Abstract
1. A double-barrelled potassium-sensitive micro-electrode was developed that was fine enough to record intracellular electrical potentials and potassium activities (aK) in the drone retina. 2. aK was measured in the photoreceptor cells, in the pigment (glial) cells, and in the extracellular space, in the superfused, cut, retina. The effect of photostimulation was studied: 20 msec light flashes, intense enough to evoke receptor potentials of maximum amplitude were presented, 1/sec, in a train lasting about 2 min. 3. In photoreceptors with membrane potentials greater than or equal to 50 mV aK in the dark was 79 mM, S.D. = 27 mM, n = 11. During photostimulation aK fell by 21.5 +/- 9.5 mM with a half-time of 30 +/- 22 sec. (A tentative conversion from activities to free concentrations can be made by taking the activity coefficient as 0.70 its value in the Ringer solution). 4. In pigment cells with membrane potentials greater than or equal to 50 mV, aK in the dark was 52 mM, S.D. = 13 mM, n = 11. During photostimulation aK increased by 14 +/- 5 mM. 5. In the extracellular space aK increased during photostimulation with a mean half-time of less than 1.3 sec to a maximum (mean value 14 mM, S.D. = 8.4 mM, n = 22), and then fell to a plateau. 6. It is estimated from the anatomy that the photoreceptors occupy approximately 38% of the total volume of the retina, the pigment cells 57%, and extracellular space 5%. Hence, it seems possible that during photostimulation nearly all the net loss of potassium from the photoreceptors is temporarily stored in the pigment cells. 7. Recordings were made in the extracellular space of the intact animal by passing the electrode through a hole in the cornea. The mean aK in the dark was 7.7 mM, S.E. = 0.4 mM, n = 22. In the superfused retina, aK in the dark was 6.3 mM, S.E. = 0.7 mM, n = 22, even though aK in the Ringer solution was 2.2 mM. Increasing the aK of the Ringer solution to 7.0 mM had no apparent effect on aK in the extracellular space at depths greater than 20 micron. 