Abstract
1. Synaptic transmission between dorsal root afferents and motoneurones was studied in the isolated and hemisected spinal cord of frogs, using intracellular and extracellular recording techniques, and ionic substitutions of divalent cations in the bathing fluid. 2. Delayed components of excitatory post-synaptic potentials (e.p.s.p.s) evoked in motoneurones by dorsal root supramaximal stimuli, as well as the Ca2+-dependent slow after-hyperpolarization which follows antidromic spikes, were reversibly blocked by superfusing the cords with 'Ca2+-free' media containing Co2+ (4 mM) or Mg2+ (6-10 mM). However, short latency e.p.s.p.s persisted in these media for more than 8 hr. 3. The minimum synaptic delay of the Co2+ and Mg2+, resistant e.p.s.p.s, measured from the peak negativity of the extracellularly recorded presynaptic spike to the onset of the e.p.s.p., was 0.3 msec at 10 +/- 1 degrees C. 4. The Co2+, Mg2+-resistant e.p.s.p.s were graded, and could be elicited by stimulation of segmental or adjacent roots. Those evoked by each of two adjacent roots showed linear summation when the roots were stimulated simultaneously. 5. The Co2+, Mg2+-resistant e.p.s.p.s decreased in amplitude at stimulating frequencies between 10 and 100 Hz, and with paired stimuli at intervals shorter than 20-40 msec. These reductions in amplitude were paralleled by decreases in amplitude of the presynaptic population spike. 6. Solutions free of divalent ions, containing EGTA (2 mM) abolished the Co2+, Mg2+-resistant e.p.s.p.s. They remained blocked for a variable time after returning to Ca2+-free Ringer containing Mg2+ (8 mM). Their continued abolition at this stage is probably not due to changes in electrical properties of motoneuronal membranes. Eventually, the Mg2+-resistant