Abstract
The whole-cell voltage-clamp technique employing electrolyte-filled micro-pipette suction electrodes is widely used to investigate questions requiring an electrophysiological approach. With this technique, the ionic composition of the cytosol is assumed to be strongly influenced (as result of diffusion) by the ionic composition of the solution contained in the electrode. If this assumption is valid for isolated cardiac myocytes, the technique would be particularly powerful for studying the dependence of their Na,K-pump on the intracellular [Na+]. However, the relationship between the concentrations of ions in the solution filling the electrode and those in the cytosol has not been established. The relationship was investigated to determine in particular whether the [Na+] at the intracellular cation ligand binding sites for the Na-pump ([ Na+]ps) can be set and clamped by [Na+] in the pipette electrode ([ Na+]pip). If [Na+]pip can set and clamp [Na+]ps, this would provide a means for defining the dependence of the Na,K-pump on intracellular [Na+]. The relationship between [Na+]pip and [Na+]ps was analyzed using two approaches. First, a mathematical model of three-dimensional ionic diffusion within a whole-cell patch-clamped myocyte was developed and the effects of experimental parameters on mean [Na+]ps were investigated. When typical experimental values were simulated, the time course to achieve steady state mean [Na+]ps was found to be most sensitive to variations in electrode pore size, cell length and the Na+ pumping rate, but at steady state, mean [Na+]ps varies from [Na+]pip by 5% or less depending on pump rate. Second, to provide experimental support for the validity of the simulations, isolated ventricular myocytes were voltage-clamped and the reversal potential for the Na current was determined in order to estimate steady state intracellular [Na+]. The results of the mathematical and experimental analyses suggest that steady state [Na+]ps can be regulated by the [Na+] in suction pipette electrodes. These findings, while also having a broader significance, indicate for isolated cardiac myocytes that whole-cell suction micro-electrodes can provide a means to assess the dependence of the Na,K-pump on [Na+]ps.
Full text
PDF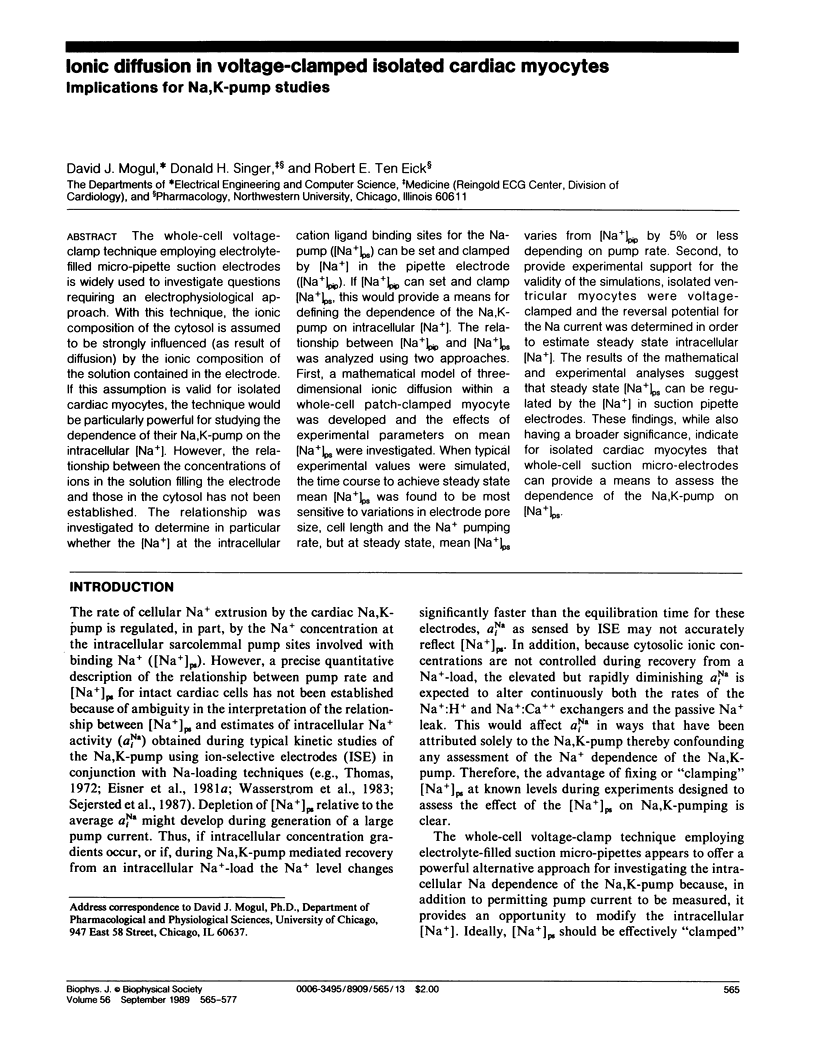
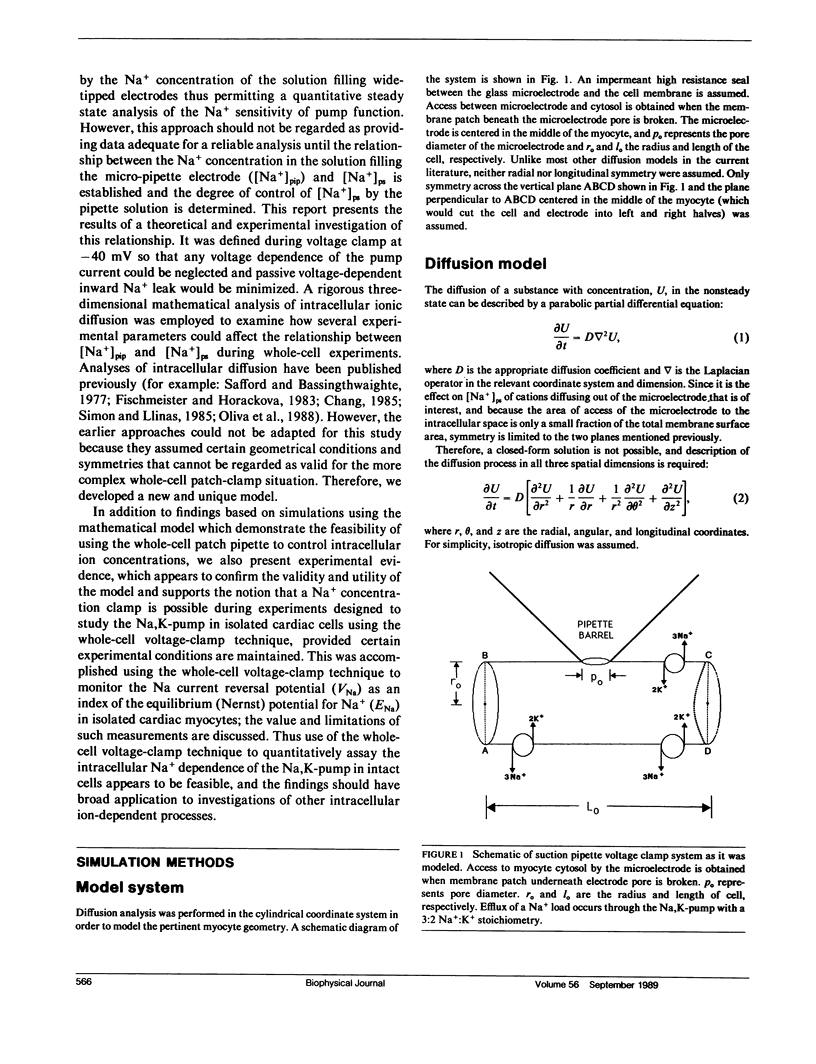
