Abstract
1. The possible function of sialic acid-containing substrates (SACS) in synaptic terminals of Aplysia was studied by intracellular injection of ruthenium red and of neuraminidase. 2. Ruthenium red, a dye known to have sialic acid as a molecular target, blocked transmission irreversibly in both cholinergic (buccal ganglion) and non-cholinergic (cerebral ganglion) synapses. 3. An intracellular site of action is likely because much less ruthenium red was necessary to block transmission when it was injected intracellularly than when it was presented by bath perfusion. 4. Ca2+ spikes recorded in the presence of tetrodotoxin or in Na+-free solution were not modified by ruthenium red or neuraminidase injections or perfusions. It is therefore improbable that these substances blocked transmission by blocking voltage-dependent Ca2+ influx. 5. Strong electrotonic depolarization of a pre-synaptic interneurone in the presence of 10(-4) M-tetrodotoxin caused a sustained post-synaptic response, which was abolished by ruthenium red. This result eliminates axonal conduction block as the principal mechanism of ruthenium red action. 6. Post-synaptic responses to ionophoretically applied acetylcholine (ACh) were not modified by bath perfusion of 2 x 10(-2) M-ruthenium red. 7. Biochemical analysis of pools of [3H]ACh was performed after injection of a precursor, [3H]acetate, into an identified interneurone. Ruthenium red appeared to increase