Abstract
Resonance Raman spectra are reported for the organometallic phenyl-FeIII complexes of horse heart myoglobin. We observed the resonance enhancement of the ring vibrational modes of the bound phenyl group. They were identified at 642, 996, 1,009, and 1,048 cm-1, which shift to 619, 961, 972, and 1,030 cm-1, respectively, upon phenyl 13C substitution. The lines at 642 and 996 cm-1 are assigned, respectively, as in-plane phenyl ring deformation mode (derived from benzene vibration No. 6a at 606 cm-1) and out-of-plane CH deformation (derived from benzene vibration No. 5 at 995 cm-1). The frequencies of the ring "breathing" modes at 1,009 and 1,048 cm-1 are higher than the corresponding ones in phenylalanine (at 1,004 and 1,033 cm-1) and benzene (at 992 and 1,010 cm-1), indicating that the ring C--C bonds are strengthened (or shortened) when coordinated to the heme iron. The excitation profiles of these phenyl ring modes and a porphyrin ring vibrational mode at 674 cm-1 exhibit peaks near its Soret absorption maximum at 431 nm. This appears to indicate that these phenyl ring modes may be enhanced via resonance with the Soret pi-pi transition. The FeIII--C bond stretching vibration has not been detected with excitation wavelengths in the 406.7-457.9-nm region.
Full text
PDF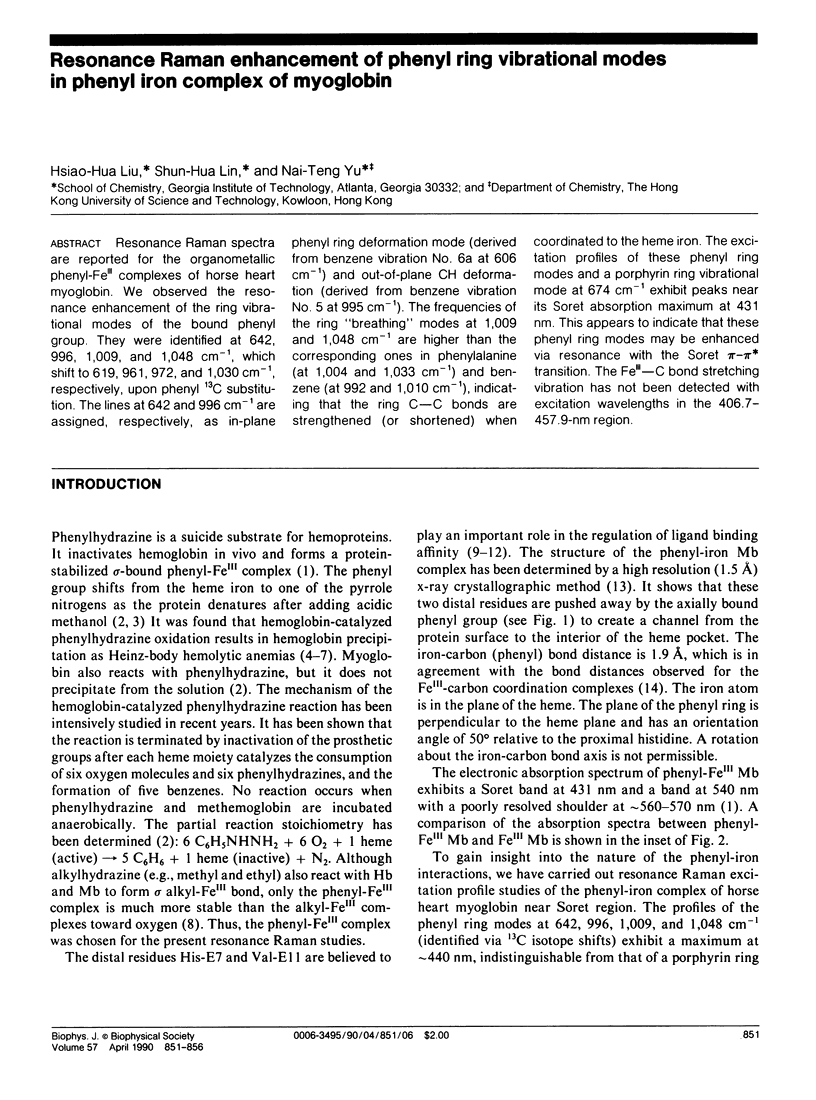
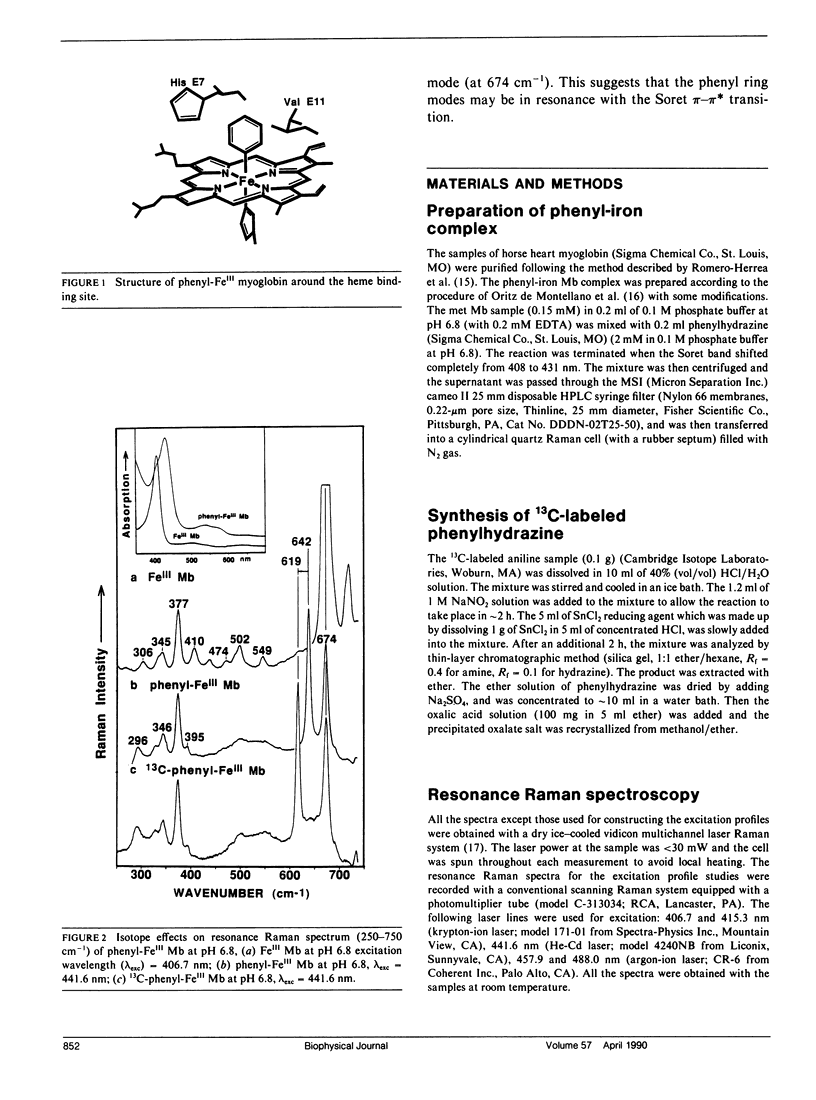
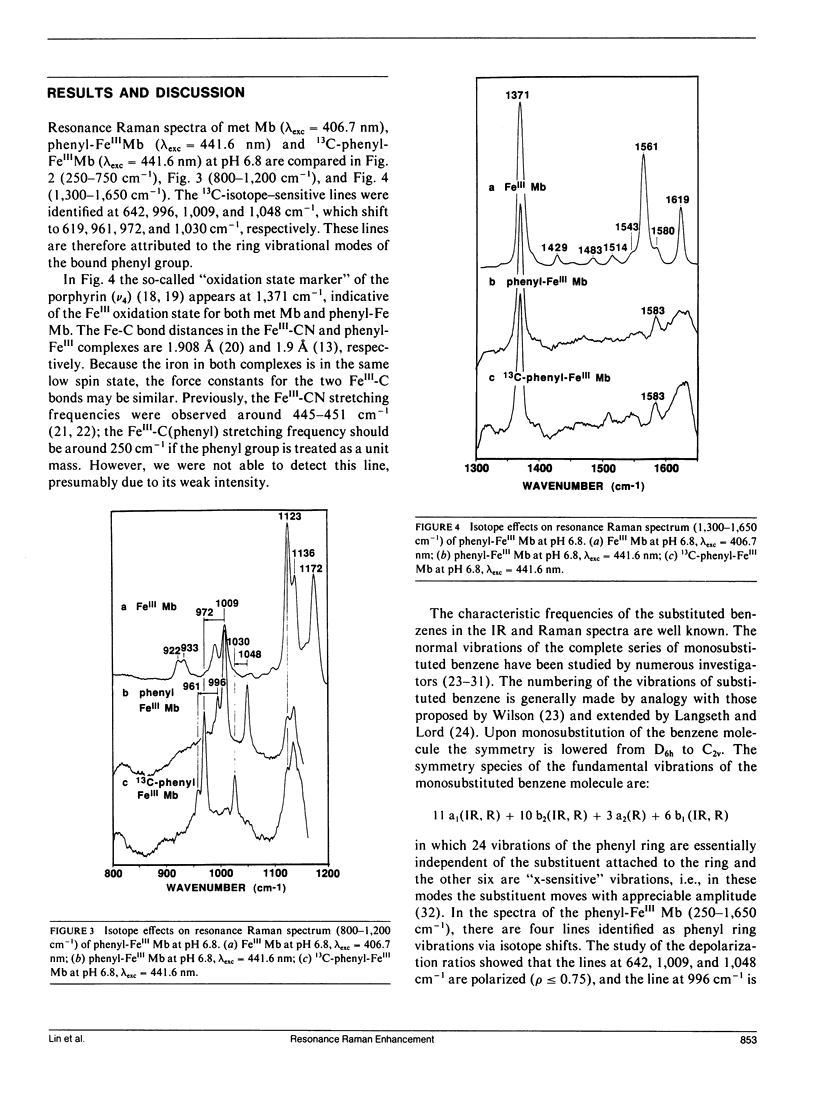
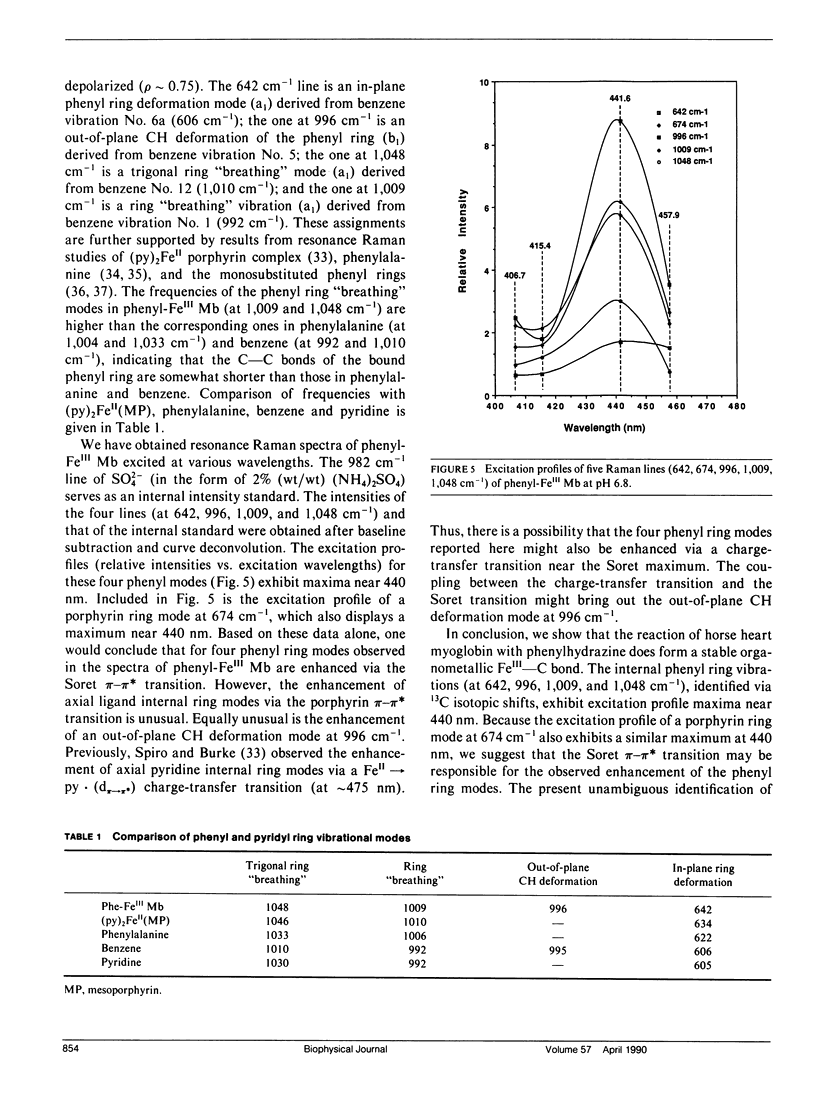
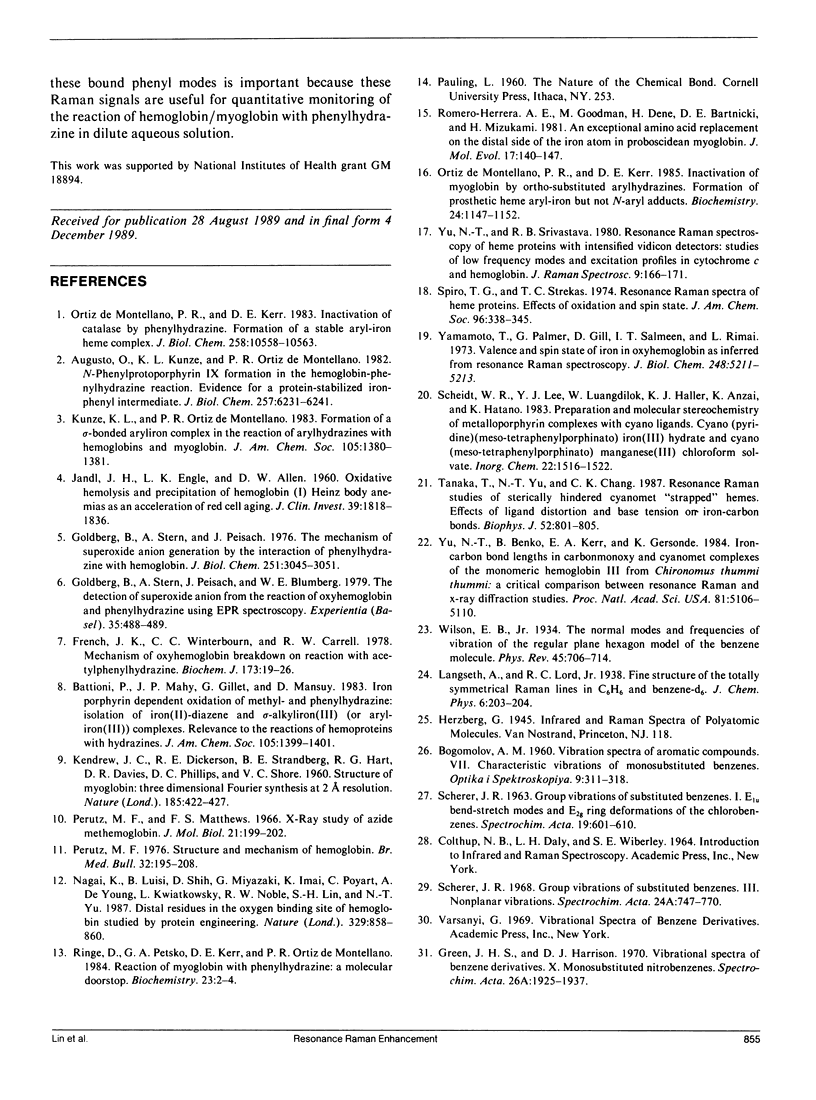
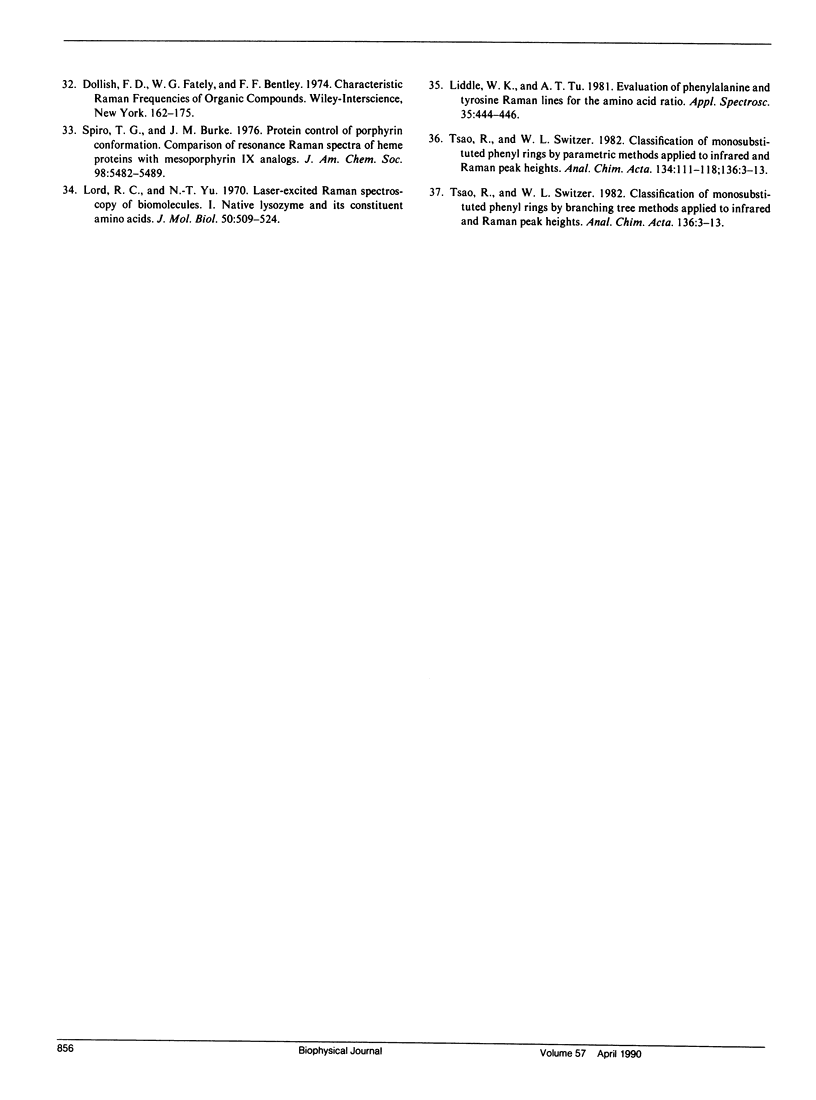
Selected References
These references are in PubMed. This may not be the complete list of references from this article.
- Augusto O., Kunze K. L., Ortiz de Montellano P. R. N-Phenylprotoporphyrin IX formation in the hemoglobin-phenylhydrazine reaction. Evidence for a protein-stabilized iron-phenyl intermediate. J Biol Chem. 1982 Jun 10;257(11):6231–6241. [PubMed] [Google Scholar]