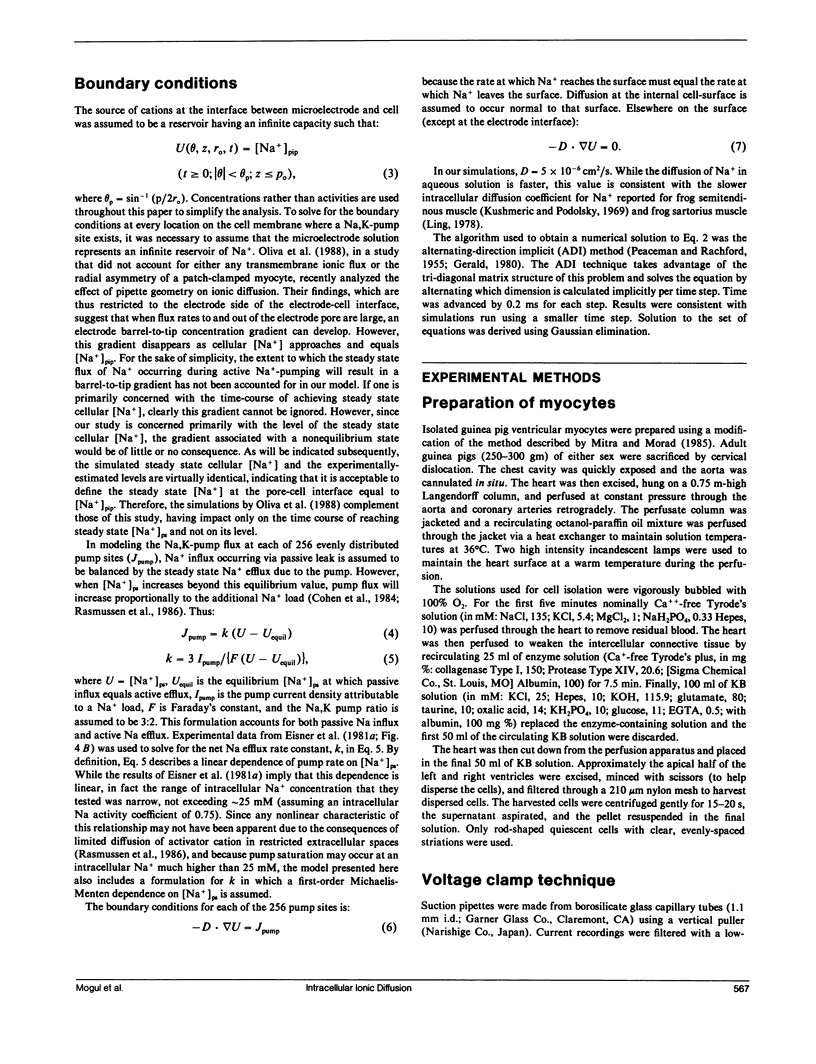
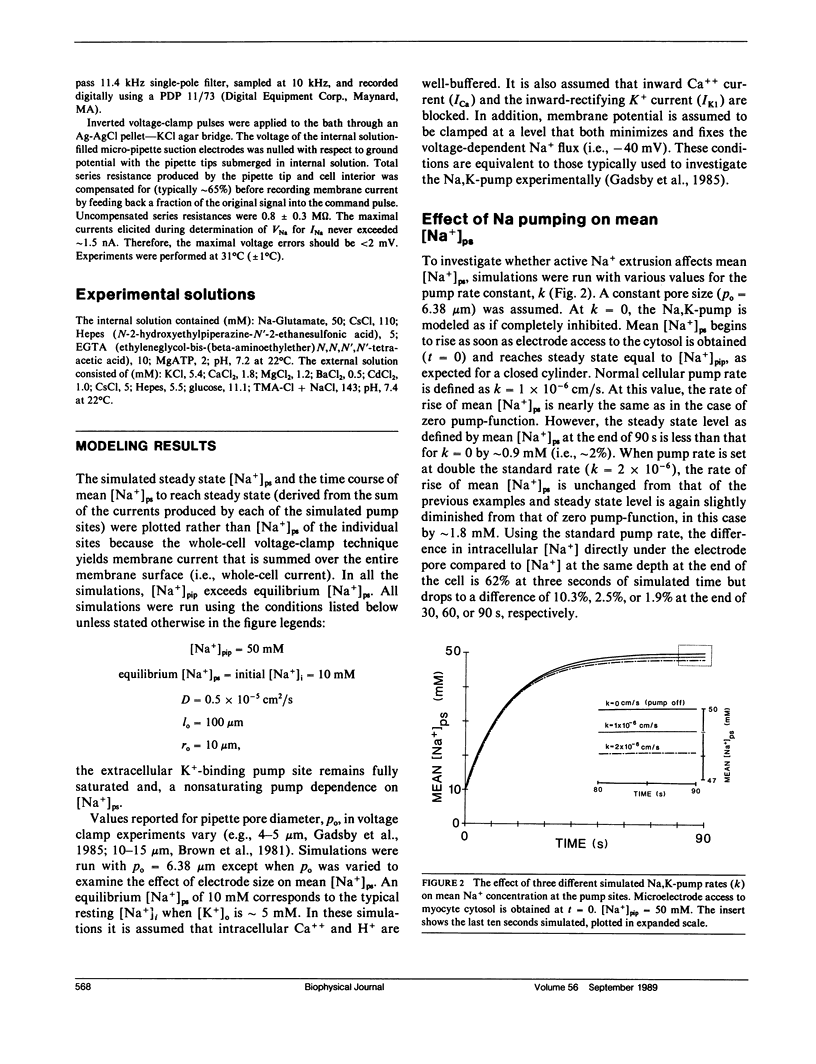
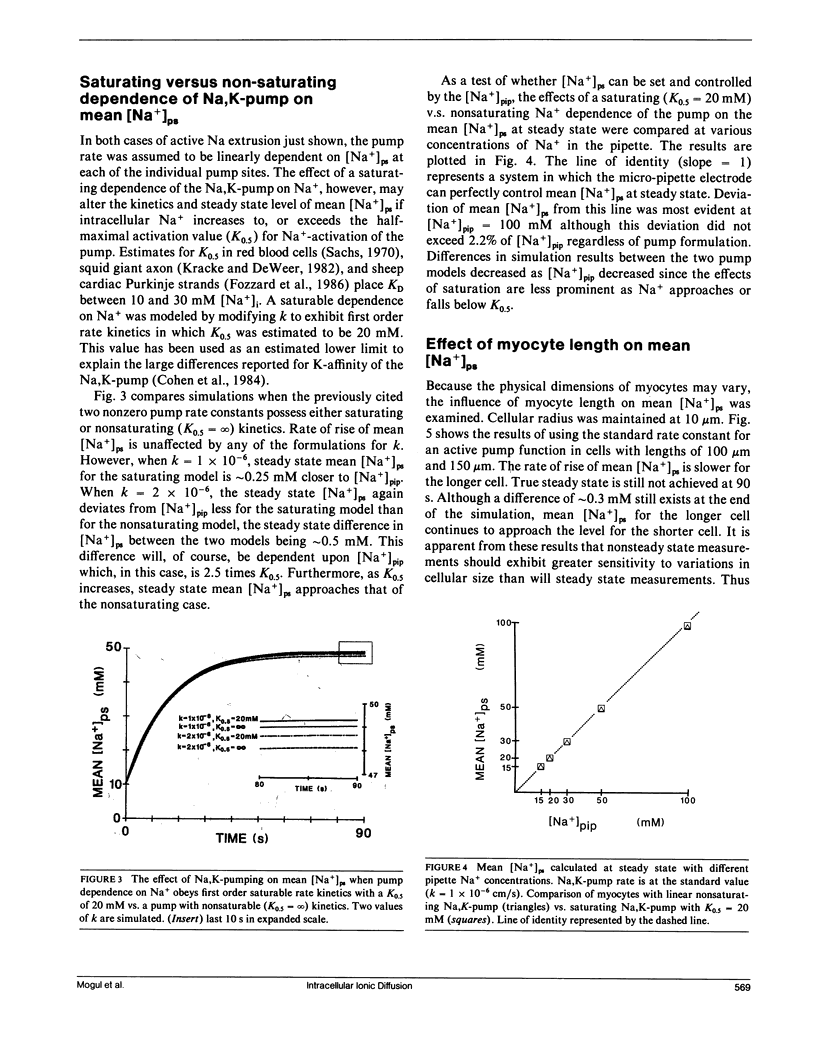
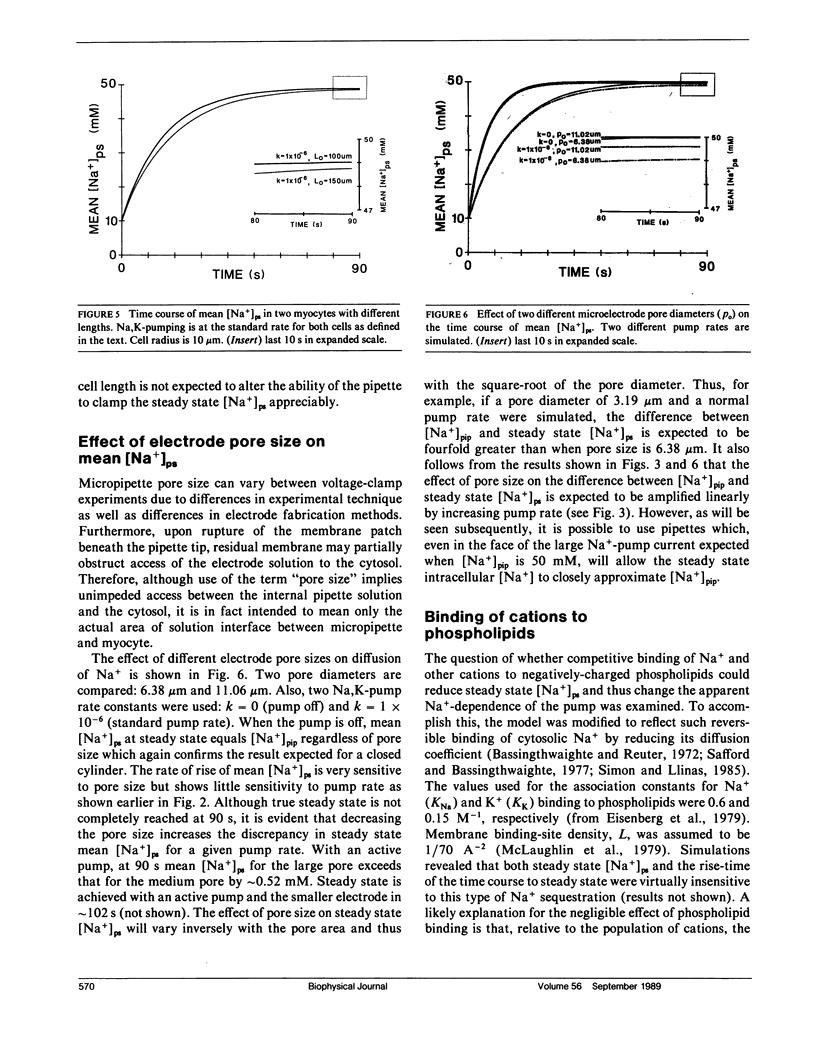
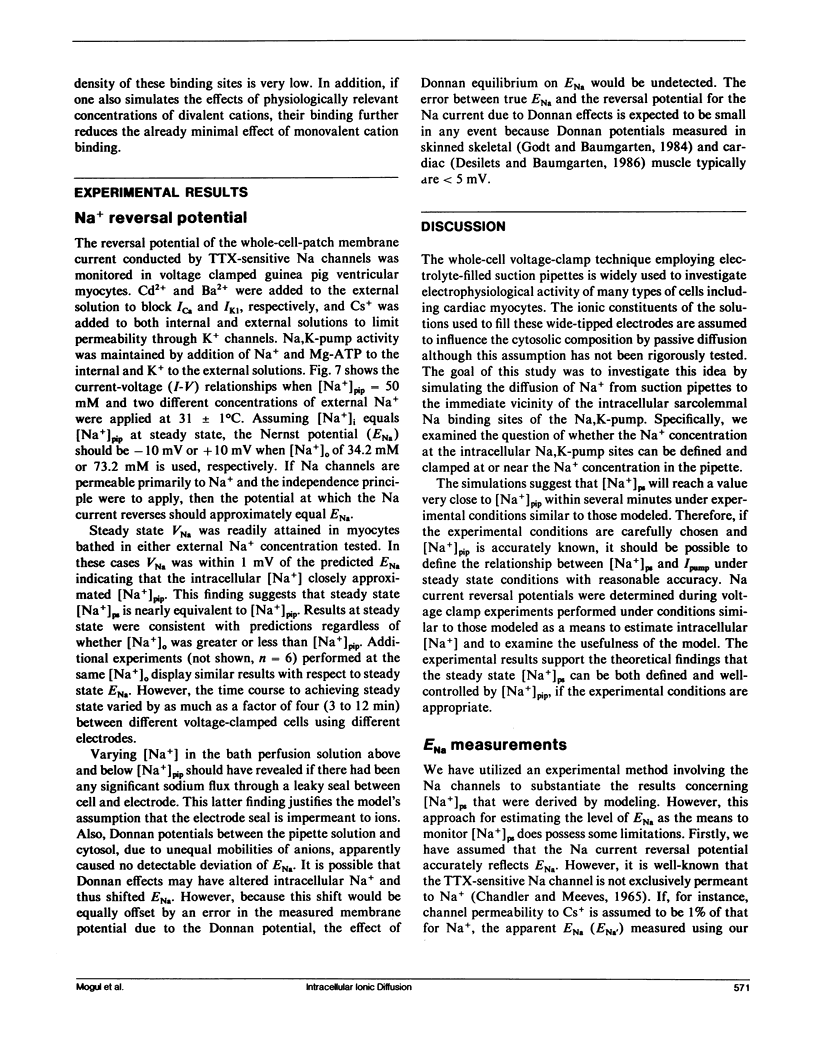
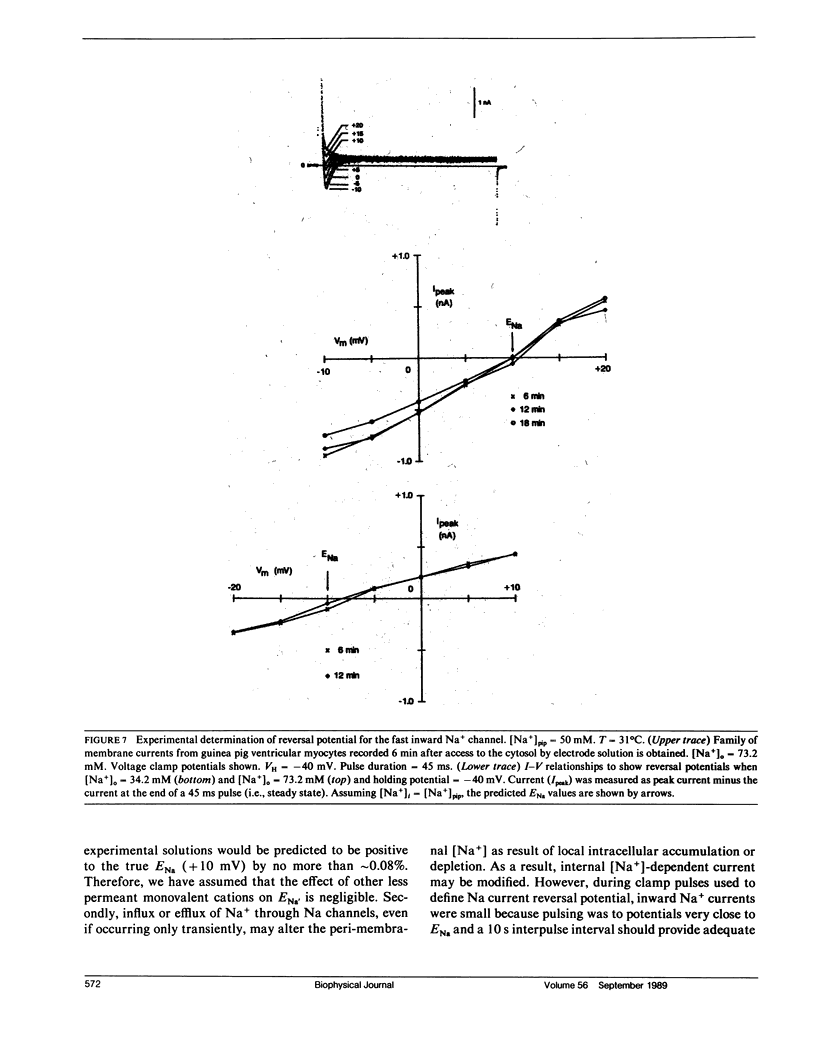
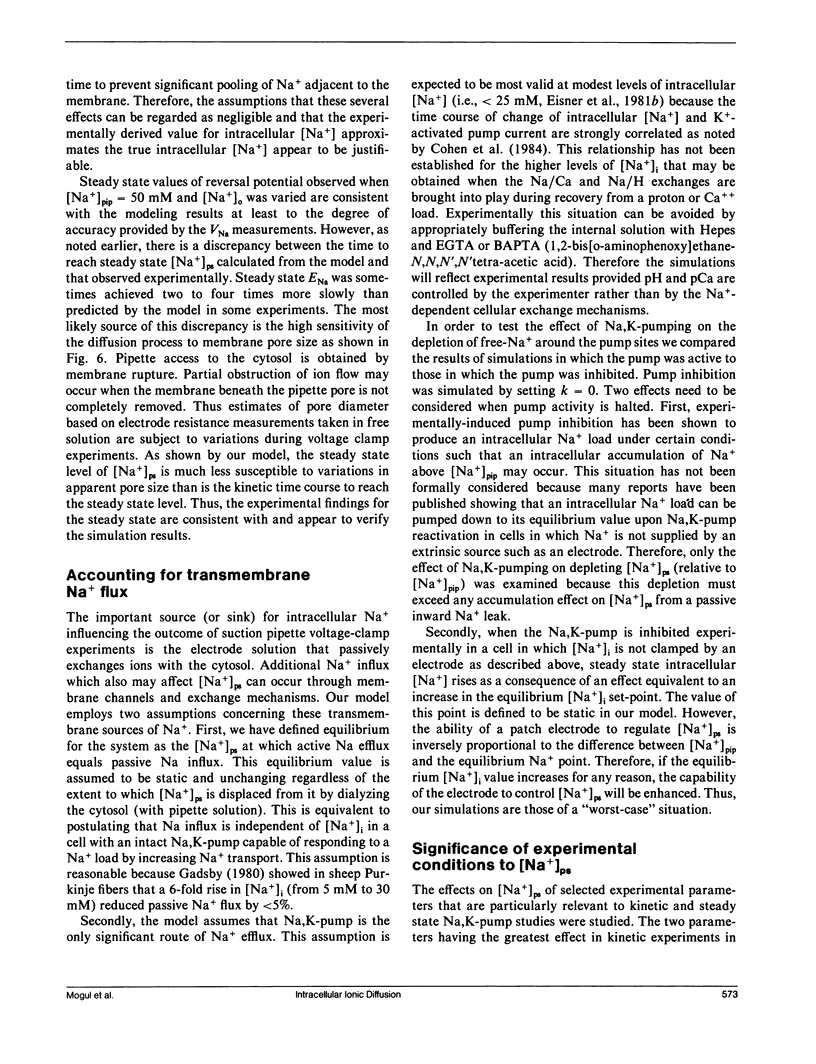
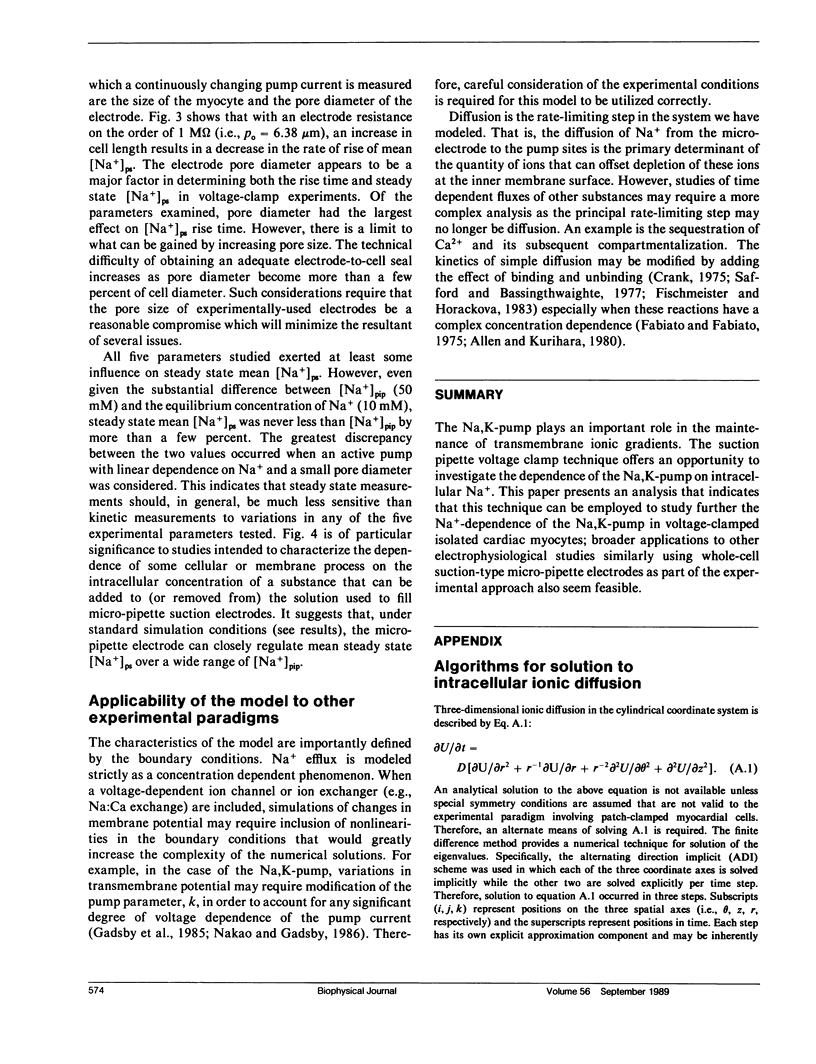
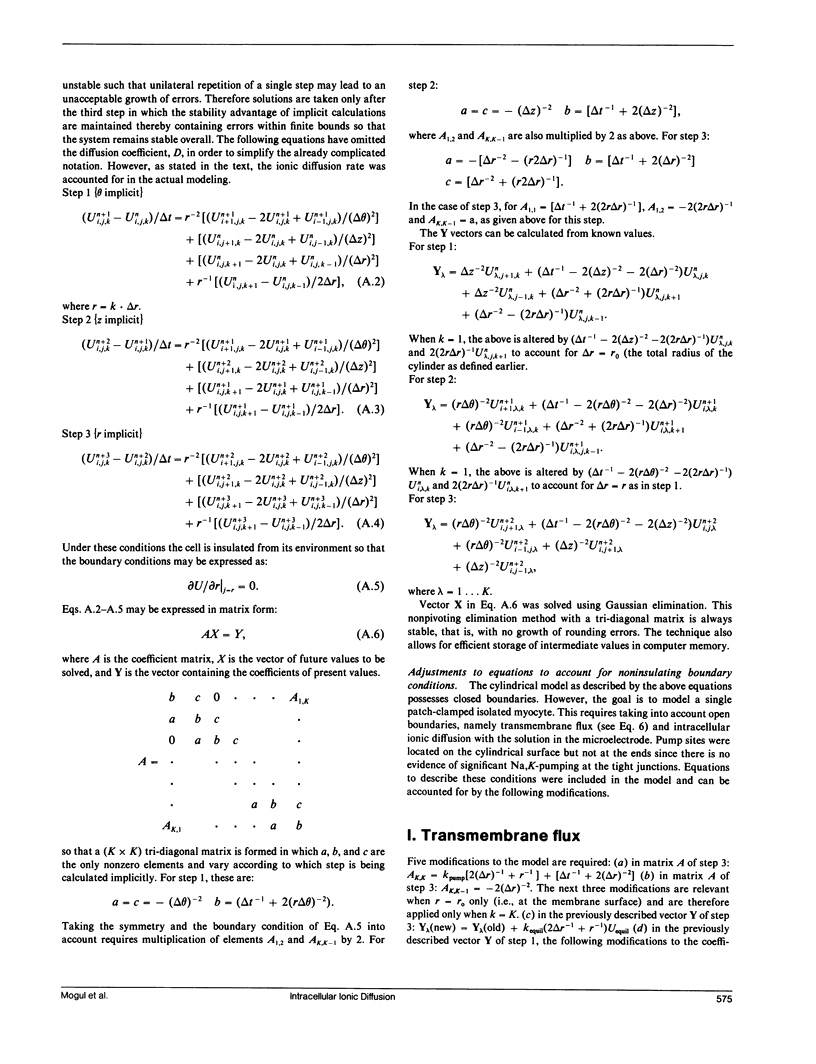
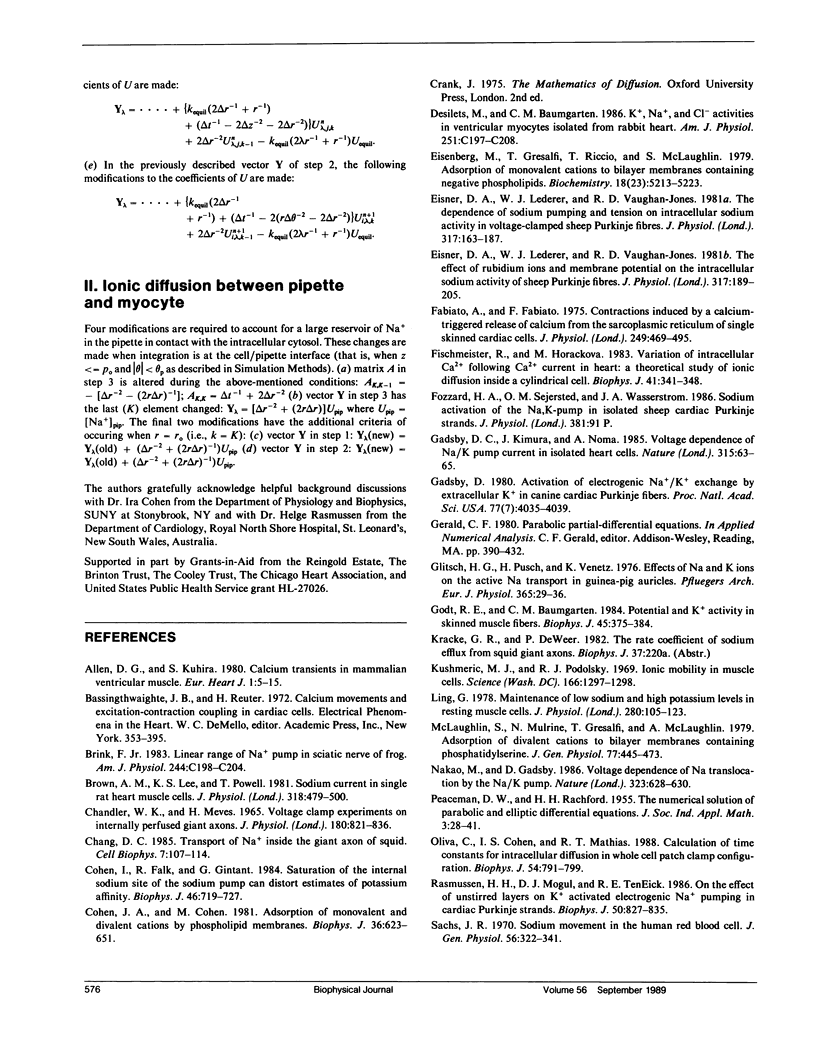
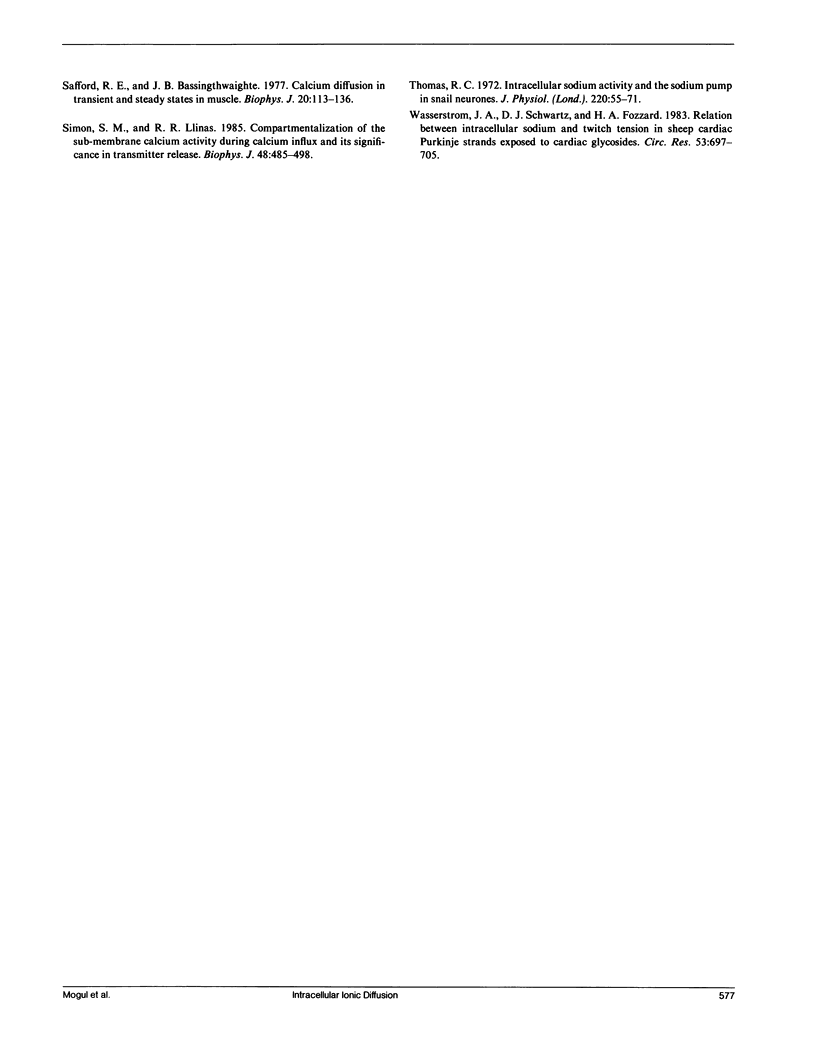
Selected References
These references are in PubMed. This may not be the complete list of references from this article.
- Allen D. G., Kurihara S. Calcium transients in mammalian ventricular muscle. Eur Heart J. 1980;Suppl A:5–15. doi: 10.1093/eurheartj/1.suppl_1.5. [DOI] [PubMed] [Google Scholar]
- Brink F., Jr Linear range of Na+ pump in sciatic nerve of frog. Am J Physiol. 1983 Mar;244(3):C198–C204. doi: 10.1152/ajpcell.1983.244.3.C198. [DOI] [PubMed] [Google Scholar]
- Brown A. M., Lee K. S., Powell T. Sodium current in single rat heart muscle cells. J Physiol. 1981 Sep;318:479–500. doi: 10.1113/jphysiol.1981.sp013879. [DOI] [PMC free article] [PubMed] [Google Scholar]
