Abstract
Fixed spherical swollen human red blood cells of blood type B adhering on a glass surface through antigen-antibody bonds to monoclonal mouse antihuman IgM, adsorbed or covalently linked on the surface, were detached by known hydrodynamic forces created in an impinging jet. The dynamic process of detachment of the specifically bound cells was recorded and analyzed. The fraction of adherent cells remaining on the surface decreased with increasing hydrodynamic force. For an IgM coverage of 0.26%, a tangential force on the order of 100 pN was able to detach almost all of the cells from the surface within 20 min. After a given time of exposure to hydrodynamic force, the fraction of adherent cells remaining increased with time, reflecting an increase in adhesion strength. The characteristic time for effective aging was approximately 4 h. Results from experiments in which the adsorbed antibody molecules were immobilized through covalent coupling and from evanescent wave light scattering of adherent cells, imply that deformation of red cells at the contact area was the principal cause for aging, rather than local clustering of the antibody through surface diffusion. Experiments with latex beads specifically bound to red blood cells suggest that, instead of breaking the antigen-antibody bonds, antigen molecules were extracted from the cell membrane during detachment.
Full text
PDF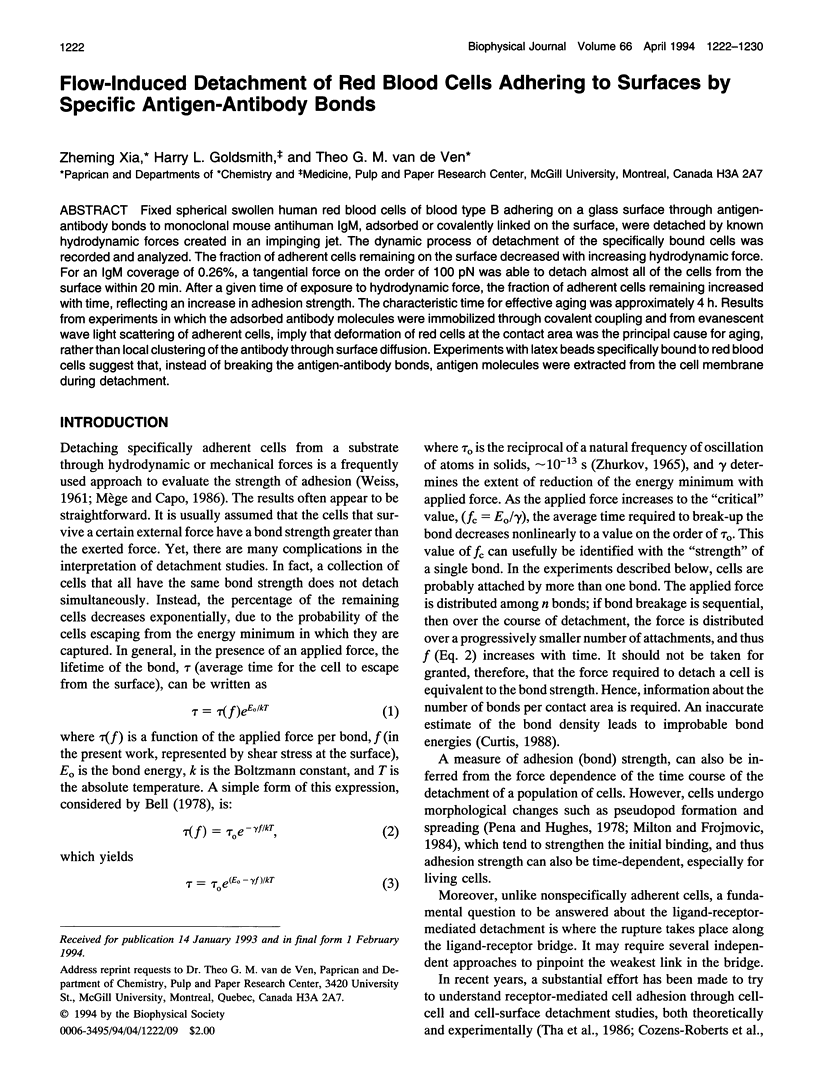
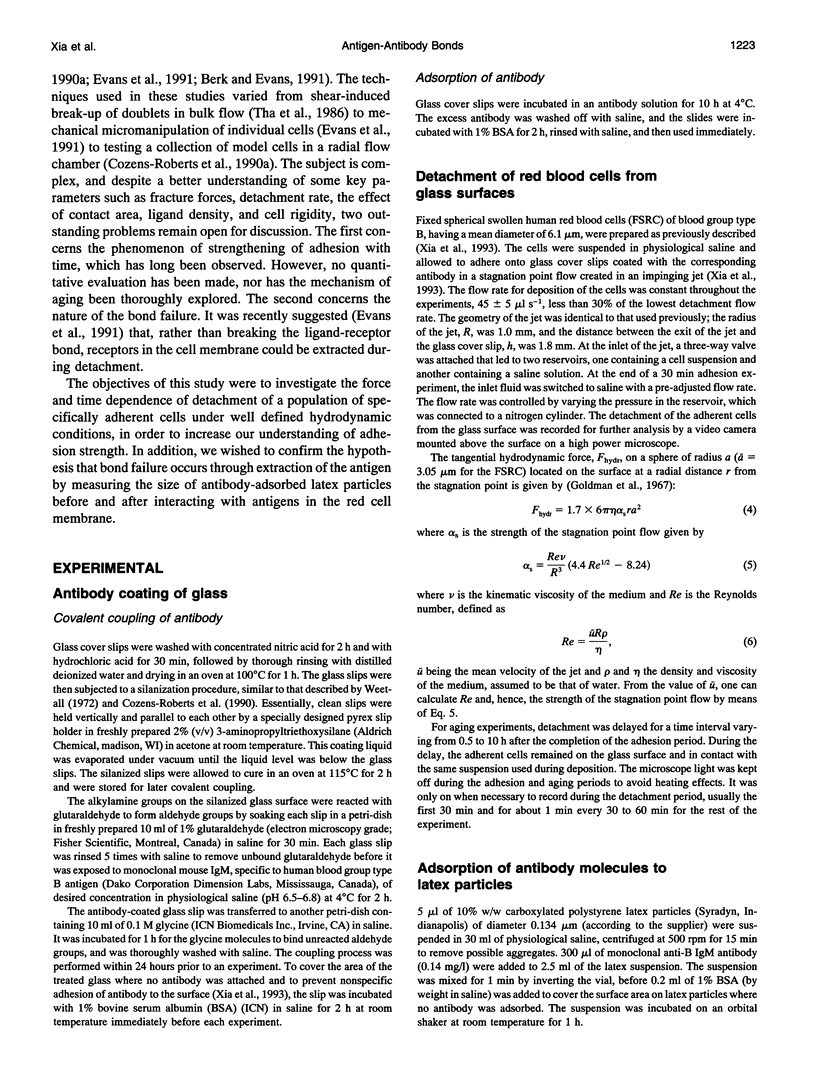
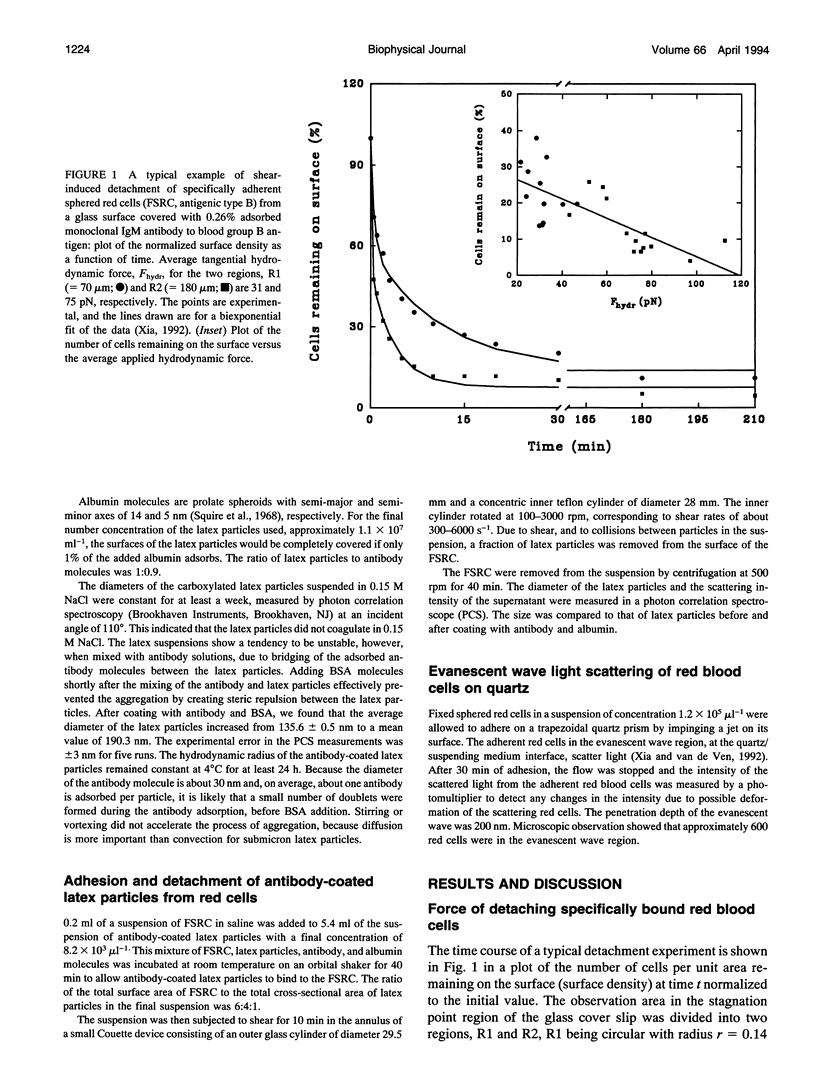
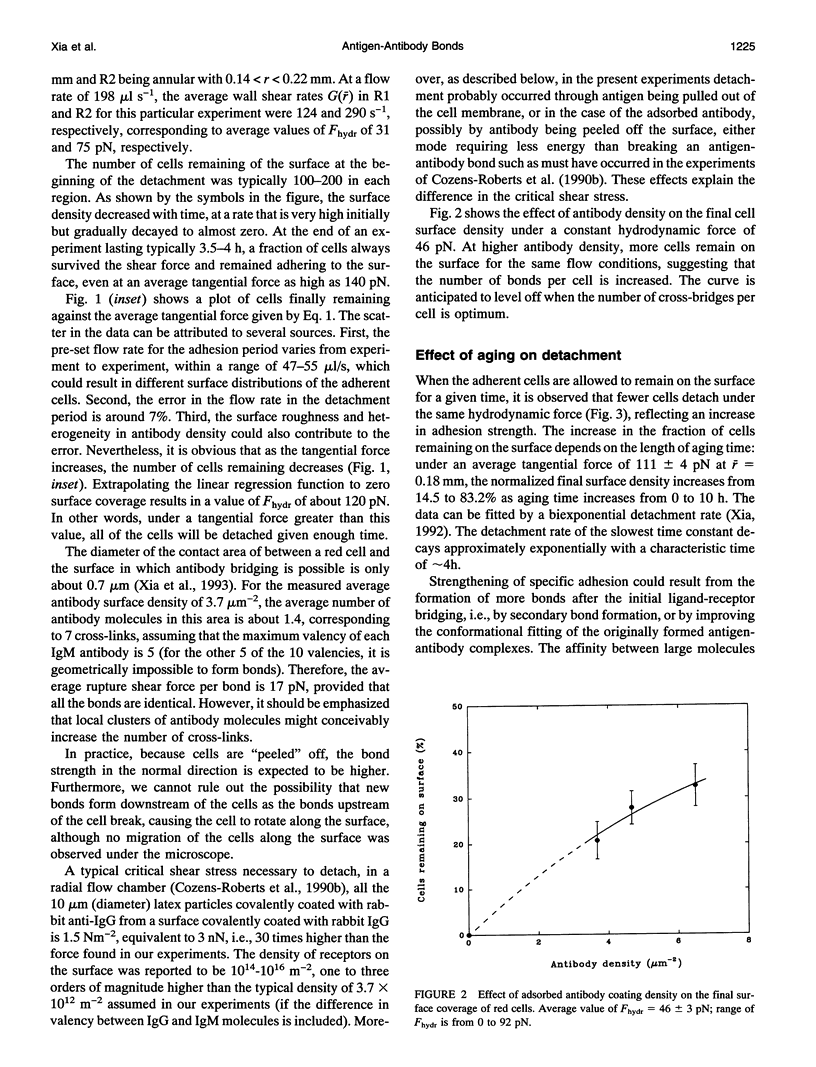
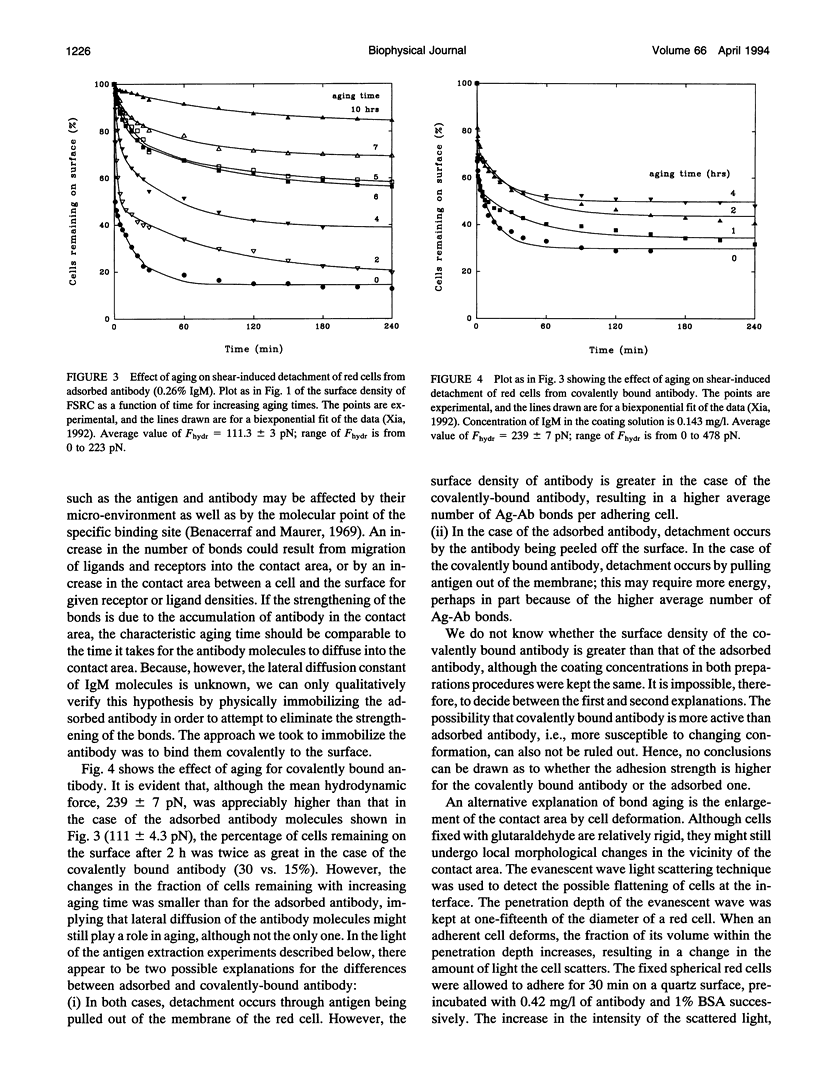
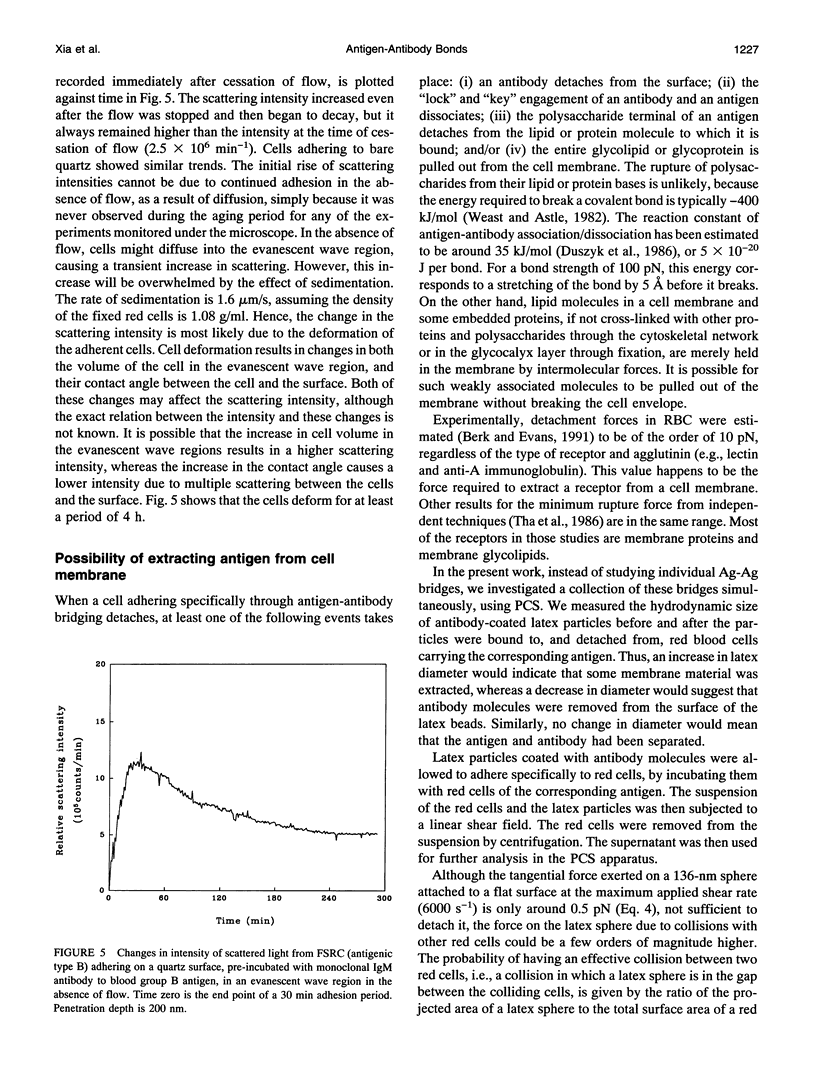