e.p.s.p.s started recovering in the Ca2+-free Ringer containing Mg2+. The time of onset of this recovery depended on the duration of exposure to EGTA. 7. Sr2+ (2-11 mM), although less effective than Ca2+, restored the composite e.p.s.p.s evoked by dorsal root supramaximal stimuli, as well as the Ca2+-dependent slow after-hyperpolarization of the motoneurone. The composite e.p.s.p.s could not be restored with Ba2+ (2-10 mM). 8. The results suggest that the Co2+, Mg2+-resistant e.p.s.p is generated by electrical coupling between some afferent fibres (probably primary afferents) and motoneurones. The after-effects of EGTA treatments probably reflect uncoupling of electrotonic junctions. In contrast, the delayed components of the composite e.p.s.p.s are generated through chemical synapses whose divalent cation requirement is similar to that of the neuromuscular junction.
Full text
PDF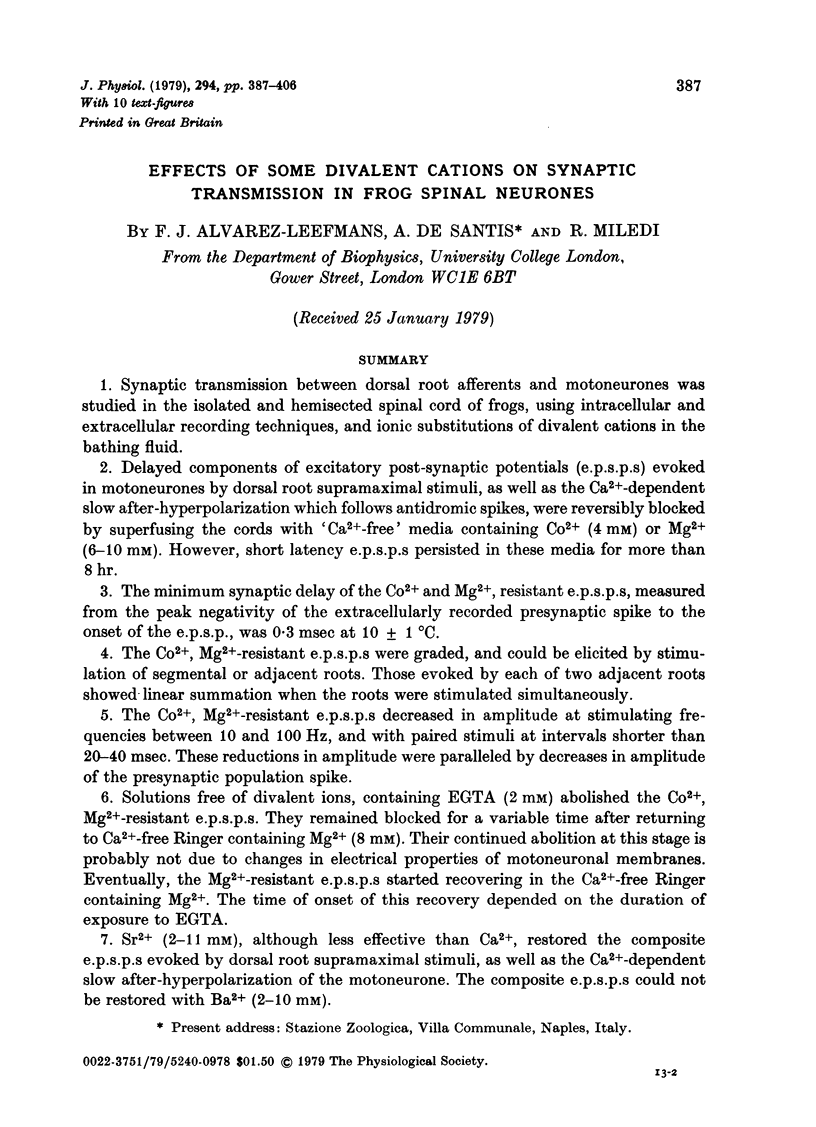
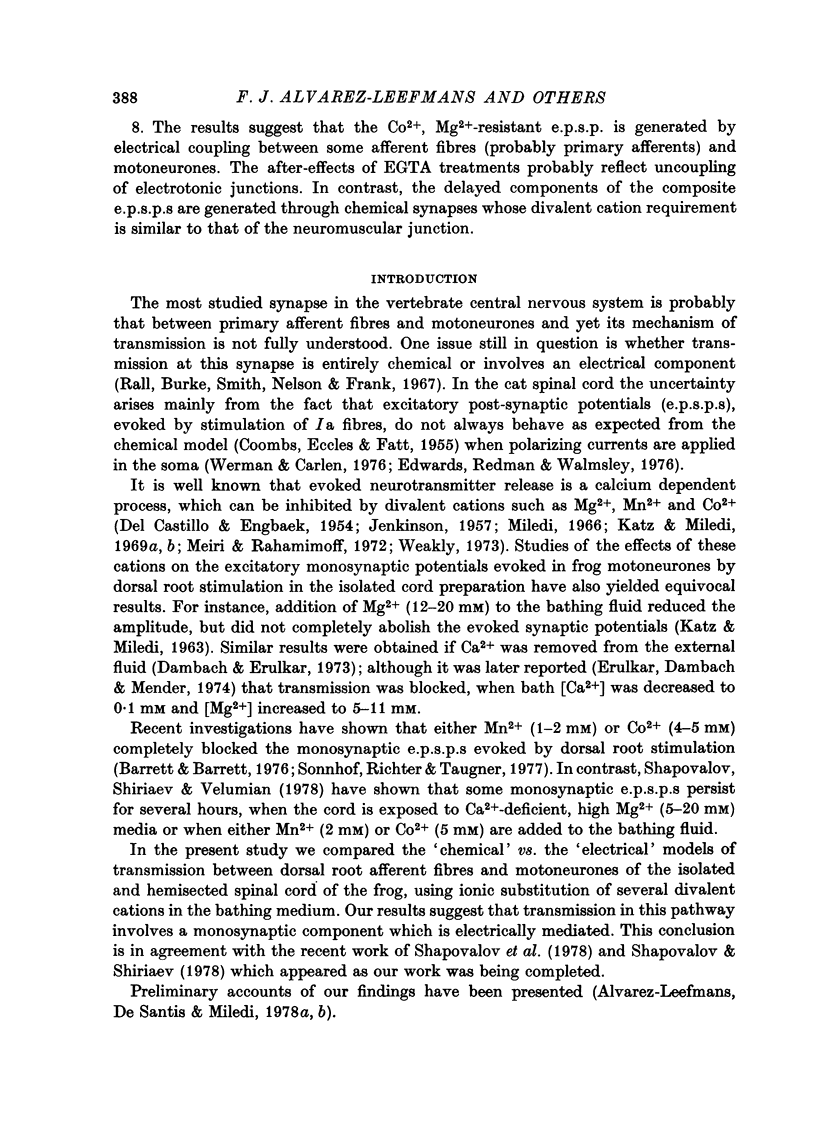
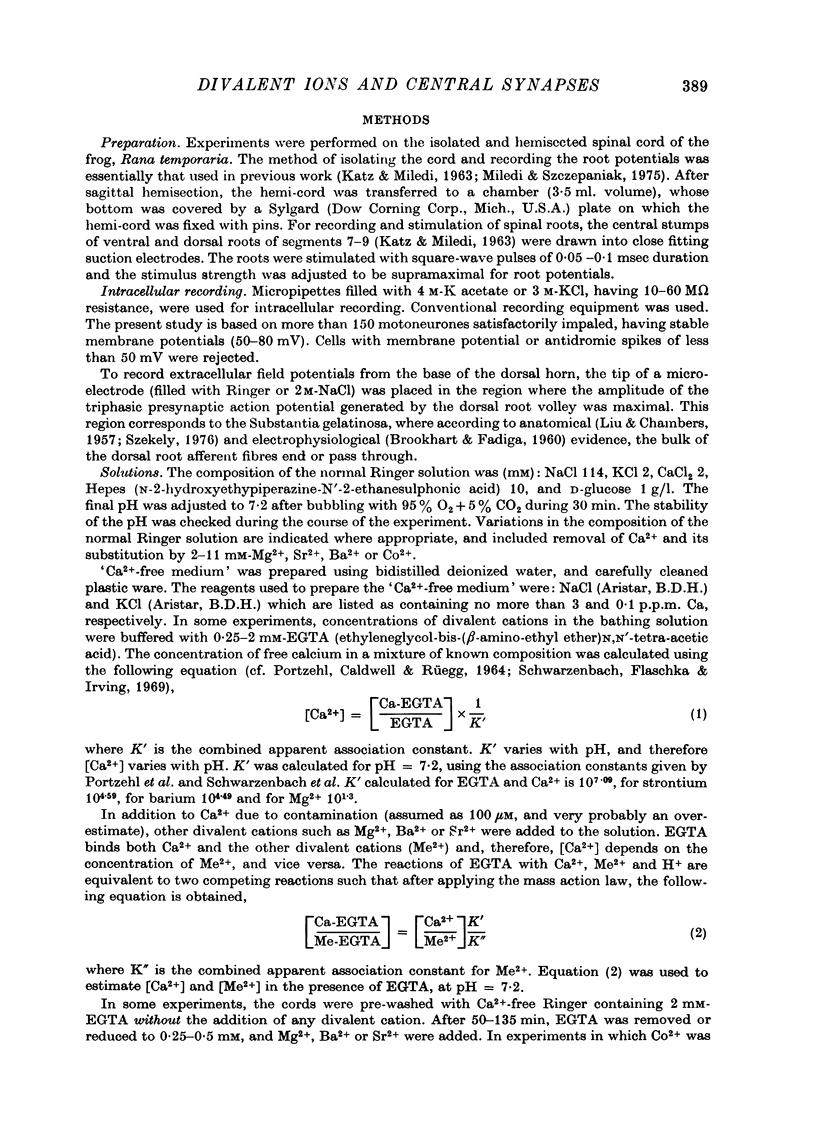
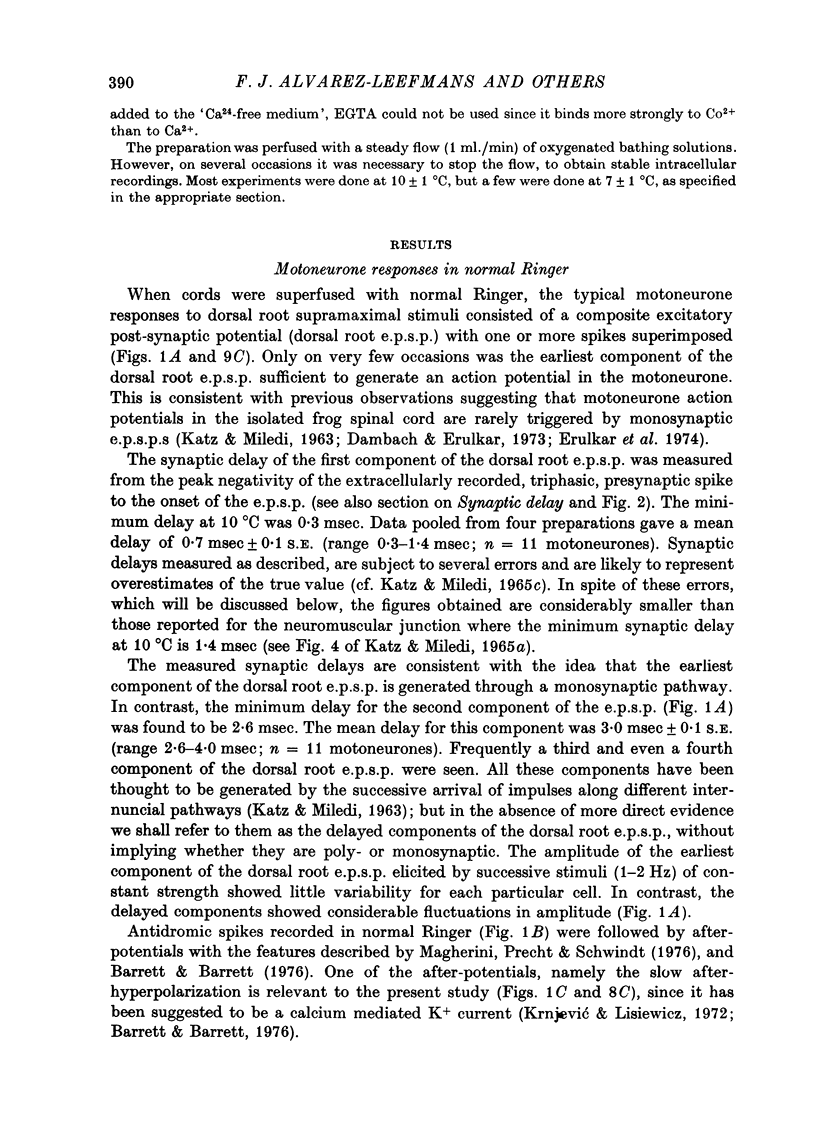
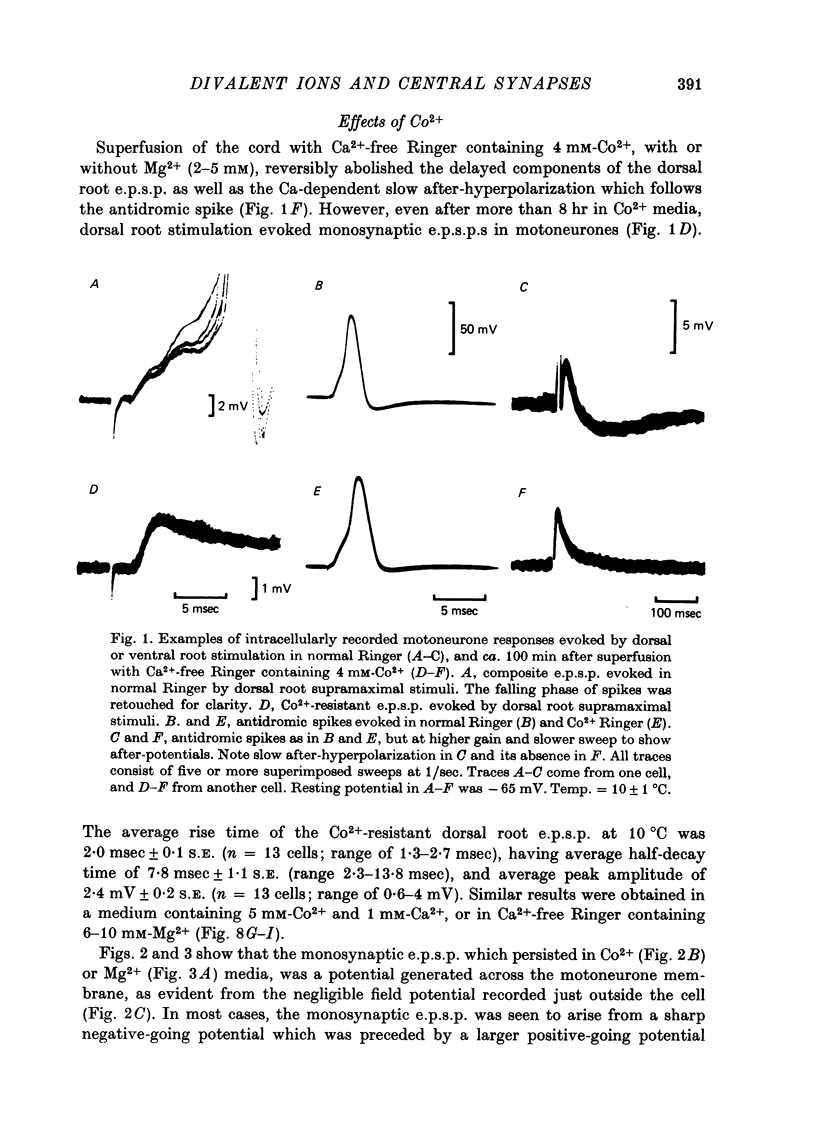
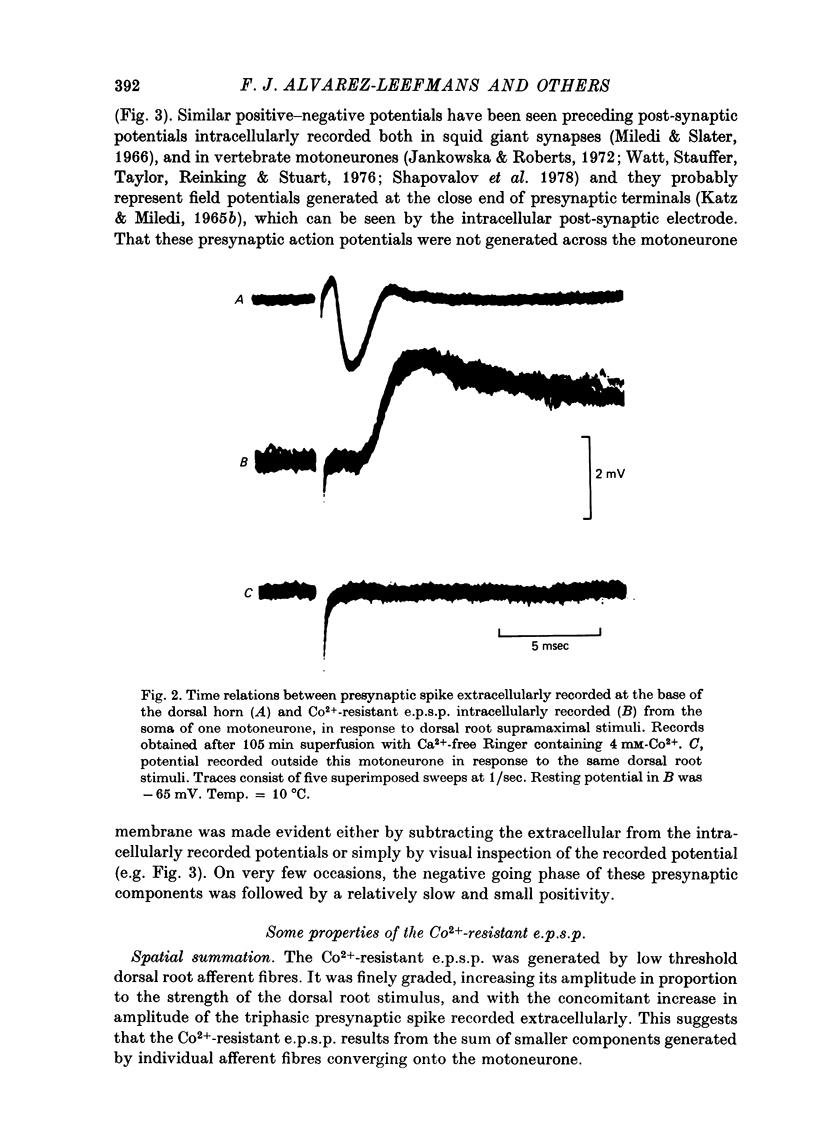
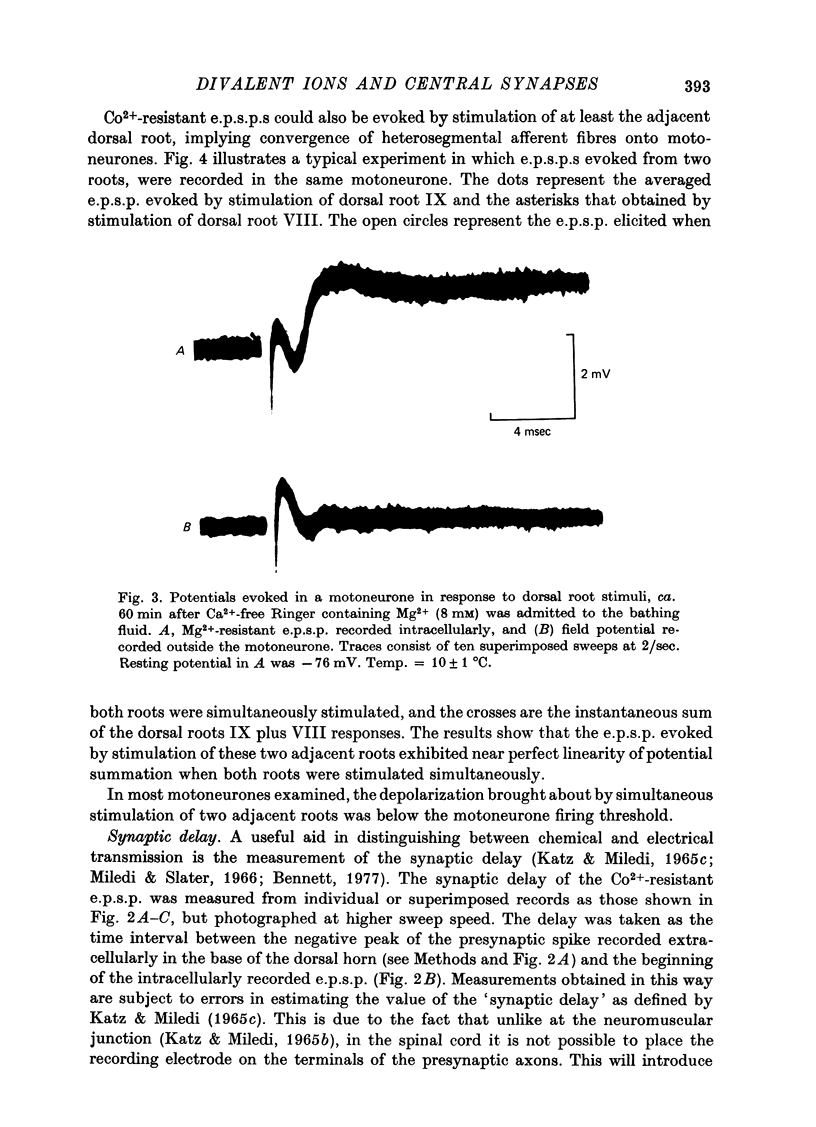
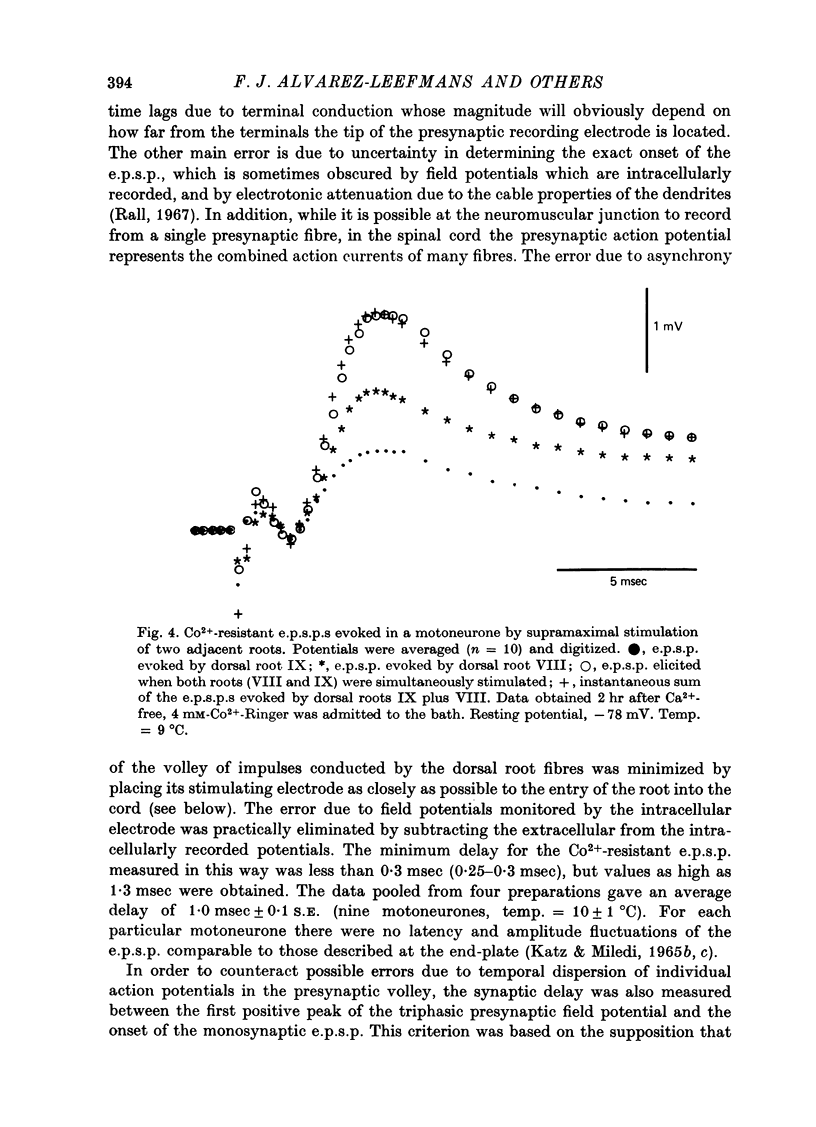
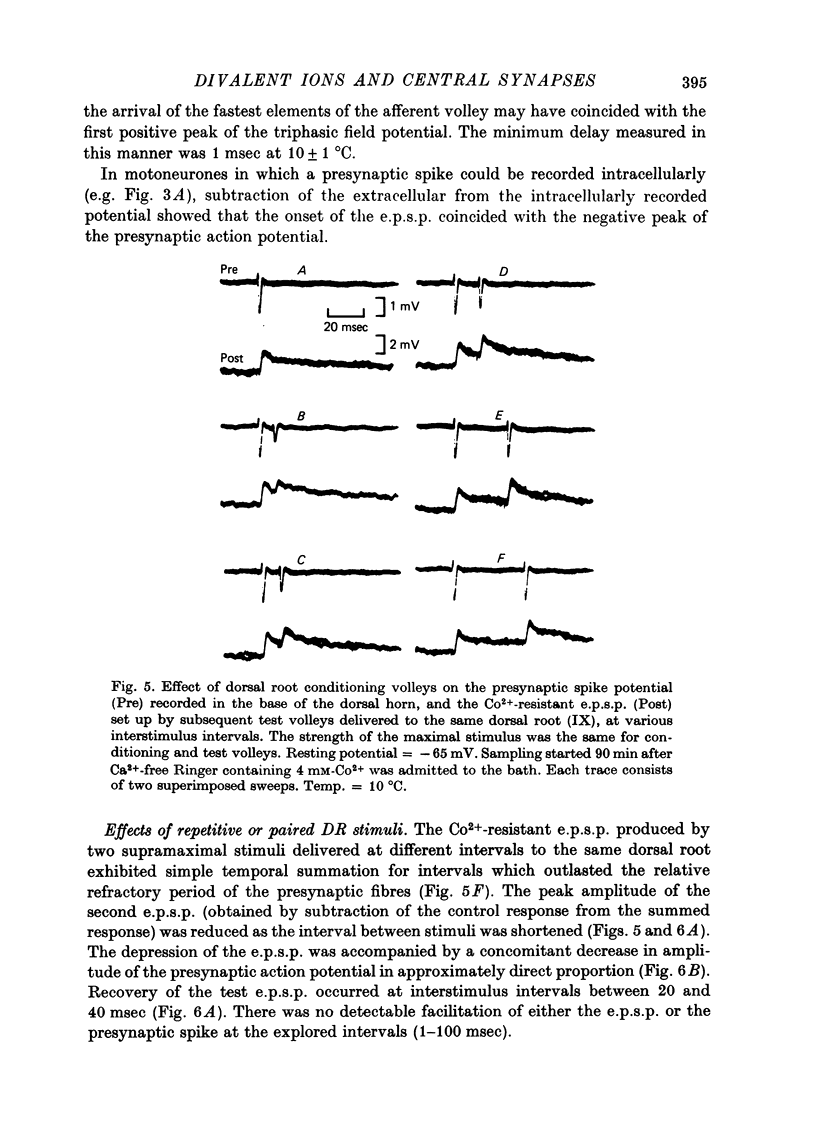
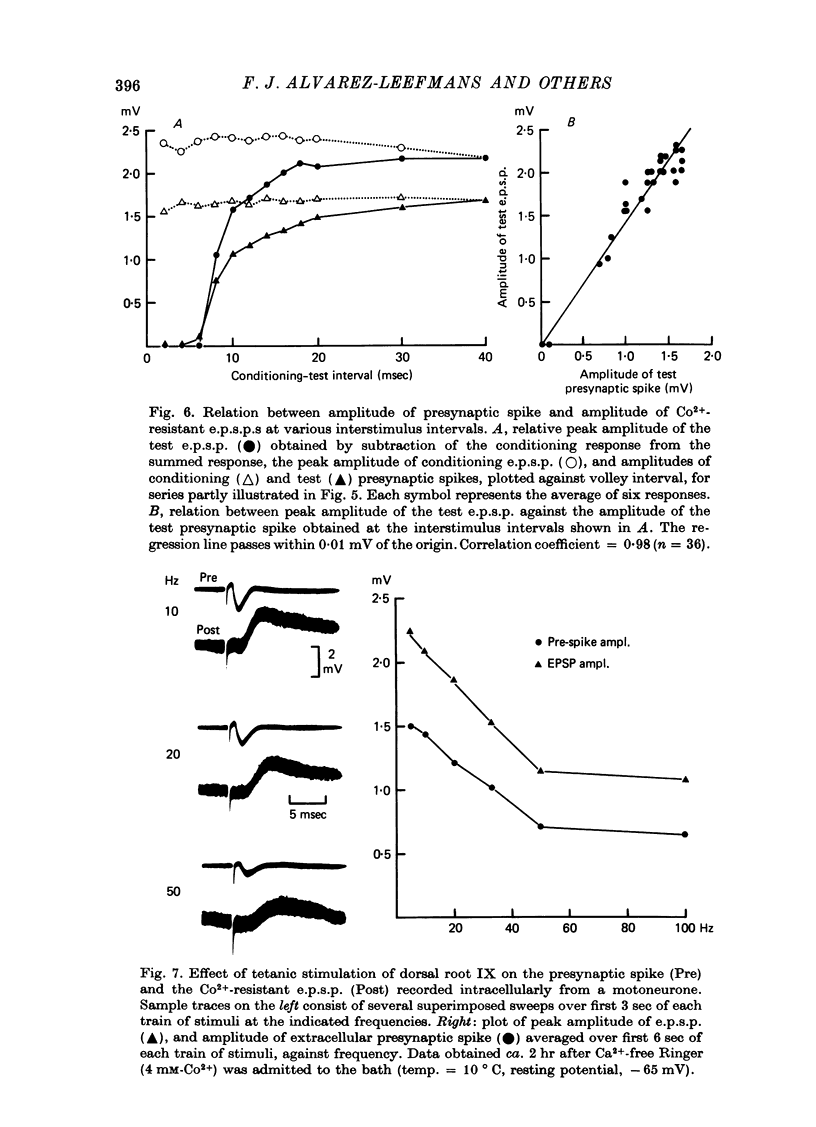
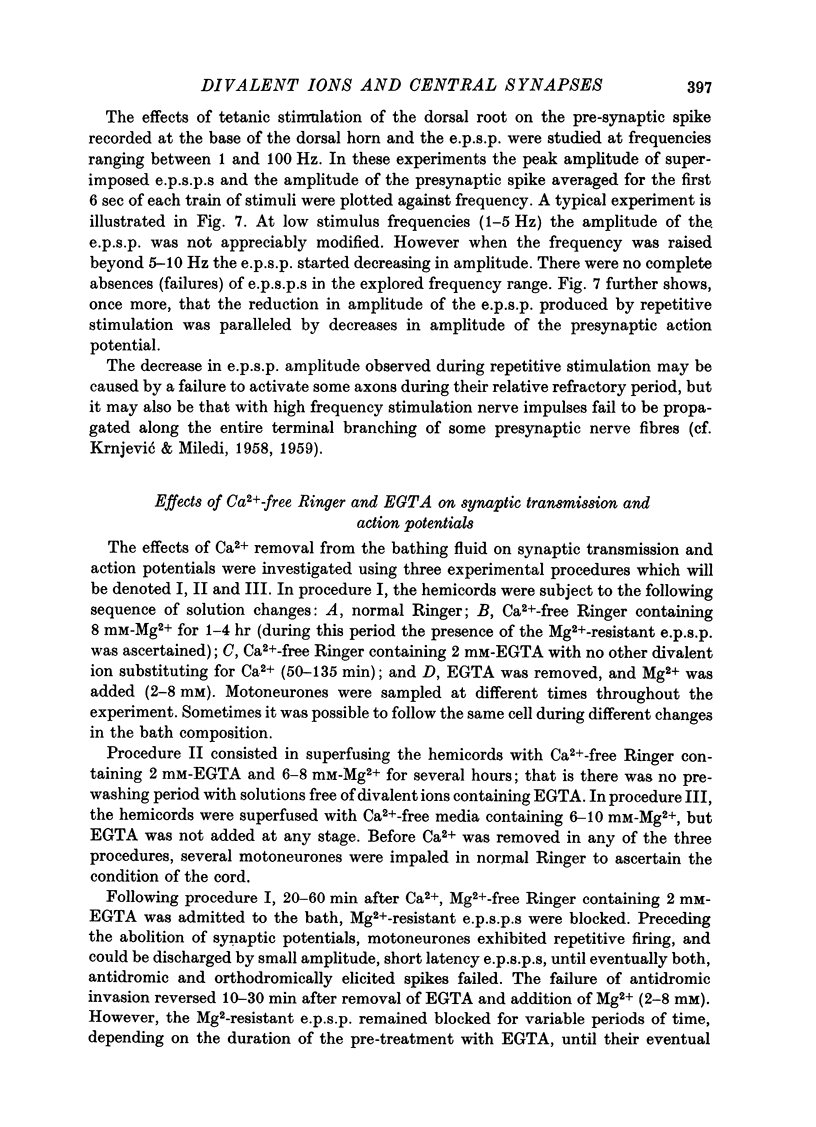
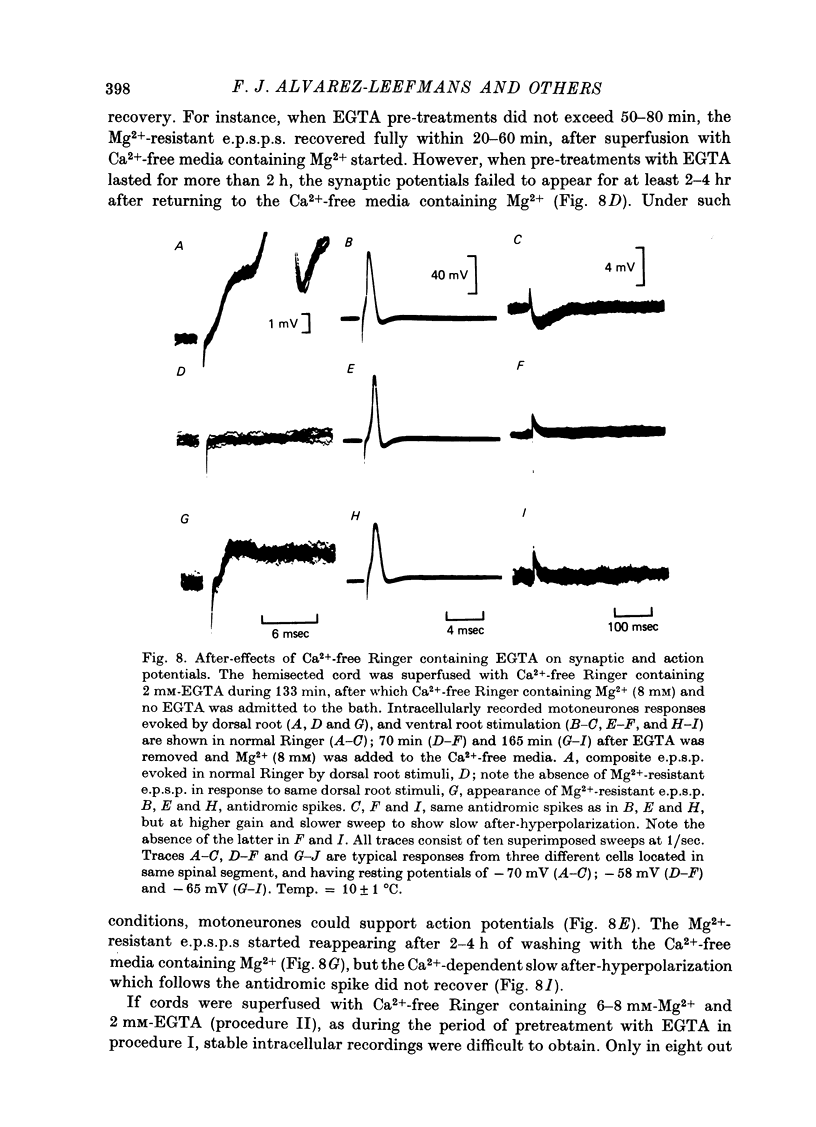
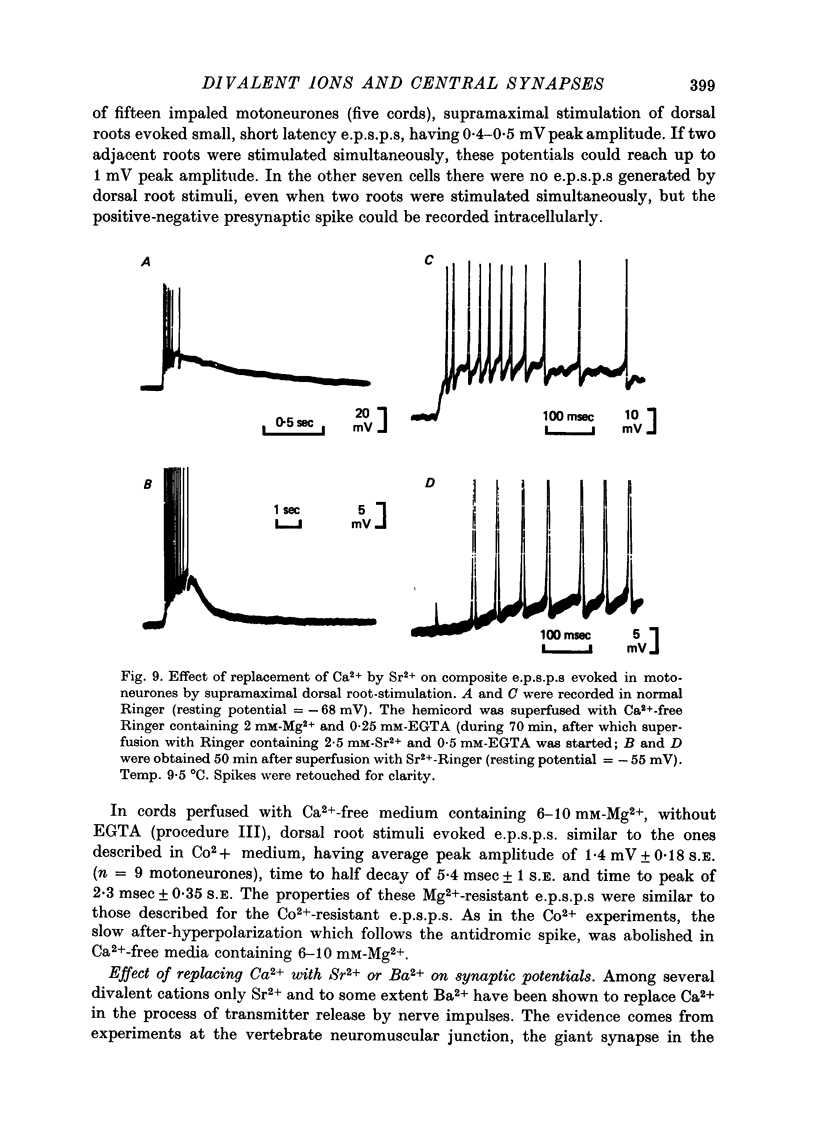
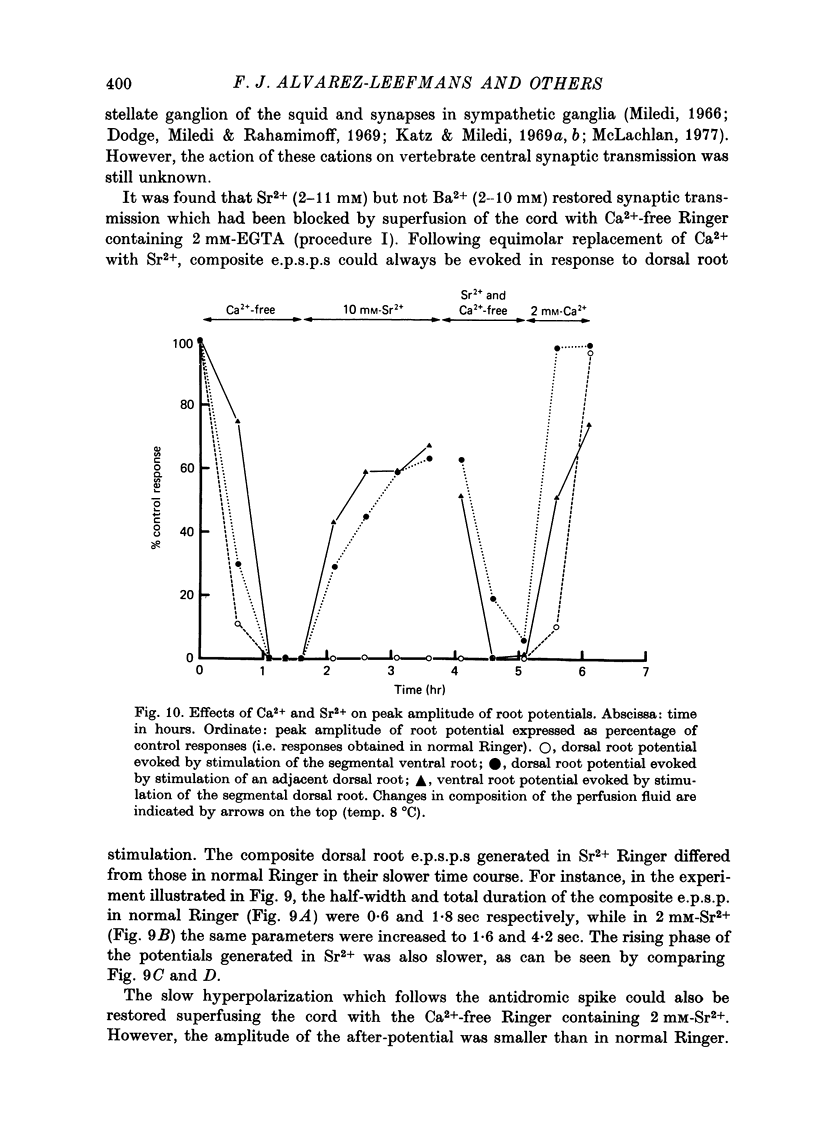
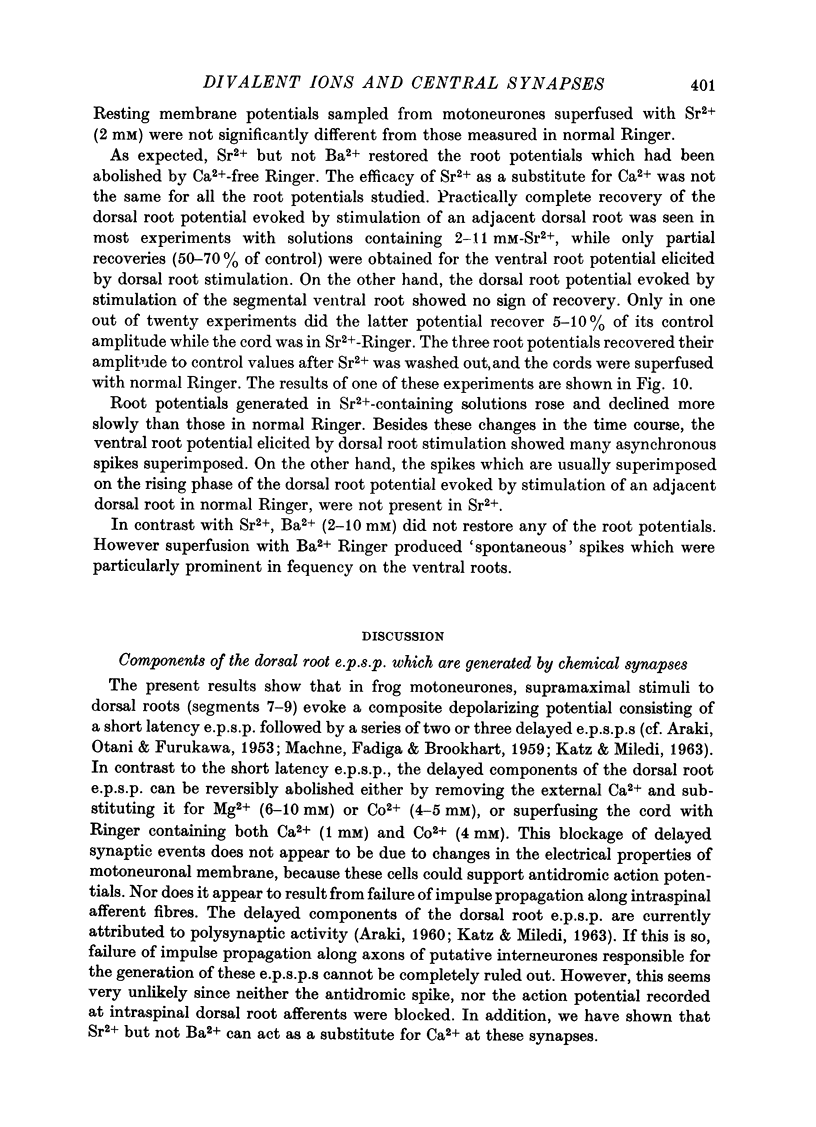
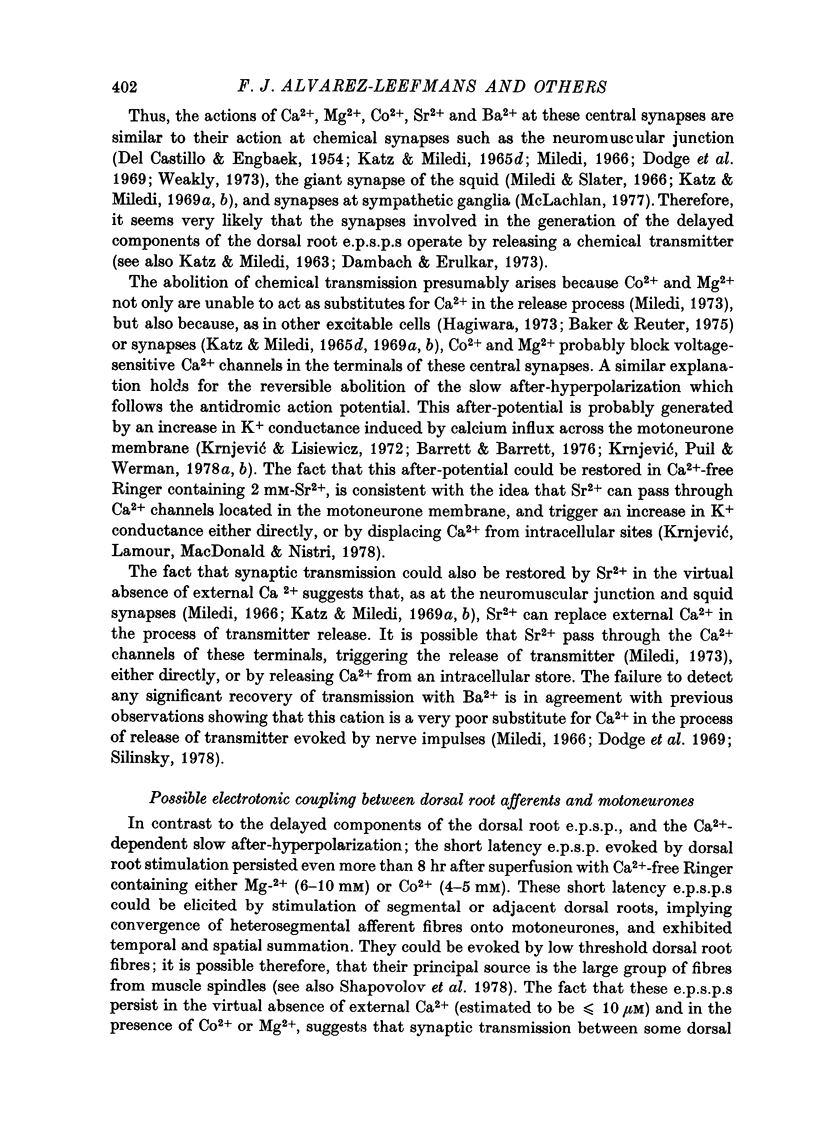
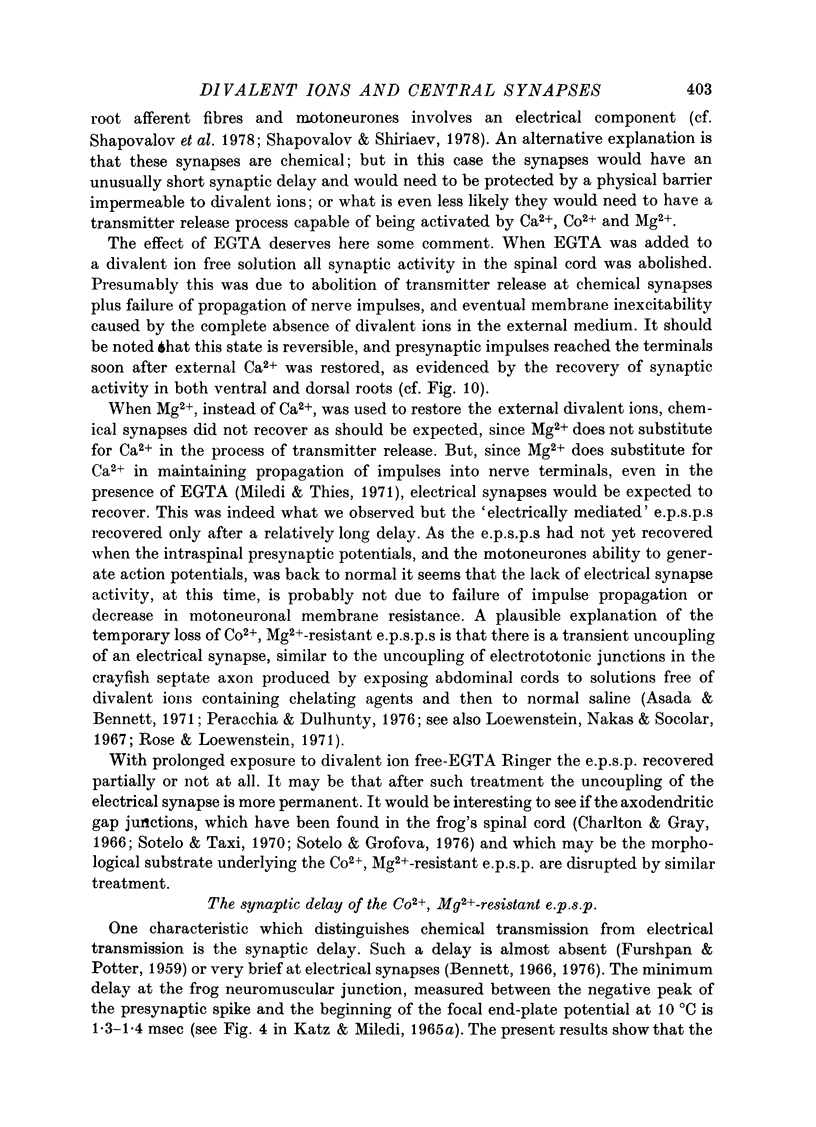
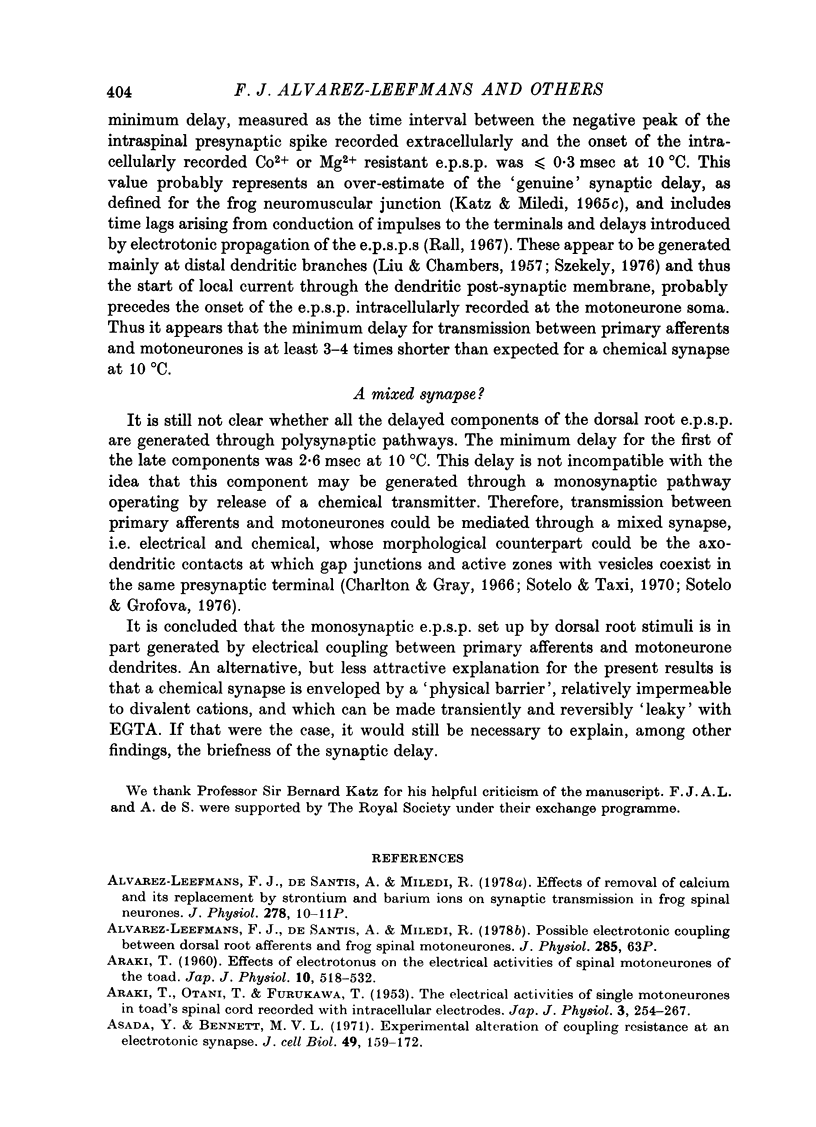
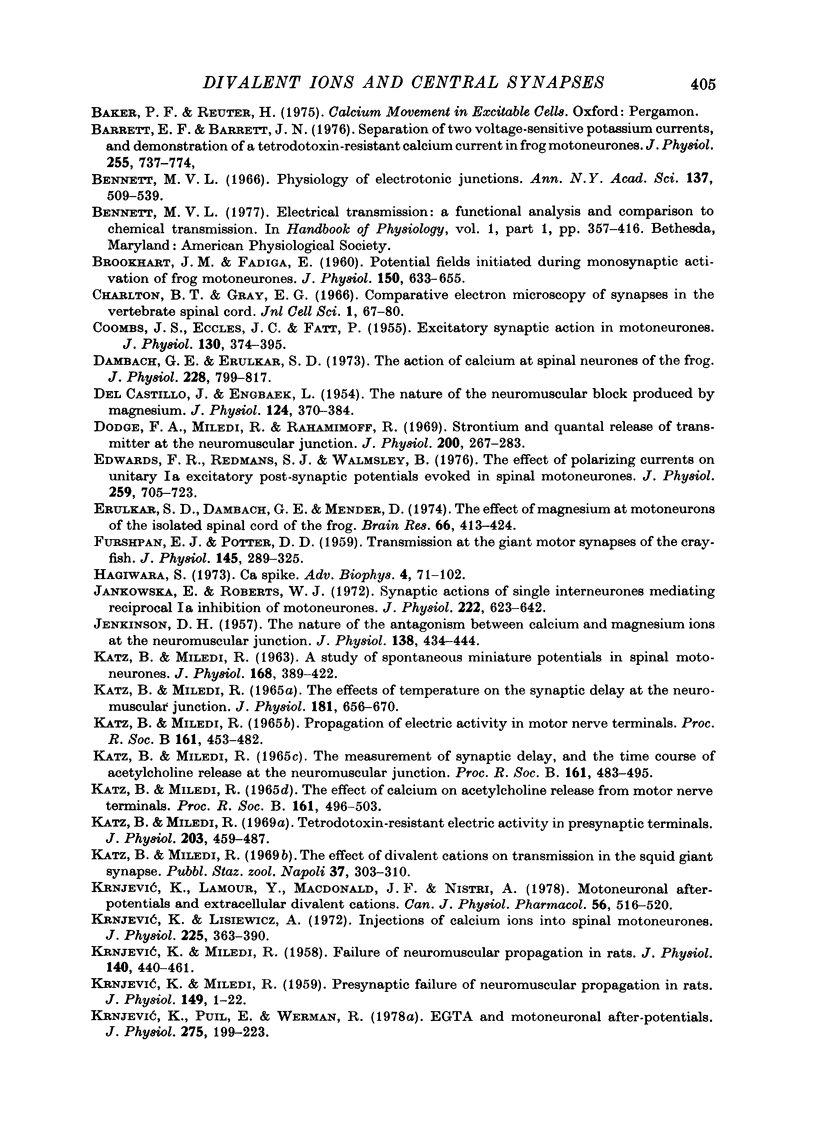
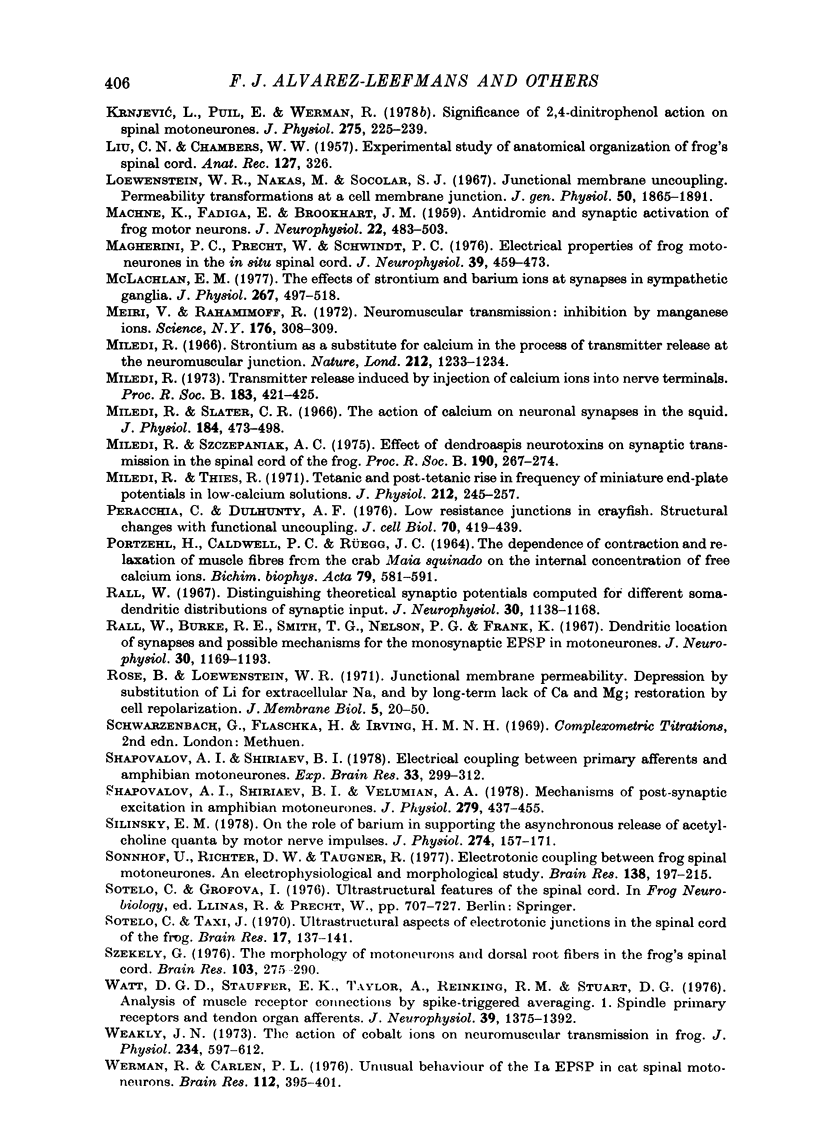
Selected References