- Chandler W. K., Hodgkin A. L., Meves H. The effect of changing the internal solution on sodium inactivation and related phenomena in giant axons. J Physiol. 1965 Oct;180(4):821–836. doi: 10.1113/jphysiol.1965.sp007733. [DOI] [PMC free article] [PubMed] [Google Scholar]
- Chang D. C. Transport of Na+ inside the giant axon of squid. Cell Biophys. 1985 Jun;7(2):107–114. doi: 10.1007/BF02784486. [DOI] [PubMed] [Google Scholar]
- Cohen I., Falk R., Gintant G. Saturation of the internal sodium site of the sodium pump can distort estimates of potassium affinity. Biophys J. 1984 Dec;46(6):719–727. doi: 10.1016/S0006-3495(84)84070-9. [DOI] [PMC free article] [PubMed] [Google Scholar]
- Cohen J. A., Cohen M. Adsorption of monovalent and divalent cations by phospholipid membranes. The monomer-dimer problem. Biophys J. 1981 Dec;36(3):623–651. doi: 10.1016/S0006-3495(81)84756-X. [DOI] [PMC free article] [PubMed] [Google Scholar]
- Désilets M., Baumgarten C. M. K+, Na+, and Cl- activities in ventricular myocytes isolated from rabbit heart. Am J Physiol. 1986 Aug;251(2 Pt 1):C197–C208. doi: 10.1152/ajpcell.1986.251.2.C197. [DOI] [PubMed] [Google Scholar]