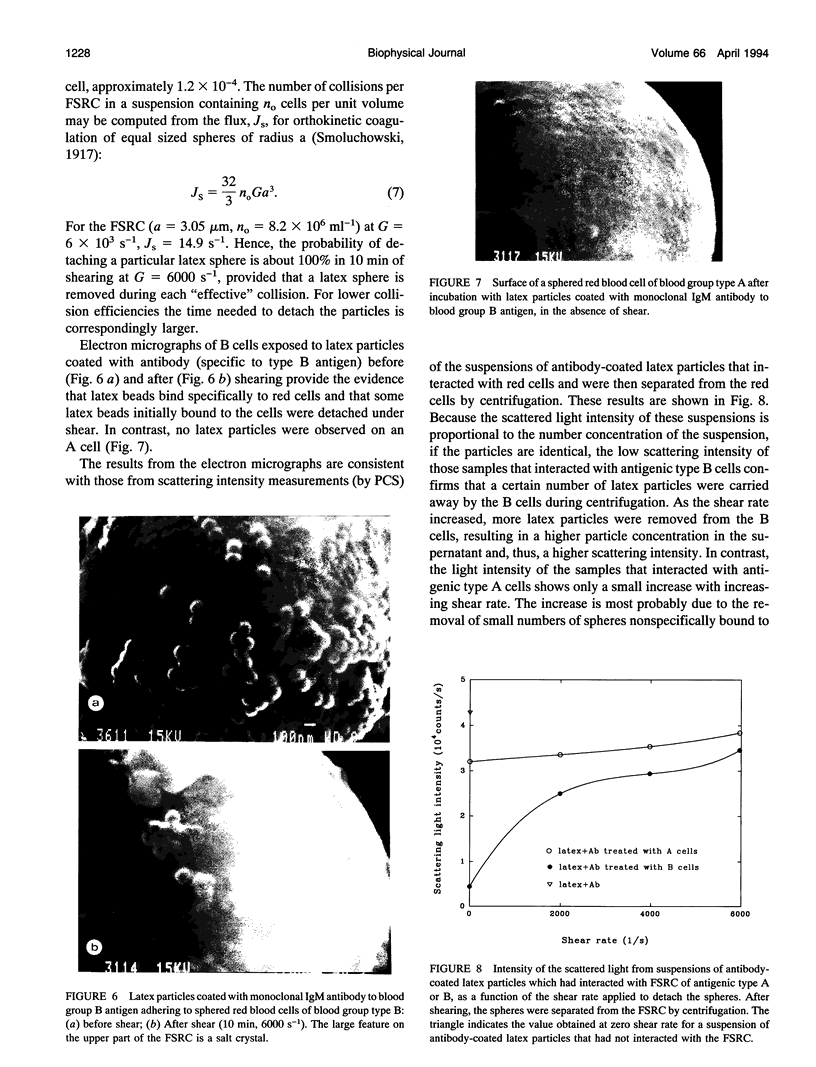
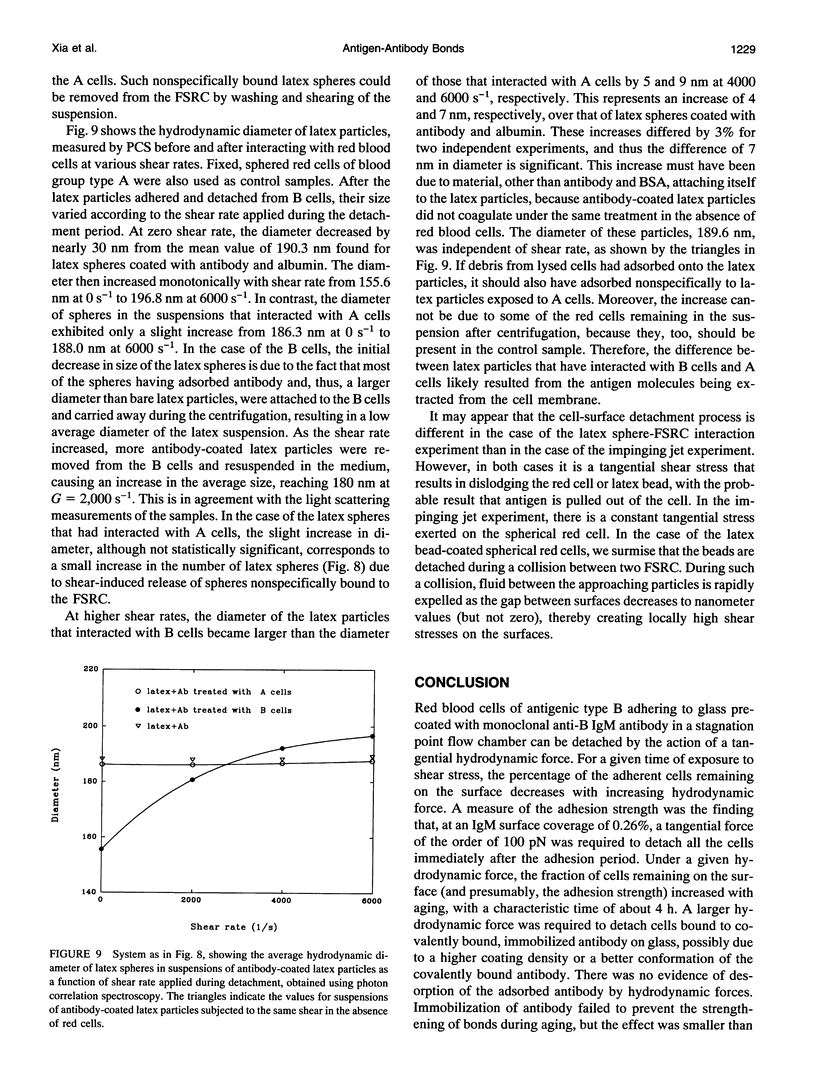
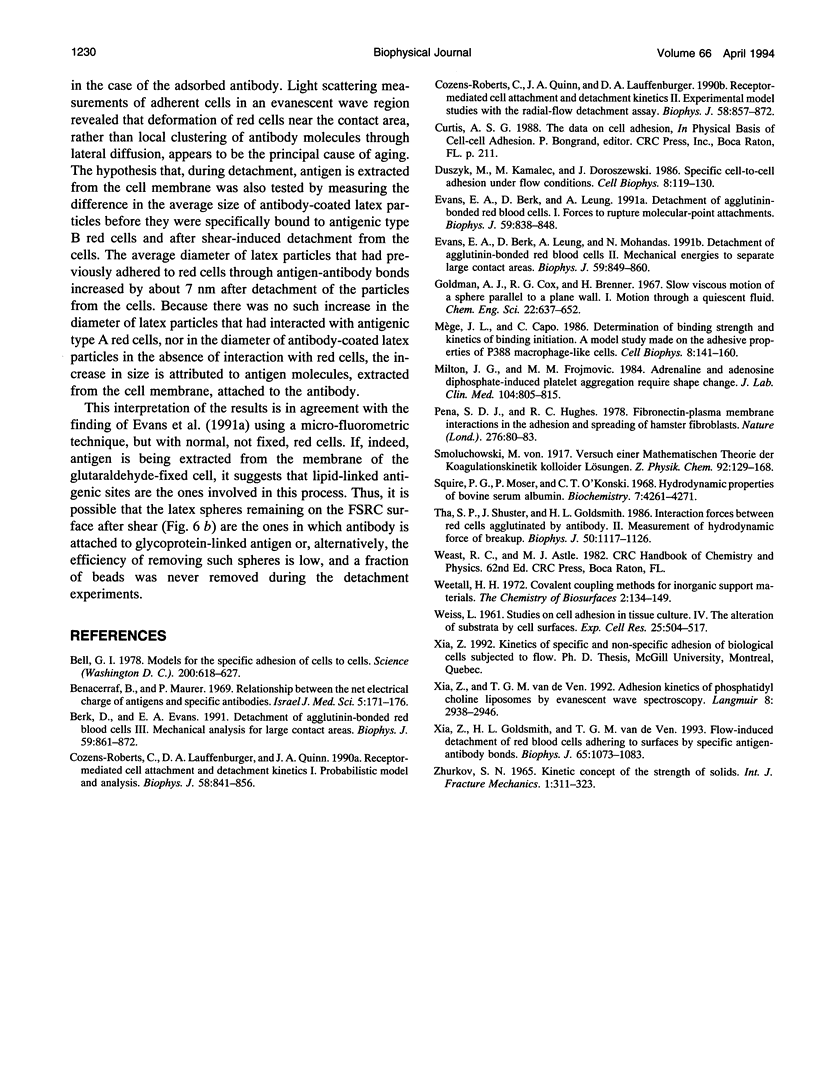
Images in this article
Selected References
These references are in PubMed. This may not be the complete list of references from this article.