These references are in PubMed. This may not be the complete list of references from this article.
- ARAKI T. Effects of electrotonus on the electrical activities of spinal motoneurons of the toad. Jpn J Physiol. 1960 Oct 15;10:518–532. doi: 10.2170/jjphysiol.10.518. [DOI] [PubMed] [Google Scholar]
- ARAKI T., OTANI T., FURUKAWA T. The electrical activities of single motoneurones in toad's spinal cord, recorded with intracellular electrodes. Jpn J Physiol. 1953 Dec 15;3(4):254–267. doi: 10.2170/jjphysiol.3.254. [DOI] [PubMed] [Google Scholar]
- Alvarez-Leefmans F. J., de Santis A., Miledi R. Possible electrotonic coupling between dorsal root afferents and frog spinal motoneurones [proceedings]. J Physiol. 1978 Dec;285:63P–63P. [PubMed] [Google Scholar]
- Asada Y., Bennett M. V. Experimental alteration of coupling resistance at an electrotonic synapse. J Cell Biol. 1971 Apr;49(1):159–172. doi: 10.1083/jcb.49.1.159. [DOI] [PMC free article] [PubMed] [Google Scholar]
- BROOKHART J. M., FADIGA E. Potential field initiated during monosynaptic activation of frog motoneurones. J Physiol. 1960 Mar;150:633–655. doi: 10.1113/jphysiol.1960.sp006409. [DOI] [PMC free article] [PubMed] [Google Scholar]
- Barrett E. F., Barret J. N. Separation of two voltage-sensitive potassium currents, and demonstration of a tetrodotoxin-resistant calcium current in frog motoneurones. J Physiol. 1976 Mar;255(3):737–774. doi: 10.1113/jphysiol.1976.sp011306. [DOI] [PMC free article] [PubMed] [Google Scholar]
- Bennett M. V. Physiology of electrotonic junctions. Ann N Y Acad Sci. 1966 Jul 14;137(2):509–539. doi: 10.1111/j.1749-6632.1966.tb50178.x. [DOI] [PubMed] [Google Scholar]
- COOMBS J. S., ECCLES J. C., FATT P. Excitatory synaptic action in motoneurones. J Physiol. 1955 Nov 28;130(2):374–395. doi: 10.1113/jphysiol.1955.sp005413. [DOI] [PMC free article] [PubMed] [Google Scholar]
