Abstract
1. The intracellular Cl activity, alpha iCl was measured inside quiescent sheep cardiac Purkinje fibres, bathed in normal Tyrode at pH 7.40, buffered with approximately 22 mM-bicarbonate/approximately 5% CO2 + 95% O2. The measurements were made using liquid ion-exchanger Cl-sensitive micro-electrodes. 2. After internal Cl levels had been depleted by prolonged exposure to Cl-free media (glururonate-substituted) when external Cl was restored, there was a rapid re-accumulation of Cl inside the fibres to levels that were much higher than those expected for a passive Cl distribution. Such a process can be conveniently defined as an active inward Cl pump. 3. The inward-pumping was noticeably temperature-sensitive (Q10 approximately 2.6), its rate was reduced about eighteenfold in the nominal absence of external bicarbonate/CO2 and it was substantially inhibited by the drug SITS (4-acetamido-4'-isothiocyanato-stilbene-2,2'-disulphonic acid). 4. The fall of alpha iCl in Cl-free solution was slow and was also equally temperature-sensitive and substantially inhibited by SITS, but was only slightly impaired in the nominal absence of external bicarbonate/CO2. 5. pHi was measured using recessed-tip pH-sensitive micro-electrodes, and in some experiments both pHi and alpha iCl were monitored simultaneously. When alpha iCl slowly declined in Cl-free solution then pHi slowly became alkaline. Upon restoring external Cl, then there was, as usual, a rapid recovery of a high alpha iCl and this was accompanied by a rapid re-acidification of pHi. Both the recovery of alpha iCl and pHi were exponential with virtually the same time constant. 