- French J. K., Winterbourn C. C., Carrell R. W. Mechanism of oxyhaemoglobin breakdown on reaction with acetylphenylhydrazine. Biochem J. 1978 Jul 1;173(1):19–26. doi: 10.1042/bj1730019. [DOI] [PMC free article] [PubMed] [Google Scholar]
- Goldberg B., Stern A., Peisach J., Blumberg W. E. The detection of superoxide anion from the reaction of oxyhemoglobin and phenylhydrazine using EPR spectroscopy. Experientia. 1979 Apr 15;35(4):488–489. doi: 10.1007/BF01922723. [DOI] [PubMed] [Google Scholar]
- Goldberg B., Stern A., Peisach J. The mechanism of superoxide anion generation by the interaction of phenylhydrazine with hemoglobin. J Biol Chem. 1976 May 25;251(10):3045–3051. [PubMed] [Google Scholar]
- JANDL J. H., ENGLE L. K., ALLEN D. W. Oxidative hemolysis and precipitation of hemoglobin. I. Heinz body anemias as an acceleration of red cell aging. J Clin Invest. 1960 Dec;39:1818–1836. doi: 10.1172/JCI104206. [DOI] [PMC free article] [PubMed] [Google Scholar]
- Lord R. C., Yu N. T. Laser-excited Raman spectroscopy of biomolecules. I. Native lysozyme and its constituent amino acids. J Mol Biol. 1970 Jun 14;50(2):509–524. doi: 10.1016/0022-2836(70)90208-1. [DOI] [PubMed] [Google Scholar]
- Nagai K., Luisi B., Shih D., Miyazaki G., Imai K., Poyart C., De Young A., Kwiatkowsky L., Noble R. W., Lin S. H. Distal residues in the oxygen binding site of haemoglobin studied by protein engineering. 1987 Oct 29-Nov 4Nature. 329(6142):858–860. doi: 10.1038/329858a0. [DOI] [PubMed] [Google Scholar]
- Ortiz de Montellano P. R., Kerr D. E. Inactivation of catalase by phenylhydrazine. Formation of a stable aryl-iron heme complex. J Biol Chem. 1983 Sep 10;258(17):10558–10563. [PubMed] [Google Scholar]
- Ortiz de Montellano P. R., Kerr D. E. Inactivation of myoglobin by ortho-substituted arylhydrazines. Formation of prosthetic heme aryl-iron but not N-aryl adducts. Biochemistry. 1985 Feb 26;24(5):1147–1152. doi: 10.1021/bi00326a013. [DOI] [PubMed] [Google Scholar]