- Bell G. I. Models for the specific adhesion of cells to cells. Science. 1978 May 12;200(4342):618–627. doi: 10.1126/science.347575. [DOI] [PubMed] [Google Scholar]
- Benacerraf B., Nussenzweig V., Maurer P. H., Stylos W. Relationship between the net electrical charge of antigens and specific antibodies. An example of selection by antigen of cells producing highest affinity antibody. Isr J Med Sci. 1969 Mar-Apr;5(2):171–176. [PubMed] [Google Scholar]
- Berk D., Evans E. Detachment of agglutinin-bonded red blood cells. III. Mechanical analysis for large contact areas. Biophys J. 1991 Apr;59(4):861–872. doi: 10.1016/S0006-3495(91)82298-6. [DOI] [PMC free article] [PubMed] [Google Scholar]
- Cozens-Roberts C., Lauffenburger D. A., Quinn J. A. Receptor-mediated cell attachment and detachment kinetics. I. Probabilistic model and analysis. Biophys J. 1990 Oct;58(4):841–856. doi: 10.1016/S0006-3495(90)82430-9. [DOI] [PMC free article] [PubMed] [Google Scholar]
- Cozens-Roberts C., Quinn J. A., Lauffenburger D. A. Receptor-mediated cell attachment and detachment kinetics. II. Experimental model studies with the radial-flow detachment assay. Biophys J. 1990 Oct;58(4):857–872. doi: 10.1016/S0006-3495(90)82431-0. [DOI] [PMC free article] [PubMed] [Google Scholar]
- Duszyk M., Doroszewski J. Poiseuille flow method for measuring cell-to-cell adhesion. Cell Biophys. 1986 Apr;8(2):119–130. doi: 10.1007/BF02788476. [DOI] [PubMed] [Google Scholar]
- Evans E., Berk D., Leung A. Detachment of agglutinin-bonded red blood cells. I. Forces to rupture molecular-point attachments. Biophys J. 1991 Apr;59(4):838–848. doi: 10.1016/S0006-3495(91)82296-2. [DOI] [PMC free article] [PubMed] [Google Scholar]
- Evans E., Berk D., Leung A., Mohandas N. Detachment of agglutinin-bonded red blood cells. II. Mechanical energies to separate large contact areas. Biophys J. 1991 Apr;59(4):849–860. doi: 10.1016/S0006-3495(91)82297-4. [DOI] [PMC free article] [PubMed] [Google Scholar]
- Mege J. L., Capo C., Benoliel A. M., Bongrand P. Determination of binding strength and kinetics of binding initiation. A model study made on the adhesive properties of P388D1 macrophage-like cells. Cell Biophys. 1986 Apr;8(2):141–160. doi: 10.1007/BF02788478. [DOI] [PubMed] [Google Scholar]
- Milton J. G., Frojmovic M. M. Adrenaline and adenosine diphosphate-induced platelet aggregation require shape change. Importance of pseudopods. J Lab Clin Med. 1984 Nov;104(5):805–815. [PubMed] [Google Scholar]
- Pena S. D., Hughes R. C. Fibronectin-plasma membrane interactions in the adhesion and spreading of hamster fibroblasts. Nature. 1978 Nov 2;276(5683):80–83. doi: 10.1038/276080a0. [DOI] [PubMed] [Google Scholar]
- Squire P. G., Moser P., O'Konski C. T. The hydrodynamic properties of bovine serum albumin monomer and dimer. Biochemistry. 1968 Dec;7(12):4261–4272. doi: 10.1021/bi00852a018. [DOI] [PubMed] [Google Scholar]
- Tha S. P., Shuster J., Goldsmith H. L. Interaction forces between red cells agglutinated by antibody. II. Measurement of hydrodynamic force of breakup. Biophys J. 1986 Dec;50(6):1117–1126. doi: 10.1016/S0006-3495(86)83556-1. [DOI] [PMC free article] [PubMed] [Google Scholar]
- WEISS L. Studies on cellular adhesion in tissue culture. IV. The alteration of substrata by cell surfaces. Exp Cell Res. 1961 Dec;25:504–517. doi: 10.1016/0014-4827(61)90186-0. [DOI] [PubMed] [Google Scholar]
- Xia Z., Goldsmith H. L., van de Ven T. G. Kinetics of specific and nonspecific adhesion of red blood cells on glass. Biophys J. 1993 Sep;65(3):1073–1083. doi: 10.1016/S0006-3495(93)81178-0. [DOI] [PMC free article] [PubMed] [Google Scholar]