6. Both the slow alkalinization of pHi in Cl-free solution and the rapid re-acidification upon restoring external Cl were substantially inhibited by the drug SITS. 7. When [k]O was raised to 45 mM or more (by removing equivalent amounts of [Na]O), there was a large depolarization of Em and a slow rise of alpha iCl, which was not accompanied by a large change of pHi. The rise of alpha iCl appeared to be unaffected by SITS. 8. It is suggested that a Cl/CHO-3 exchange mechanism can operate reversibly across the membrane of quiescent Purkinje fibres, and that it can account, at least in part, for the high levels of alpha iCl measured in the resting state. It is also concluded that Cl can cross the membrane in other ways, especially in high-K solution possibly by moving passively through conductance channels that are open under these conditions.
Full text
PDF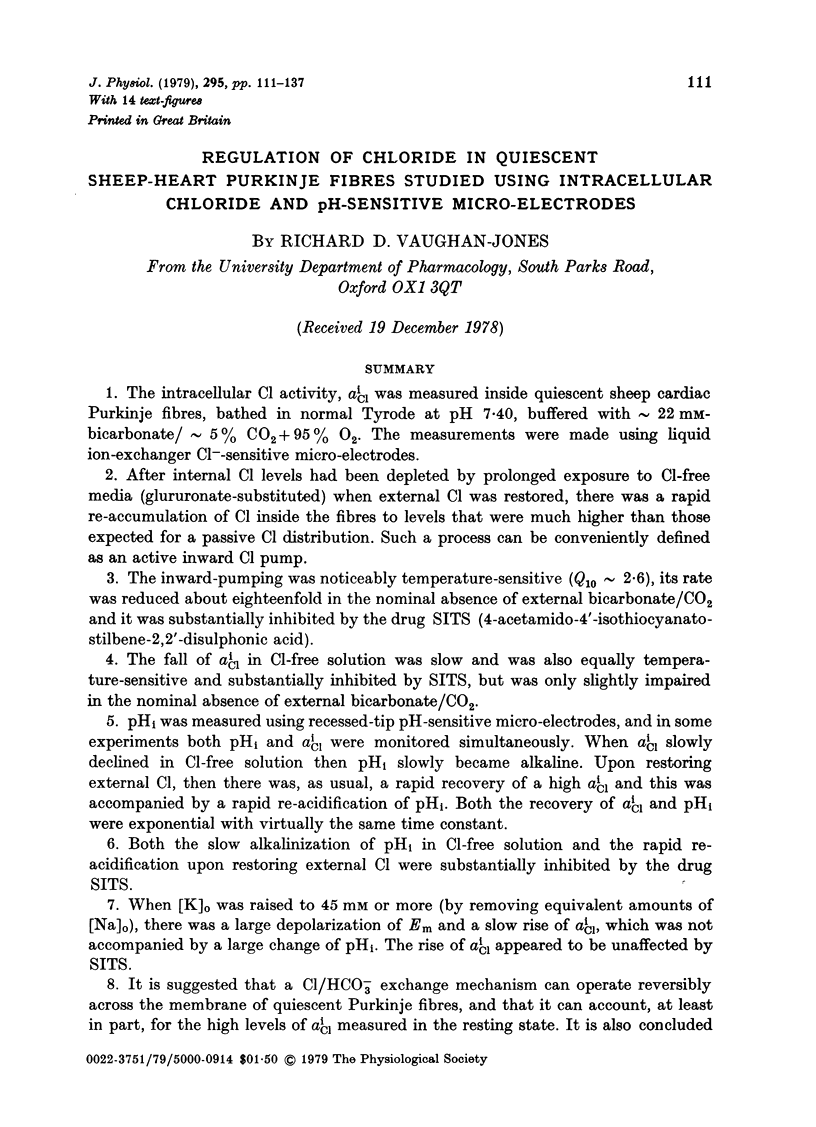
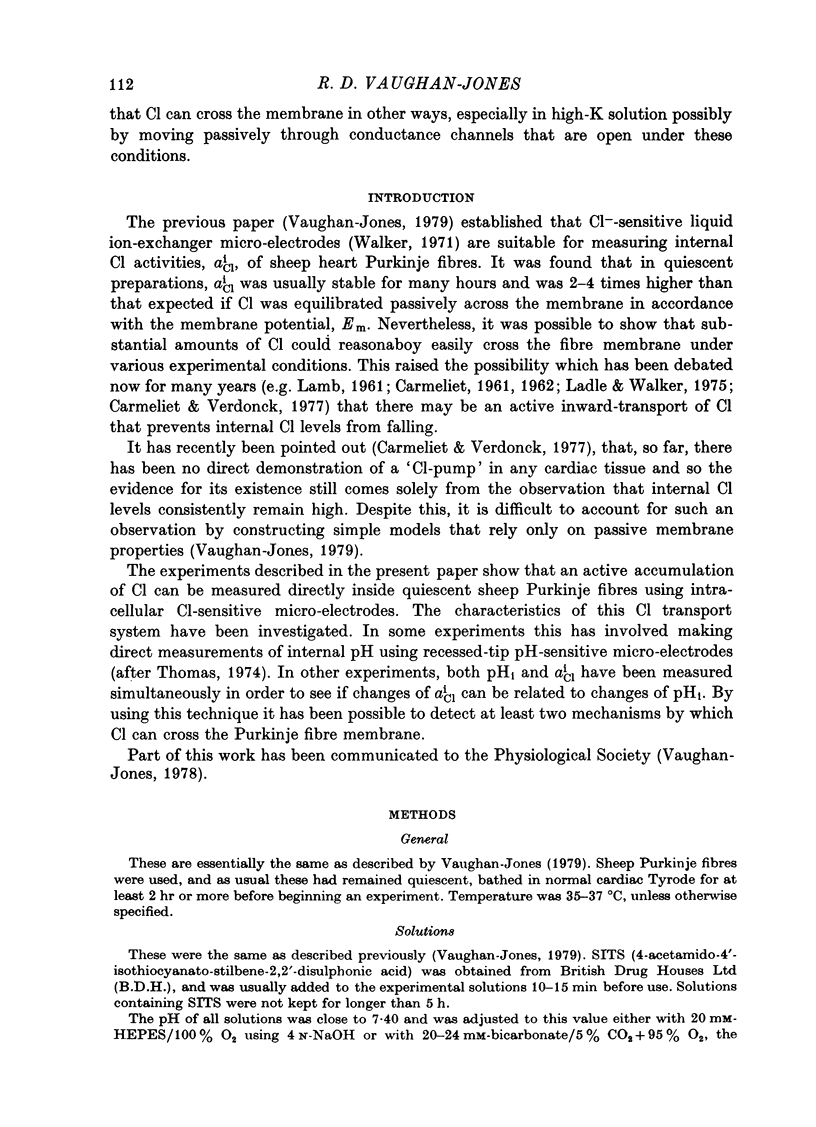
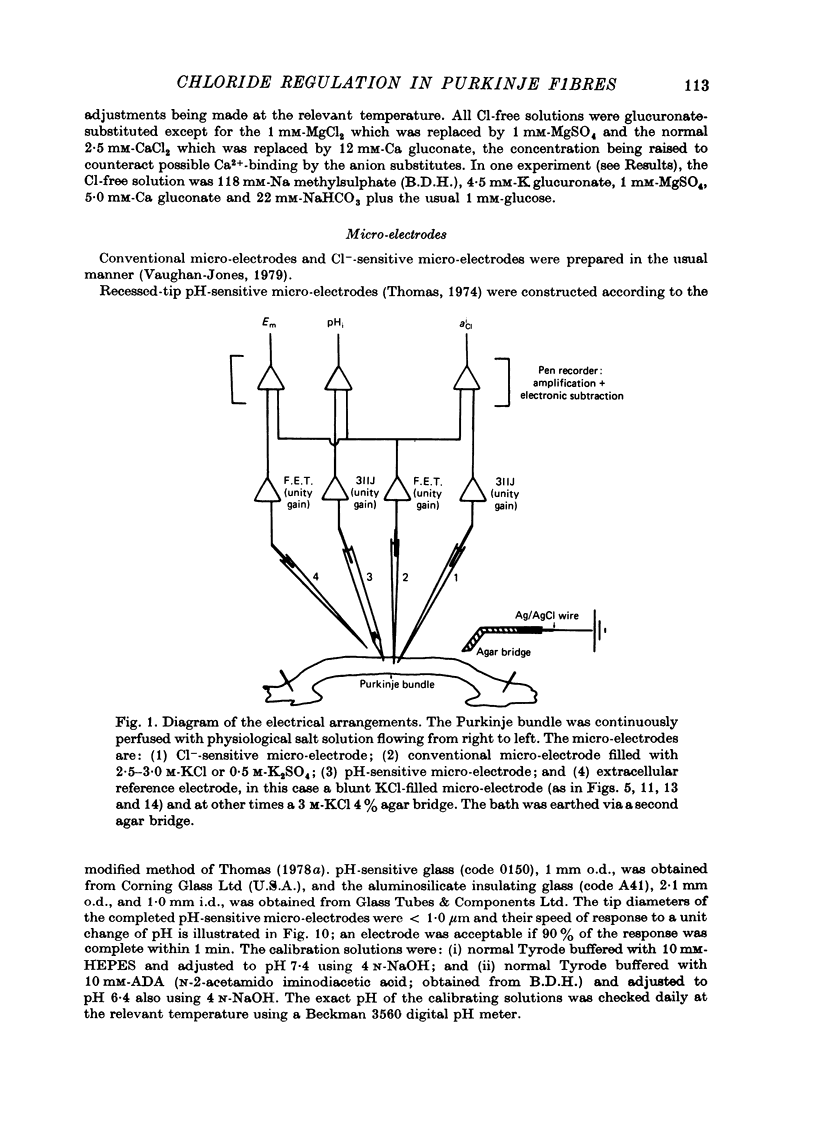
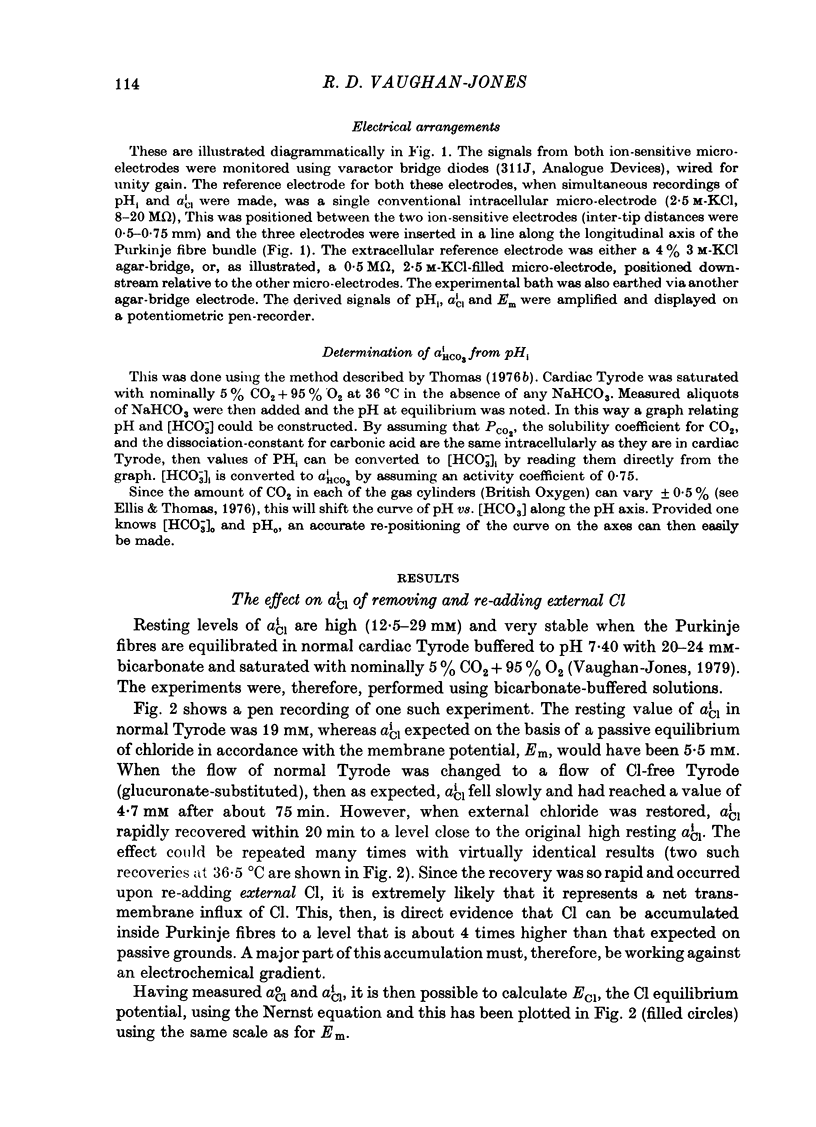