- Perutz M. F., Mathews F. S. An x-ray study of azide methaemoglobin. J Mol Biol. 1966 Oct 28;21(1):199–202. doi: 10.1016/0022-2836(66)90088-x. [DOI] [PubMed] [Google Scholar]
- Perutz M. F. Structure and mechanism of haemoglobin. Br Med Bull. 1976 Sep;32(3):195–208. doi: 10.1093/oxfordjournals.bmb.a071363. [DOI] [PubMed] [Google Scholar]
- Romero-Herrera A. E., Goodman M., Dene H., Bartnicki D. E., Mizukami H. An exceptional amino acid replacement on the distal side of the iron atom in proboscidean myoglobin. J Mol Evol. 1981;17(3):140–147. doi: 10.1007/BF01733907. [DOI] [PubMed] [Google Scholar]
- Spiro T. G., Burke J. M. Protein control of porphyrin conformation. Comparison of resonance Raman spectra of heme proteins with mesoporphyrin IX analogues. J Am Chem Soc. 1976 Sep 1;98(18):5482–5489. doi: 10.1021/ja00434a013. [DOI] [PubMed] [Google Scholar]
- Spiro T. G., Strekas T. C. Resonance Raman spectra of heme proteins. Effects of oxidation and spin state. J Am Chem Soc. 1974 Jan 23;96(2):338–345. doi: 10.1021/ja00809a004. [DOI] [PubMed] [Google Scholar]
- Tanaka T., Yu N. T., Chang C. K. Resonance Raman studies of sterically hindered cyanomet "strapped" hemes. Effects of ligand distortion and base tension on iron-carbon bond. Biophys J. 1987 Nov;52(5):801–805. doi: 10.1016/S0006-3495(87)83274-5. [DOI] [PMC free article] [PubMed] [Google Scholar]
- Yammoto T., Palmer G. The valence and spin state of iron in oxyhemoglobin as inferred from resonance Raman spectroscopy. J Biol Chem. 1973 Jul 25;248(14):5211–5213. [PubMed] [Google Scholar]
- Yu N. T., Benko B., Kerr E. A., Gersonde K. Iron-carbon bond lengths in carbonmonoxy and cyanomet complexes of the monomeric hemoglobin III from Chironomus thummi thummi: a critical comparison between resonance Raman and x-ray diffraction studies. Proc Natl Acad Sci U S A. 1984 Aug;81(16):5106–5110. doi: 10.1073/pnas.81.16.5106. [DOI] [PMC free article] [PubMed] [Google Scholar]