- Charlton B. T., Gray E. G. Comparative electron microscopy of synapses in the vertebrate spinal cord. J Cell Sci. 1966 Mar;1(1):67–80. doi: 10.1242/jcs.1.1.67. [DOI] [PubMed] [Google Scholar]
- DEL CASTILLO J., ENGBAEK L. The nature of the neuromuscular block produced by magnesium. J Physiol. 1954 May 28;124(2):370–384. doi: 10.1113/jphysiol.1954.sp005114. [DOI] [PMC free article] [PubMed] [Google Scholar]
- Dambach G. E., Erulkar S. D. The action of calcium at spinal neurones of the frog. J Physiol. 1973 Feb;228(3):799–817. doi: 10.1113/jphysiol.1973.sp010113. [DOI] [PMC free article] [PubMed] [Google Scholar]
- Dodge F. A., Jr, Miledi R., Rahamimoff R. Strontium and quantal release of transmitter at the neuromuscular junction. J Physiol. 1969 Jan;200(1):267–283. doi: 10.1113/jphysiol.1969.sp008692. [DOI] [PMC free article] [PubMed] [Google Scholar]
- Edwards F. R., Redman S. J., Walmsley B. The effect of polarizing currents on unitary Ia excitatory post-synaptic potentials evoked in spinal motoneurones. J Physiol. 1976 Aug;259(3):705–723. doi: 10.1113/jphysiol.1976.sp011490. [DOI] [PMC free article] [PubMed] [Google Scholar]
- FURSHPAN E. J., POTTER D. D. Transmission at the giant motor synapses of the crayfish. J Physiol. 1959 Mar 3;145(2):289–325. doi: 10.1113/jphysiol.1959.sp006143. [DOI] [PMC free article] [PubMed] [Google Scholar]
- Hagiwara S. Ca spike. Adv Biophys. 1973;4:71–102. [PubMed] [Google Scholar]
- JENKINSON D. H. The nature of the antagonism between calcium and magnesium ions at the neuromuscular junction. J Physiol. 1957 Oct 30;138(3):434–444. doi: 10.1113/jphysiol.1957.sp005860. [DOI] [PMC free article] [PubMed] [Google Scholar]