significantly the 'free' (cytoplasmic) ACh pool without any change of 'bound' (vesicular) [3H]ACh-pool. 8. A model is proposed in which SACS act as intracellular Ca2+ receptors involved in transmitter release.
Full text
PDF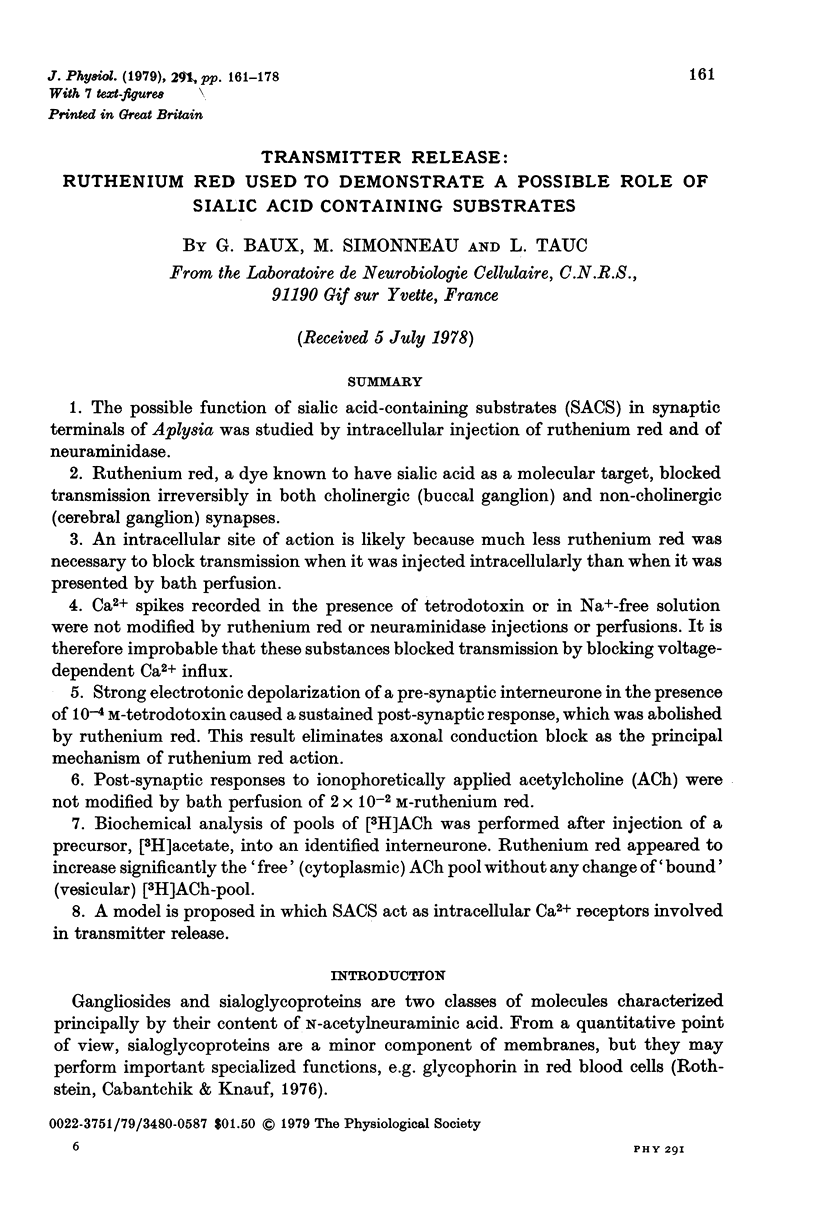
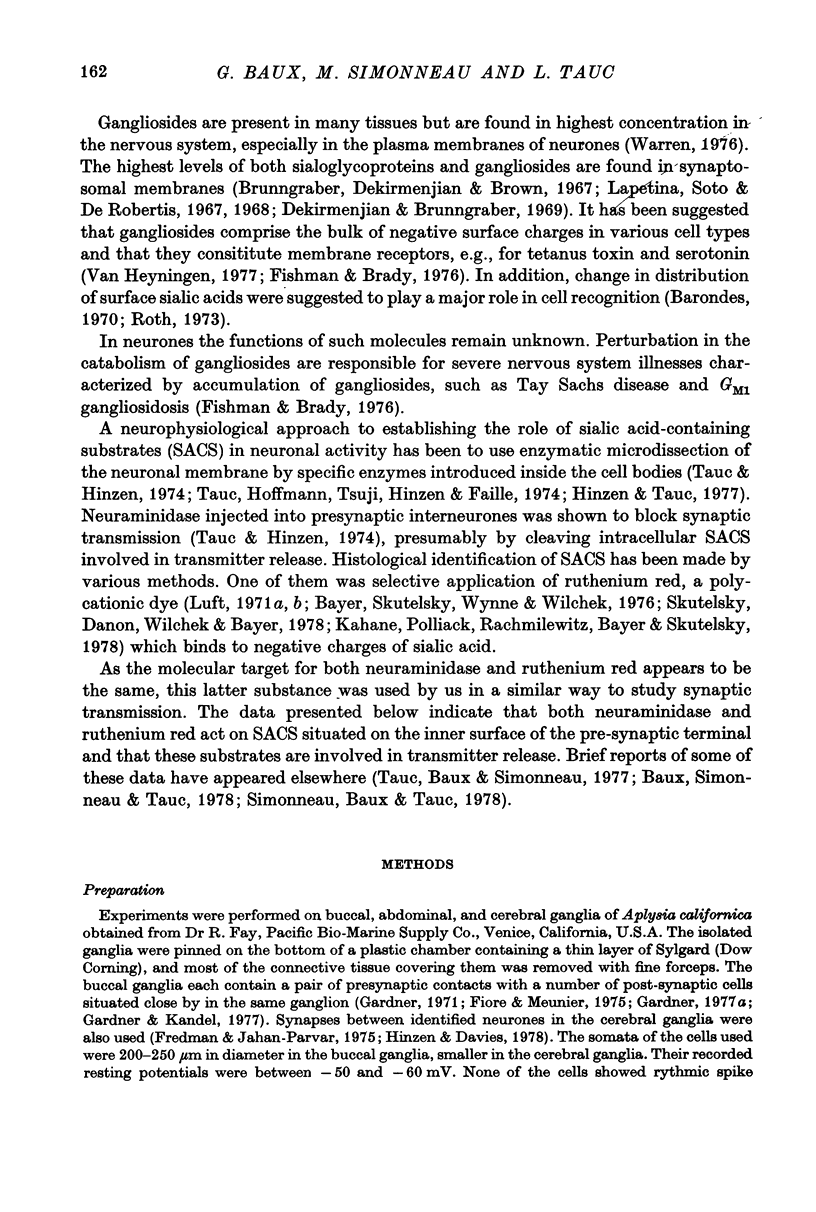