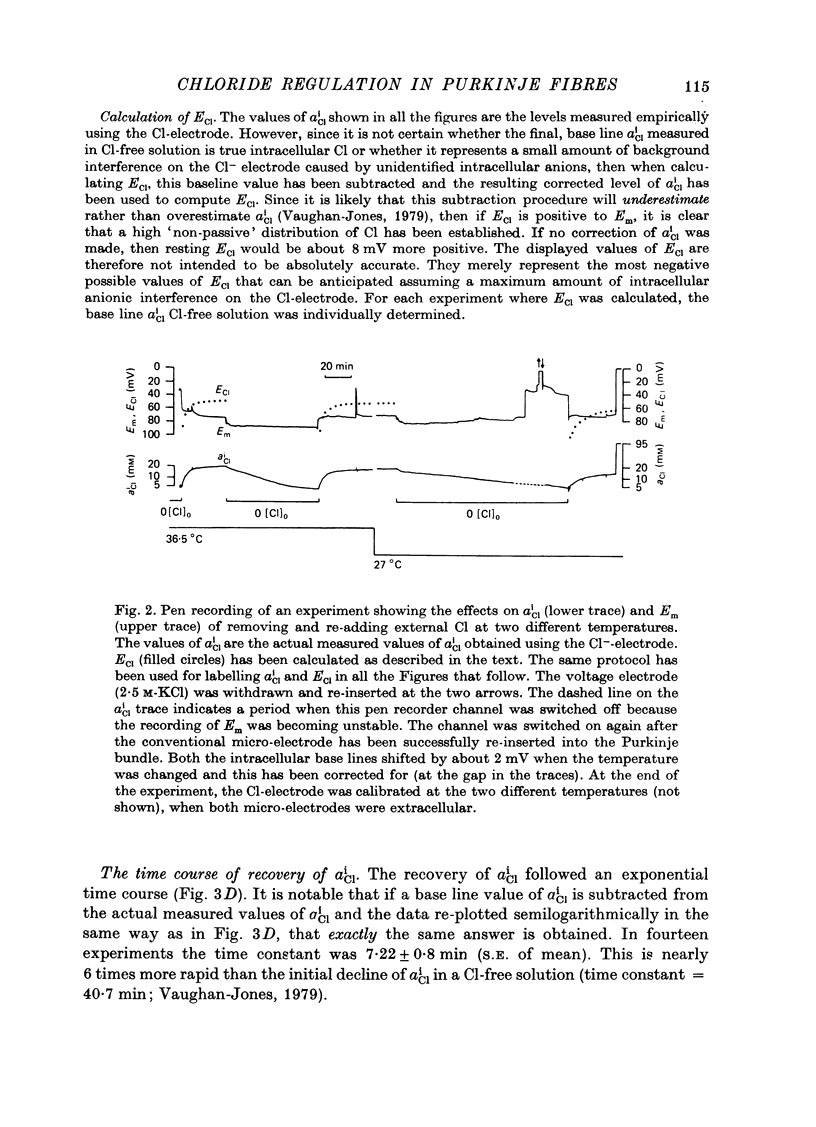
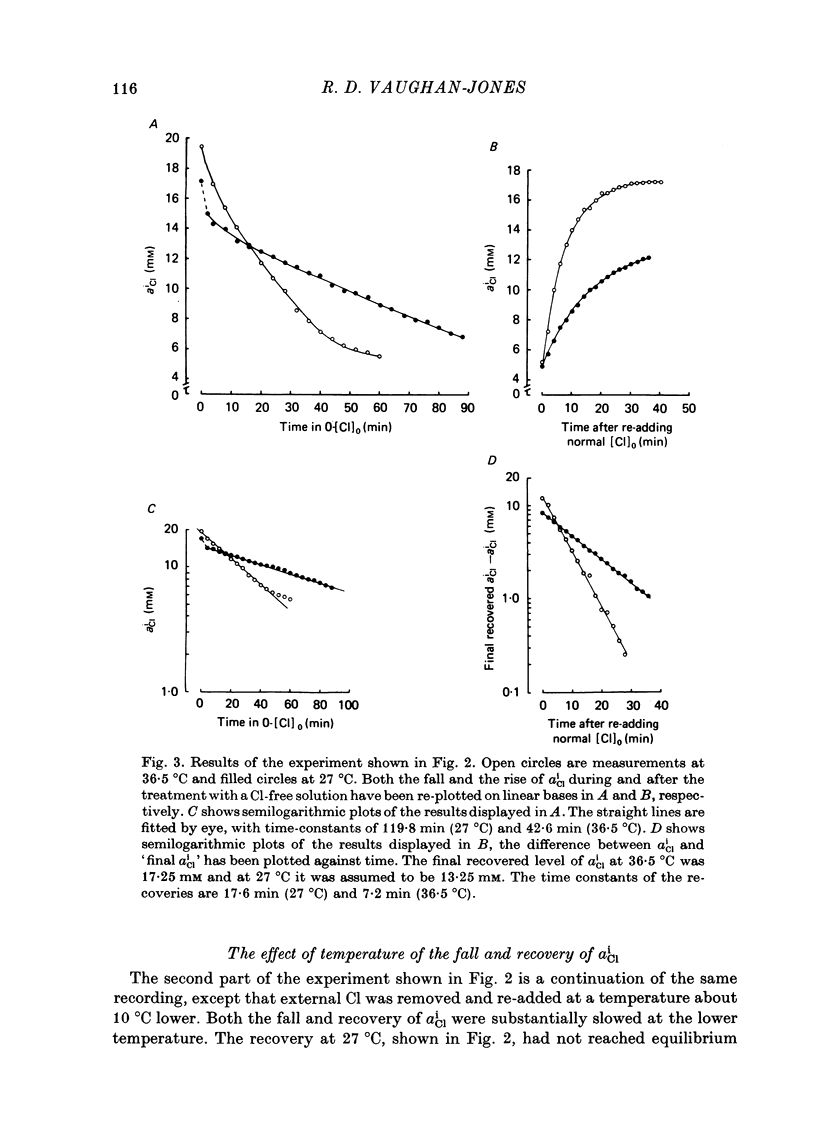
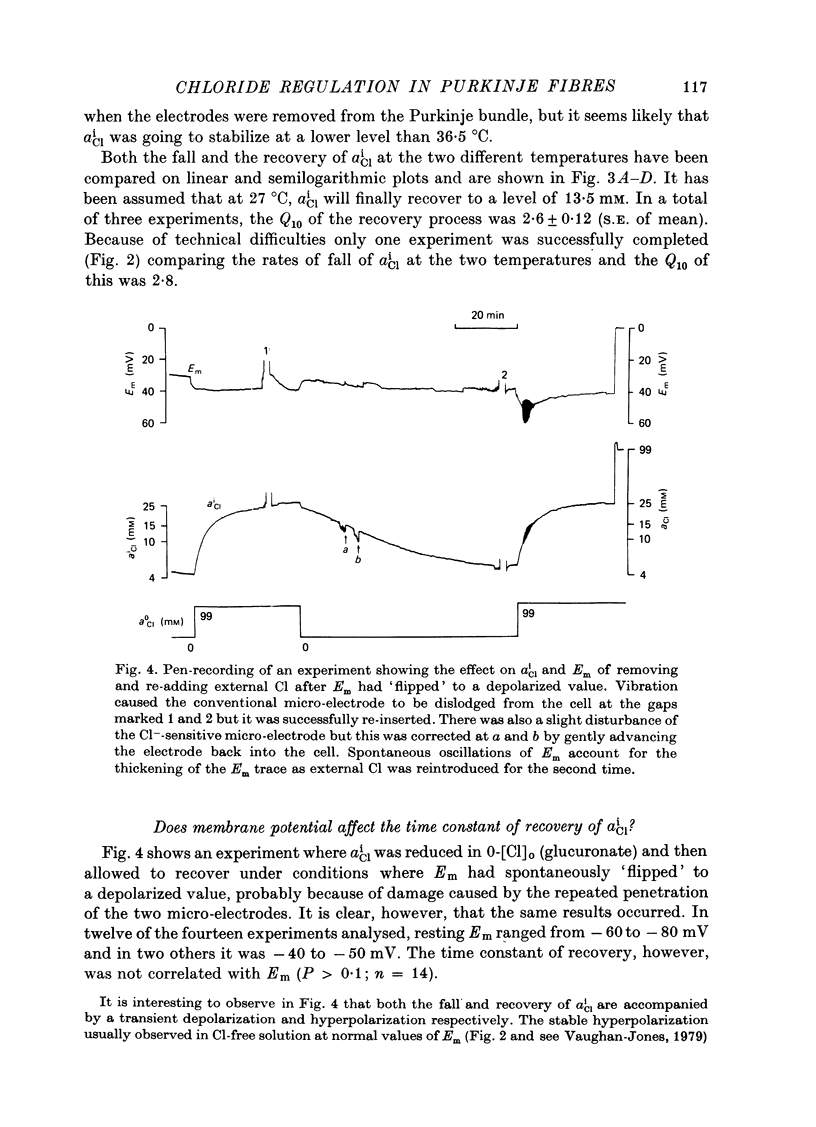
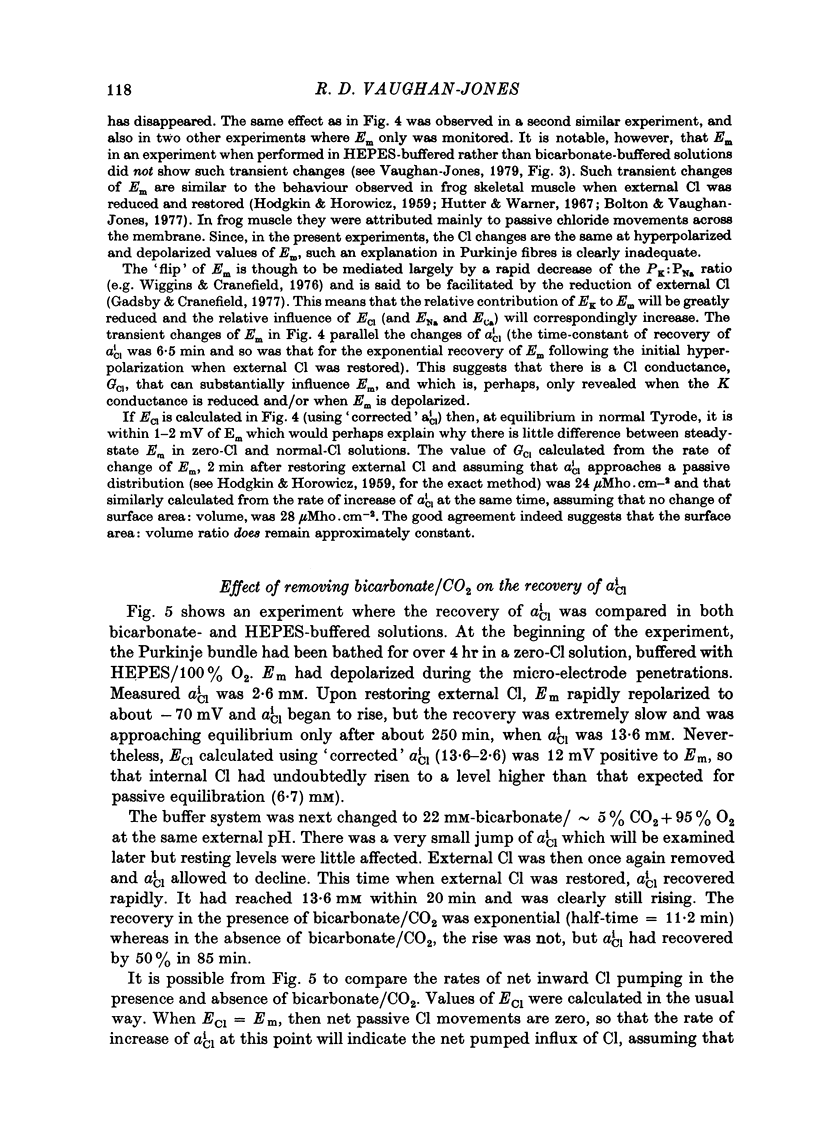
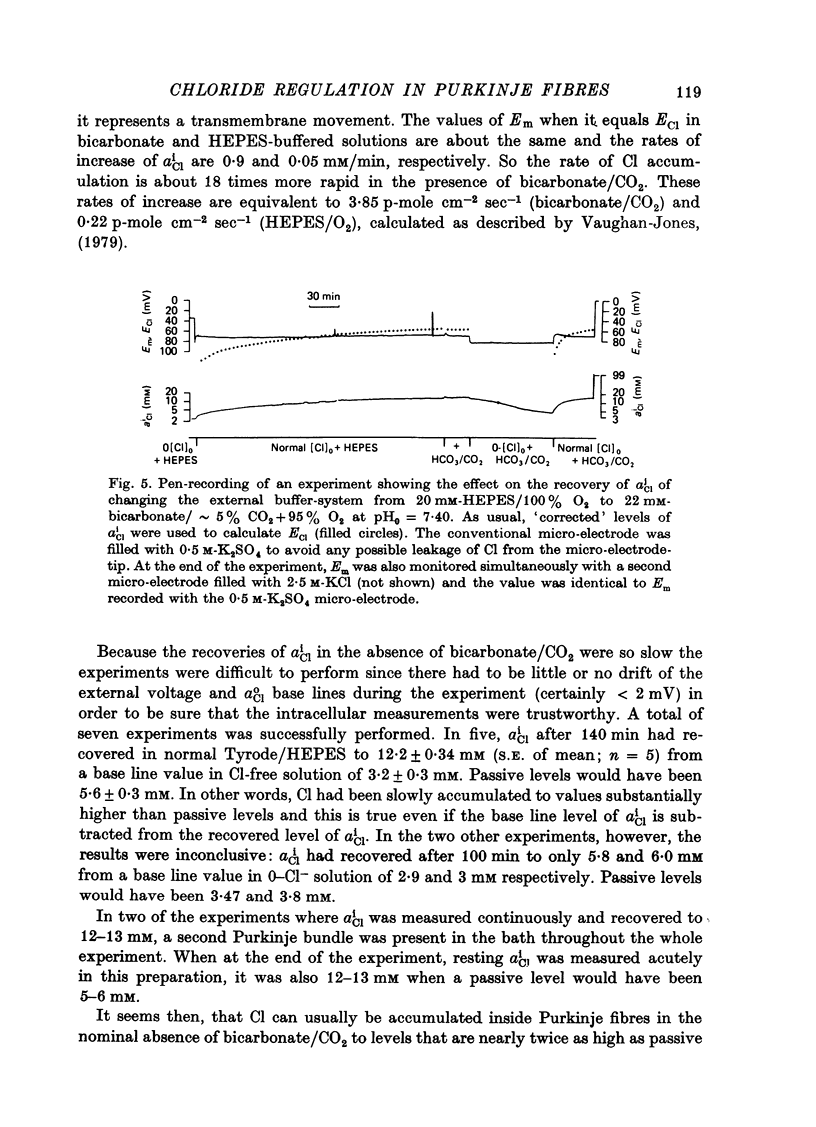
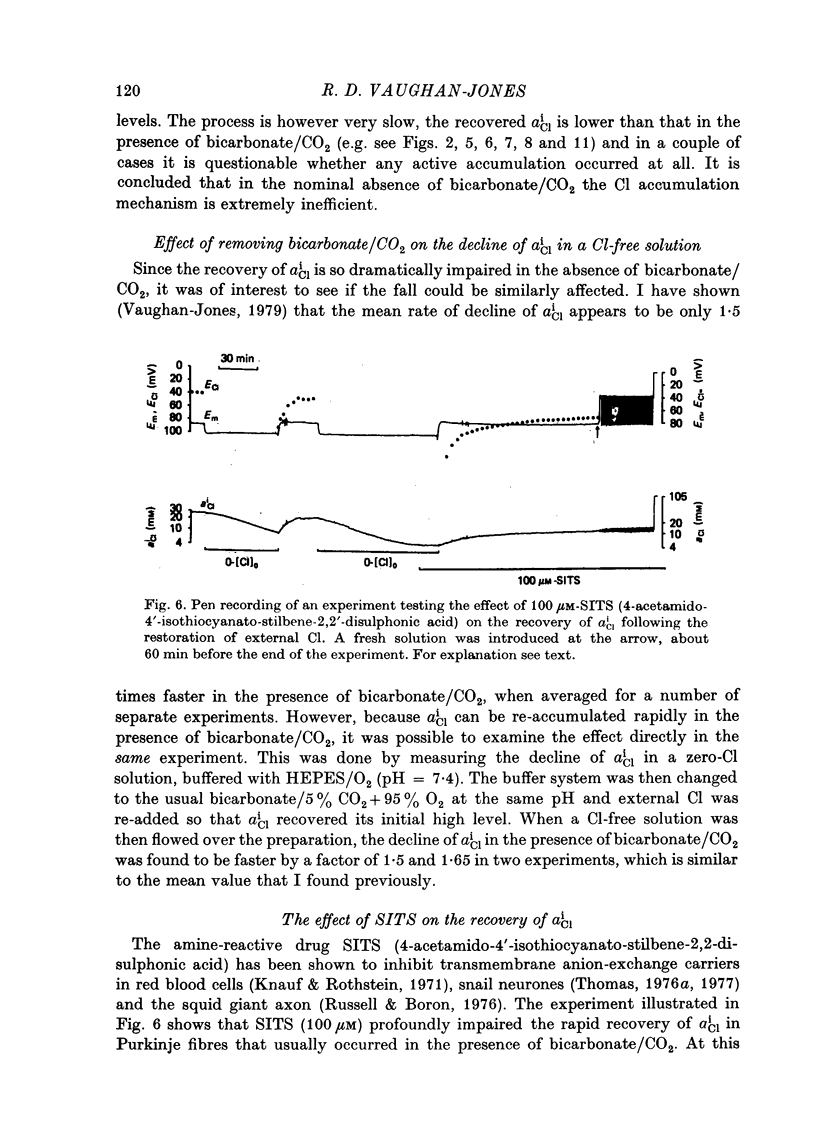
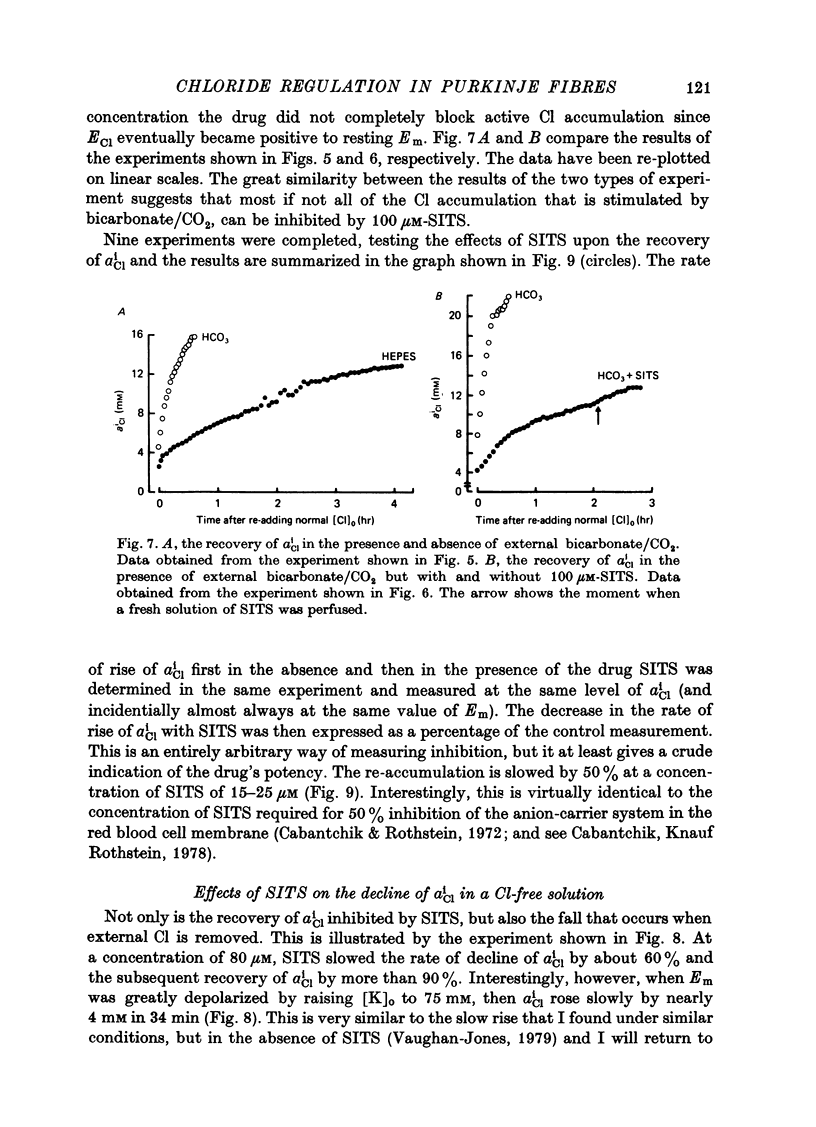
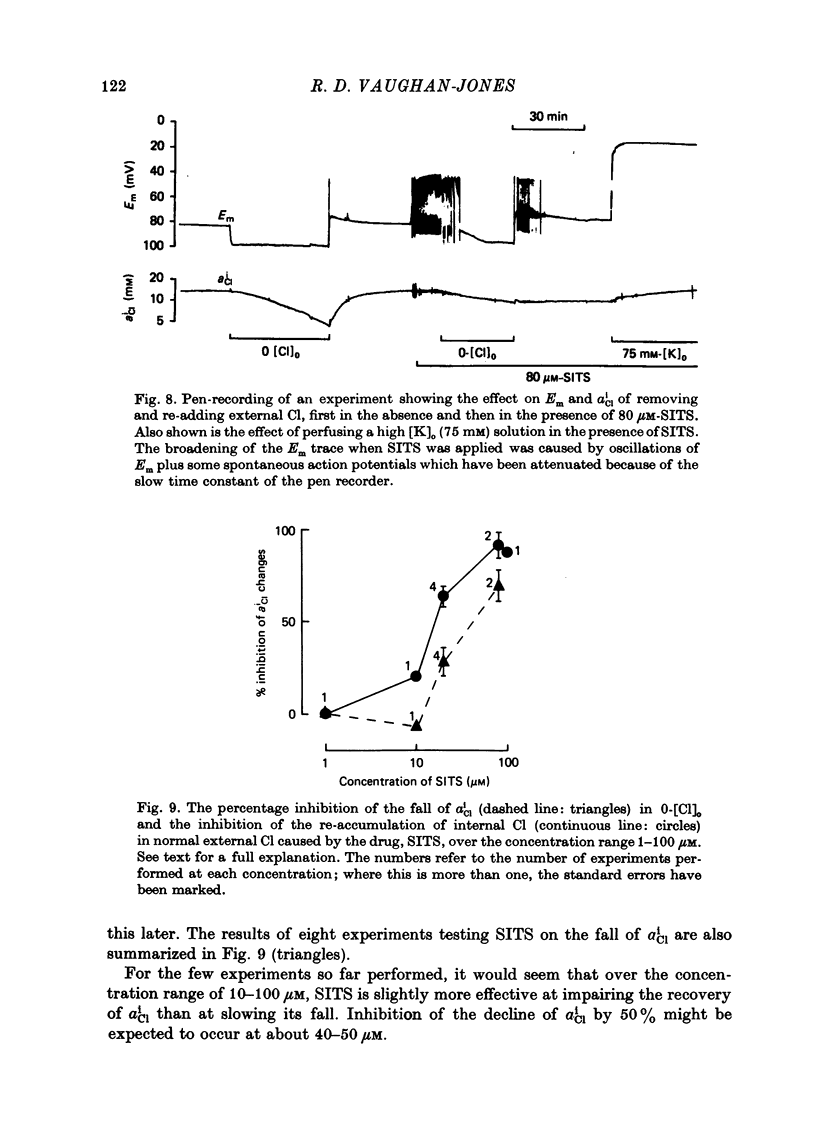
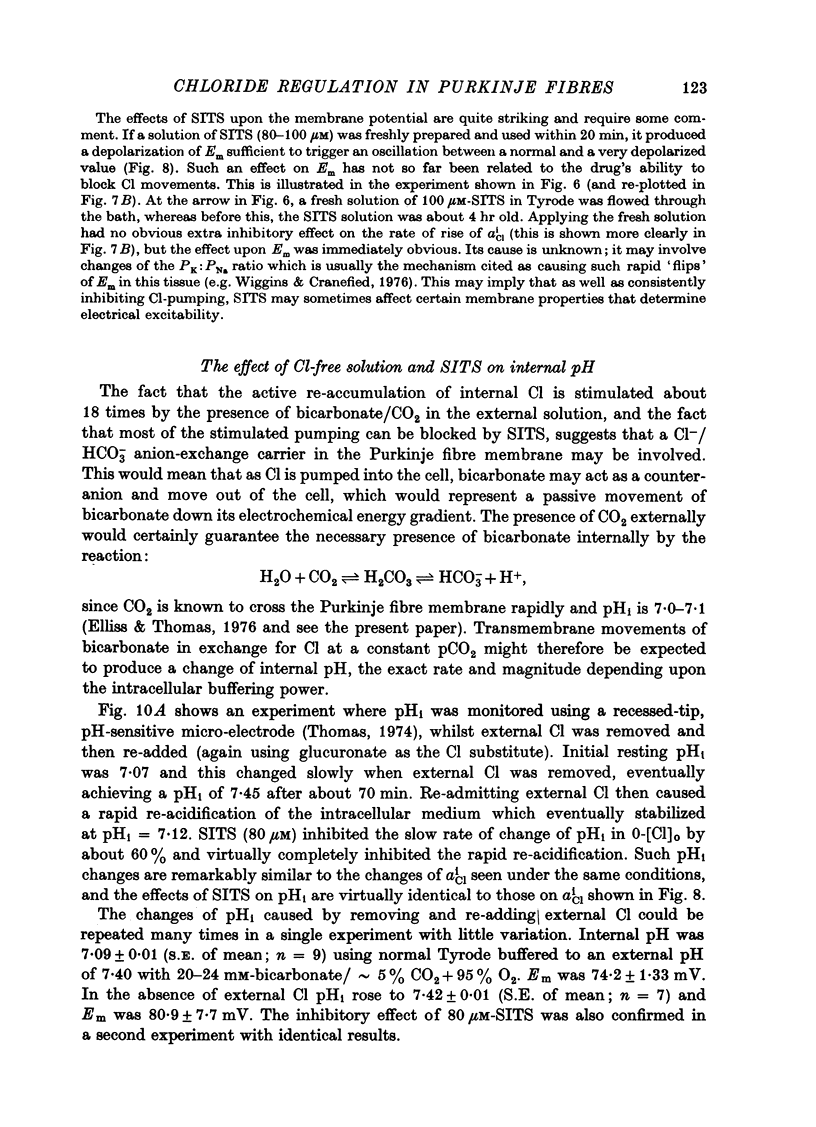
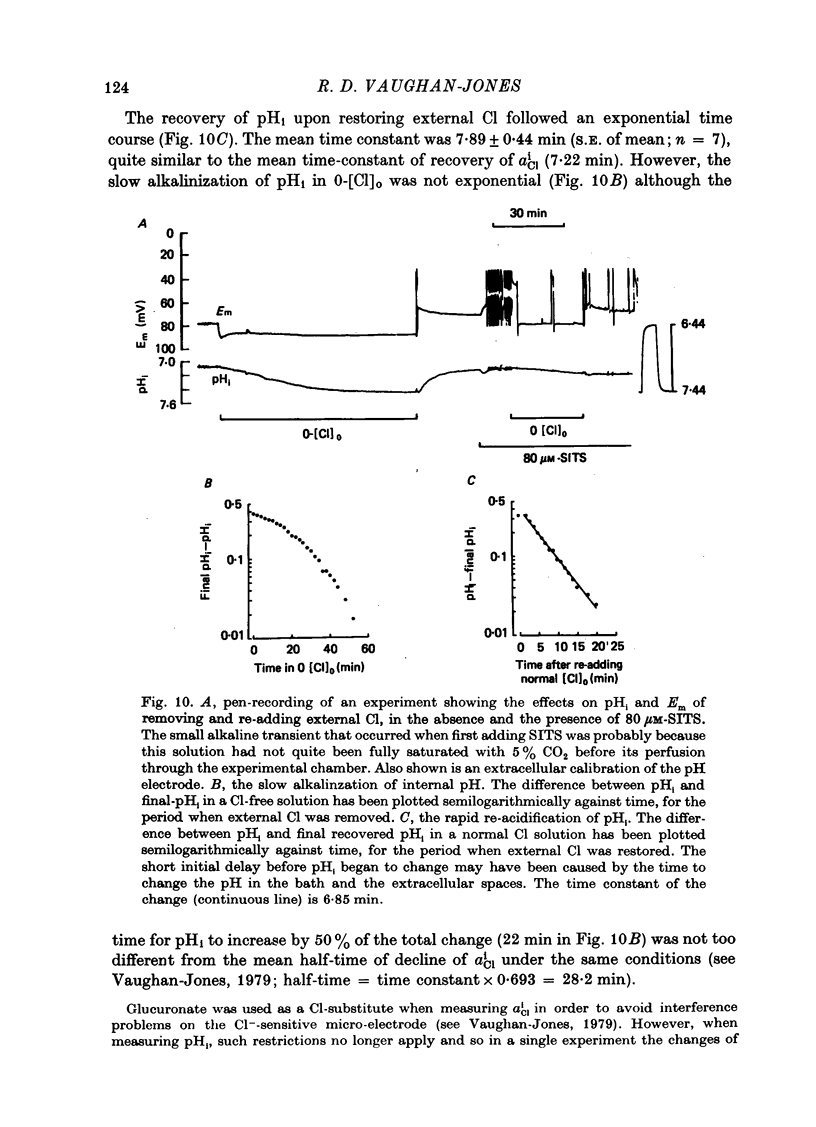
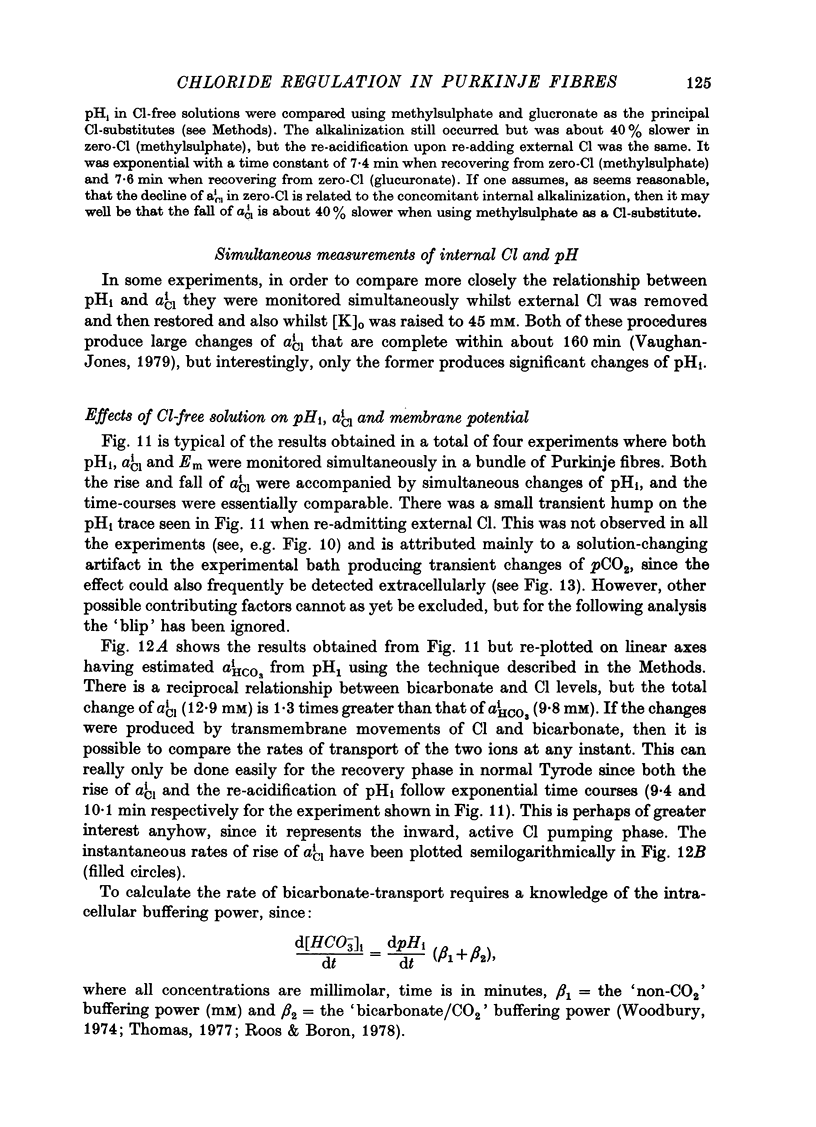
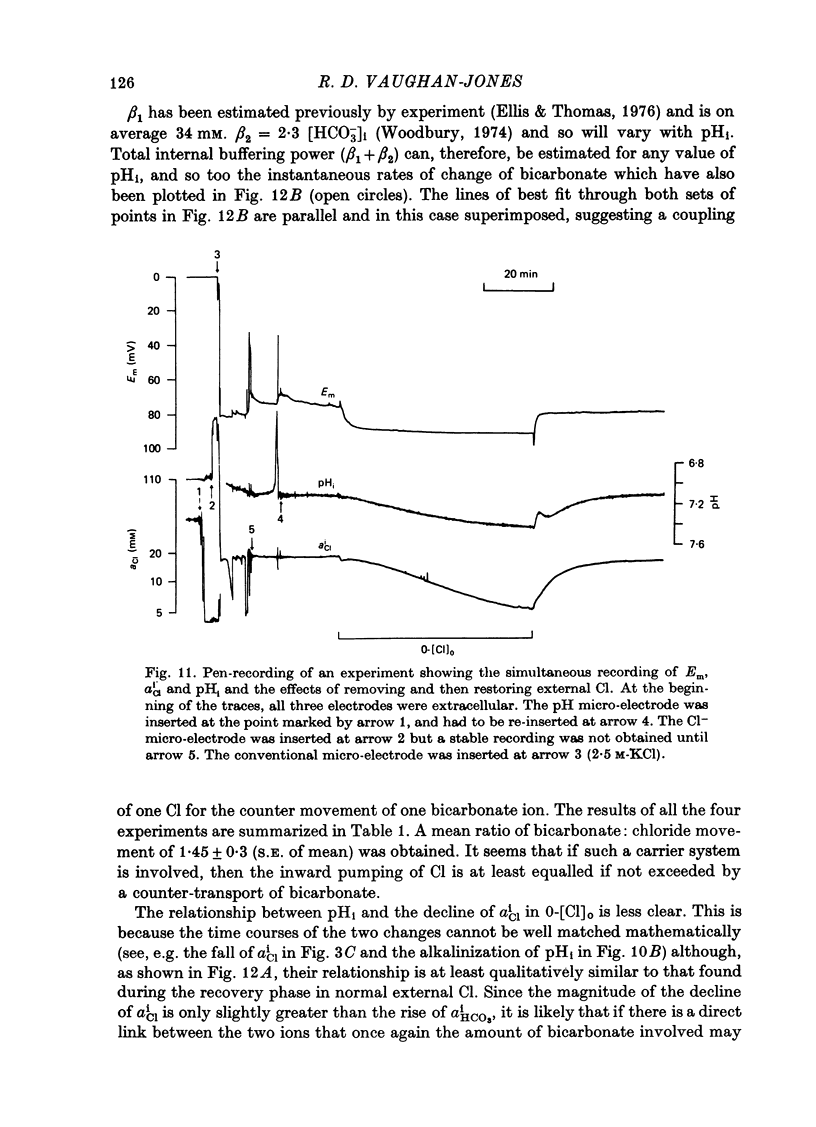
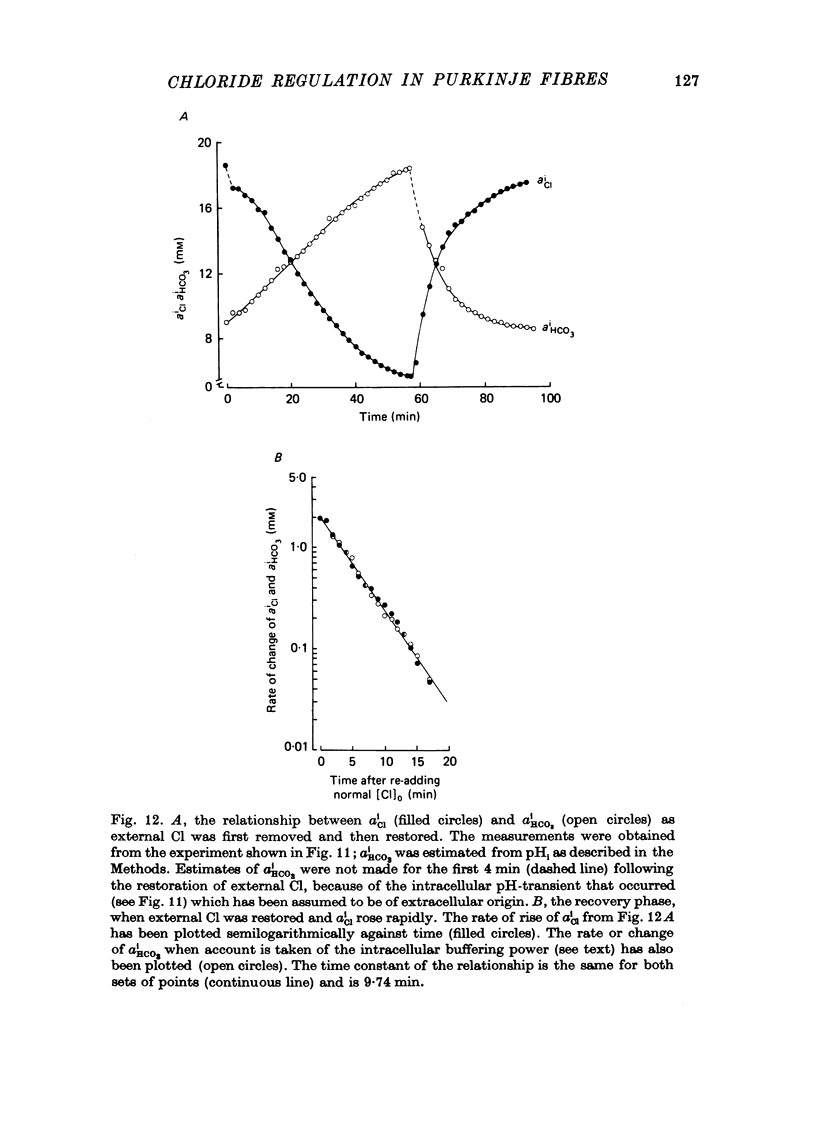