- Eisenberg M., Gresalfi T., Riccio T., McLaughlin S. Adsorption of monovalent cations to bilayer membranes containing negative phospholipids. Biochemistry. 1979 Nov 13;18(23):5213–5223. doi: 10.1021/bi00590a028. [DOI] [PubMed] [Google Scholar]
- Eisner D. A., Lederer W. J., Vaughan-Jones R. D. The dependence of sodium pumping and tension on intracellular sodium activity in voltage-clamped sheep Purkinje fibres. J Physiol. 1981 Aug;317:163–187. doi: 10.1113/jphysiol.1981.sp013819. [DOI] [PMC free article] [PubMed] [Google Scholar]
- Eisner D. A., Lederer W. J., Vaughan-Jones R. D. The effects of rubidium ions and membrane potentials on the intracellular sodium activity of sheep Purkinje fibres. J Physiol. 1981 Aug;317:189–205. doi: 10.1113/jphysiol.1981.sp013820. [DOI] [PMC free article] [PubMed] [Google Scholar]
- Fabiato A., Fabiato F. Contractions induced by a calcium-triggered release of calcium from the sarcoplasmic reticulum of single skinned cardiac cells. J Physiol. 1975 Aug;249(3):469–495. doi: 10.1113/jphysiol.1975.sp011026. [DOI] [PMC free article] [PubMed] [Google Scholar]
- Fischmeister R., Horackova M. Variation of intracellular Ca2+ following Ca2+ current in heart. A theoretical study of ionic diffusion inside a cylindrical cell. Biophys J. 1983 Mar;41(3):341–348. doi: 10.1016/S0006-3495(83)84445-2. [DOI] [PMC free article] [PubMed] [Google Scholar]