8. In the intact animal the amplitude and time course of the change in extracellular aK evoked by the standard pattern of photostimulation were within the range observed in the superfused preparation.
Full text
PDF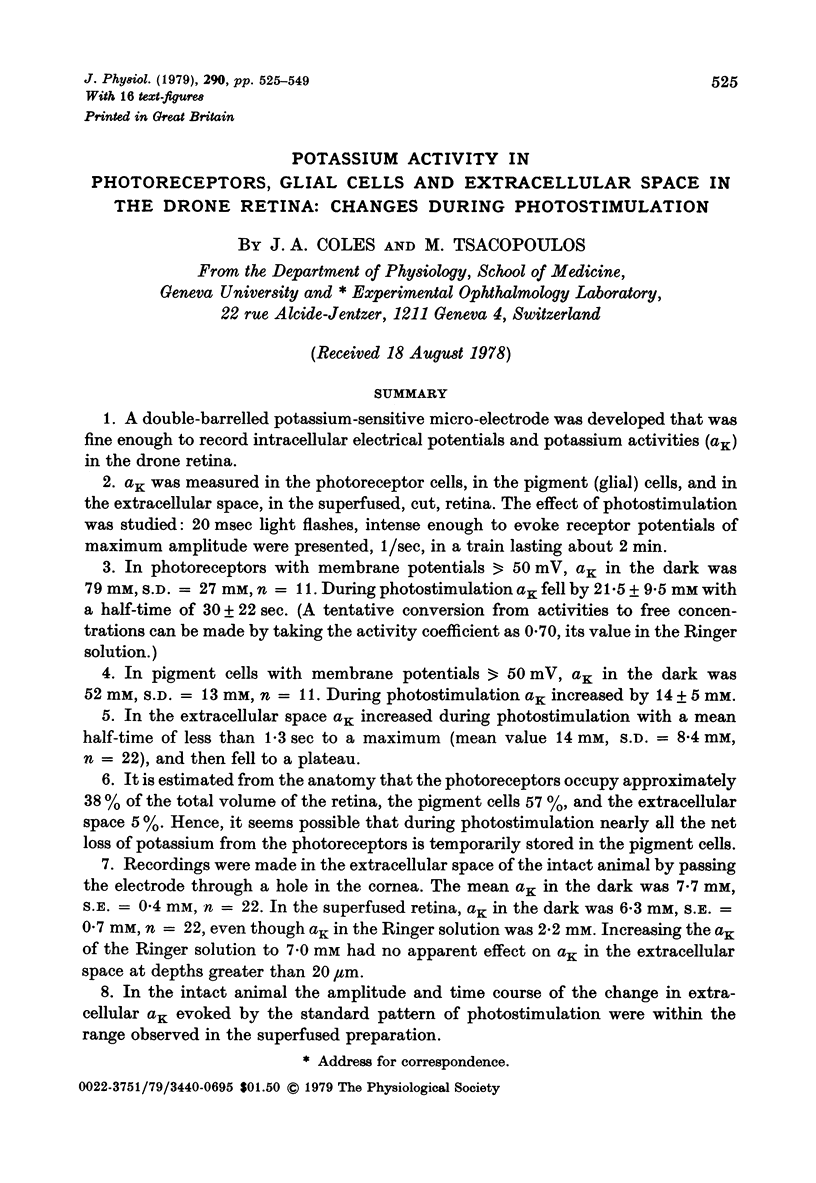
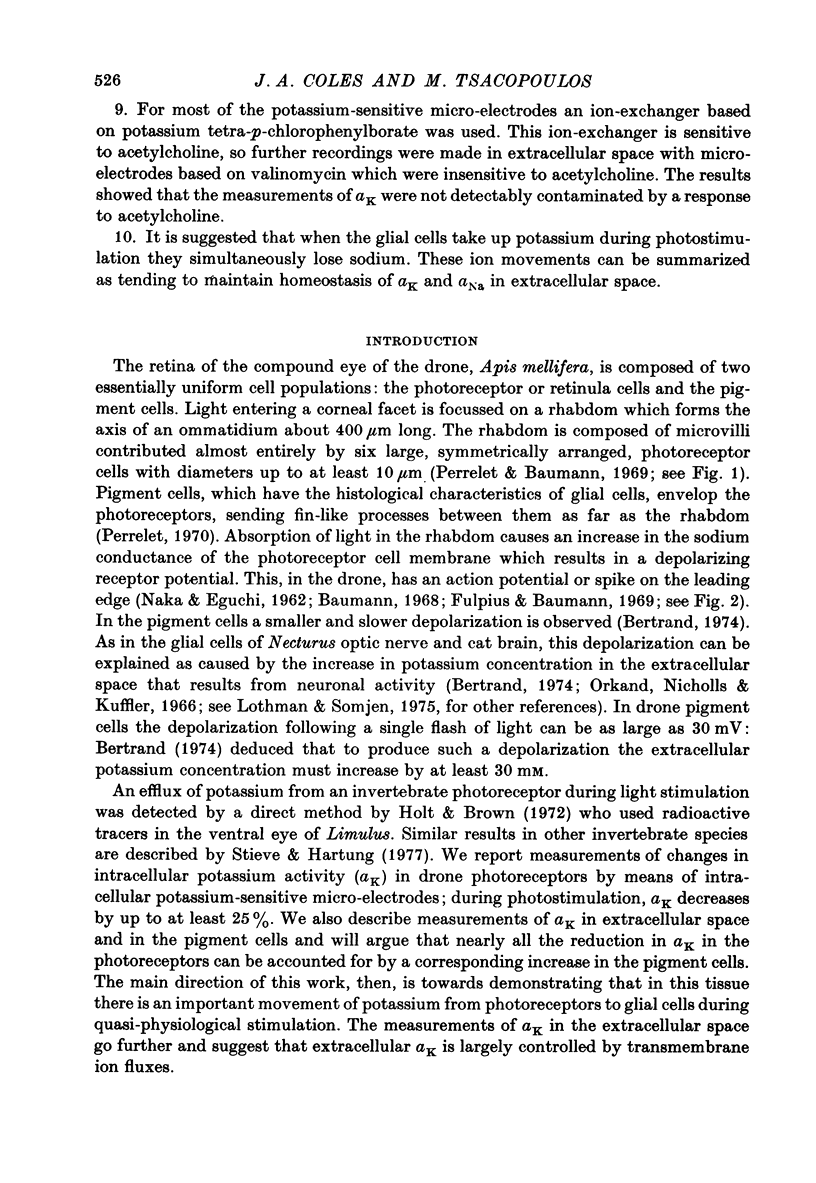
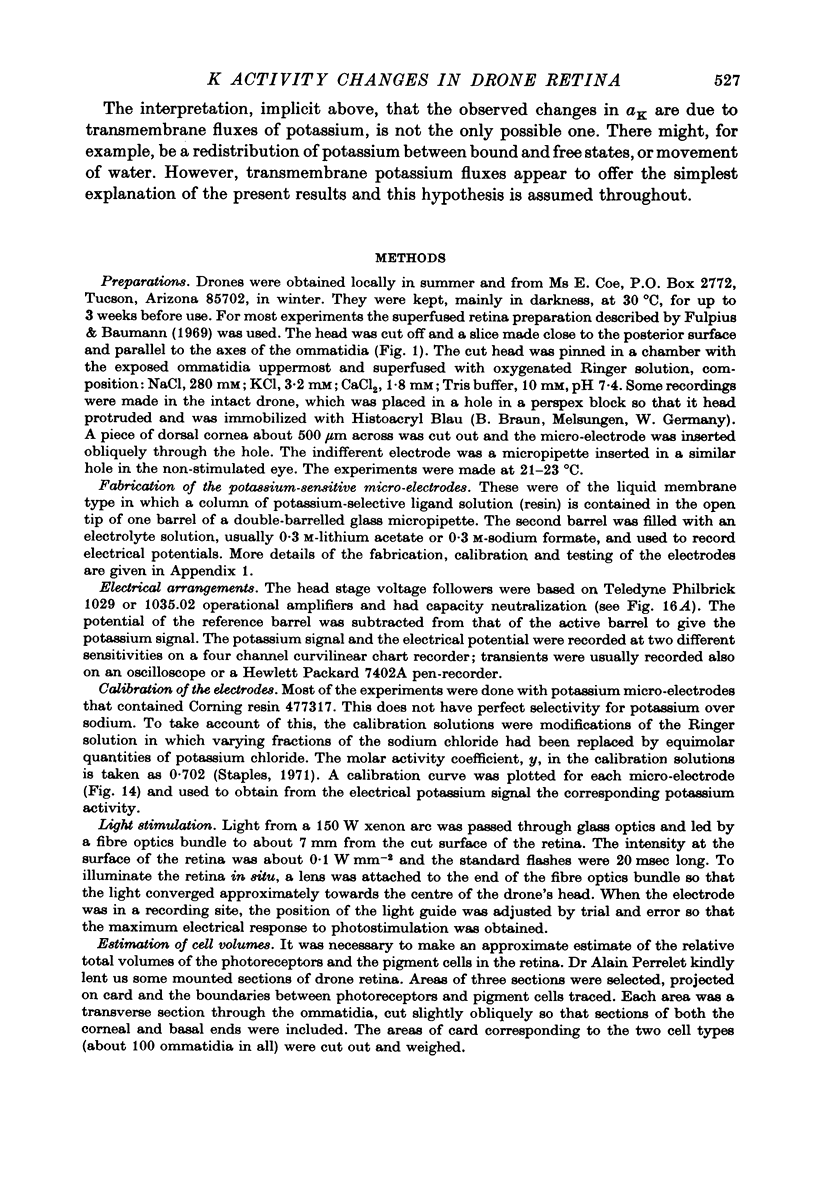
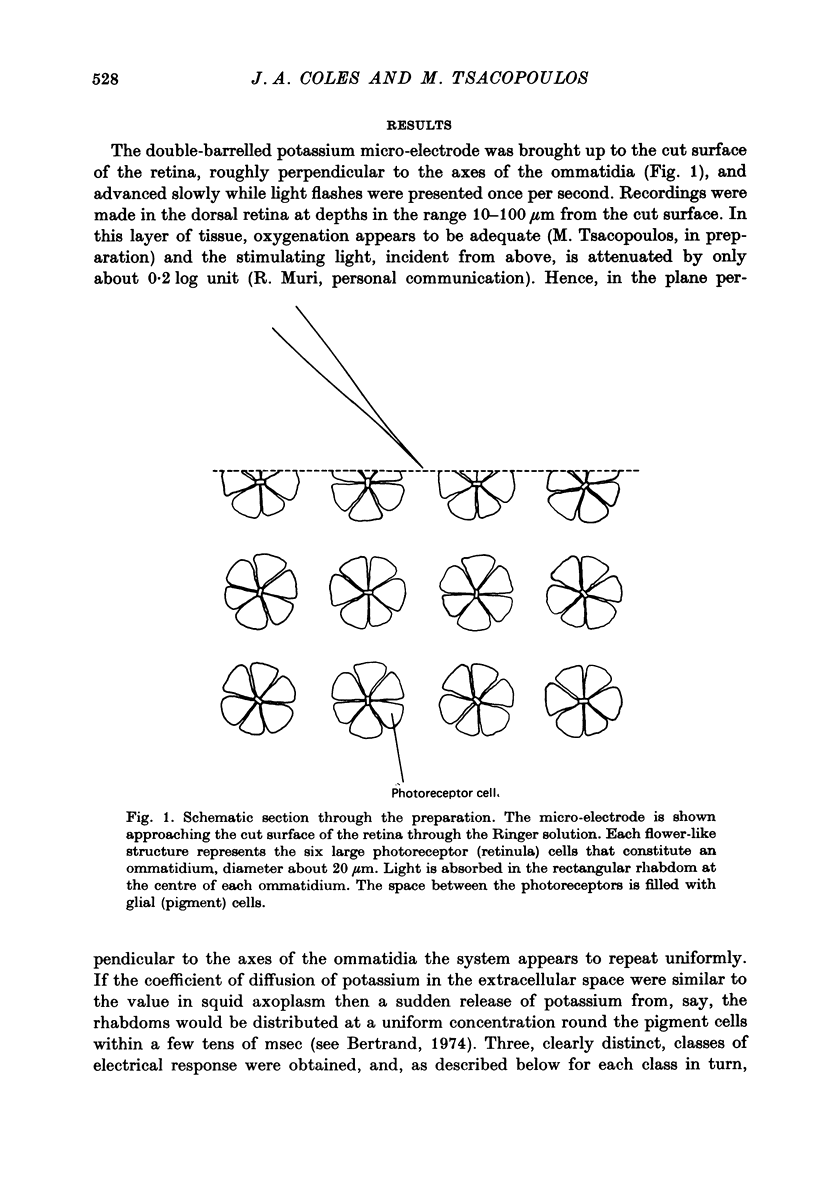
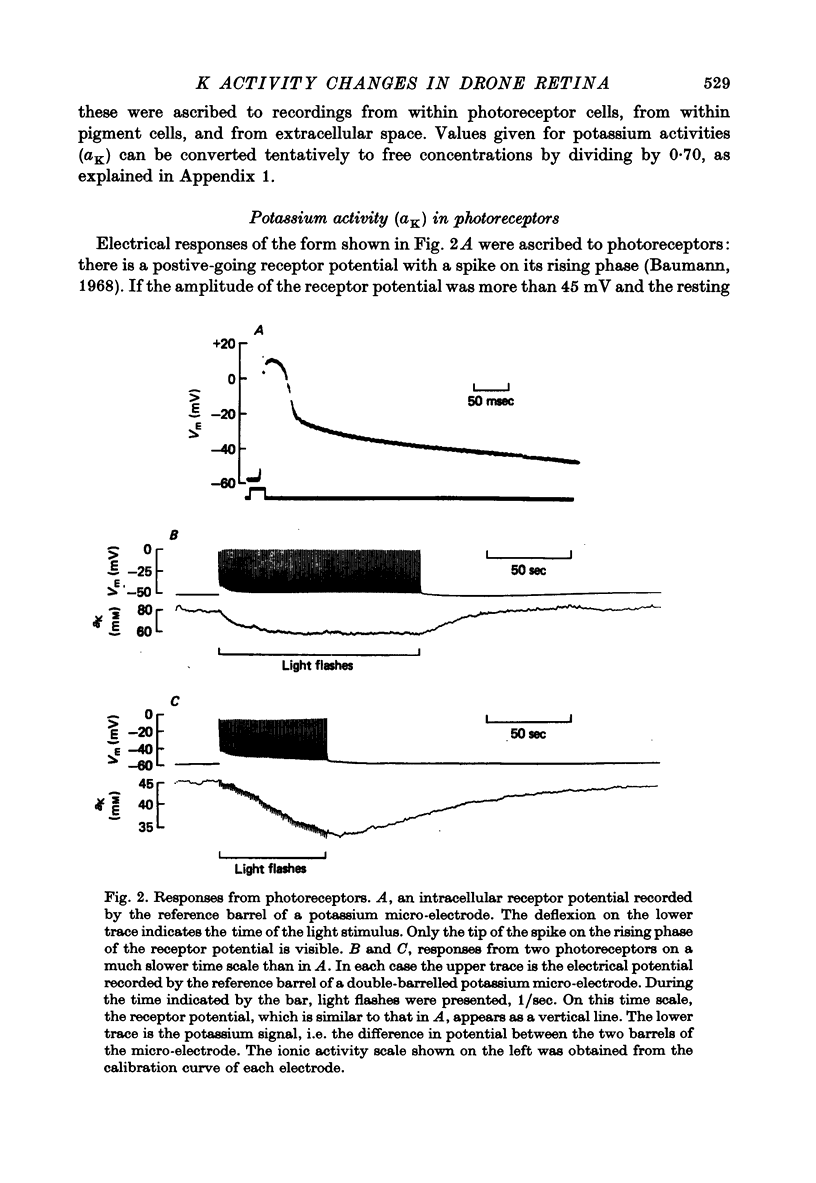
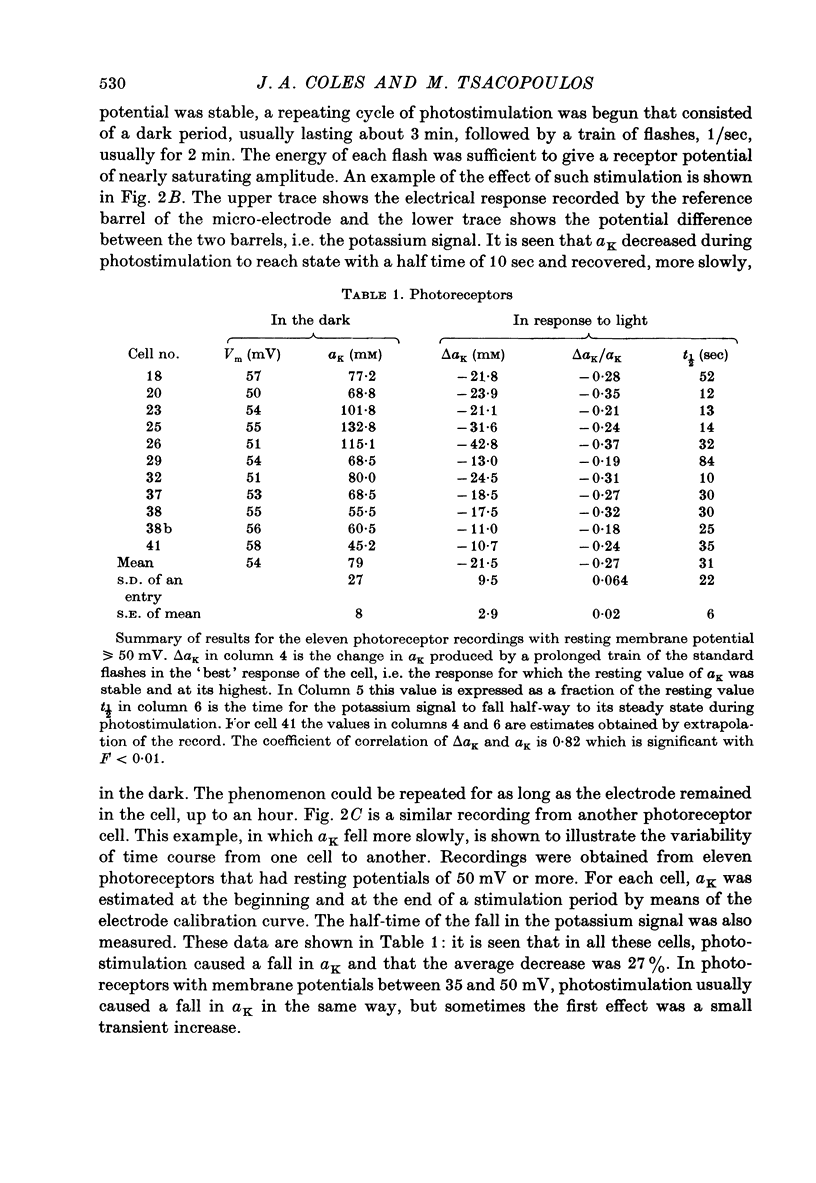
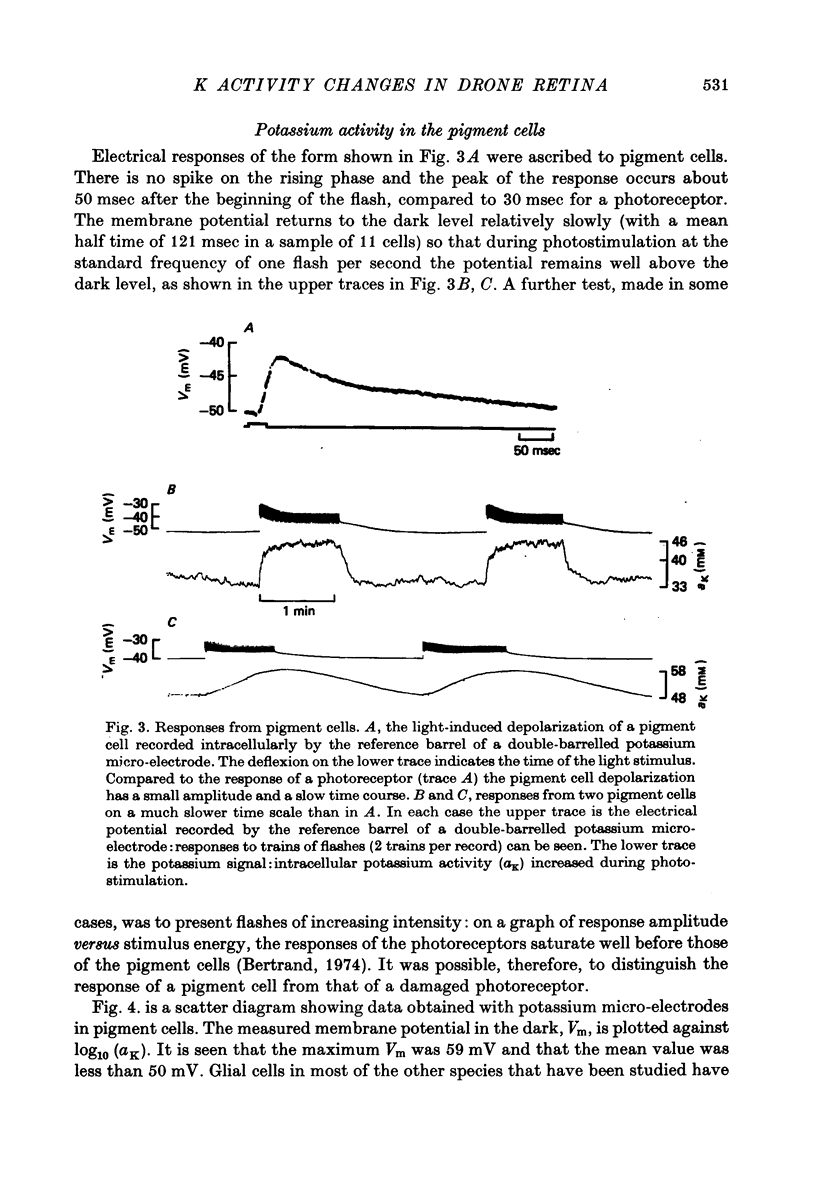
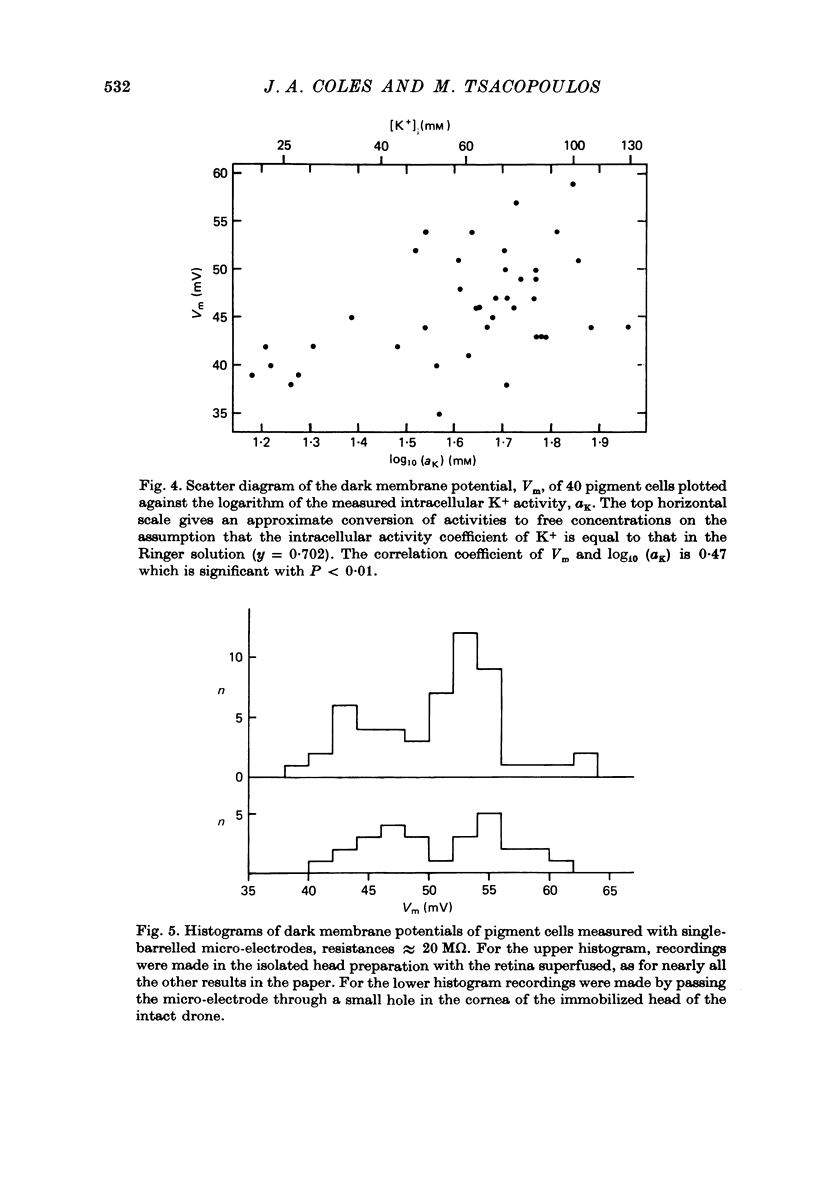
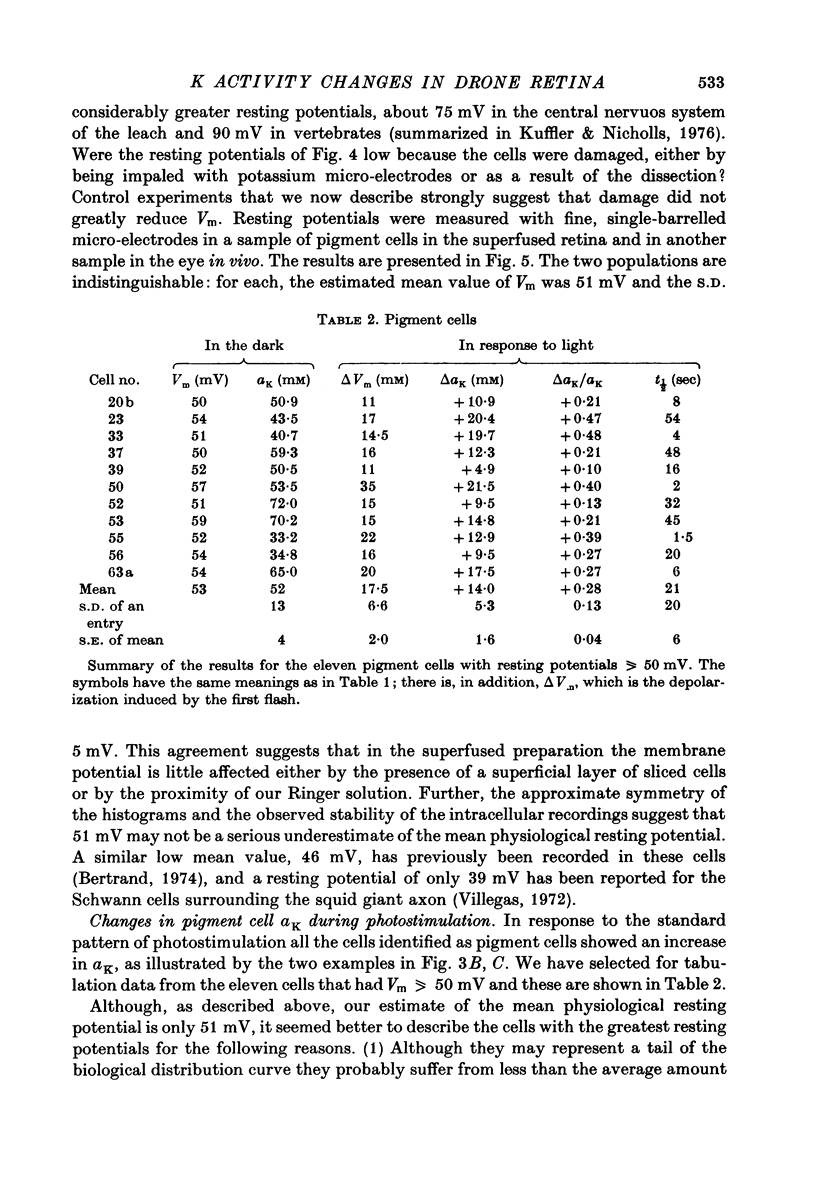
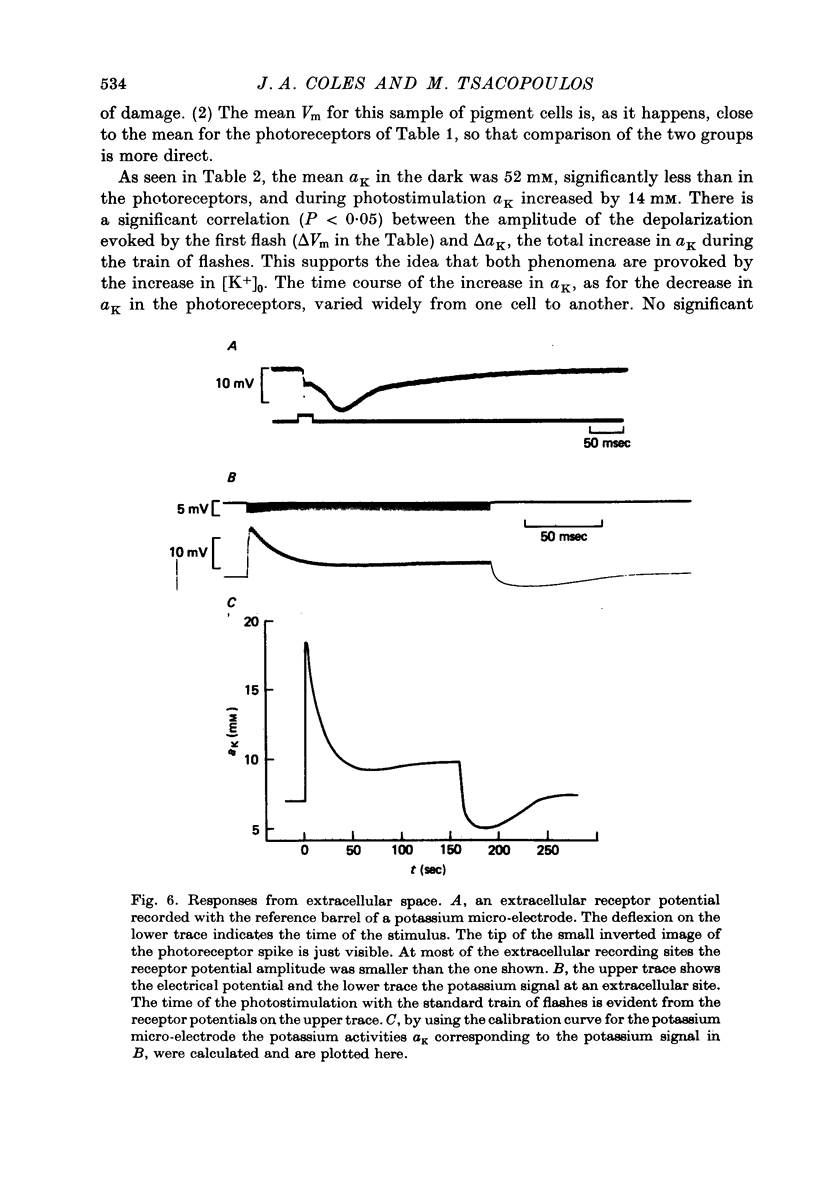
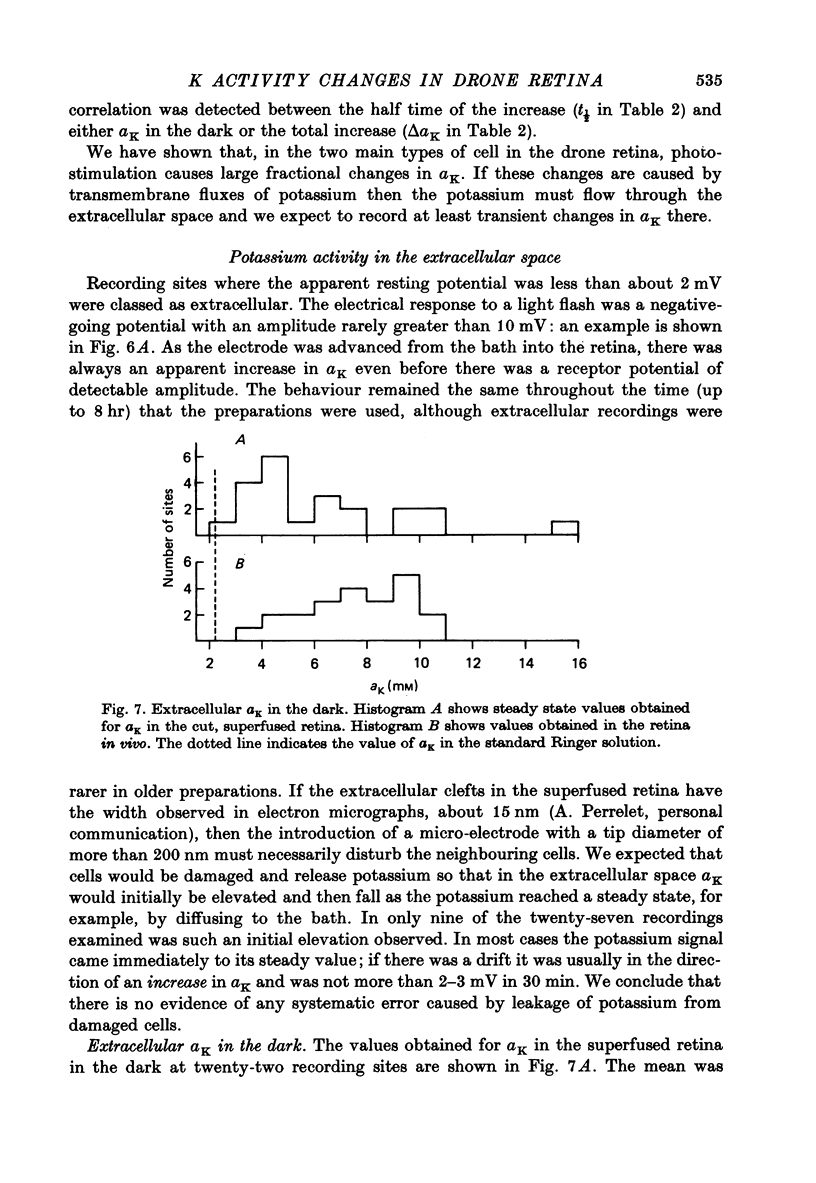
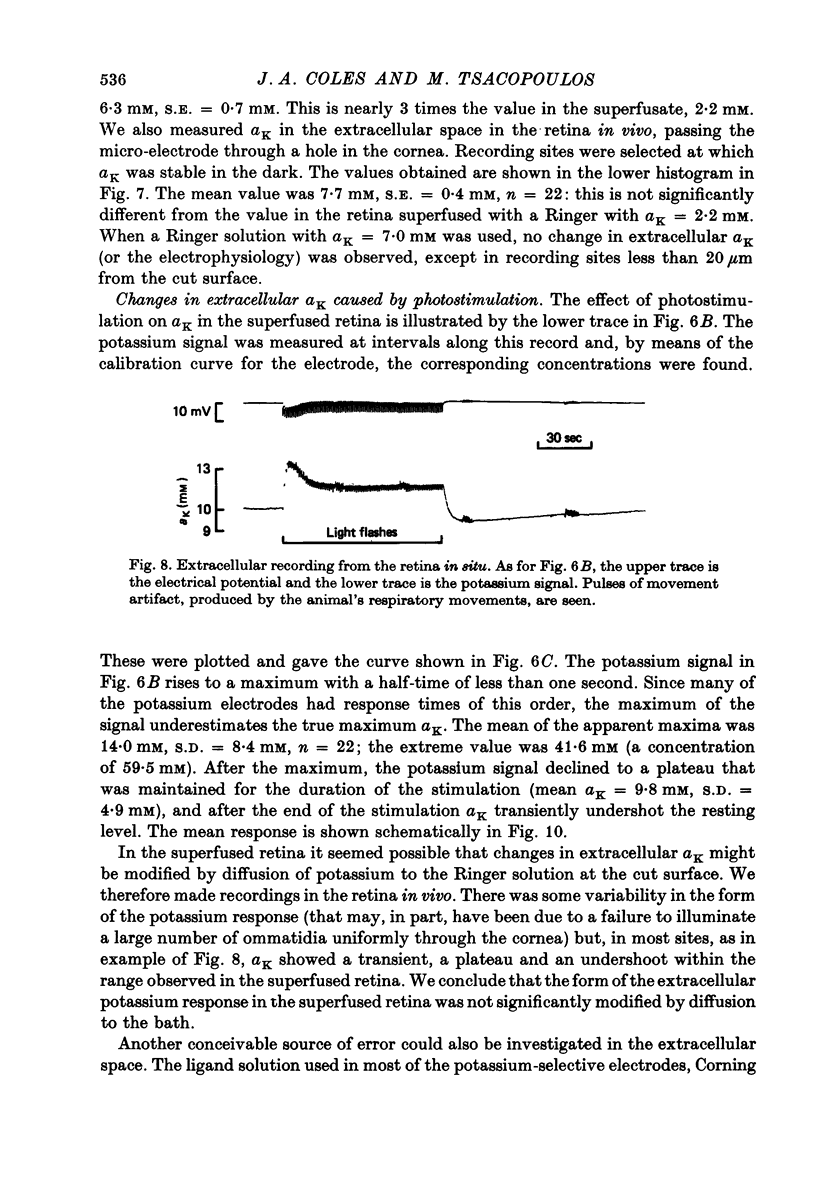
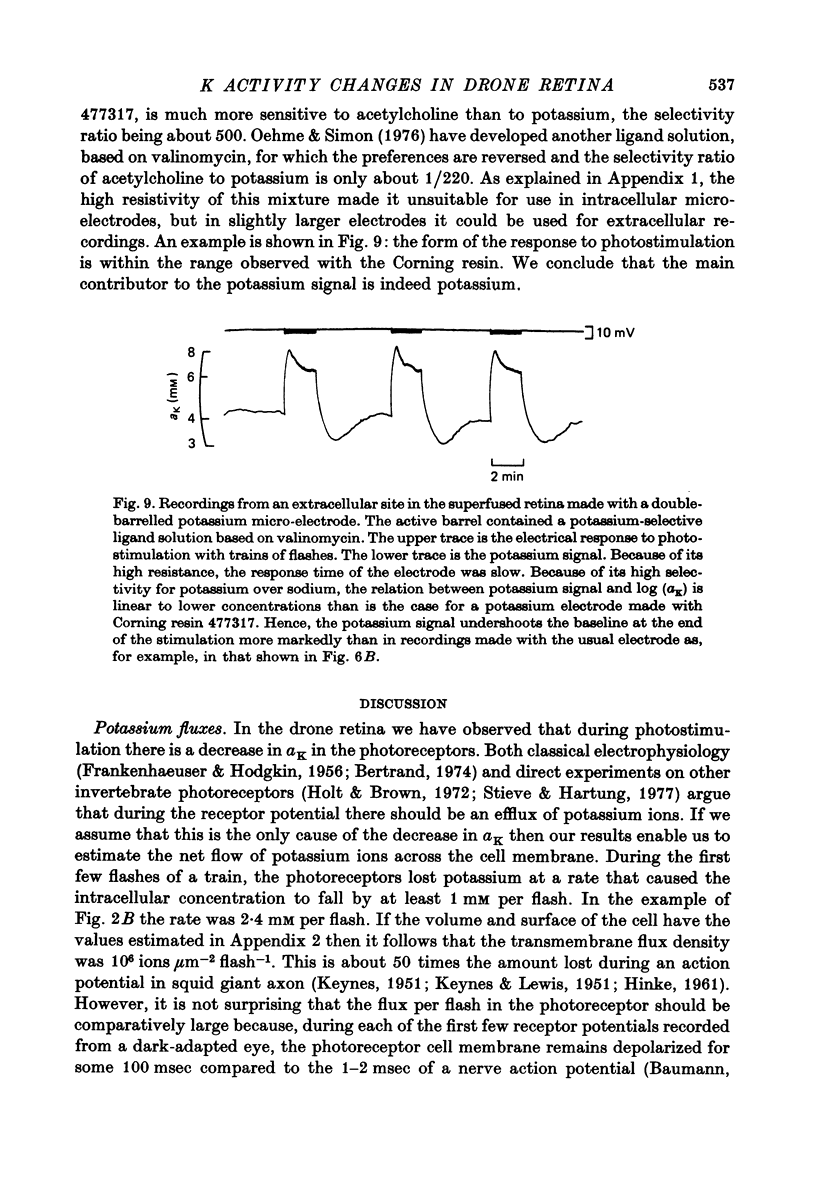
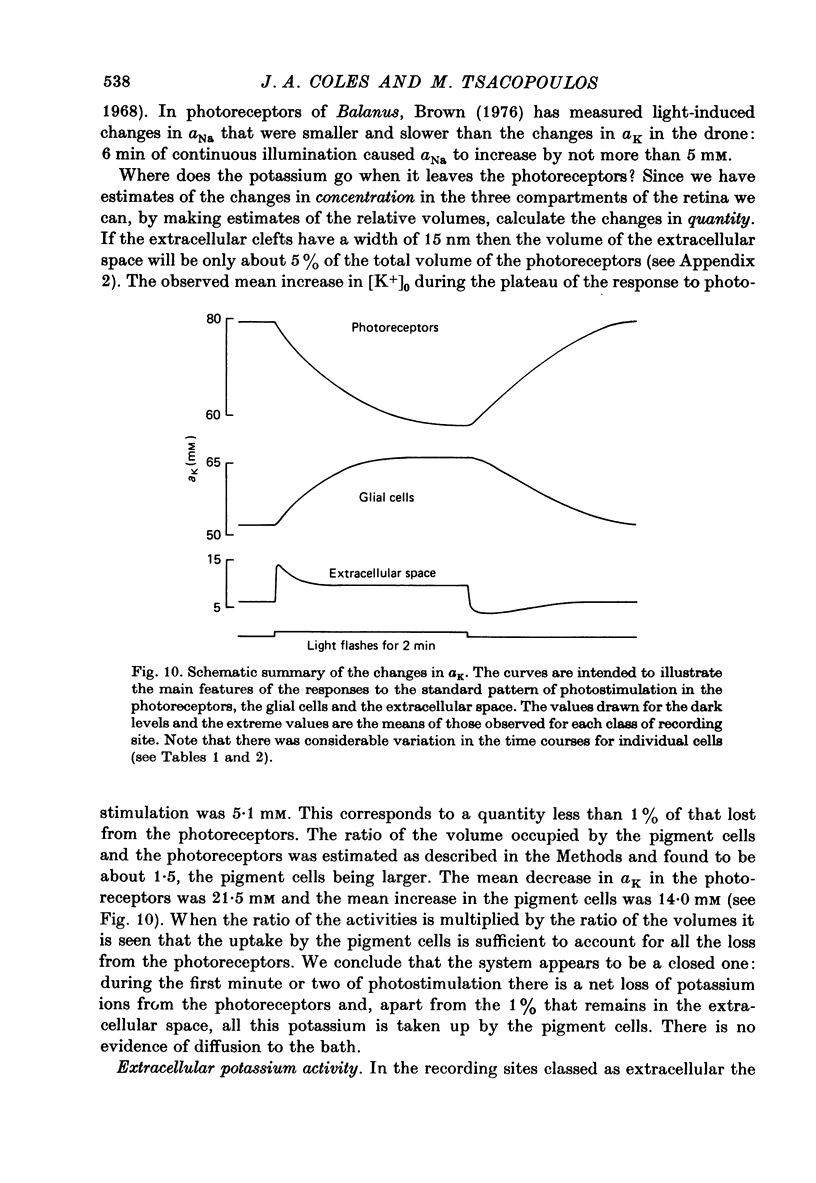
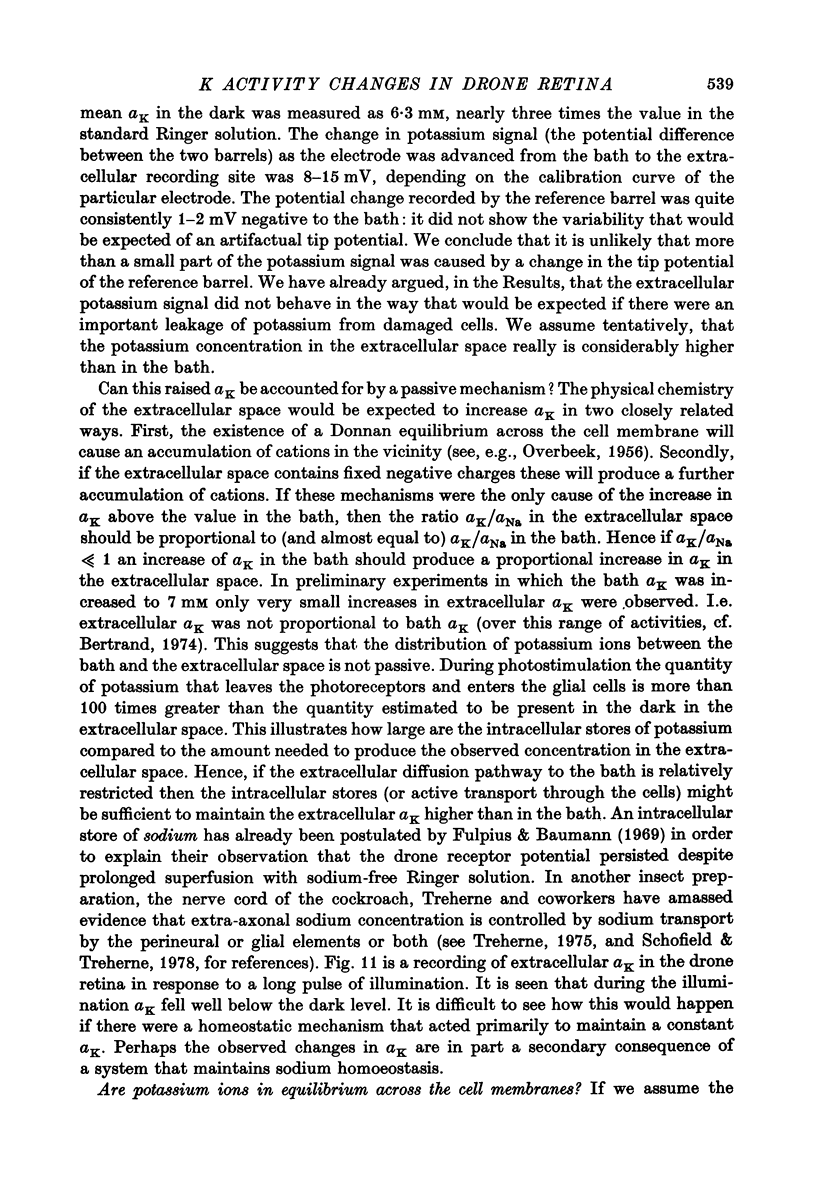
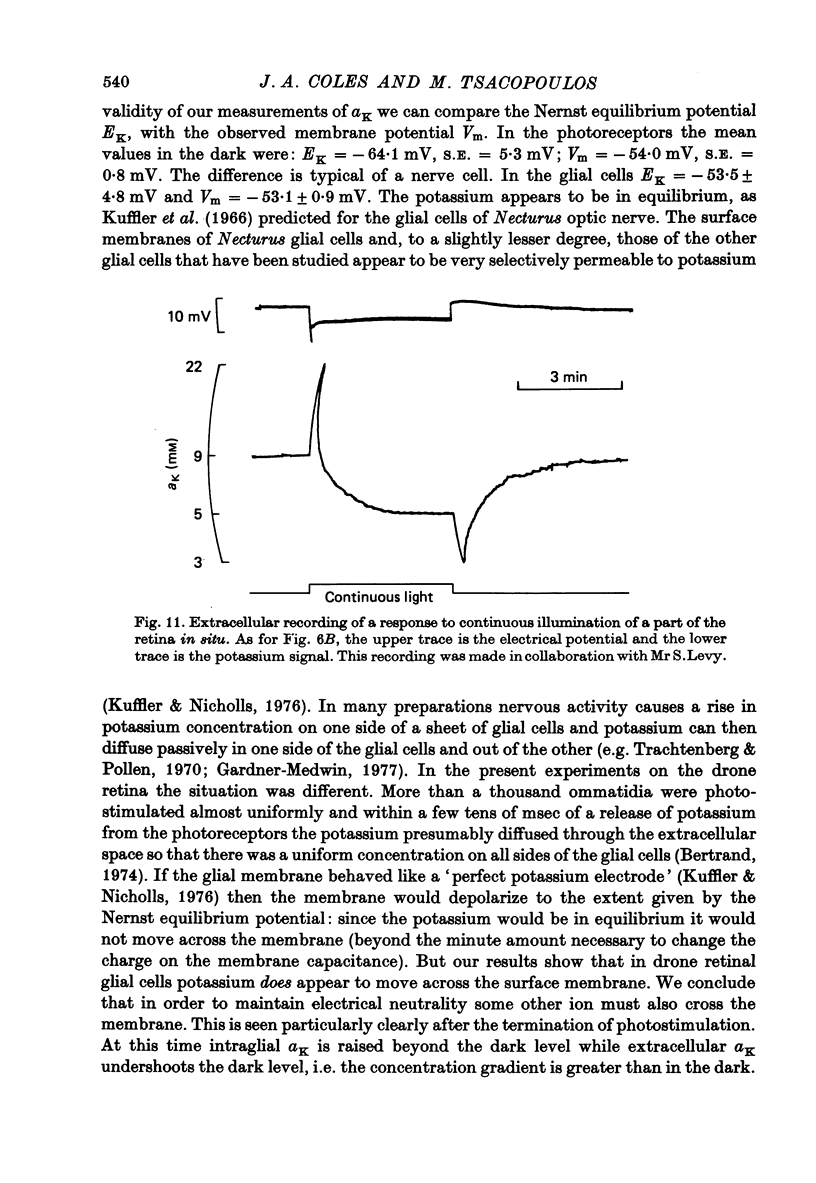
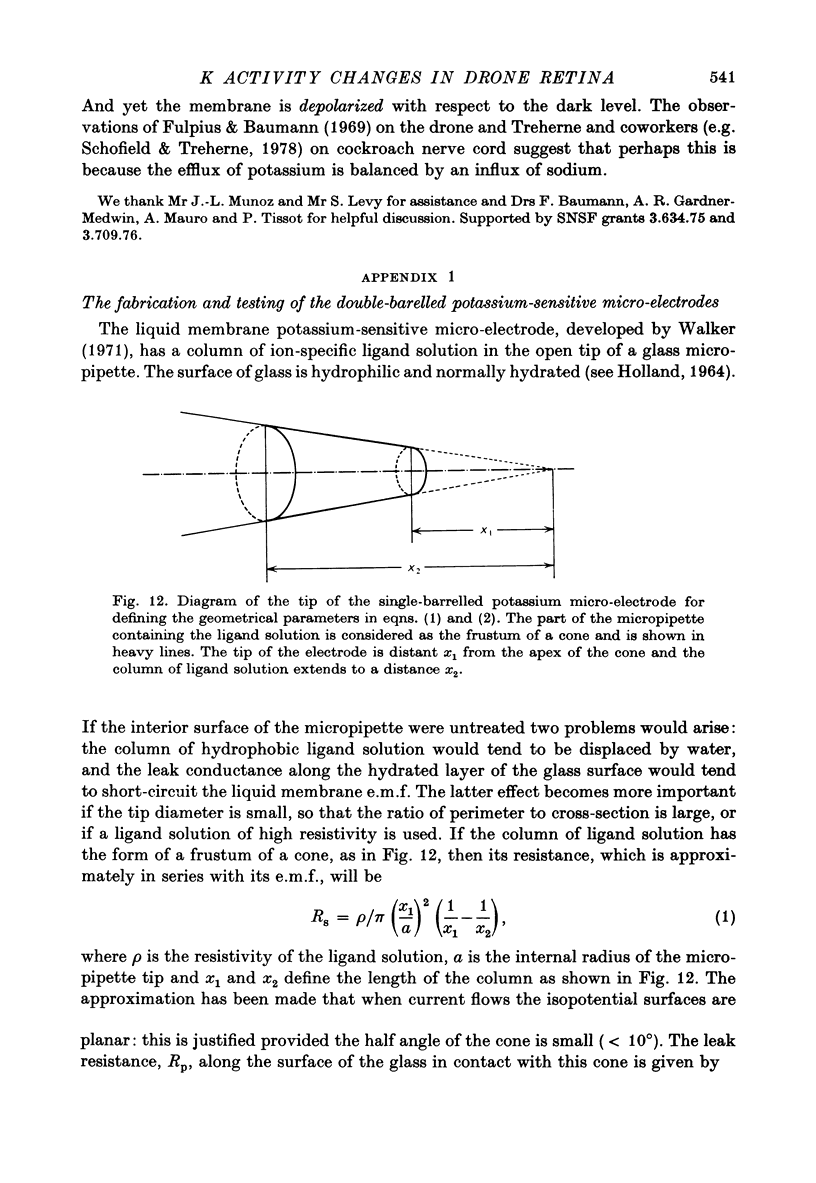
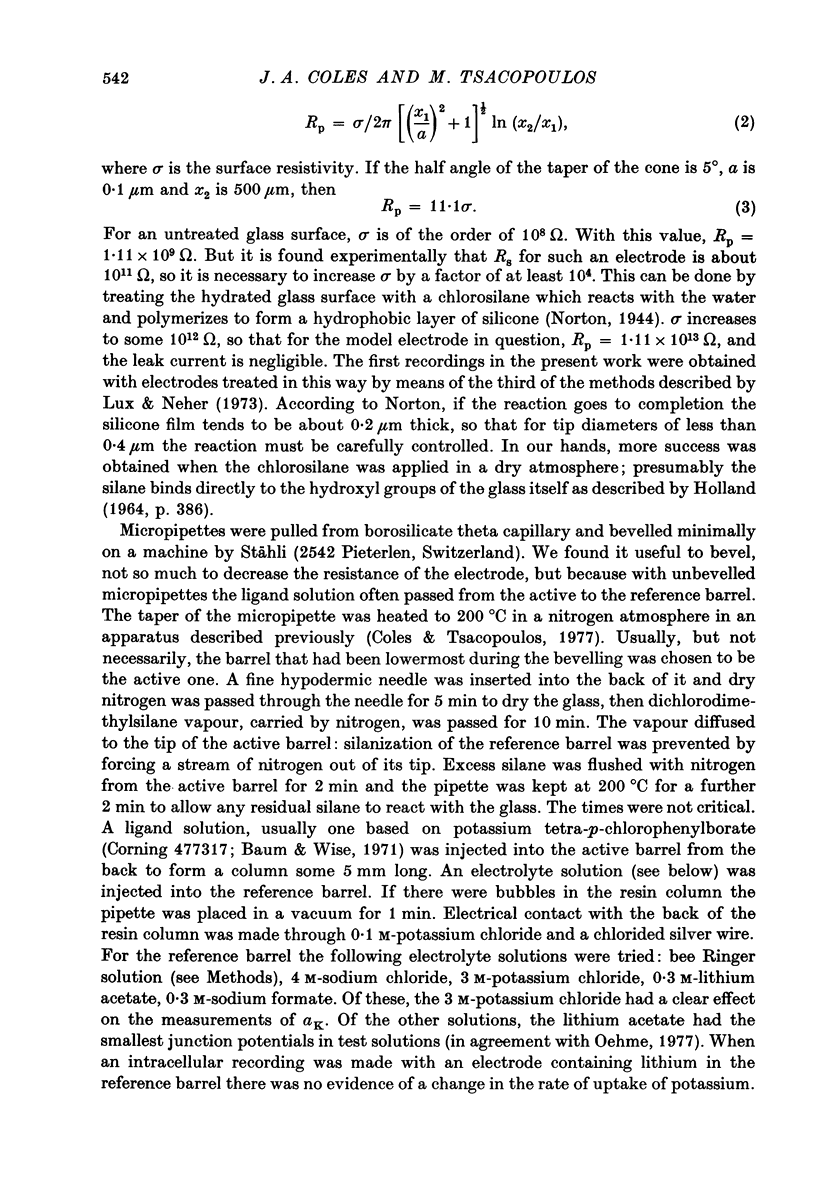
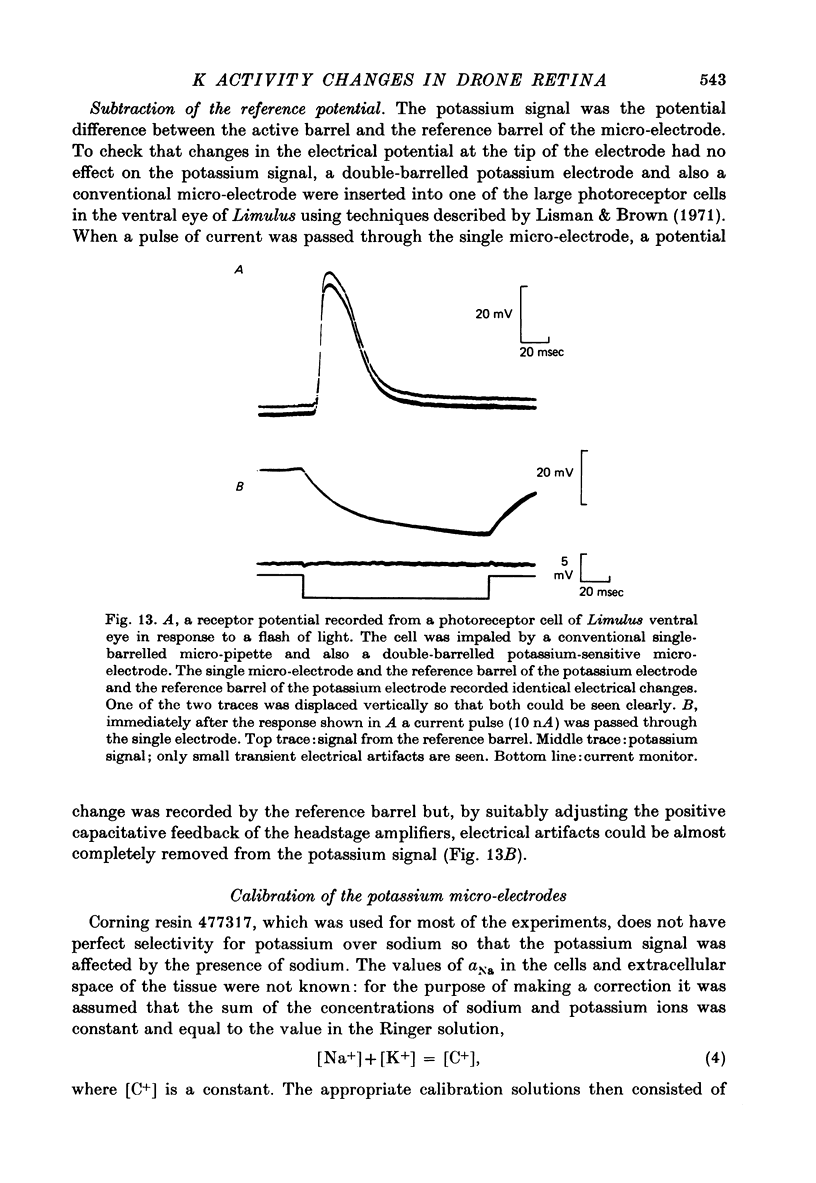
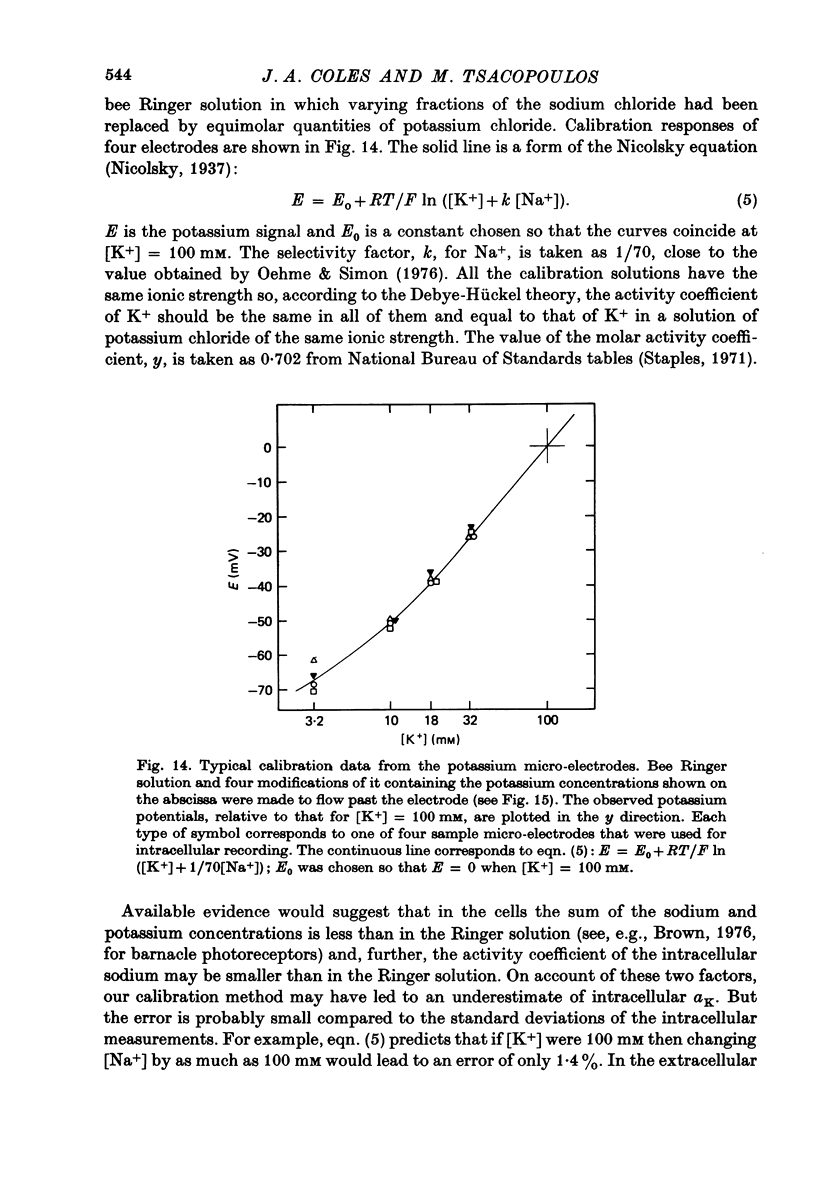
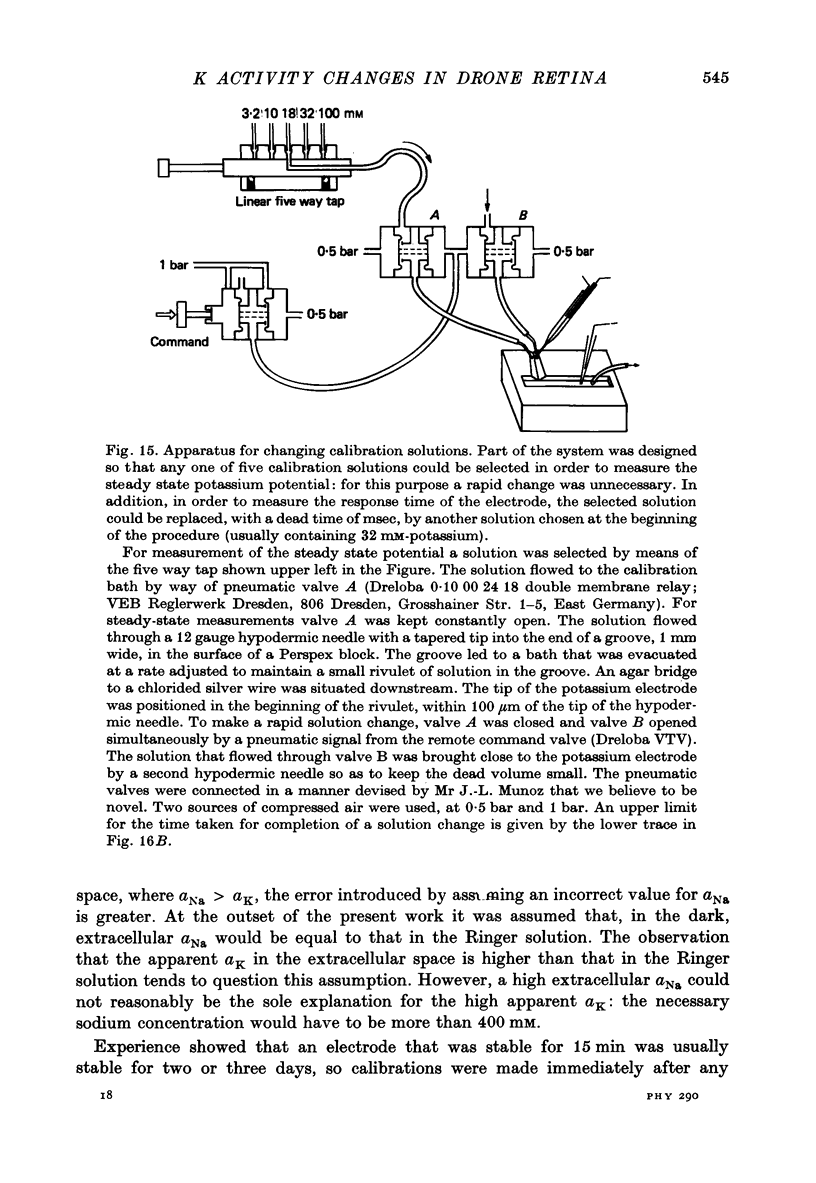