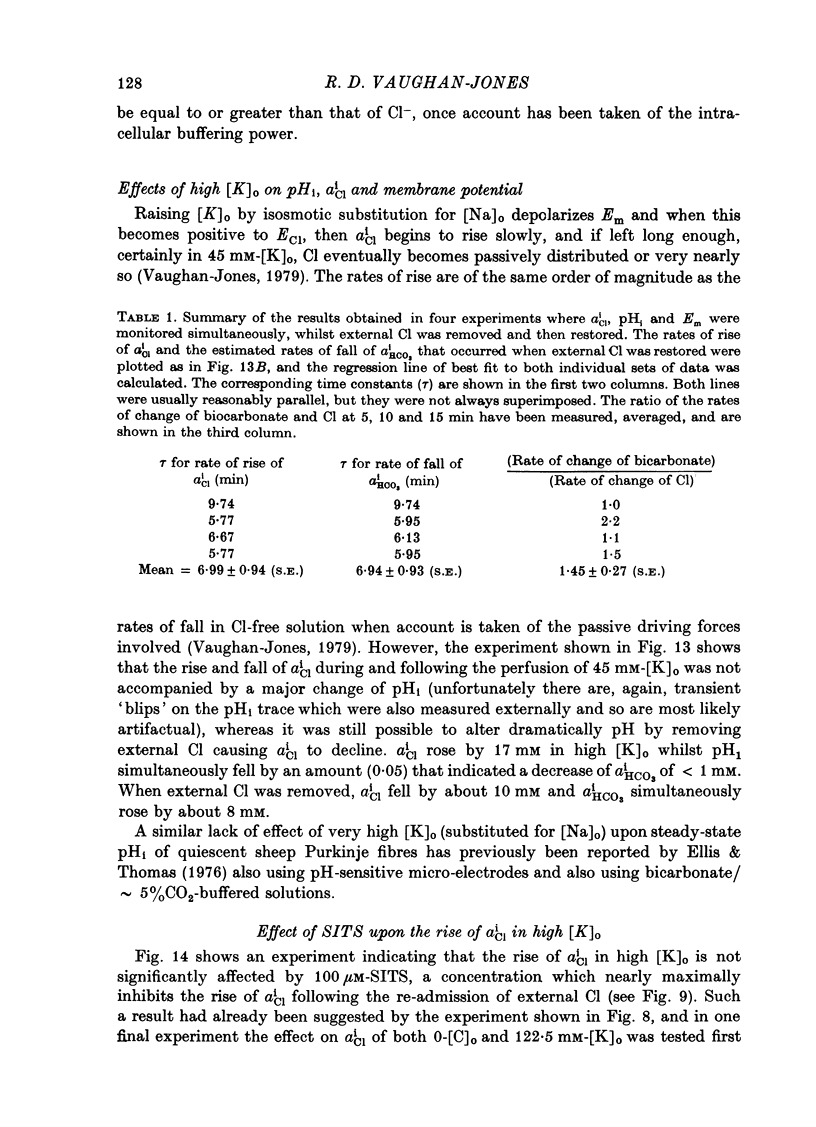
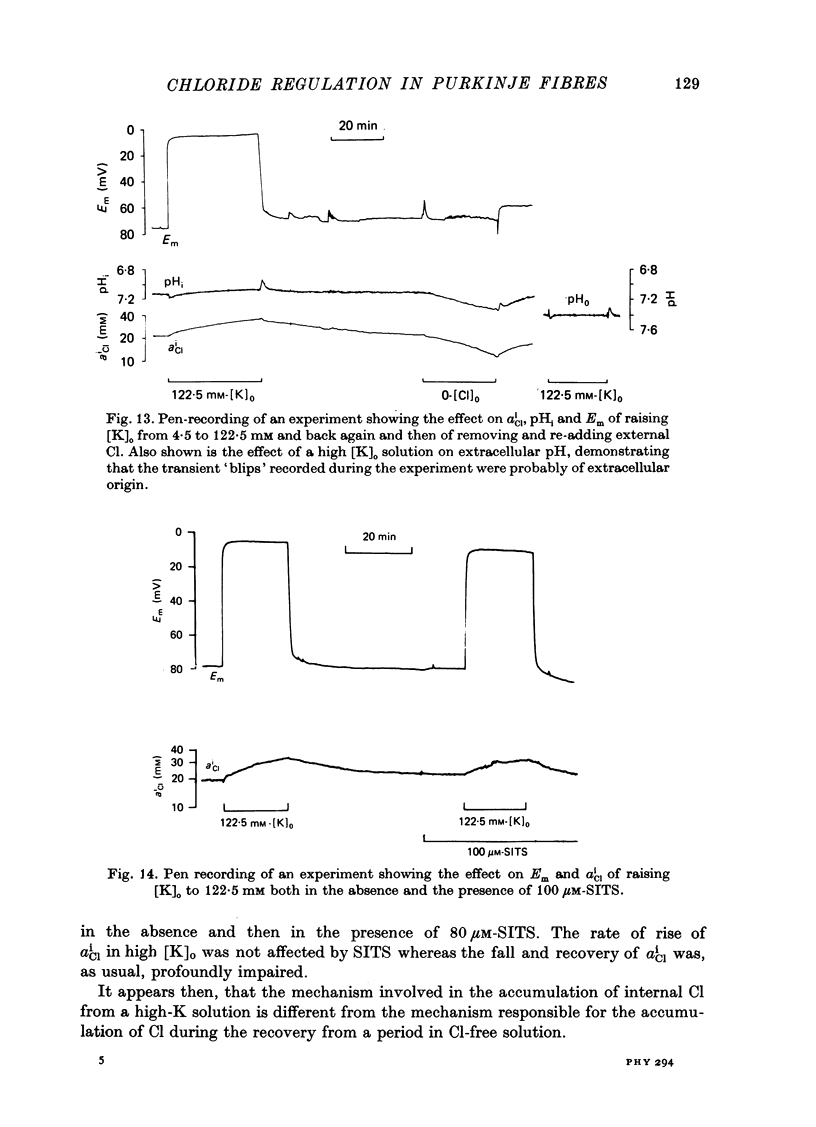
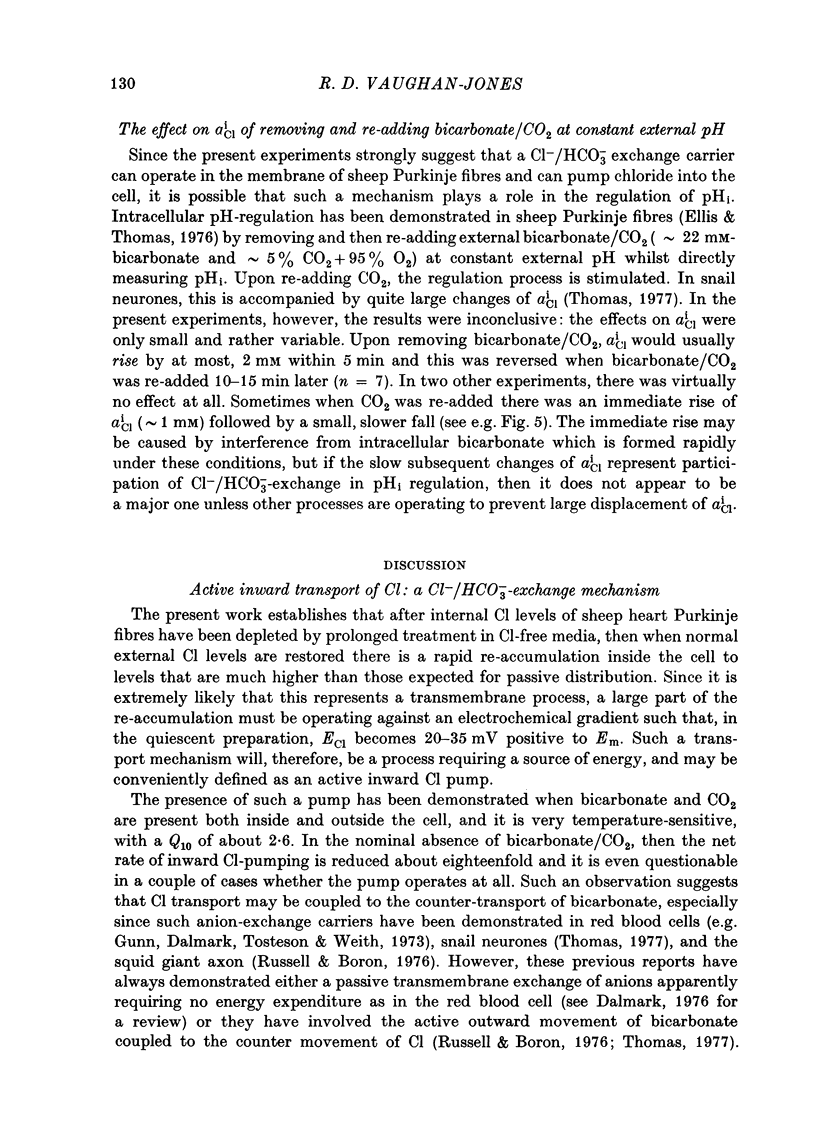
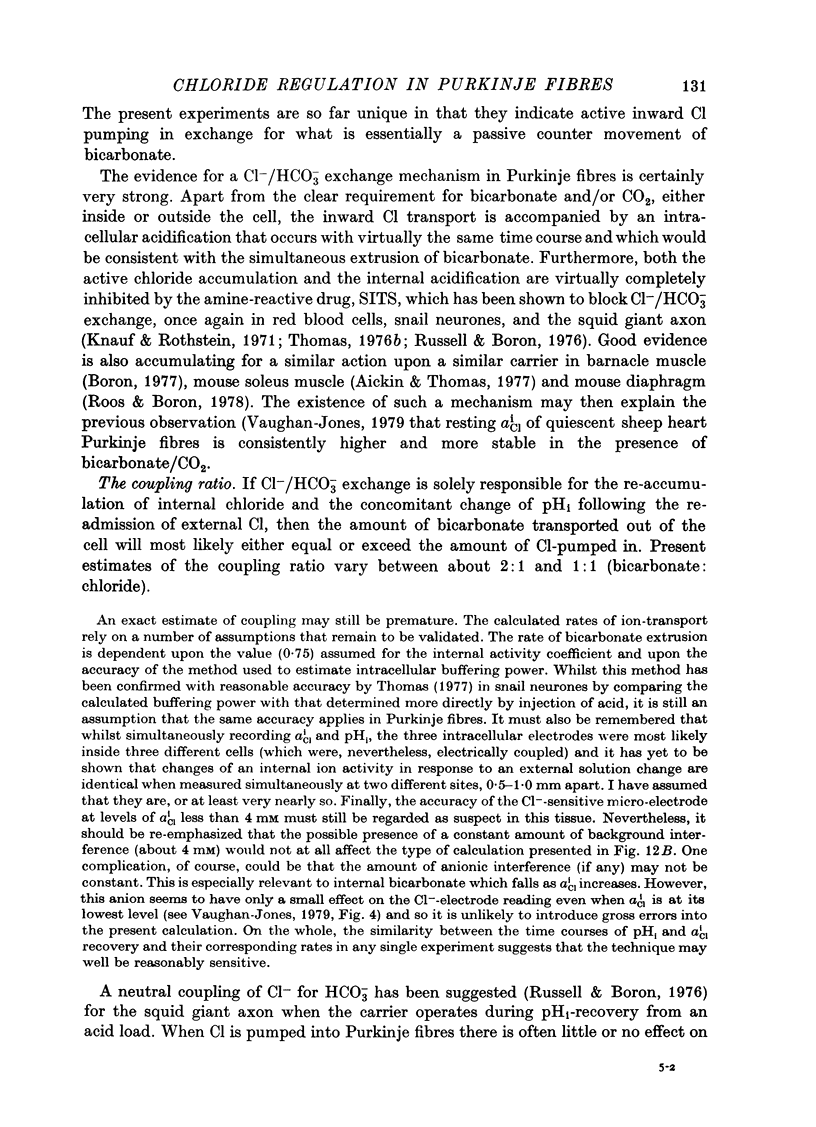
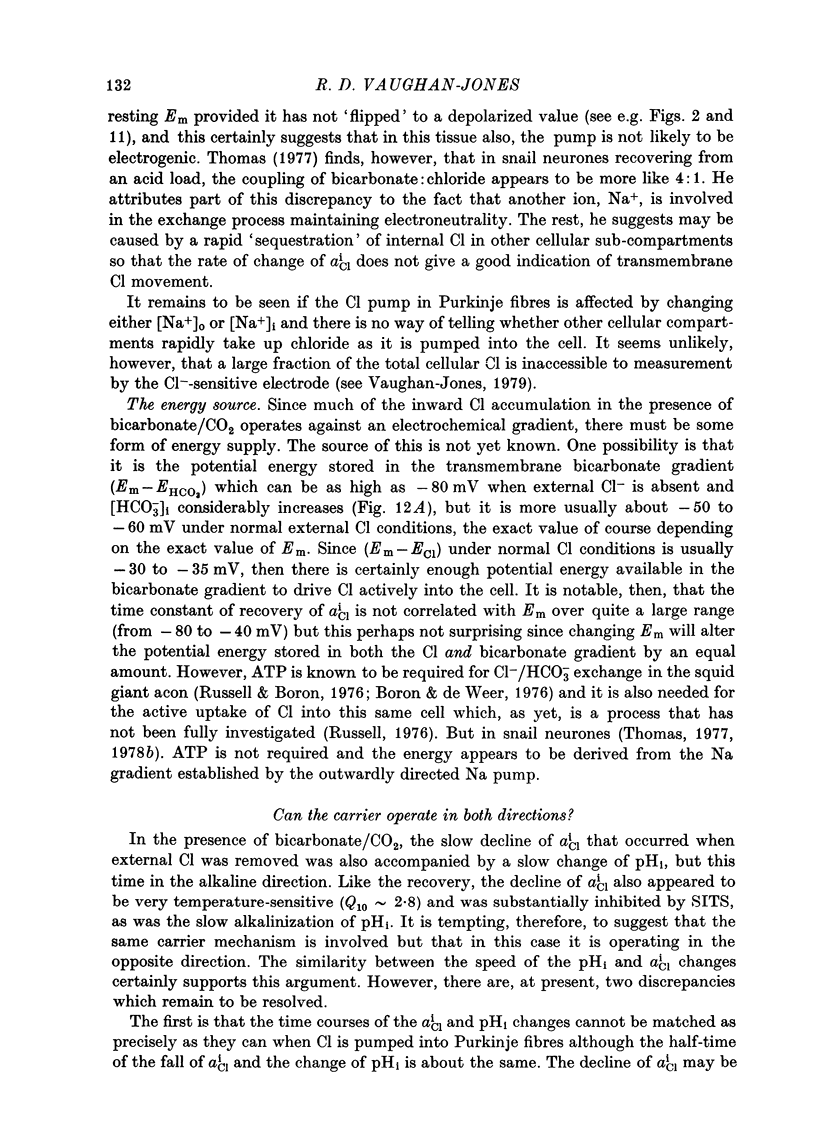
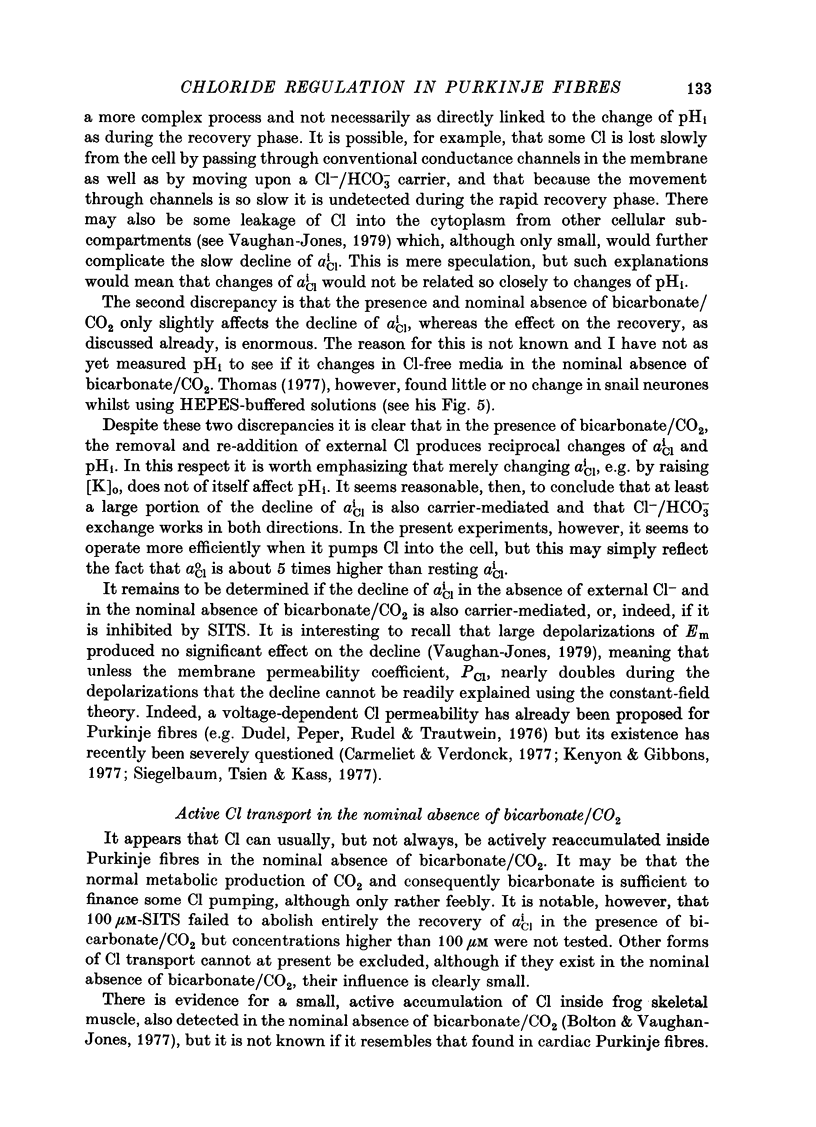
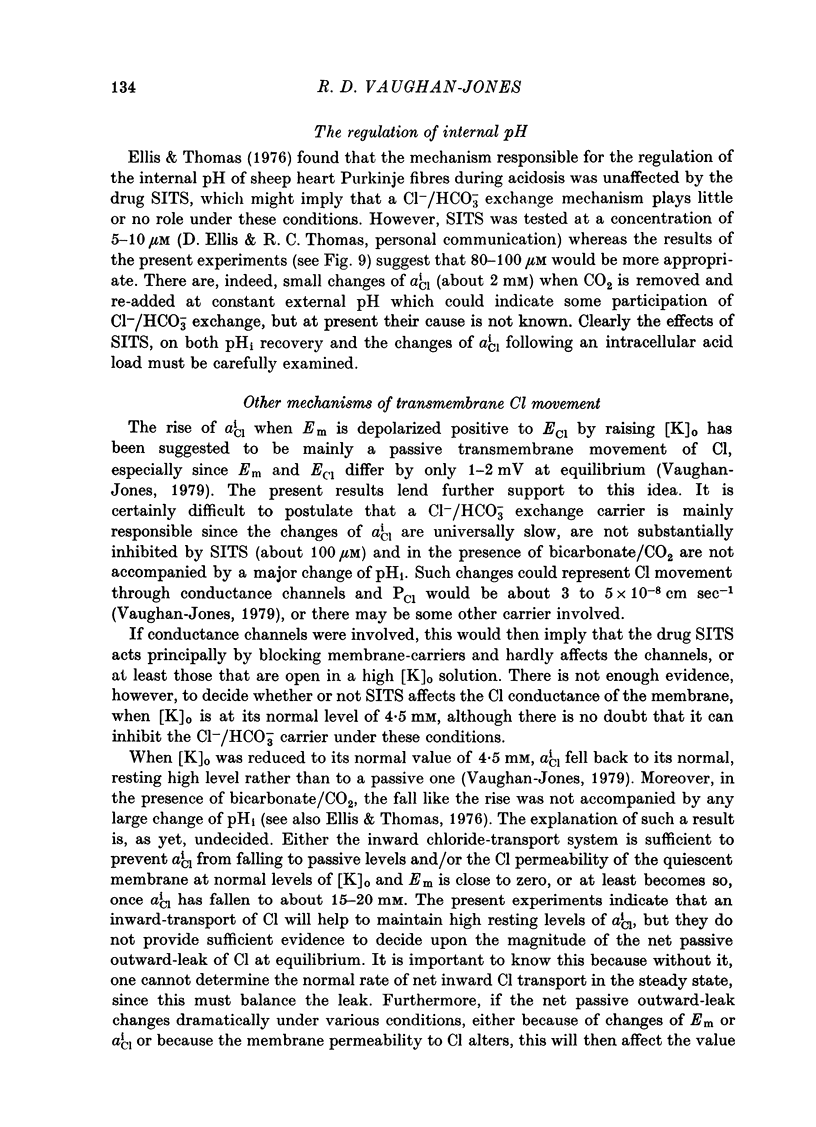
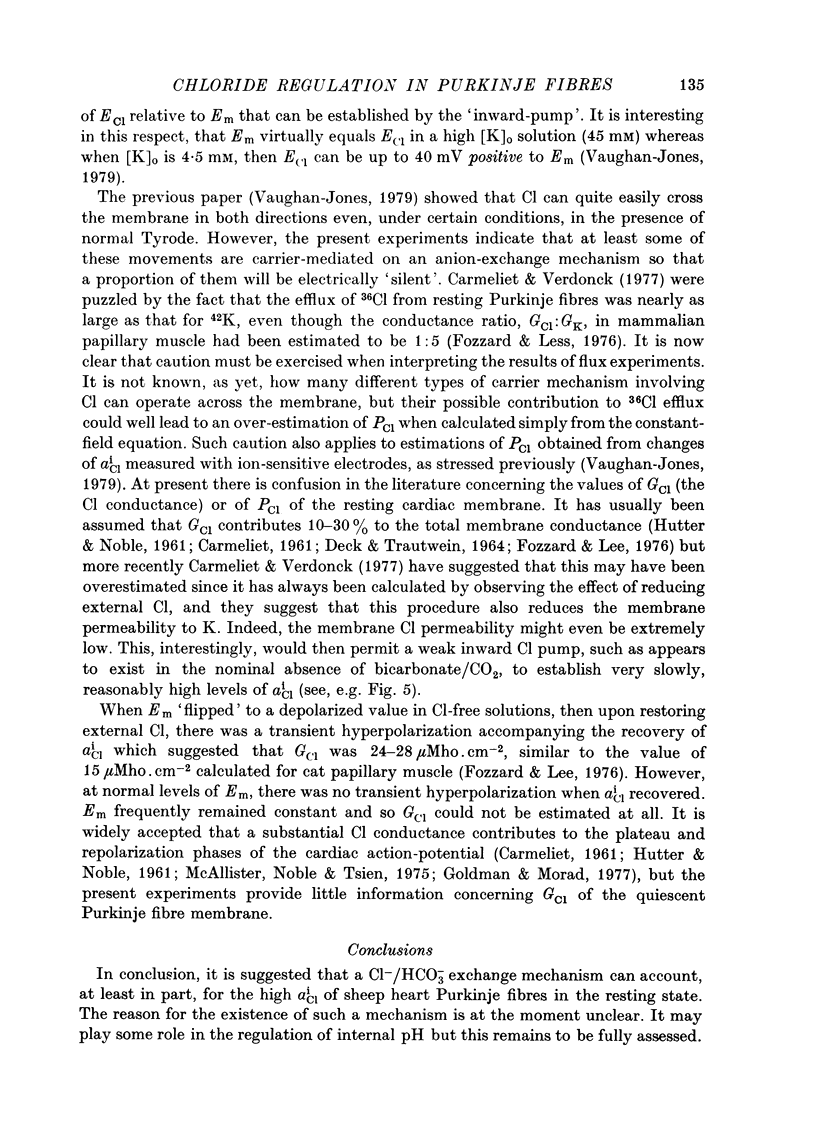
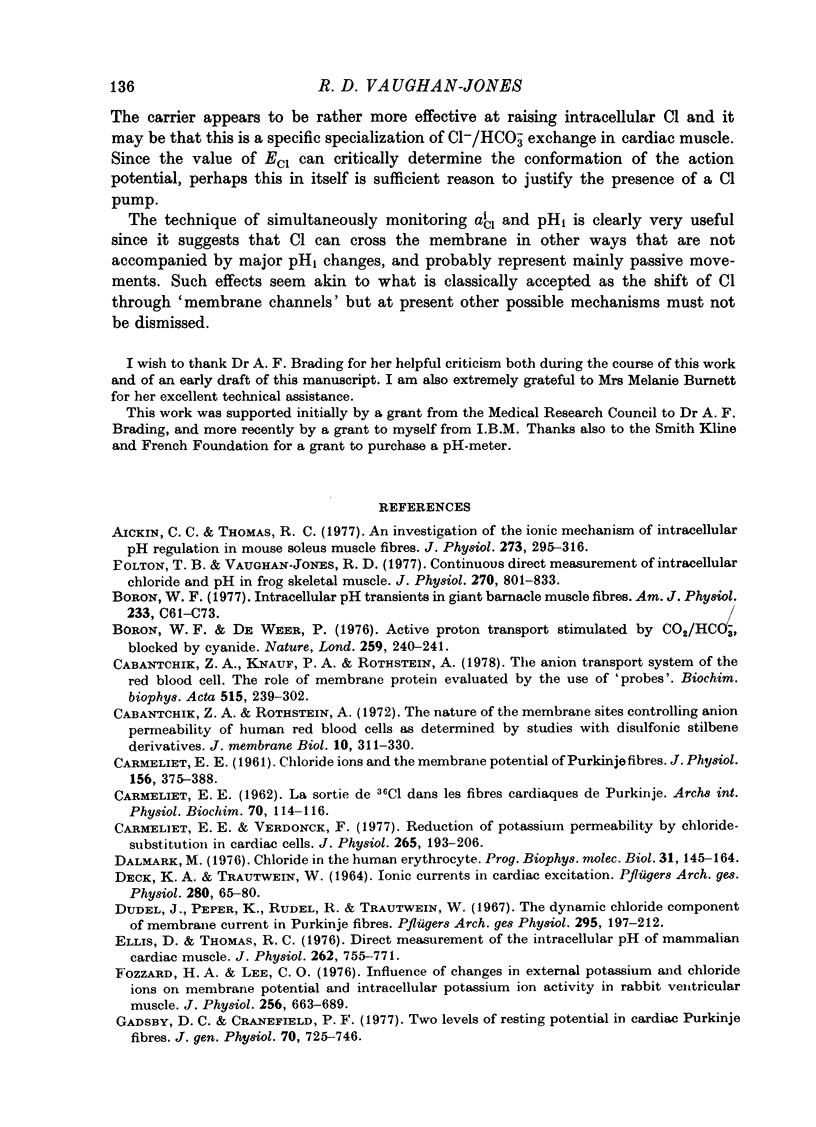
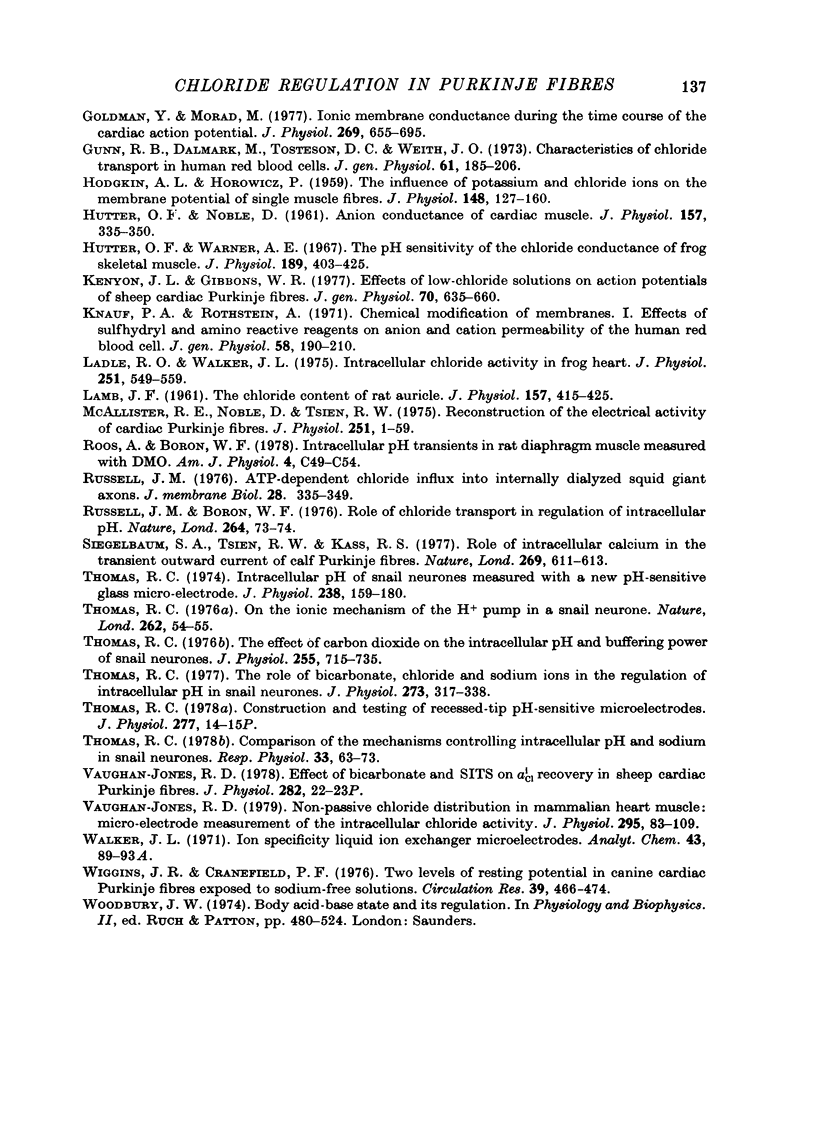
Selected References
These references are in PubMed. This may not be the complete list of references from this article.