- Gadsby D. C. Activation of electrogenic Na+/K+ exchange by extracellular K+ in canine cardiac Purkinje fibers. Proc Natl Acad Sci U S A. 1980 Jul;77(7):4035–4039. doi: 10.1073/pnas.77.7.4035. [DOI] [PMC free article] [PubMed] [Google Scholar]
- Gadsby D. C., Kimura J., Noma A. Voltage dependence of Na/K pump current in isolated heart cells. Nature. 1985 May 2;315(6014):63–65. doi: 10.1038/315063a0. [DOI] [PubMed] [Google Scholar]
- Glitsch H. G., Pusch H., Venetz K. Effects of Na and K ions on the active Na transport in guinea-pig auricles. Pflugers Arch. 1976 Sep 3;365(1):29–36. doi: 10.1007/BF00583625. [DOI] [PubMed] [Google Scholar]
- Godt R. E., Baumgarten C. M. Potential and K+ activity in skinned muscle fibers. Evidence against a simple Donnan equilibrium. Biophys J. 1984 Feb;45(2):375–382. doi: 10.1016/S0006-3495(84)84161-2. [DOI] [PMC free article] [PubMed] [Google Scholar]
- Kushmerick M. J., Podolsky R. J. Ionic mobility in muscle cells. Science. 1969 Dec 5;166(3910):1297–1298. doi: 10.1126/science.166.3910.1297. [DOI] [PubMed] [Google Scholar]