- Jankowska E., Roberts W. J. Synaptic actions of single interneurones mediating reciprocal Ia inhibition of motoneurones. J Physiol. 1972 May;222(3):623–642. doi: 10.1113/jphysiol.1972.sp009818. [DOI] [PMC free article] [PubMed] [Google Scholar]
- KATZ B., MILEDI R. A STUDY OF SPONTANEOUS MINIATURE POTENTIALS IN SPINAL MOTONEURONES. J Physiol. 1963 Sep;168:389–422. doi: 10.1113/jphysiol.1963.sp007199. [DOI] [PMC free article] [PubMed] [Google Scholar]
- KATZ B., MILEDI R. THE MEASUREMENT OF SYNAPTIC DELAY, AND THE TIME COURSE OF ACETYLCHOLINE RELEASE AT THE NEUROMUSCULAR JUNCTION. Proc R Soc Lond B Biol Sci. 1965 Feb 16;161:483–495. doi: 10.1098/rspb.1965.0016. [DOI] [PubMed] [Google Scholar]
- KRNJEVIC K., MILEDI R. Failure of neuromuscular propagation in rats. J Physiol. 1958 Mar 11;140(3):440–461. [PMC free article] [PubMed] [Google Scholar]
- KRNJEVIC K., MILEDI R. Presynaptic failure of neuromuscular propagation in rats. J Physiol. 1959 Dec;149:1–22. doi: 10.1113/jphysiol.1959.sp006321. [DOI] [PMC free article] [PubMed] [Google Scholar]
- Katz B., Miledi R. Tetrodotoxin-resistant electric activity in presynaptic terminals. J Physiol. 1969 Aug;203(2):459–487. doi: 10.1113/jphysiol.1969.sp008875. [DOI] [PMC free article] [PubMed] [Google Scholar]
- Katz B., Miledi R. The effect of temperature on the synaptic delay at the neuromuscular junction. J Physiol. 1965 Dec;181(3):656–670. doi: 10.1113/jphysiol.1965.sp007790. [DOI] [PMC free article] [PubMed] [Google Scholar]
- Krnjević K., Lamour Y., MacDonald J. F., Nistri A. Motoneuronal after-potentials and extracellular divalent cations. Can J Physiol Pharmacol. 1978 Jun;56(3):516–520. doi: 10.1139/y78-079. [DOI] [PubMed] [Google Scholar]
- Krnjević K., Puil E., Werman R. EGTA and motoneuronal after-potentials. J Physiol. 1978 Feb;275:199–223. doi: 10.1113/jphysiol.1978.sp012186. [DOI] [PMC free article] [PubMed] [Google Scholar]