- Aickin C. C., Thomas R. C. An investigation of the ionic mechanism of intracellular pH regulation in mouse soleus muscle fibres. J Physiol. 1977 Dec;273(1):295–316. doi: 10.1113/jphysiol.1977.sp012095. [DOI] [PMC free article] [PubMed] [Google Scholar]
- Bolton T. B., Vaughan-Jones R. D. Continuous direct measurement of intracellular chloride and pH in frog skeletal muscle. J Physiol. 1977 Sep;270(3):801–833. doi: 10.1113/jphysiol.1977.sp011983. [DOI] [PMC free article] [PubMed] [Google Scholar]
- Boron W. F., De Weer P. Active proton transport stimulated by CO2/HCO3-, blocked by cyanide. Nature. 1976 Jan 22;259(5540):240–241. doi: 10.1038/259240a0. [DOI] [PubMed] [Google Scholar]
- Boron W. F. Intracellular pH transients in giant barnacle muscle fibers. Am J Physiol. 1977 Sep;233(3):C61–C73. doi: 10.1152/ajpcell.1977.233.3.C61. [DOI] [PubMed] [Google Scholar]
- CARMELIET E. E. Chloride ions and the membrane potential of Purkinje fibres. J Physiol. 1961 Apr;156:375–388. doi: 10.1113/jphysiol.1961.sp006682. [DOI] [PMC free article] [PubMed] [Google Scholar]
- Cabantchik Z. I., Knauf P. A., Rothstein A. The anion transport system of the red blood cell. The role of membrane protein evaluated by the use of 'probes'. Biochim Biophys Acta. 1978 Sep 29;515(3):239–302. doi: 10.1016/0304-4157(78)90016-3. [DOI] [PubMed] [Google Scholar]
- Cabantchik Z. I., Rothstein A. The nature of the membrane sites controlling anion permeability of human red blood cells as determined by studies with disulfonic stilbene derivatives. J Membr Biol. 1972 Dec 29;10(3):311–330. doi: 10.1007/BF01867863. [DOI] [PubMed] [Google Scholar]
- Carmeliet E., Verdonck F. Reduction of potassium permeability by chloride substitution in cardiac cells. J Physiol. 1977 Feb;265(1):193–206. doi: 10.1113/jphysiol.1977.sp011712. [DOI] [PMC free article] [PubMed] [Google Scholar]
- DECK K. A., TRAUTWEIN W. IONIC CURRENTS IN CARDIAC EXCITATION. Pflugers Arch Gesamte Physiol Menschen Tiere. 1964 Jun 9;280:63–80. doi: 10.1007/BF00412616. [DOI] [PubMed] [Google Scholar]
- Dalmark M. Chloride in the human erythrocyte: distribution and transport between cellular and extracellular fluids and structural features of the cell membrane. Prog Biophys Mol Biol. 1976;31(2):145–164. [PubMed] [Google Scholar]
- Dudel J., Peper K., Rüdel R., Trautwein W. The dynamic chloride component of membrane current in Purkinje fibers. Pflugers Arch Gesamte Physiol Menschen Tiere. 1967;295(3):197–212. doi: 10.1007/BF01844100. [DOI] [PubMed] [Google Scholar]
- Ellis D., Thomas R. C. Direct measurement of the intracellular pH of mammalian cardiac muscle. J Physiol. 1976 Nov;262(3):755–771. doi: 10.1113/jphysiol.1976.sp011619. [DOI] [PMC free article] [PubMed] [Google Scholar]
- Fozzard H. A., Lee C. O. Influence of changes in external potassium and chloride ions on membrane potential and intracellular potassium ion activity in rabbit ventricular muscle. J Physiol. 1976 Apr;256(3):663–689. doi: 10.1113/jphysiol.1976.sp011345. [DOI] [PMC free article] [PubMed] [Google Scholar]
- Gadsby D. C., Cranefield P. F. Two levels of resting potential in cardiac Purkinje fibers. J Gen Physiol. 1977 Dec;70(6):725–746. doi: 10.1085/jgp.70.6.725. [DOI] [PMC free article] [PubMed] [Google Scholar]
- Goldman Y., Morad M. Ionic membrane conductance during the time course of the cardiac action potential. J Physiol. 1977 Jul;268(3):655–695. doi: 10.1113/jphysiol.1977.sp011876. [DOI] [PMC free article] [PubMed] [Google Scholar]
- Gunn R. B., Dalmark M., Tosteson D. C., Wieth J. O. Characteristics of chloride transport in human red blood cells. J Gen Physiol. 1973 Feb;61(2):185–206. doi: 10.1085/jgp.61.2.185. [DOI] [PMC free article] [PubMed] [Google Scholar]
- HODGKIN A. L., HOROWICZ P. The influence of potassium and chloride ions on the membrane potential of single muscle fibres. J Physiol. 1959 Oct;148:127–160. doi: 10.1113/jphysiol.1959.sp006278. [DOI] [PMC free article] [PubMed] [Google Scholar]
- HUTTER O. F., NOBLE D. Anion conductance of cardiac muscle. J Physiol. 1961 Jul;157:335–350. doi: 10.1113/jphysiol.1961.sp006726. [DOI] [PMC free article] [PubMed] [Google Scholar]
- Hutter O. F., Warner A. E. The pH sensitivity of the chloride conductance of frog skeletal muscle. J Physiol. 1967 Apr;189(3):403–425. doi: 10.1113/jphysiol.1967.sp008176. [DOI] [PMC free article] [PubMed] [Google Scholar]
- Kenyon J. L., Gibbons W. R. Effects of low-chloride solutions on action potentials of sheep cardiac Purkinje fibers. J Gen Physiol. 1977 Nov;70(5):635–660. doi: 10.1085/jgp.70.5.635. [DOI] [PMC free article] [PubMed] [Google Scholar]
- Knauf P. A., Rothstein A. Chemical modification of membranes. I. Effects of sulfhydryl and amino reactive reagents on anion and cation permeability of the human red blood cell. J Gen Physiol. 1971 Aug;58(2):190–210. doi: 10.1085/jgp.58.2.190. [DOI] [PMC free article] [PubMed] [Google Scholar]
- LAMB J. F. The chloride content of rat auricle. J Physiol. 1961 Aug;157:415–425. doi: 10.1113/jphysiol.1961.sp006733. [DOI] [PMC free article] [PubMed] [Google Scholar]
- Ladle R. O., Walker J. L. Intracellular chloride activity in frog heart. J Physiol. 1975 Oct;251(2):549–559. doi: 10.1113/jphysiol.1975.sp011107. [DOI] [PMC free article] [PubMed] [Google Scholar]
- McAllister R. E., Noble D., Tsien R. W. Reconstruction of the electrical activity of cardiac Purkinje fibres. J Physiol. 1975 Sep;251(1):1–59. doi: 10.1113/jphysiol.1975.sp011080. [DOI] [PMC free article] [PubMed] [Google Scholar]
- Roos A., Boron W. F. Intracellular pH transients in rat diaphragm muscle measured with DMO. Am J Physiol. 1978 Jul;235(1):C49–C54. doi: 10.1152/ajpcell.1978.235.1.C49. [DOI] [PubMed] [Google Scholar]
- Russell J. M., Boron W. F. Role of choloride transport in regulation of intracellular pH. Nature. 1976 Nov 4;264(5581):73–74. doi: 10.1038/264073a0. [DOI] [PubMed] [Google Scholar]
- Siegelbaum S. A., Tsien R. W., Kass R. S. Role of intracellular calcium in the transient outward current of calf Purkinje fibres. Nature. 1977 Oct 13;269(5629):611–613. doi: 10.1038/269611a0. [DOI] [PubMed] [Google Scholar]
- Thomas R. C. Comparison of the mechanisms controlling intracellular pH and sodium in snail neurones. Respir Physiol. 1978 Apr;33(1):63–73. doi: 10.1016/0034-5687(78)90085-3. [DOI] [PubMed] [Google Scholar]
- Thomas R. C. Construction and testing of recessed-tip pH-sensitive micro-electrodes [proceedings]. J Physiol. 1978 Apr;277:14P–15P. [PubMed] [Google Scholar]
- Thomas R. C. Intracellular pH of snail neurones measured with a new pH-sensitive glass mirco-electrode. J Physiol. 1974 Apr;238(1):159–180. doi: 10.1113/jphysiol.1974.sp010516. [DOI] [PMC free article] [PubMed] [Google Scholar]
- Thomas R. C. Ionic mechanism of the H+ pump in a snail neurone. Nature. 1976 Jul 1;262(5563):54–55. doi: 10.1038/262054a0. [DOI] [PubMed] [Google Scholar]
- Thomas R. C. The effect of carbon dioxide on the intracellular pH and buffering power of snail neurones. J Physiol. 1976 Mar;255(3):715–735. doi: 10.1113/jphysiol.1976.sp011305. [DOI] [PMC free article] [PubMed] [Google Scholar]
- Thomas R. C. The role of bicarbonate, chloride and sodium ions in the regulation of intracellular pH in snail neurones. J Physiol. 1977 Dec;273(1):317–338. doi: 10.1113/jphysiol.1977.sp012096. [DOI] [PMC free article] [PubMed] [Google Scholar]
- Vaughan-Jones R. D. Effect of bicarbonate and SITS on alphaiCl recovery in sheep cardiac Purkinje fibres [proceedings]. J Physiol. 1978 Sep;282:22P–23P. [PubMed] [Google Scholar]
- Vaughan-Jones R. D. Non-passive chloride distribution in mammalian heart muscle: micro-electrode measurement of the intracellular chloride activity. J Physiol. 1979 Oct;295:83–109. doi: 10.1113/jphysiol.1979.sp012956. [DOI] [PMC free article] [PubMed] [Google Scholar]
- Wiggins J. R., Cranefield P. F. Two levels of resting potential in canine cardiac Purkinje fibers exposed to sodium-free solutions. Circ Res. 1976 Oct;39(4):466–474. doi: 10.1161/01.res.39.4.466. [DOI] [PubMed] [Google Scholar]