- Ling G. N. Maintenance of low sodium and high potassium levels in resting muscle cells. J Physiol. 1978 Jul;280:105–123. doi: 10.1113/jphysiol.1978.sp012375. [DOI] [PMC free article] [PubMed] [Google Scholar]
- McLaughlin S., Mulrine N., Gresalfi T., Vaio G., McLaughlin A. Adsorption of divalent cations to bilayer membranes containing phosphatidylserine. J Gen Physiol. 1981 Apr;77(4):445–473. doi: 10.1085/jgp.77.4.445. [DOI] [PMC free article] [PubMed] [Google Scholar]
- Nakao M., Gadsby D. C. Voltage dependence of Na translocation by the Na/K pump. Nature. 1986 Oct 16;323(6089):628–630. doi: 10.1038/323628a0. [DOI] [PubMed] [Google Scholar]
- Oliva C., Cohen I. S., Mathias R. T. Calculation of time constants for intracellular diffusion in whole cell patch clamp configuration. Biophys J. 1988 Nov;54(5):791–799. doi: 10.1016/S0006-3495(88)83017-0. [DOI] [PMC free article] [PubMed] [Google Scholar]
- Rasmussen H. H., Mogul D. J., TenEick R. E. On the effect of unstirred layers on K+-activated electrogenic Na+ pumping in cardiac Purkinje strands. Biophys J. 1986 Nov;50(5):827–835. doi: 10.1016/S0006-3495(86)83523-8. [DOI] [PMC free article] [PubMed] [Google Scholar]