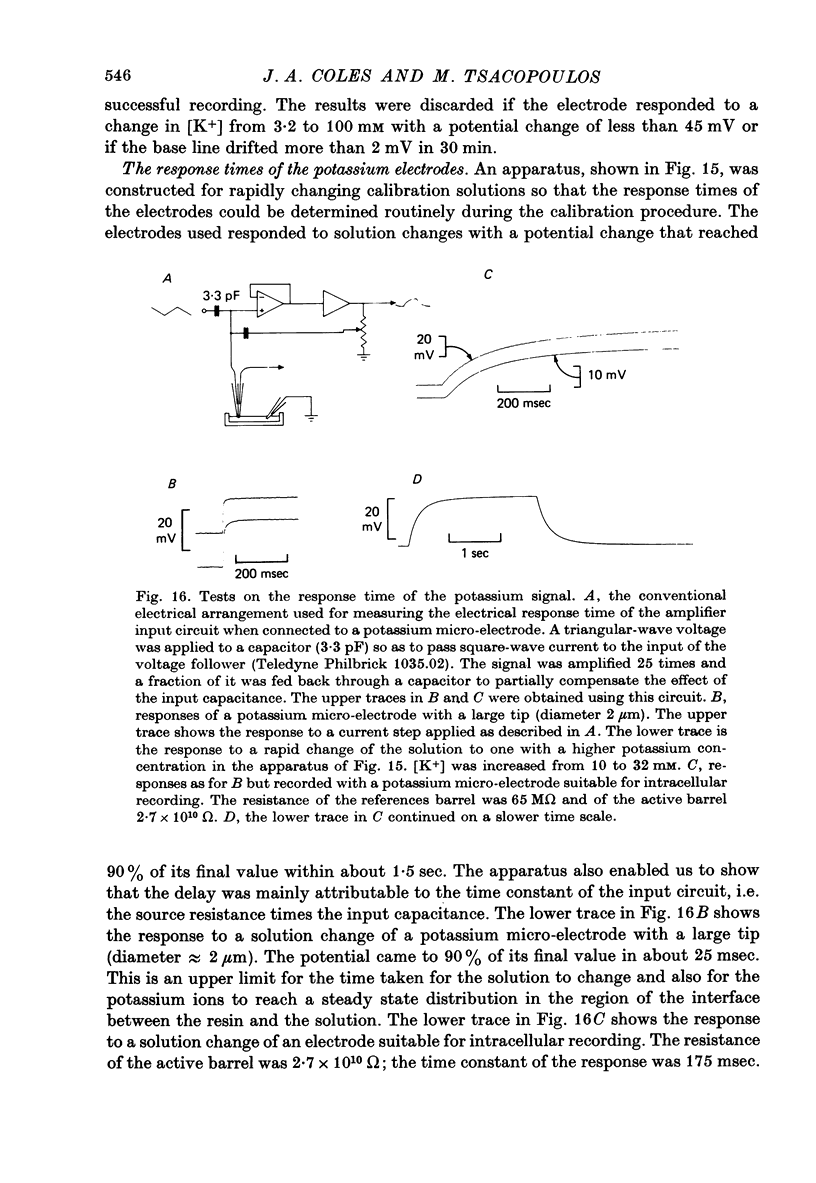
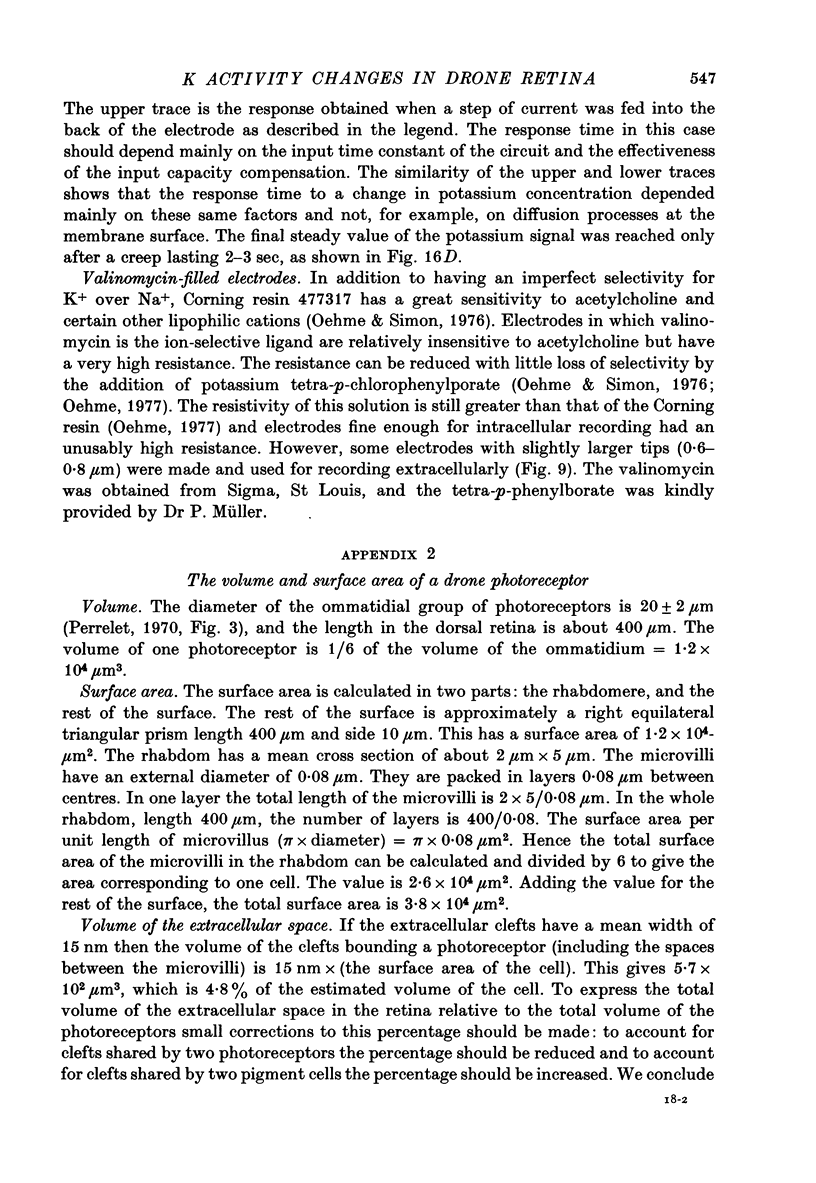
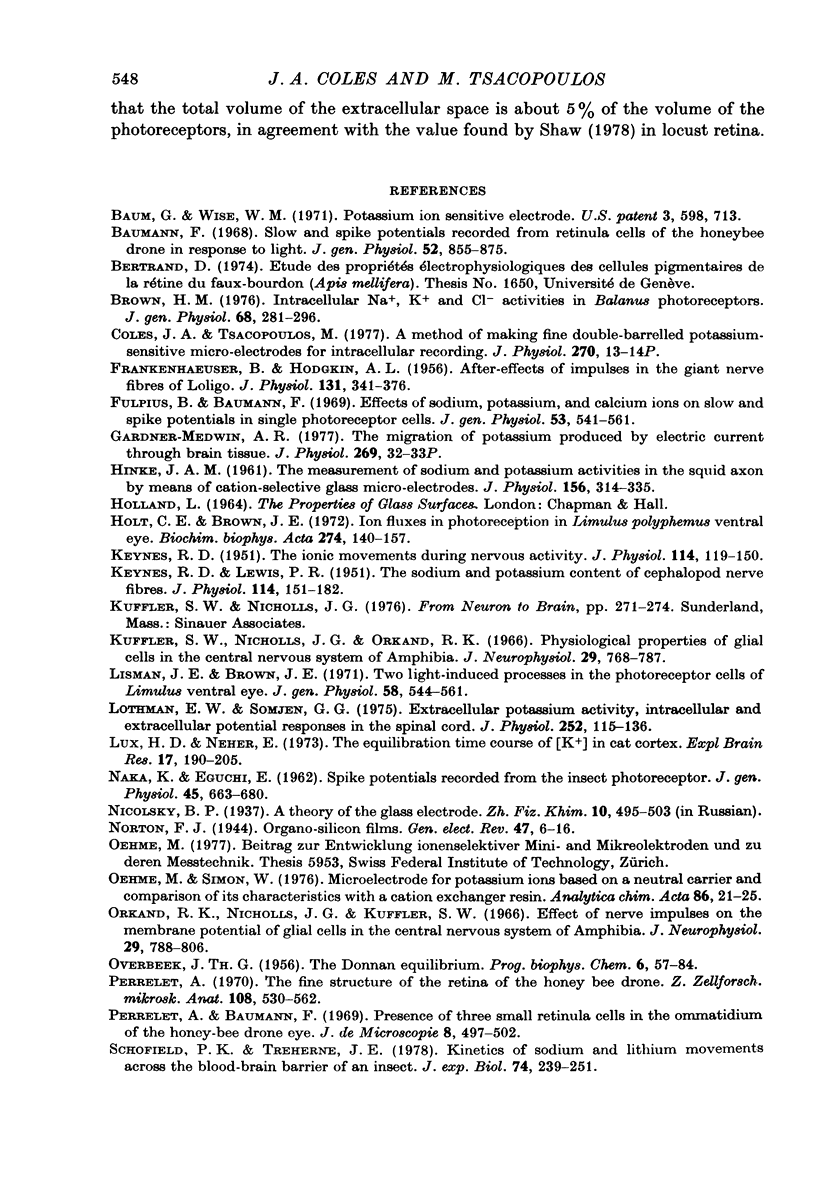
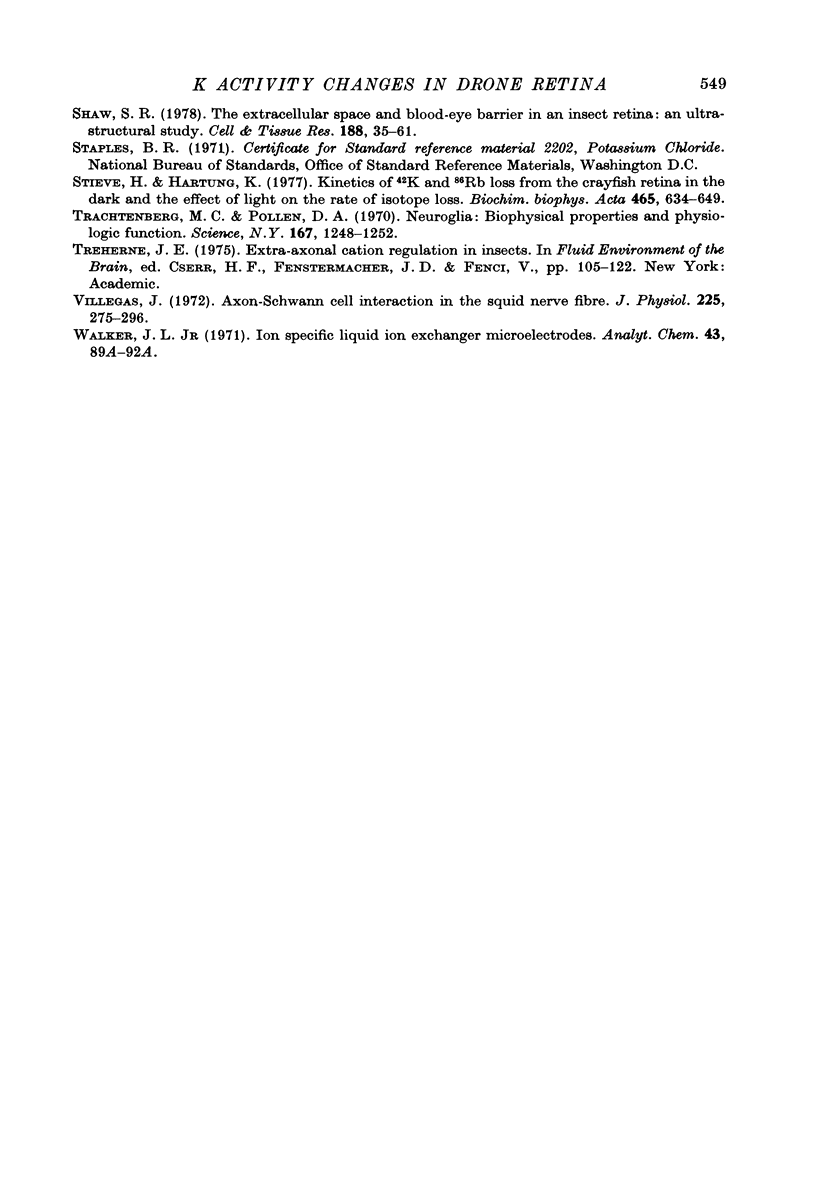
Selected References
These references are in PubMed. This may not be the complete list of references from this article.
- Baumann F. Slow and spike potentials recorded from retinula cells of the honeybee drone in response to light. J Gen Physiol. 1968 Dec;52(6):855–875. doi: 10.1085/jgp.52.6.855. [DOI] [PMC free article] [PubMed] [Google Scholar]
- Brown H. M. Intracellular Na+, K+, and C1- activities in Balanus photoreceptors. J Gen Physiol. 1976 Sep;68(3):281–296. doi: 10.1085/jgp.68.3.281. [DOI] [PMC free article] [PubMed] [Google Scholar]
- Coles J. A., Tsacopoulos M. A method of making fine double-barrelled potassium-sensitive micro-electrodes for intracellular recording [proceedings]. J Physiol. 1977 Aug;270(1):12P–14P. [PubMed] [Google Scholar]
- FRANKENHAEUSER B., HODGKIN A. L. The after-effects of impulses in the giant nerve fibres of Loligo. J Physiol. 1956 Feb 28;131(2):341–376. doi: 10.1113/jphysiol.1956.sp005467. [DOI] [PMC free article] [PubMed] [Google Scholar]