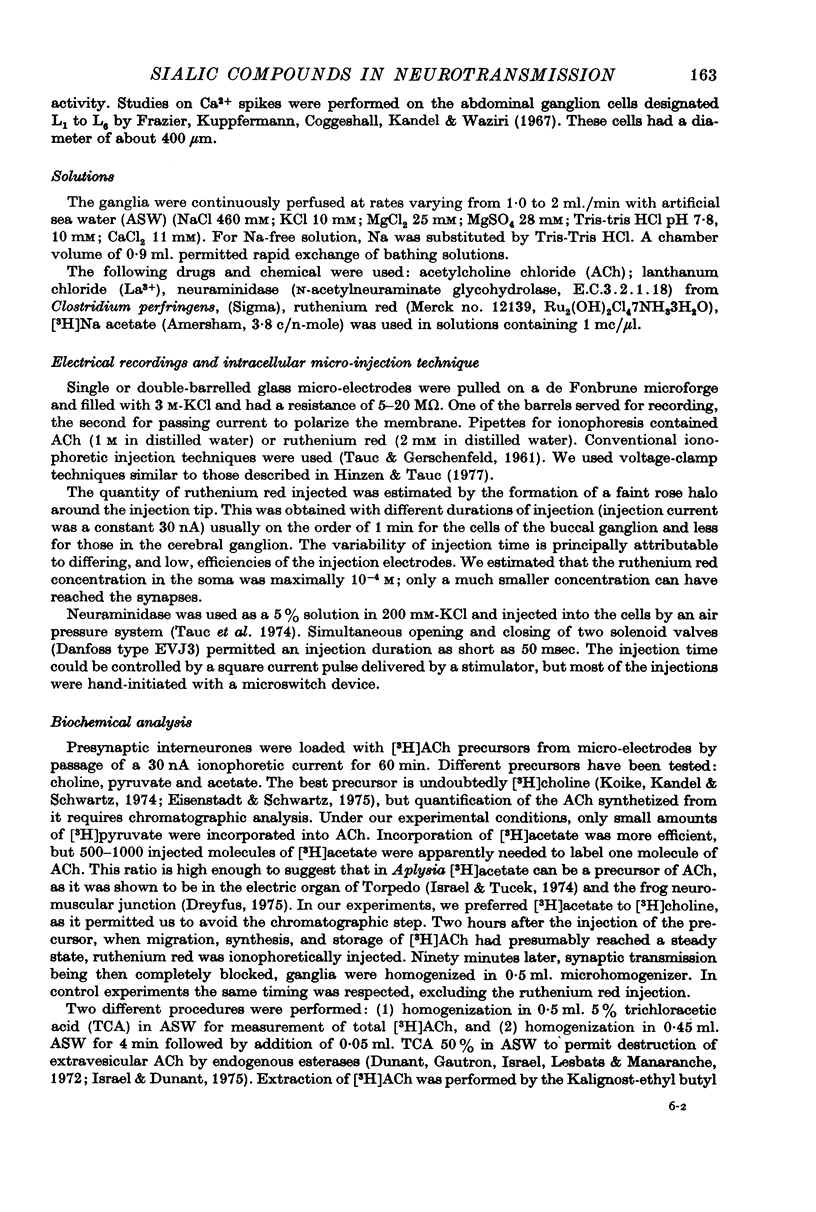
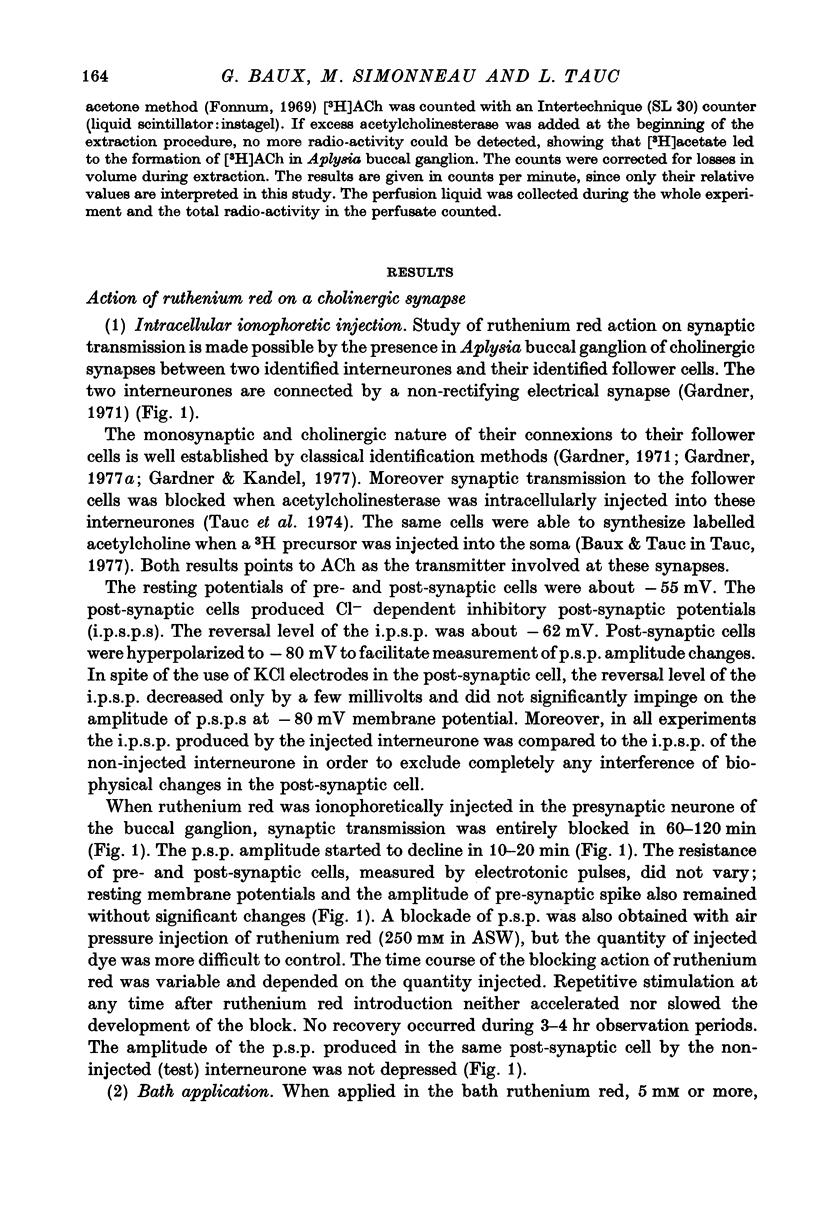
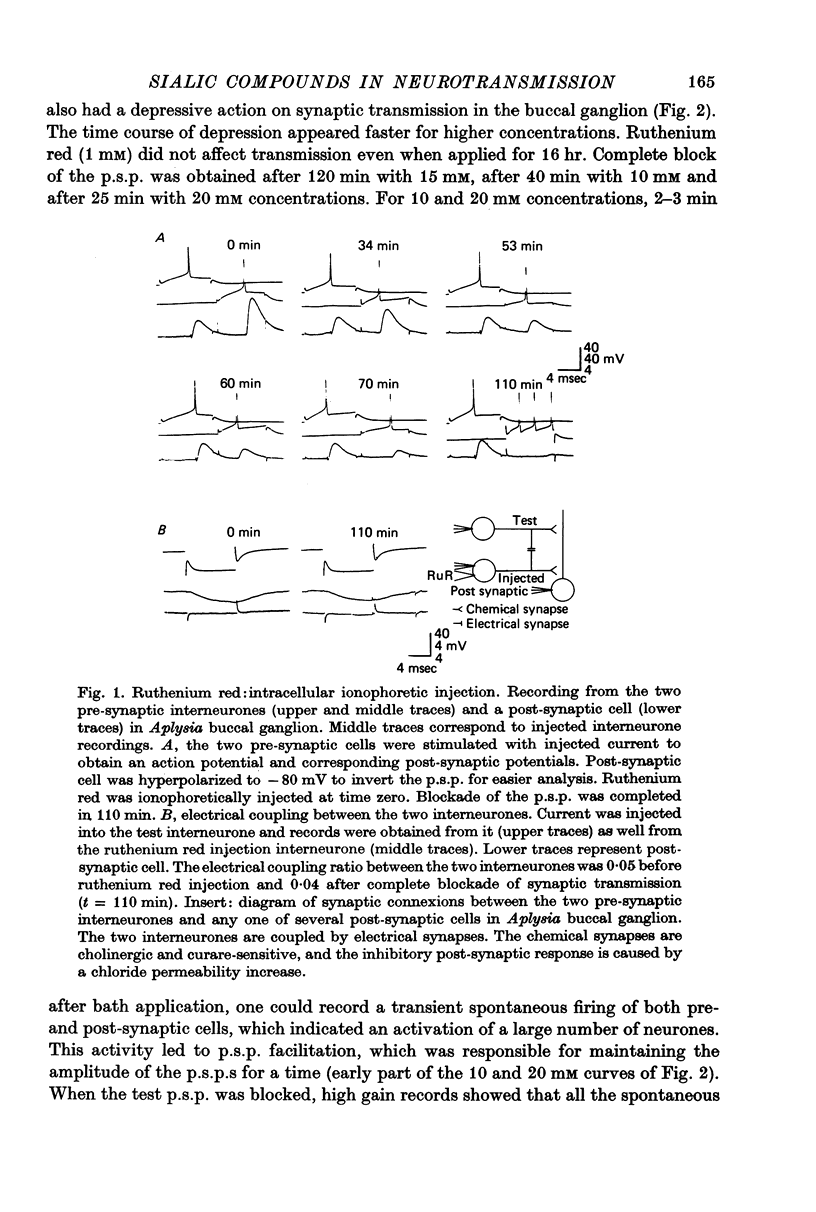
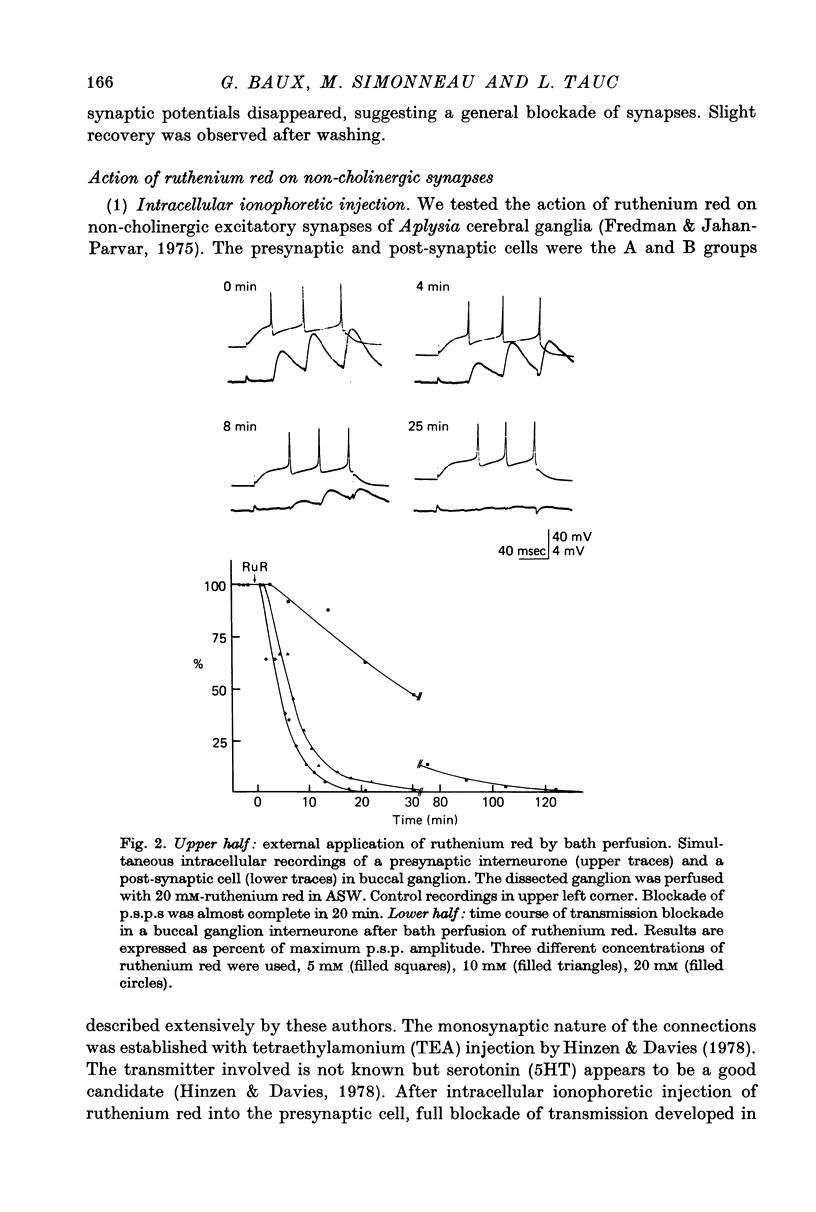
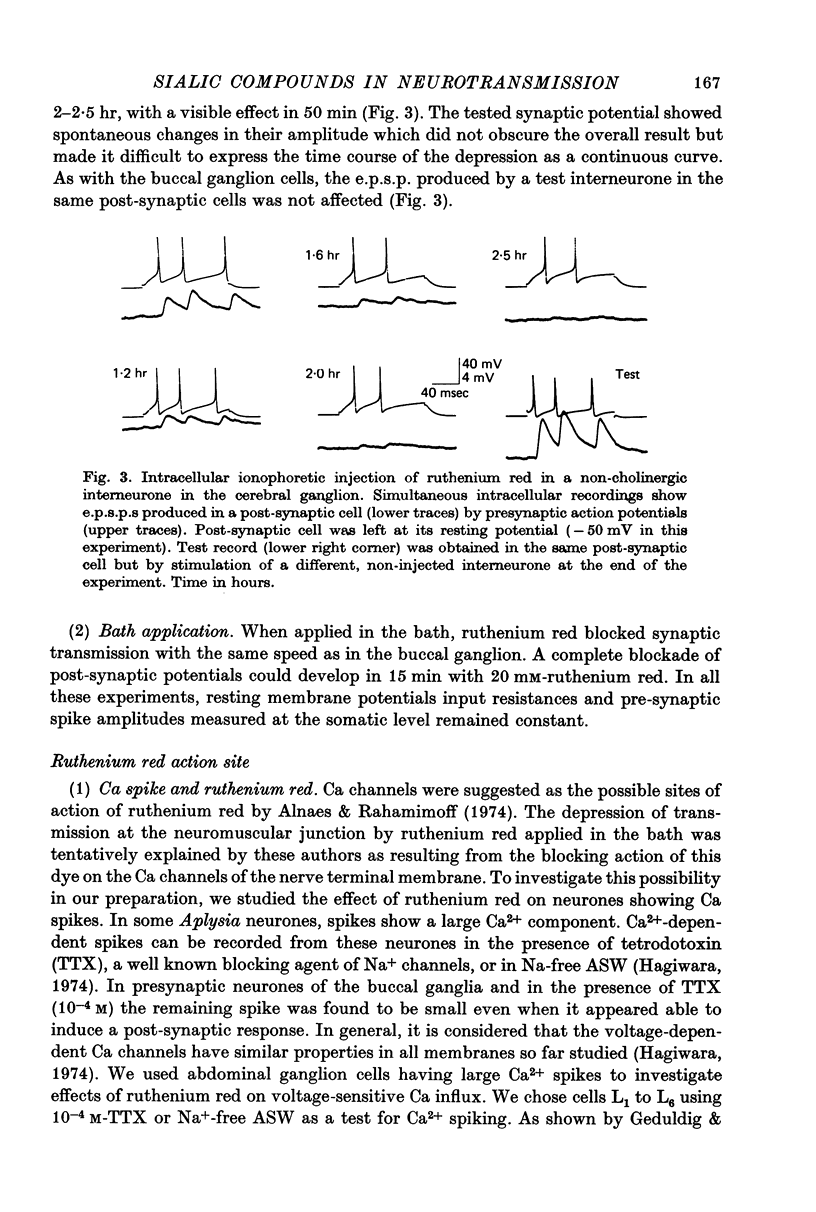
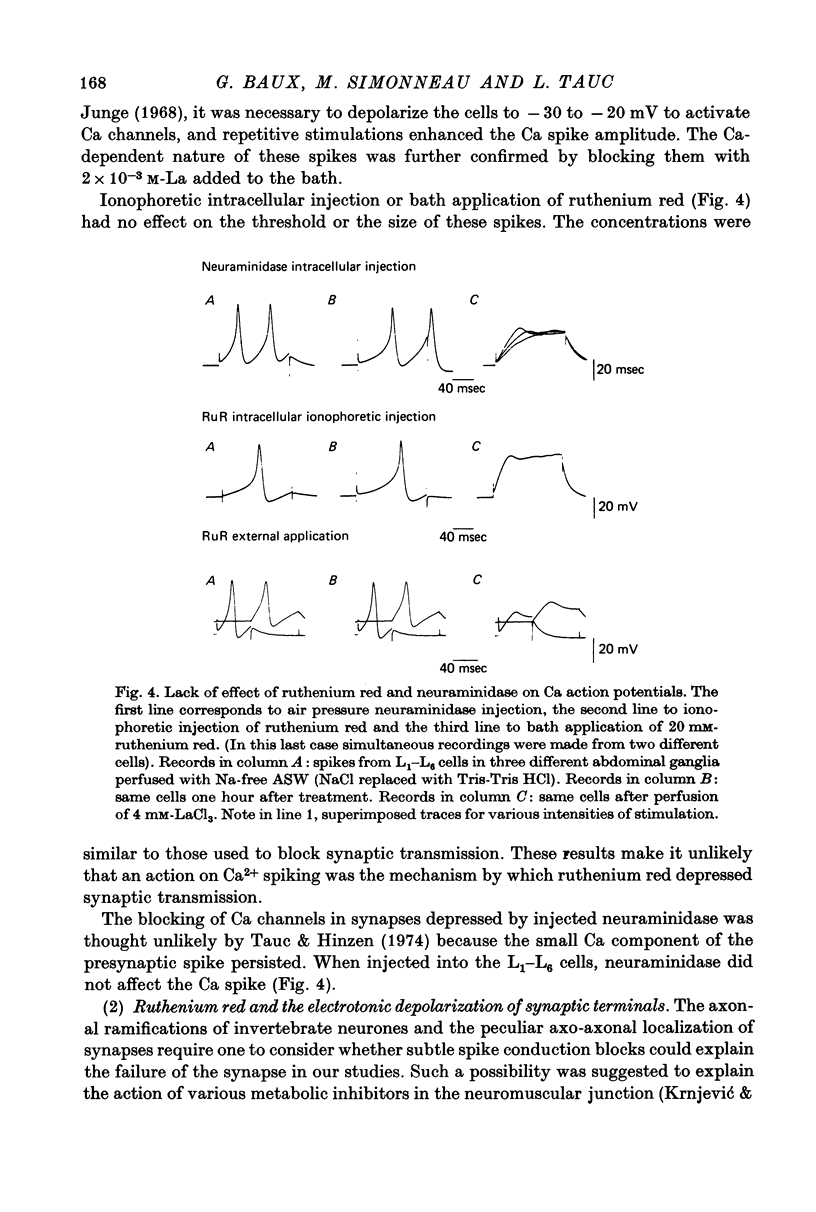
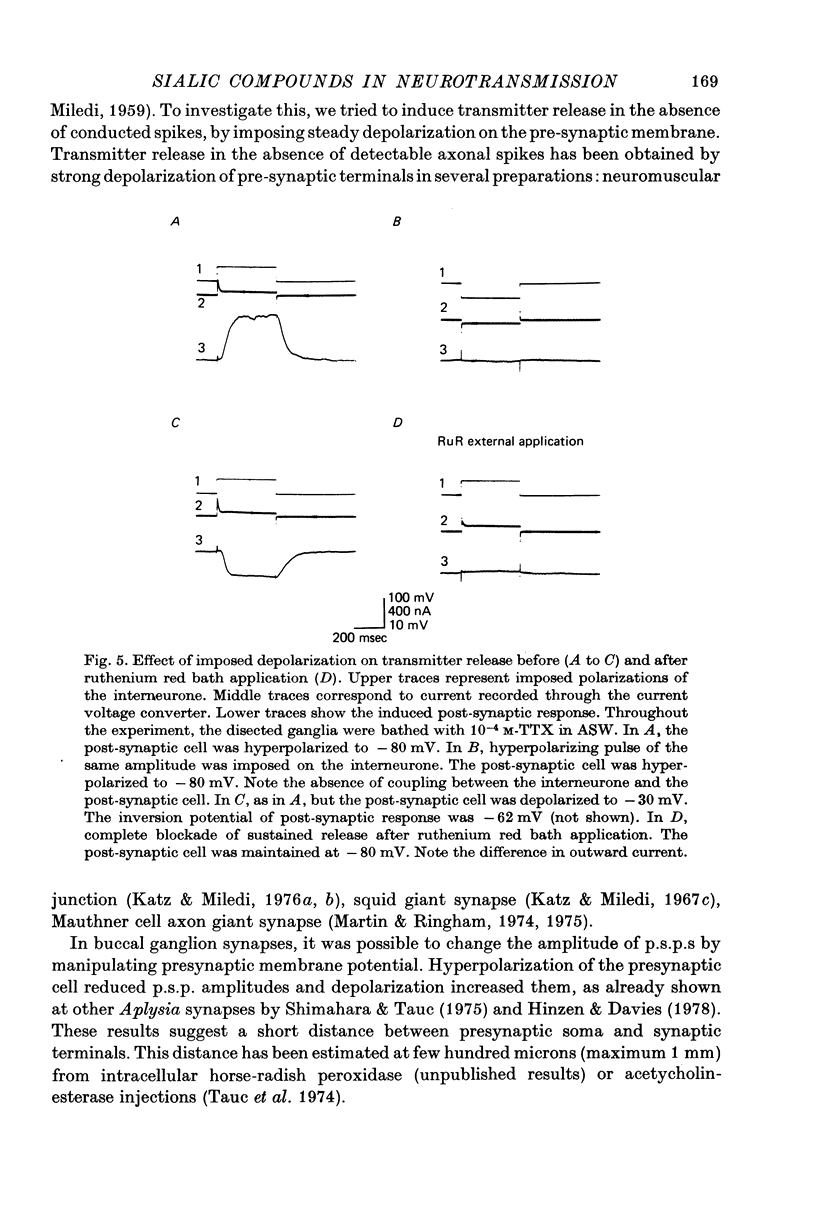