- Krnjević K., Puil E., Werman R. Significance of 2,4-dinitrophenol action on spinal motoneurones. J Physiol. 1978 Feb;275:225–239. doi: 10.1113/jphysiol.1978.sp012187. [DOI] [PMC free article] [PubMed] [Google Scholar]
- Loewenstein W. R., Nakas M., Socolar S. J. Junctional membrane uncoupling. Permeability transformations at a cell membrane junction. J Gen Physiol. 1967 Aug;50(7):1865–1891. doi: 10.1085/jgp.50.7.1865. [DOI] [PMC free article] [PubMed] [Google Scholar]
- MACHNE X., FADIGA E., BROOKHART J. M. Antidromic and synaptic activation of frog motor neurons. J Neurophysiol. 1959 Sep;22:483–503. doi: 10.1152/jn.1959.22.5.483. [DOI] [PubMed] [Google Scholar]
- Magherini P. C., Precht W. Electrical properties of frog motoneurons in the in situ spinal cord. J Neurophysiol. 1976 May;39(3):459–473. doi: 10.1152/jn.1976.39.3.459. [DOI] [PubMed] [Google Scholar]
- McLachlan E. M. The effects of strontium and barium ions at synapses in sympathetic ganglia. J Physiol. 1977 May;267(2):497–518. doi: 10.1113/jphysiol.1977.sp011823. [DOI] [PMC free article] [PubMed] [Google Scholar]
- Meiri U., Rahamimoff R. Neuromuscular transmission: inhibition by manganese ions. Science. 1972 Apr 21;176(4032):308–309. doi: 10.1126/science.176.4032.308. [DOI] [PubMed] [Google Scholar]
- Miledi R., Slater C. R. The action of calcium on neuronal synapses in the squid. J Physiol. 1966 May;184(2):473–498. doi: 10.1113/jphysiol.1966.sp007927. [DOI] [PMC free article] [PubMed] [Google Scholar]
- Miledi R., Szczepaniak A. C. Effect of Dendroaspis neurotoxins on synaptic transmission in the spinal cord of the frog. Proc R Soc Lond B Biol Sci. 1975 Jul 1;190(1099):267–274. doi: 10.1098/rspb.1975.0092. [DOI] [PubMed] [Google Scholar]
