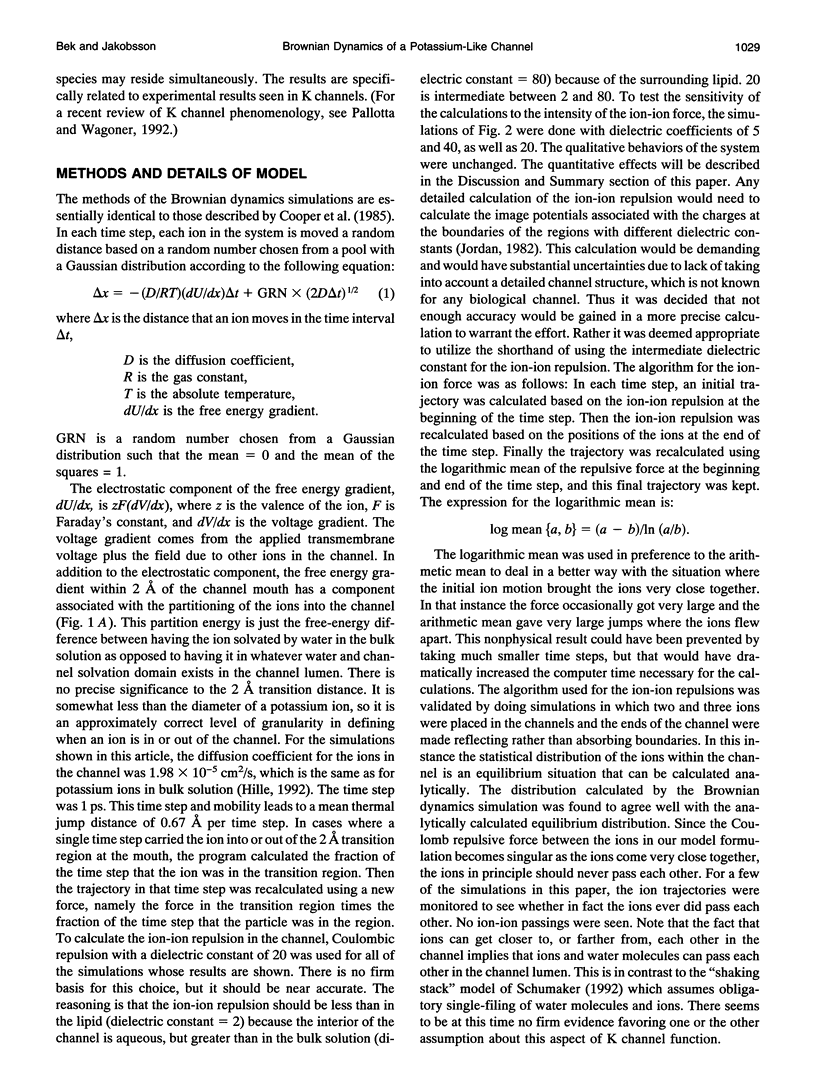
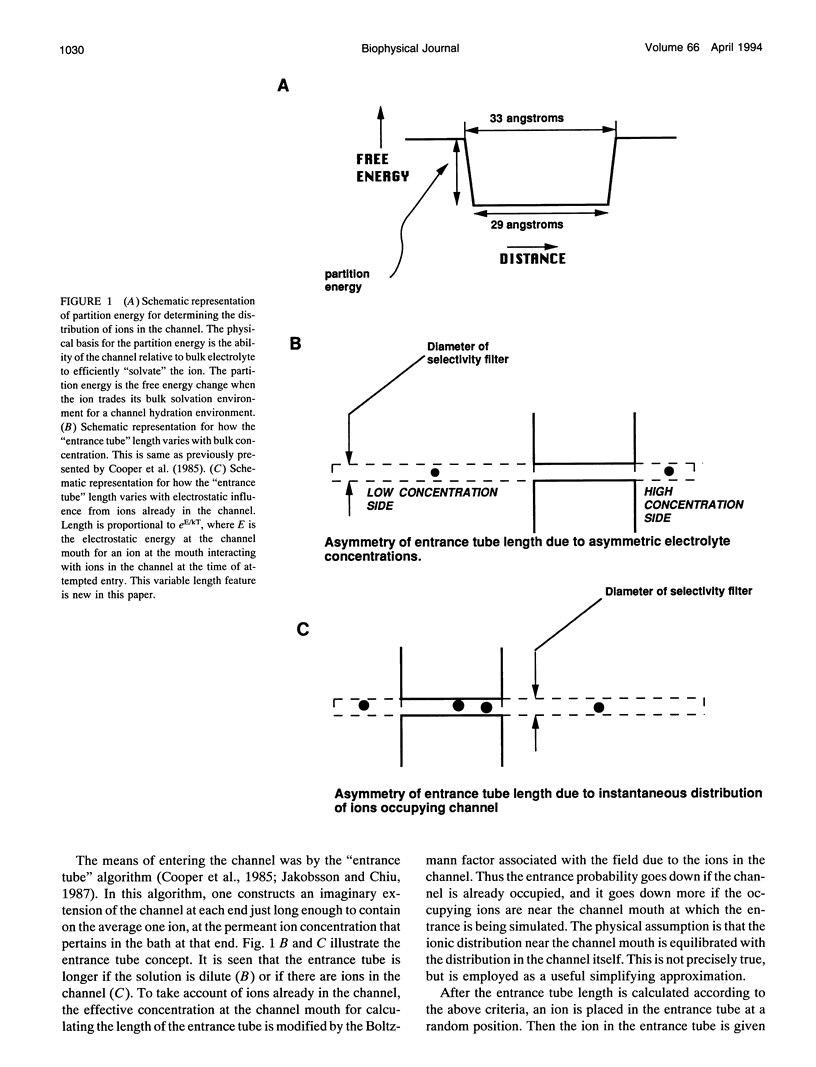
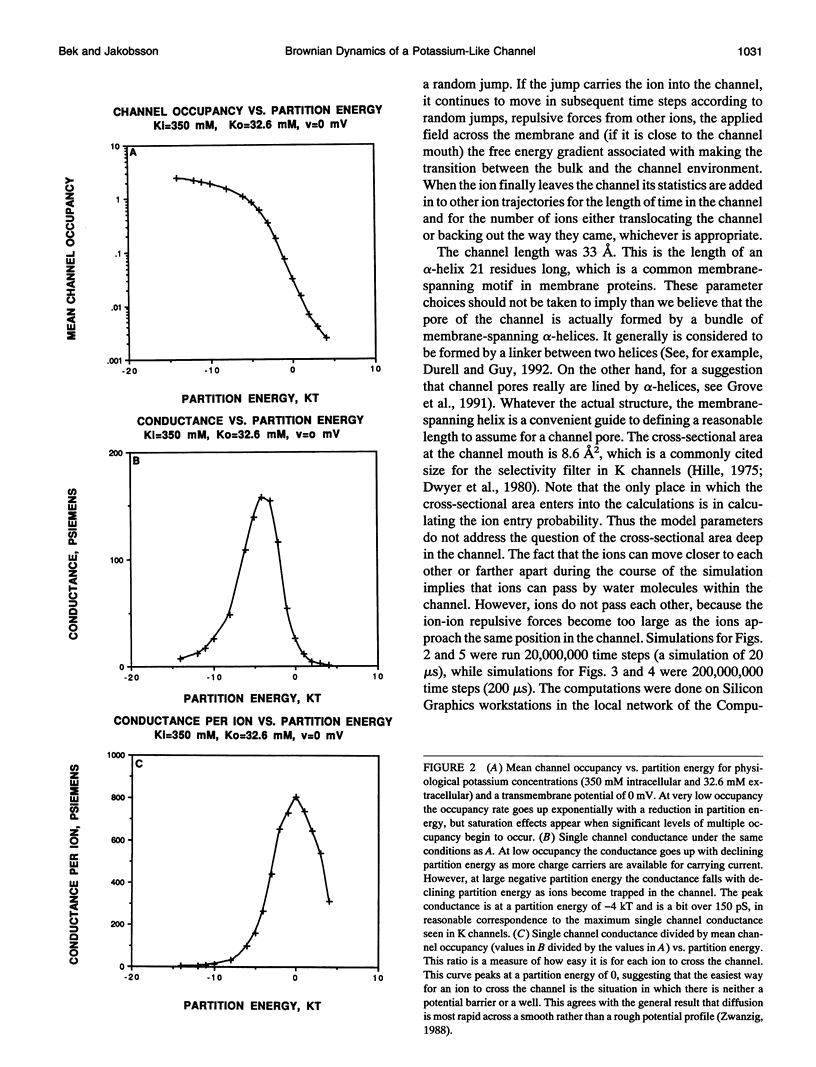
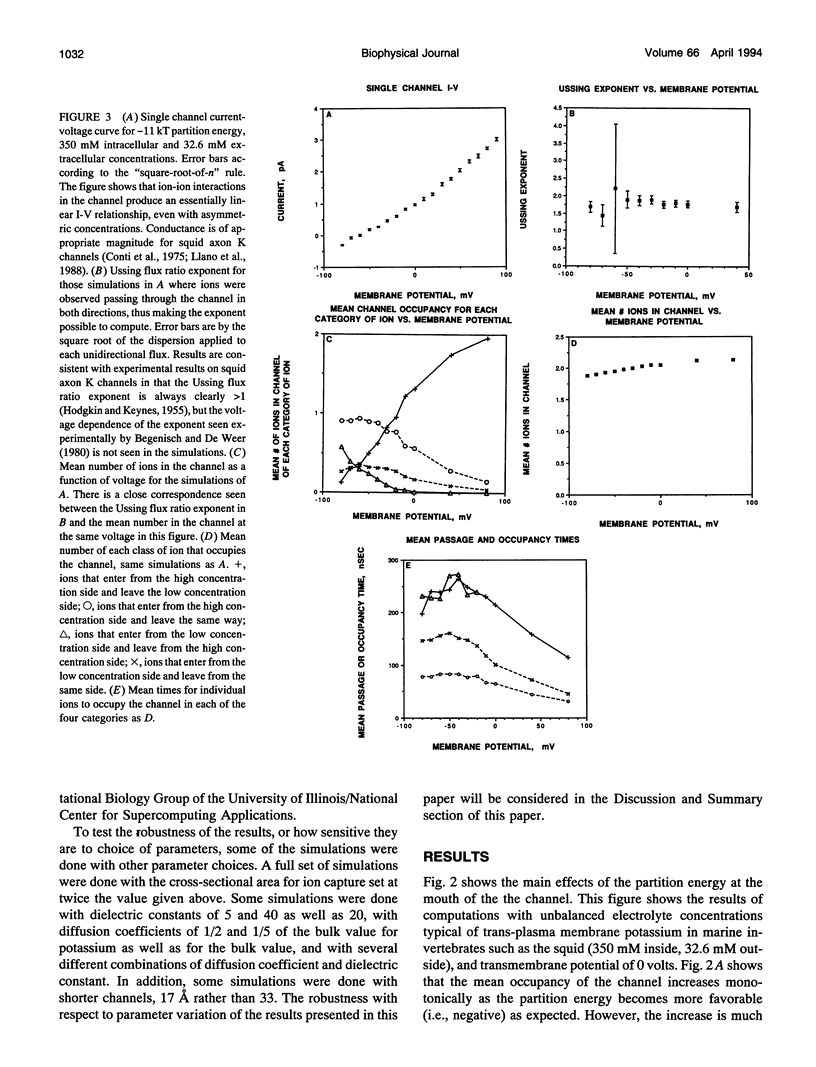
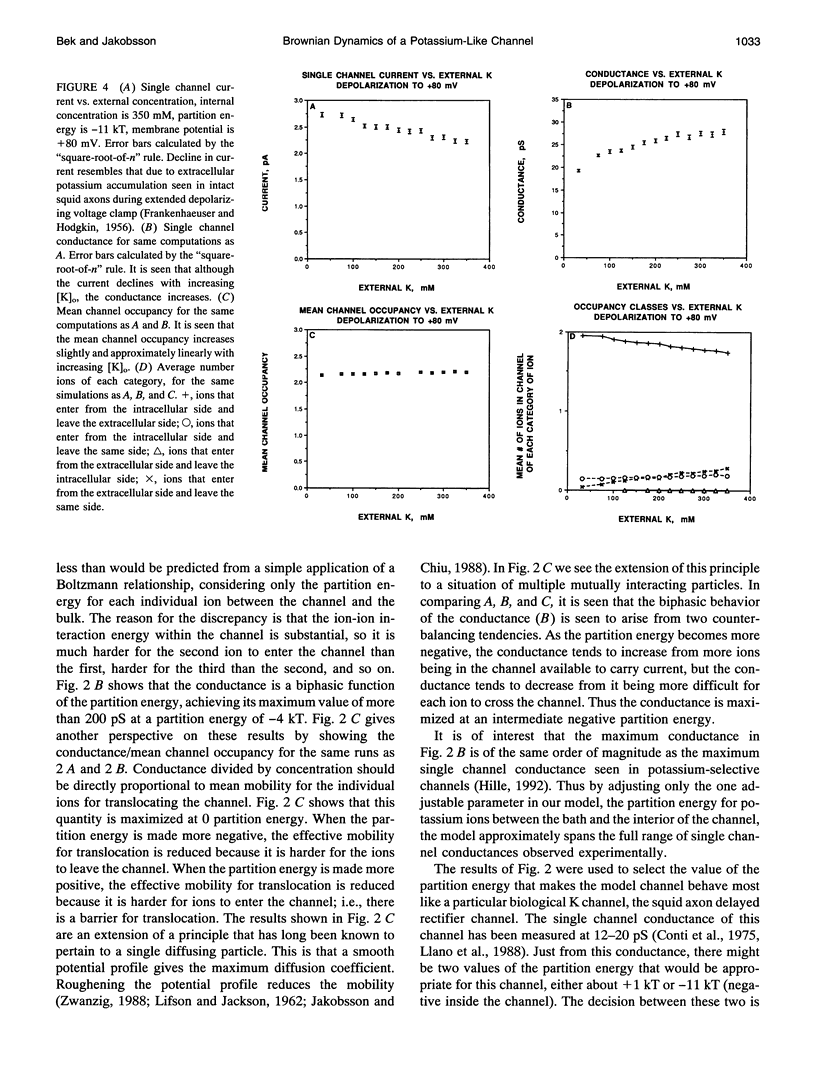
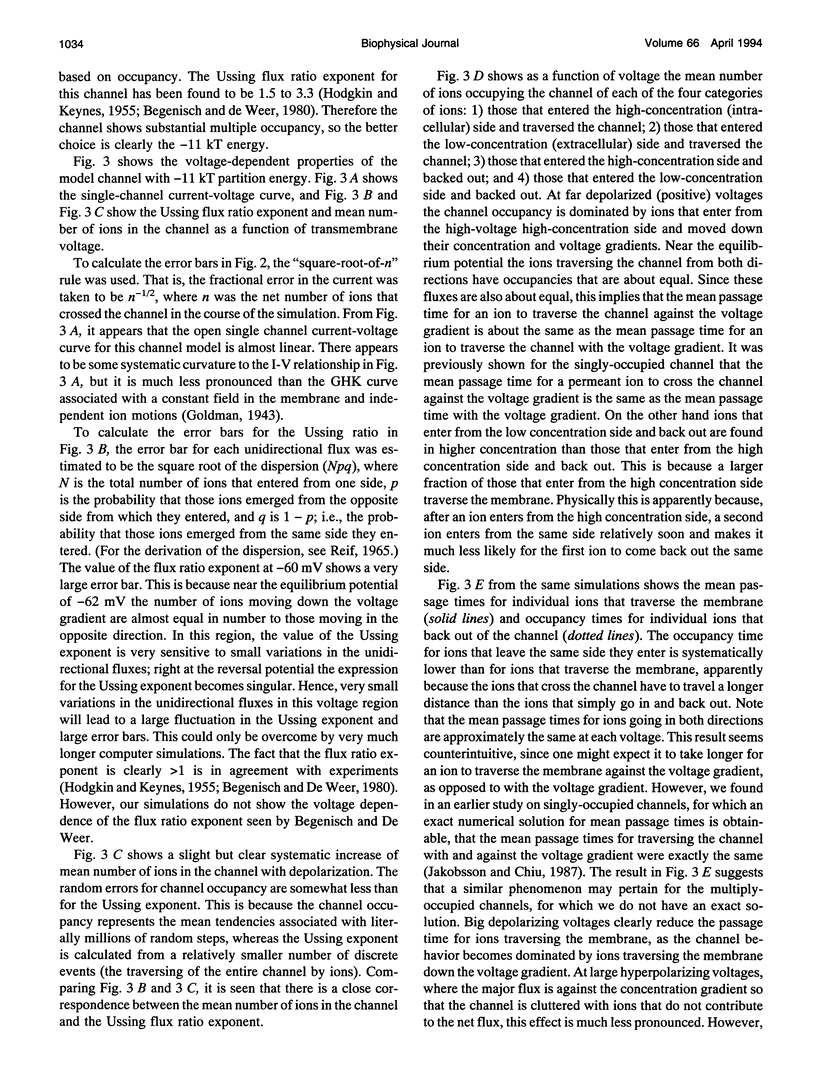
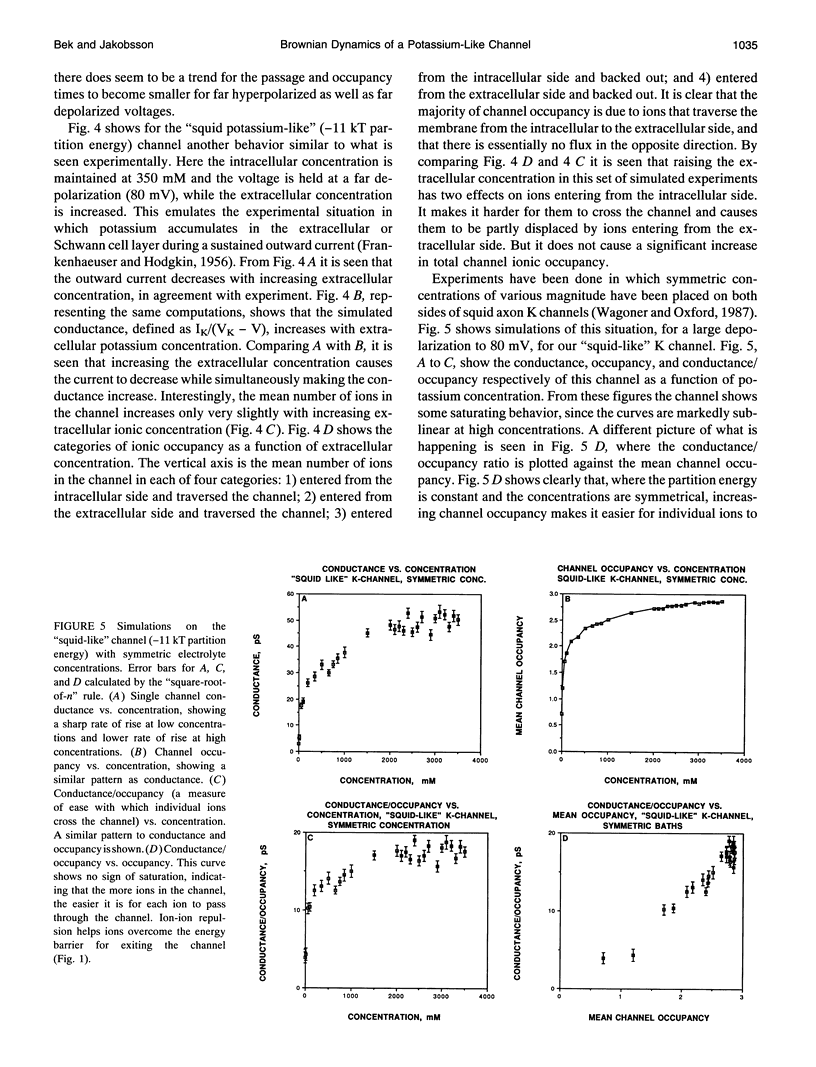
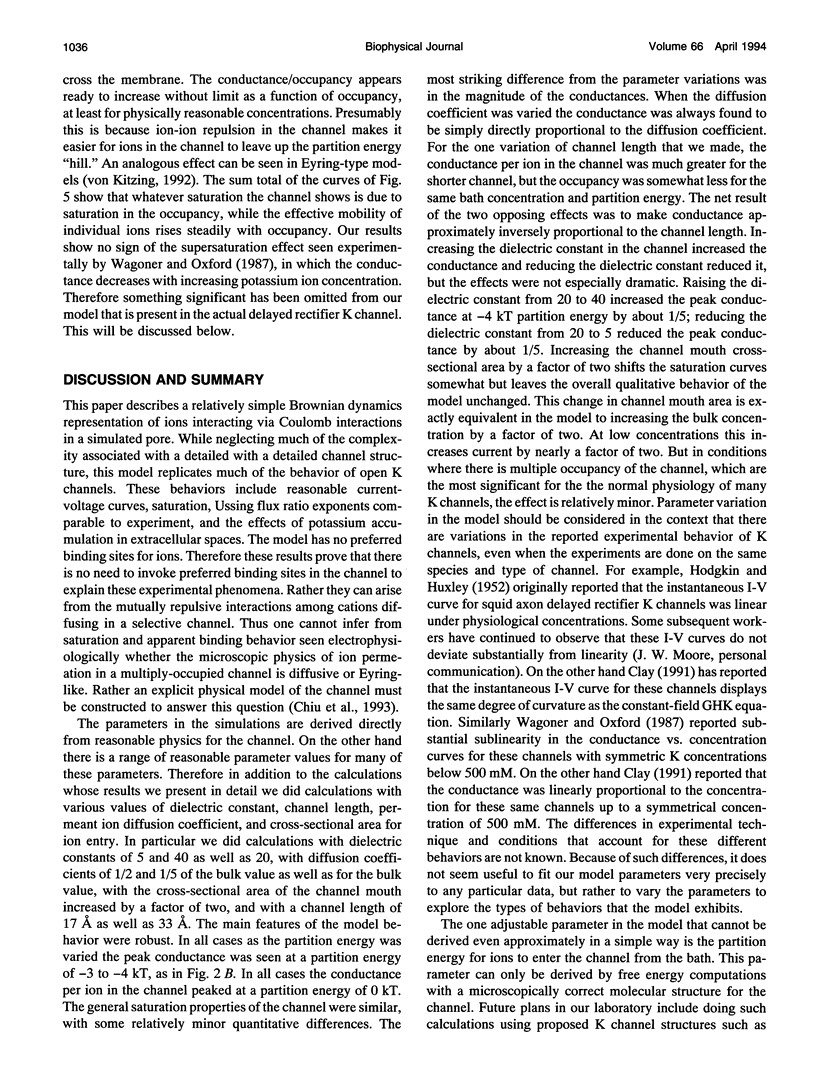
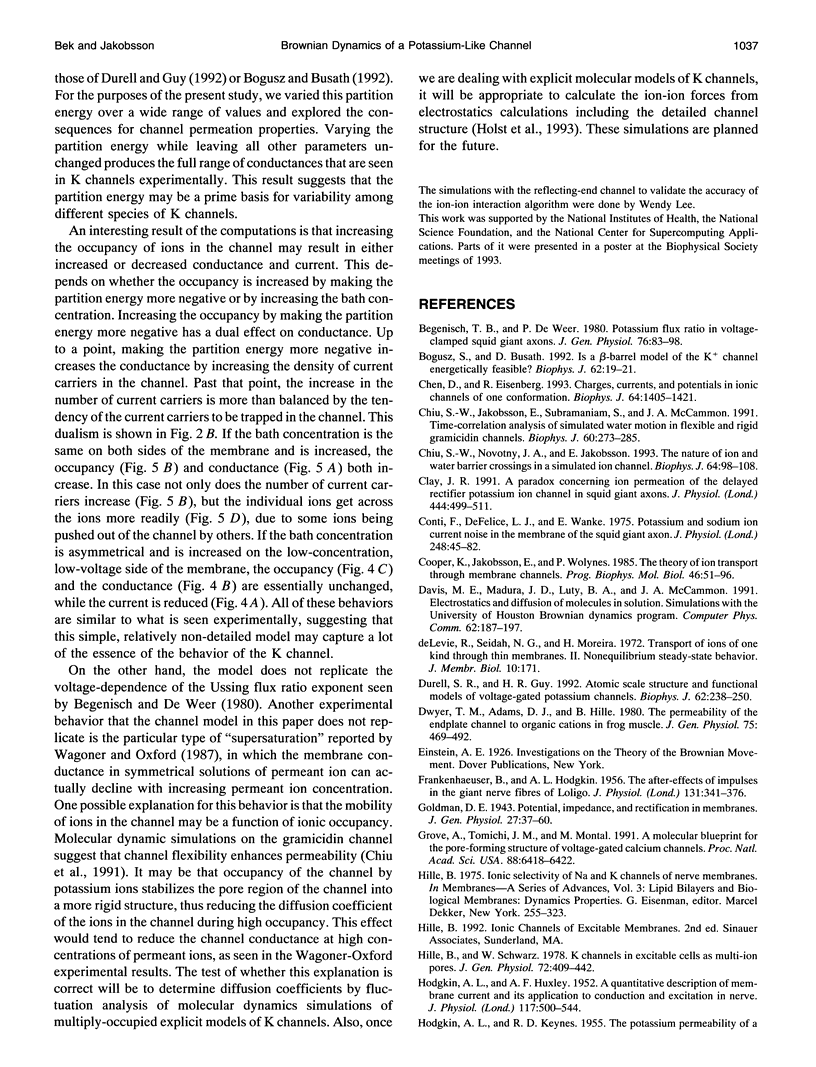
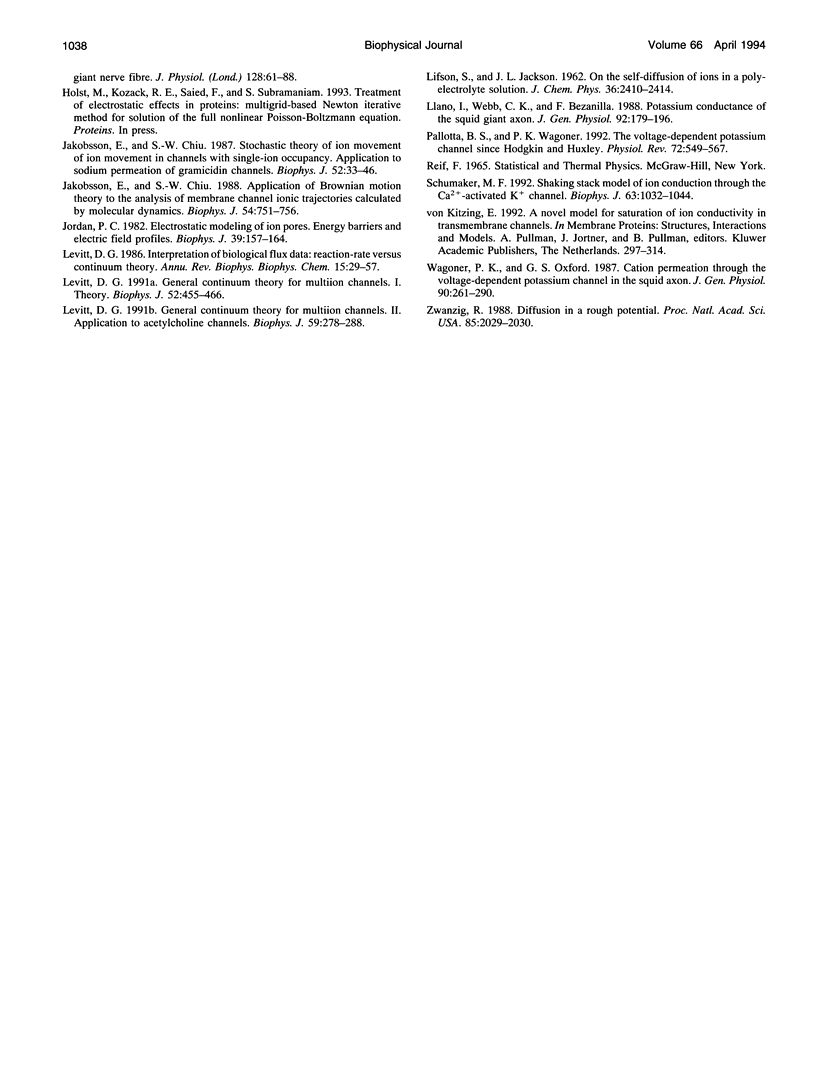
Selected References
These references are in PubMed. This may not be the complete list of references from this article.
- Begenisich T., De Weer P. Potassium flux ratio in voltage-clamped squid giant axons. J Gen Physiol. 1980 Jul;76(1):83–98. doi: 10.1085/jgp.76.1.83. [DOI] [PMC free article] [PubMed] [Google Scholar]