- Sachs J. R. Sodium movements in the human red blood cell. J Gen Physiol. 1970 Sep;56(3):322–341. doi: 10.1085/jgp.56.3.322. [DOI] [PMC free article] [PubMed] [Google Scholar]
- Safford R. E., Bassingthwaighte J. B. Calcium diffusion in transient and steady states in muscle. Biophys J. 1977 Oct;20(1):113–136. doi: 10.1016/S0006-3495(77)85539-2. [DOI] [PMC free article] [PubMed] [Google Scholar]
- Simon S. M., Llinás R. R. Compartmentalization of the submembrane calcium activity during calcium influx and its significance in transmitter release. Biophys J. 1985 Sep;48(3):485–498. doi: 10.1016/S0006-3495(85)83804-2. [DOI] [PMC free article] [PubMed] [Google Scholar]
- Thomas R. C. Intracellular sodium activity and the sodium pump in snail neurones. J Physiol. 1972 Jan;220(1):55–71. doi: 10.1113/jphysiol.1972.sp009694. [DOI] [PMC free article] [PubMed] [Google Scholar]
- Wasserstrom J. A., Schwartz D. J., Fozzard H. A. Relation between intracellular sodium and twitch tension in sheep cardiac Purkinje strands exposed to cardiac glycosides. Circ Res. 1983 Jun;52(6):697–705. doi: 10.1161/01.res.52.6.697. [DOI] [PubMed] [Google Scholar]