- Miledi R., Thies R. Tetanic and post-tetanic rise in frequency of miniature end-plate potentials in low-calcium solutions. J Physiol. 1971 Jan;212(1):245–257. doi: 10.1113/jphysiol.1971.sp009320. [DOI] [PMC free article] [PubMed] [Google Scholar]
- Miledi R. Transmitter release induced by injection of calcium ions into nerve terminals. Proc R Soc Lond B Biol Sci. 1973 Jul 3;183(1073):421–425. doi: 10.1098/rspb.1973.0026. [DOI] [PubMed] [Google Scholar]
- PORTZEHL H., CALDWELL P. C., RUEEGG J. C. THE DEPENDENCE OF CONTRACTION AND RELAXATION OF MUSCLE FIBRES FROM THE CRAB MAIA SQUINADO ON THE INTERNAL CONCENTRATION OF FREE CALCIUM IONS. Biochim Biophys Acta. 1964 May 25;79:581–591. doi: 10.1016/0926-6577(64)90224-4. [DOI] [PubMed] [Google Scholar]
- Peracchia C., Dulhunty A. F. Low resistance junctions in crayfish. Structural changes with functional uncoupling. J Cell Biol. 1976 Aug;70(2 Pt 1):419–439. doi: 10.1083/jcb.70.2.419. [DOI] [PMC free article] [PubMed] [Google Scholar]
- Rall W., Burke R. E., Smith T. G., Nelson P. G., Frank K. Dendritic location of synapses and possible mechanisms for the monosynaptic EPSP in motoneurons. J Neurophysiol. 1967 Sep;30(5):1169–1193. doi: 10.1152/jn.1967.30.5.1169. [DOI] [PubMed] [Google Scholar]
- Rall W. Distinguishing theoretical synaptic potentials computed for different soma-dendritic distributions of synaptic input. J Neurophysiol. 1967 Sep;30(5):1138–1168. doi: 10.1152/jn.1967.30.5.1138. [DOI] [PubMed] [Google Scholar]
- Shapovalov A. I., Shiriaev B. I. Electrical coupling between primary afferents and amphibian motoneurons. Exp Brain Res. 1978 Nov 15;33(3-4):299–312. doi: 10.1007/BF00235555. [DOI] [PubMed] [Google Scholar]