- Bogusz S., Busath D. Is a beta-barrel model of the K+ channel energetically feasible? Biophys J. 1992 Apr;62(1):19–21. doi: 10.1016/S0006-3495(92)81765-4. [DOI] [PMC free article] [PubMed] [Google Scholar]
- Chen D., Eisenberg R. Charges, currents, and potentials in ionic channels of one conformation. Biophys J. 1993 May;64(5):1405–1421. doi: 10.1016/S0006-3495(93)81507-8. [DOI] [PMC free article] [PubMed] [Google Scholar]
- Chiu S. W., Jakobsson E., Subramaniam S., McCammon J. A. Time-correlation analysis of simulated water motion in flexible and rigid gramicidin channels. Biophys J. 1991 Jul;60(1):273–285. doi: 10.1016/S0006-3495(91)82049-5. [DOI] [PMC free article] [PubMed] [Google Scholar]
- Chiu S. W., Novotny J. A., Jakobsson E. The nature of ion and water barrier crossings in a simulated ion channel. Biophys J. 1993 Jan;64(1):98–109. doi: 10.1016/S0006-3495(93)81344-4. [DOI] [PMC free article] [PubMed] [Google Scholar]
- Clay J. R. A paradox concerning ion permeation of the delayed rectifier potassium ion channel in squid giant axons. J Physiol. 1991 Dec;444:499–511. doi: 10.1113/jphysiol.1991.sp018890. [DOI] [PMC free article] [PubMed] [Google Scholar]
- Conti F., De Felice L. J., Wanke E. Potassium and sodium ion current noise in the membrane of the squid giant axon. J Physiol. 1975 Jun;248(1):45–82. doi: 10.1113/jphysiol.1975.sp010962. [DOI] [PMC free article] [PubMed] [Google Scholar]
- Cooper K., Jakobsson E., Wolynes P. The theory of ion transport through membrane channels. Prog Biophys Mol Biol. 1985;46(1):51–96. doi: 10.1016/0079-6107(85)90012-4. [DOI] [PubMed] [Google Scholar]