- Fulpius B., Baumann F. Effects of sodium, potassium, and calcium ions on slow and spike potentials in single photoreceptor cells. J Gen Physiol. 1969 May;53(5):541–561. doi: 10.1085/jgp.53.5.541. [DOI] [PMC free article] [PubMed] [Google Scholar]
- Gardner-Medwin A. R. The migration of potassium produced by electric current through brain tissue [proceedings]. J Physiol. 1977 Jul;269(1):32P–33P. [PubMed] [Google Scholar]
- HINKE J. A. The measurement of sodium and potassium activities in the squid axon by means of cation-selective glass micro-electrodes. J Physiol. 1961 Apr;156:314–335. doi: 10.1113/jphysiol.1961.sp006678. [DOI] [PMC free article] [PubMed] [Google Scholar]
- Holt C. E., Brown J. E. Ion fluxes in photoreception in Limulus polyphemus ventral eye. I. The response of potassium efflux to light. Biochim Biophys Acta. 1972 Jul 3;274(1):140–157. doi: 10.1016/0005-2736(72)90289-1. [DOI] [PubMed] [Google Scholar]
- KEYNES R. D., LEWIS P. R. The sodium and potassium content of cephalopod nerve fibers. J Physiol. 1951 Jun;114(1-2):151–182. doi: 10.1113/jphysiol.1951.sp004609. [DOI] [PMC free article] [PubMed] [Google Scholar]
- KEYNES R. D. The ionic movements during nervous activity. J Physiol. 1951 Jun;114(1-2):119–150. doi: 10.1113/jphysiol.1951.sp004608. [DOI] [PMC free article] [PubMed] [Google Scholar]
- Kuffler S. W., Nicholls J. G., Orkand R. K. Physiological properties of glial cells in the central nervous system of amphibia. J Neurophysiol. 1966 Jul;29(4):768–787. doi: 10.1152/jn.1966.29.4.768. [DOI] [PubMed] [Google Scholar]
- Lisman J. E., Brown J. E. Two light-induced processes in the photoreceptor cells of Limulus ventral eye. J Gen Physiol. 1971 Nov;58(5):544–561. doi: 10.1085/jgp.58.5.544. [DOI] [PMC free article] [PubMed] [Google Scholar]
- Lothman E. W., Somjen G. G. Extracellular potassium activity, intracellular and extracellular potential responses in the spinal cord. J Physiol. 1975 Oct;252(1):115–136. doi: 10.1113/jphysiol.1975.sp011137. [DOI] [PMC free article] [PubMed] [Google Scholar]