- Shapovalov A. I., Shiriaev B. I., Velumian A. A. Mechanisms of post-synaptic excitation in amphibian motoneurones. J Physiol. 1978 Jun;279:437–455. doi: 10.1113/jphysiol.1978.sp012355. [DOI] [PMC free article] [PubMed] [Google Scholar]
- Silinsky E. M. On the role of barium in supporting the asynchronous release of acetylcholine quanta by motor nerve impulses. J Physiol. 1978 Jan;274:157–171. doi: 10.1113/jphysiol.1978.sp012141. [DOI] [PMC free article] [PubMed] [Google Scholar]
- Sonnhof U., Richter D. W., Taugner R. Electrotonic coupling between frog spinal motoneurons. An electrophysiological and morphological study. Brain Res. 1977 Dec 16;138(2):197–215. doi: 10.1016/0006-8993(77)90740-5. [DOI] [PubMed] [Google Scholar]
- Sotelo C., Taxi J. Ultrastructural aspects of electrotonic junctions in the spinal cord of the frog. Brain Res. 1970 Jan 6;17(1):137–141. doi: 10.1016/0006-8993(70)90315-x. [DOI] [PubMed] [Google Scholar]
- Székely G. The morphology of motoneurons and dorsal root fibers in the frog's spinal cord. Brain Res. 1976 Feb 20;103(2):275–290. doi: 10.1016/0006-8993(76)90799-x. [DOI] [PubMed] [Google Scholar]
- Watt D. G., Stauffer E. K., Taylor A., Reinking R. M., Stuart D. G. Analysis of muscle receptor connections by spike-triggered averaging. 1. Spindle primary and tendon organ afferents. J Neurophysiol. 1976 Nov;39(6):1375–1392. doi: 10.1152/jn.1976.39.6.1375. [DOI] [PubMed] [Google Scholar]
- Weakly J. N. The action of cobalt ions on neuromuscular transmission in the frog. J Physiol. 1973 Nov;234(3):597–612. doi: 10.1113/jphysiol.1973.sp010363. [DOI] [PMC free article] [PubMed] [Google Scholar]
- Werman R., Carlen P. L. Unusual behavior of the La EPSP in cat spinal motoneurons. Brain Res. 1976 Aug 13;112(2):395–401. doi: 10.1016/0006-8993(76)90294-8. [DOI] [PubMed] [Google Scholar]