- De Levie R., Seidah N. G., Moreira H. Transport of ions of one kind through thin membranes. II. Nonequilibrium steady-state behavior. J Membr Biol. 1972;10(2):171–192. doi: 10.1007/BF01867852. [DOI] [PubMed] [Google Scholar]
- Durell S. R., Guy H. R. Atomic scale structure and functional models of voltage-gated potassium channels. Biophys J. 1992 Apr;62(1):238–250. doi: 10.1016/S0006-3495(92)81809-X. [DOI] [PMC free article] [PubMed] [Google Scholar]
- Dwyer T. M., Adams D. J., Hille B. The permeability of the endplate channel to organic cations in frog muscle. J Gen Physiol. 1980 May;75(5):469–492. doi: 10.1085/jgp.75.5.469. [DOI] [PMC free article] [PubMed] [Google Scholar]
- FRANKENHAEUSER B., HODGKIN A. L. The after-effects of impulses in the giant nerve fibres of Loligo. J Physiol. 1956 Feb 28;131(2):341–376. doi: 10.1113/jphysiol.1956.sp005467. [DOI] [PMC free article] [PubMed] [Google Scholar]
- Goldman D. E. POTENTIAL, IMPEDANCE, AND RECTIFICATION IN MEMBRANES. J Gen Physiol. 1943 Sep 20;27(1):37–60. doi: 10.1085/jgp.27.1.37. [DOI] [PMC free article] [PubMed] [Google Scholar]
- Grove A., Tomich J. M., Montal M. A molecular blueprint for the pore-forming structure of voltage-gated calcium channels. Proc Natl Acad Sci U S A. 1991 Aug 1;88(15):6418–6422. doi: 10.1073/pnas.88.15.6418. [DOI] [PMC free article] [PubMed] [Google Scholar]
- HODGKIN A. L., HUXLEY A. F. A quantitative description of membrane current and its application to conduction and excitation in nerve. J Physiol. 1952 Aug;117(4):500–544. doi: 10.1113/jphysiol.1952.sp004764. [DOI] [PMC free article] [PubMed] [Google Scholar]