- Lux H. D., Neher E. The equilibration time course of (K + ) 0 in cat cortex. Exp Brain Res. 1973 Apr 30;17(2):190–205. doi: 10.1007/BF00235028. [DOI] [PubMed] [Google Scholar]
- NAKA K. I., EGUCHI E. Spike potentials recorded from the insect photoreceptor. J Gen Physiol. 1962 Mar;45:663–680. doi: 10.1085/jgp.45.4.663. [DOI] [PMC free article] [PubMed] [Google Scholar]
- OVERBEEK J. T. The Donnan equilibrium. Prog Biophys Biophys Chem. 1956;6:57–84. [PubMed] [Google Scholar]
- Orkand R. K., Nicholls J. G., Kuffler S. W. Effect of nerve impulses on the membrane potential of glial cells in the central nervous system of amphibia. J Neurophysiol. 1966 Jul;29(4):788–806. doi: 10.1152/jn.1966.29.4.788. [DOI] [PubMed] [Google Scholar]
- Perrelet A. The fine structure of the retina of the honey bee drone. An electron microscopical study. Z Zellforsch Mikrosk Anat. 1970;108(4):530–562. doi: 10.1007/BF00339658. [DOI] [PubMed] [Google Scholar]
- Schofield P. K., Treherne J. E. Kinetics of sodium and lithium movements across the blood-brain barrier of an insect. J Exp Biol. 1978 Jun;74:239–251. doi: 10.1242/jeb.74.1.239. [DOI] [PubMed] [Google Scholar]
- Shaw S. R. The extracellular space and blood-eye barrier in an insect retina: an ultrastructural study. Cell Tissue Res. 1978 Mar 31;188(1):35–61. doi: 10.1007/BF00220513. [DOI] [PubMed] [Google Scholar]
- Stieve H., Hartung K. Kinetics of 42K and 86Rb loss from the crayfish retina in the dark and the effect of light on the rate of isotope loss. Biochim Biophys Acta. 1977 Mar 17;465(3):634–649. doi: 10.1016/0005-2736(77)90279-6. [DOI] [PubMed] [Google Scholar]
- Trachtenberg M. C., Pollen D. A. Neuroglia: biophysical properties and physiologic function. Science. 1970 Feb 27;167(3922):1248–1252. doi: 10.1126/science.167.3922.1248. [DOI] [PubMed] [Google Scholar]
- Villegas J. Axon-Schwann cell interaction in the squid nerve fibre. J Physiol. 1972 Sep;225(2):275–296. doi: 10.1113/jphysiol.1972.sp009940. [DOI] [PMC free article] [PubMed] [Google Scholar]