- HODGKIN A. L., KEYNES R. D. The potassium permeability of a giant nerve fibre. J Physiol. 1955 Apr 28;128(1):61–88. doi: 10.1113/jphysiol.1955.sp005291. [DOI] [PMC free article] [PubMed] [Google Scholar]
- Hille B., Schwarz W. Potassium channels as multi-ion single-file pores. J Gen Physiol. 1978 Oct;72(4):409–442. doi: 10.1085/jgp.72.4.409. [DOI] [PMC free article] [PubMed] [Google Scholar]
- Jakobsson E., Chiu S. W. Application of Brownian motion theory to the analysis of membrane channel ionic trajectories calculated by molecular dynamics. Biophys J. 1988 Oct;54(4):751–756. doi: 10.1016/S0006-3495(88)83012-1. [DOI] [PMC free article] [PubMed] [Google Scholar]
- Jakobsson E., Chiu S. W. Stochastic theory of ion movement in channels with single-ion occupancy. Application to sodium permeation of gramicidin channels. Biophys J. 1987 Jul;52(1):33–45. doi: 10.1016/S0006-3495(87)83186-7. [DOI] [PMC free article] [PubMed] [Google Scholar]
- Jordan P. C. Electrostatic modeling of ion pores. Energy barriers and electric field profiles. Biophys J. 1982 Aug;39(2):157–164. doi: 10.1016/S0006-3495(82)84503-7. [DOI] [PMC free article] [PubMed] [Google Scholar]
- Levitt D. G. General continuum theory for multiion channel. II. Application to acetylcholine channel. Biophys J. 1991 Feb;59(2):278–288. doi: 10.1016/S0006-3495(91)82221-4. [DOI] [PMC free article] [PubMed] [Google Scholar]
- Levitt D. G. Interpretation of biological ion channel flux data--reaction-rate versus continuum theory. Annu Rev Biophys Biophys Chem. 1986;15:29–57. doi: 10.1146/annurev.bb.15.060186.000333. [DOI] [PubMed] [Google Scholar]
- Llano I., Webb C. K., Bezanilla F. Potassium conductance of the squid giant axon. Single-channel studies. J Gen Physiol. 1988 Aug;92(2):179–196. doi: 10.1085/jgp.92.2.179. [DOI] [PMC free article] [PubMed] [Google Scholar]
- Schumaker M. F. Shaking stack model of ion conduction through the Ca(2+)-activated K+ channel. Biophys J. 1992 Oct;63(4):1032–1044. doi: 10.1016/S0006-3495(92)81668-5. [DOI] [PMC free article] [PubMed] [Google Scholar]
- Wagoner P. K., Oxford G. S. Cation permeation through the voltage-dependent potassium channel in the squid axon. Characteristics and mechanisms. J Gen Physiol. 1987 Aug;90(2):261–290. doi: 10.1085/jgp.90.2.261. [DOI] [PMC free article] [PubMed] [Google Scholar]
- Zwanzig R. Diffusion in a rough potential. Proc Natl Acad Sci U S A. 1988 Apr;85(7):2029–2030. doi: 10.1073/pnas.85.7.2029. [DOI] [PMC free article] [PubMed] [Google Scholar]