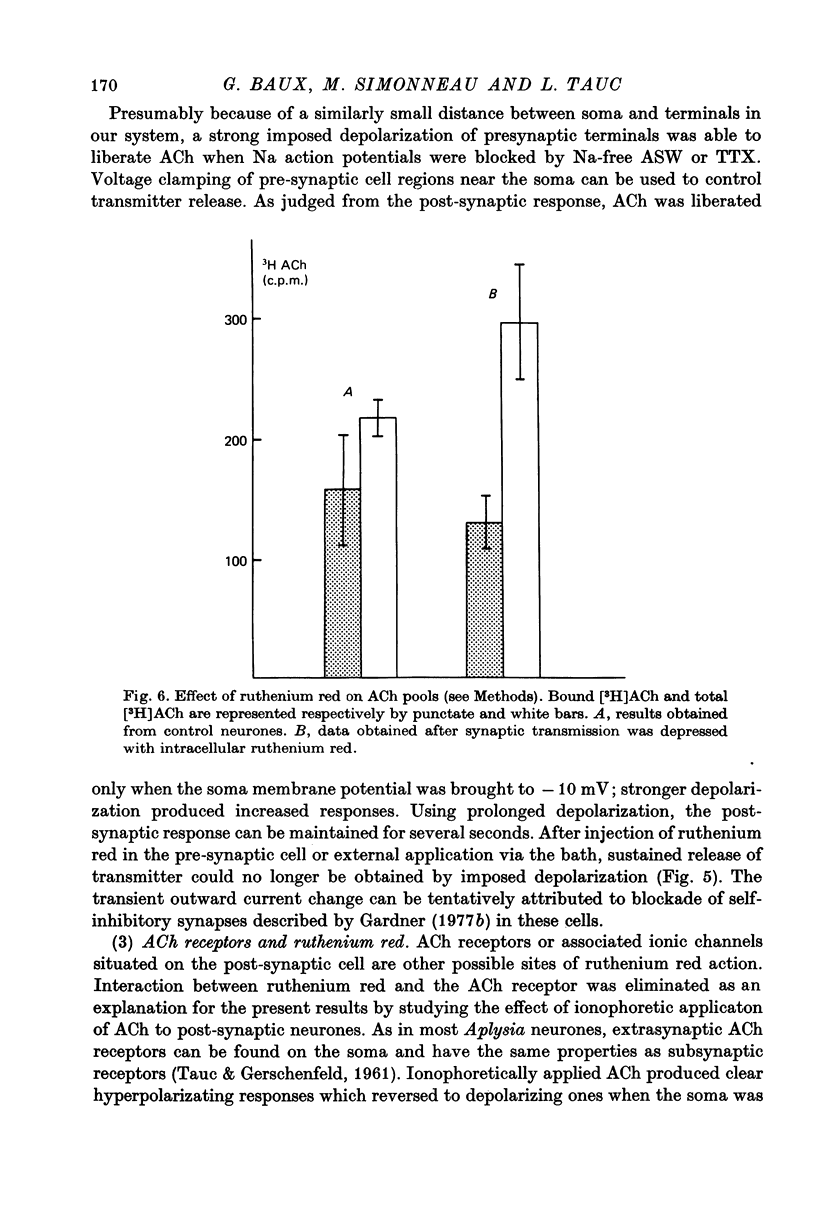
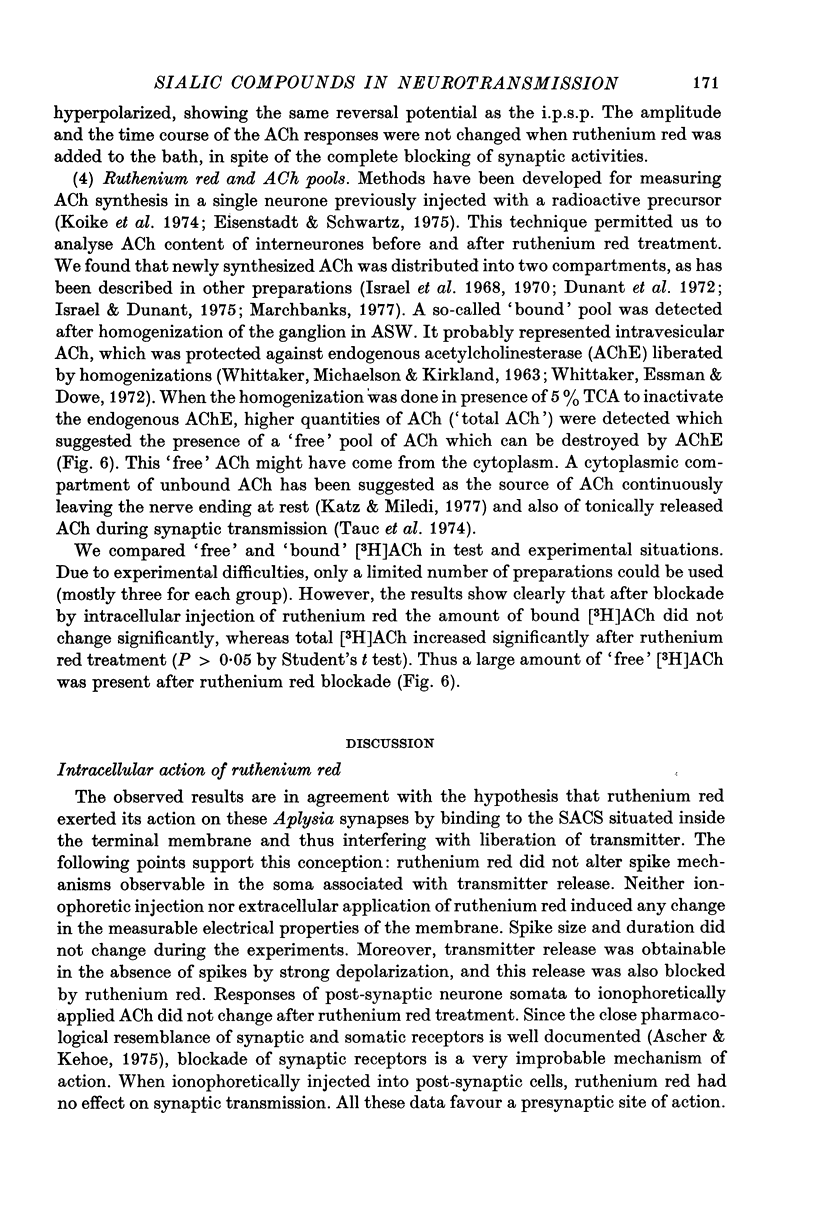
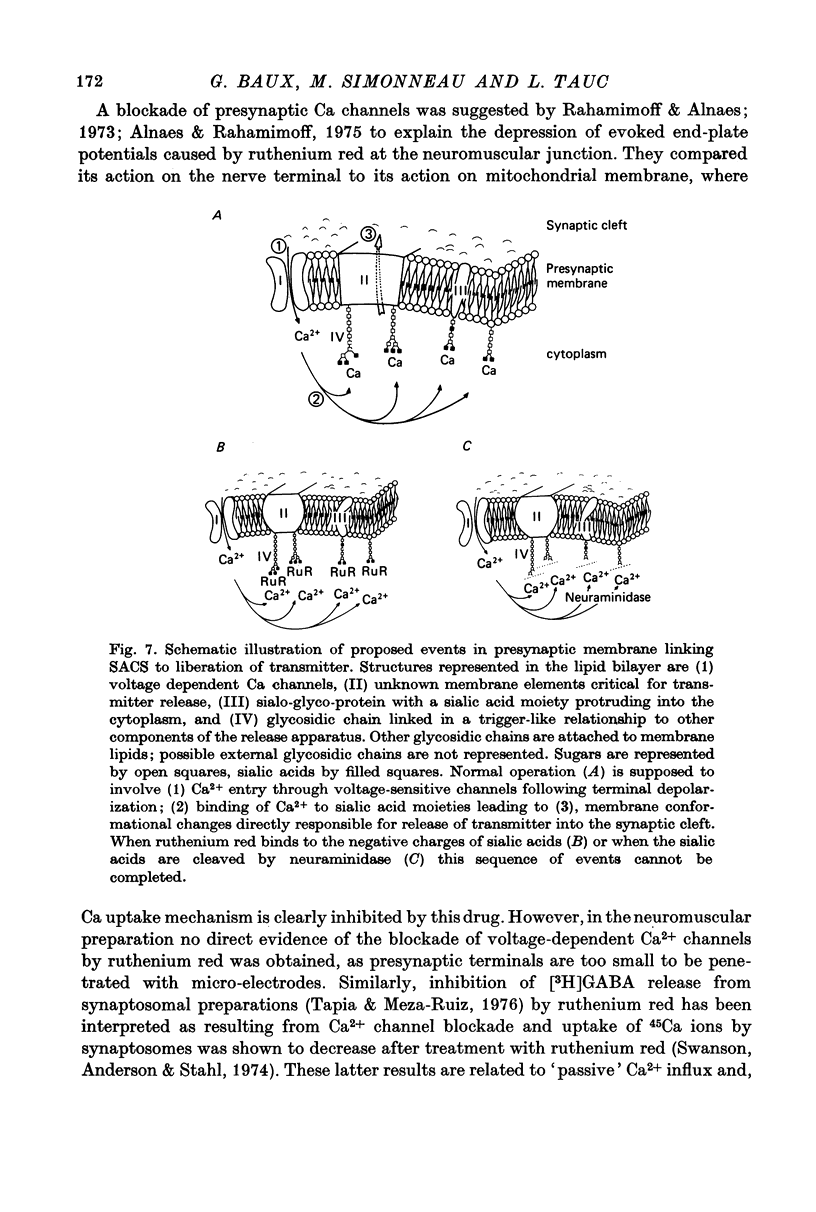
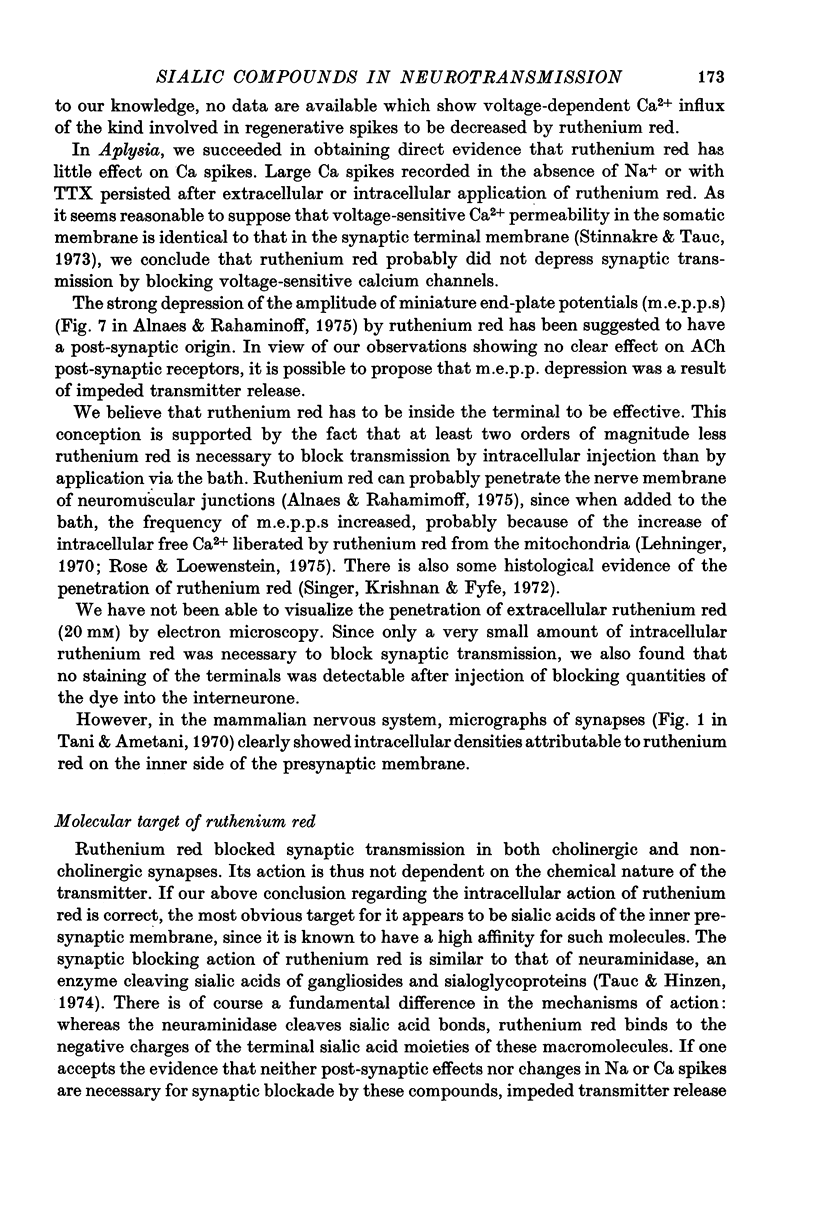
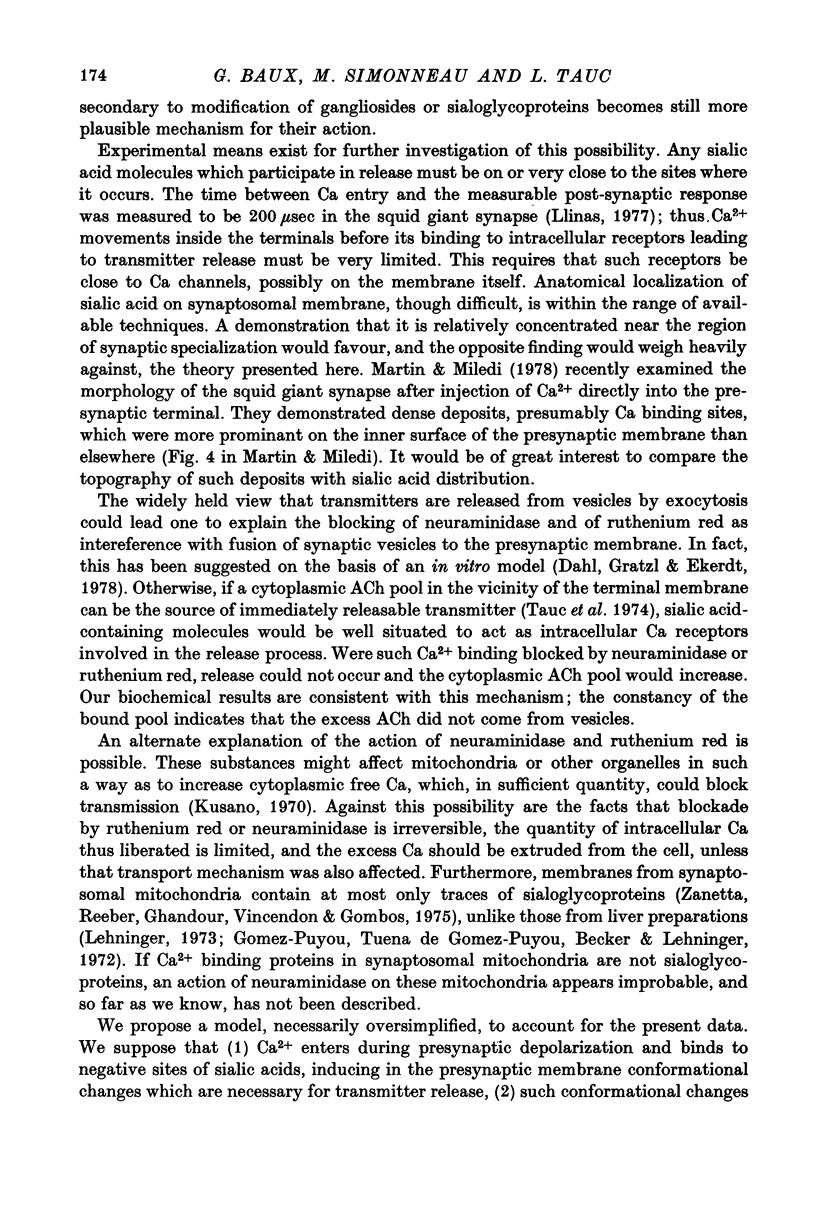
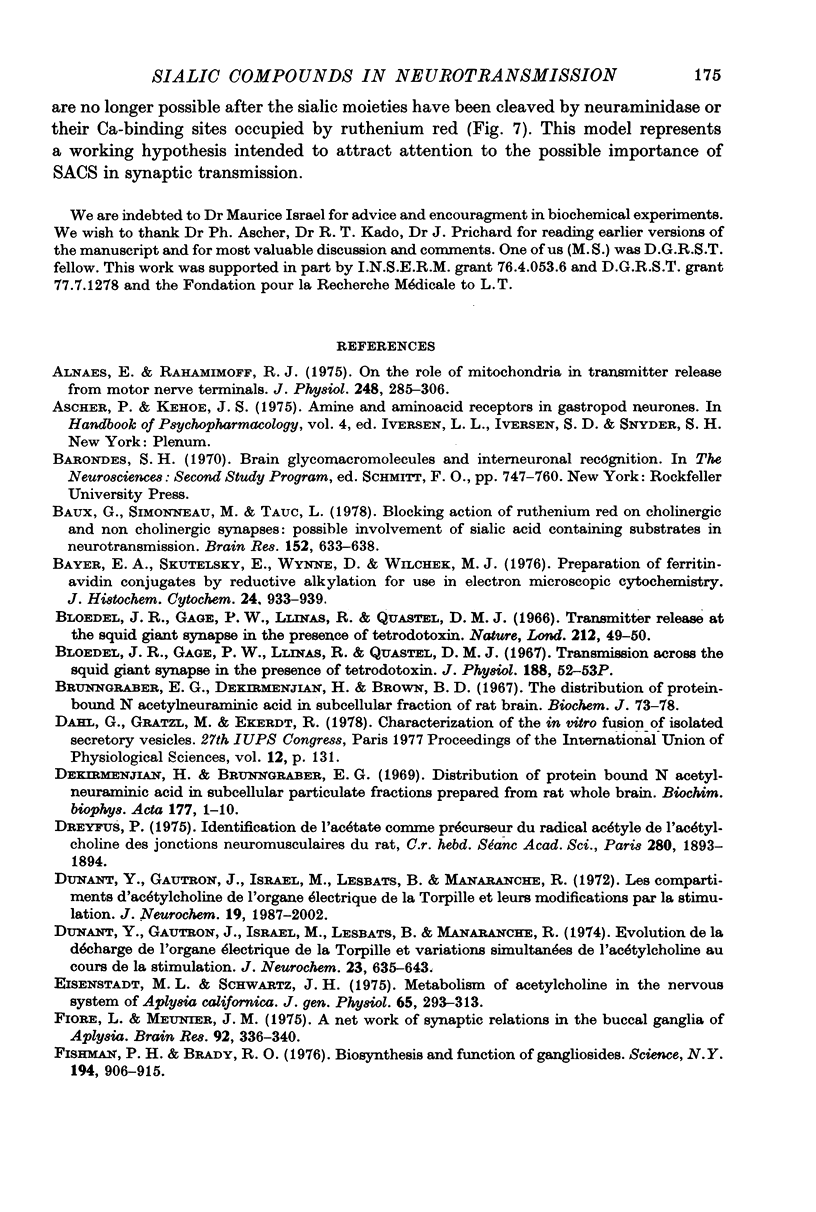
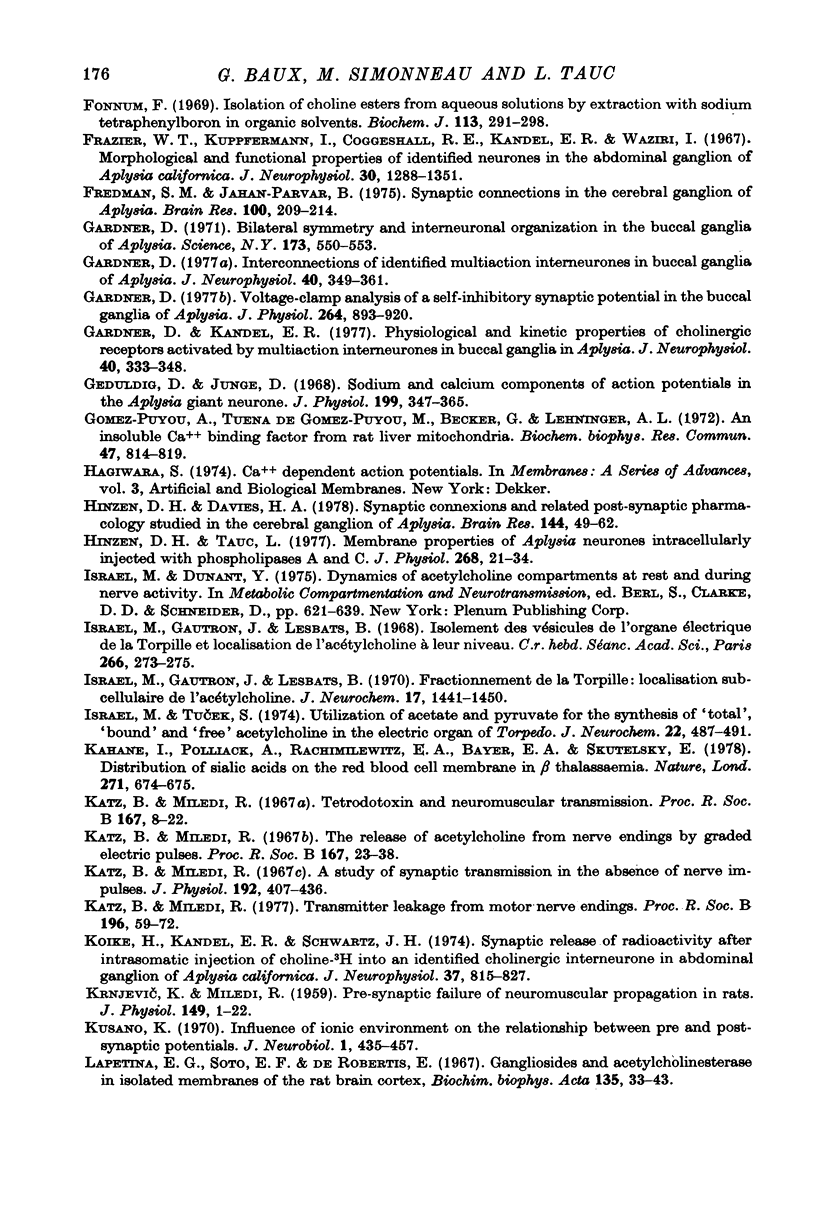
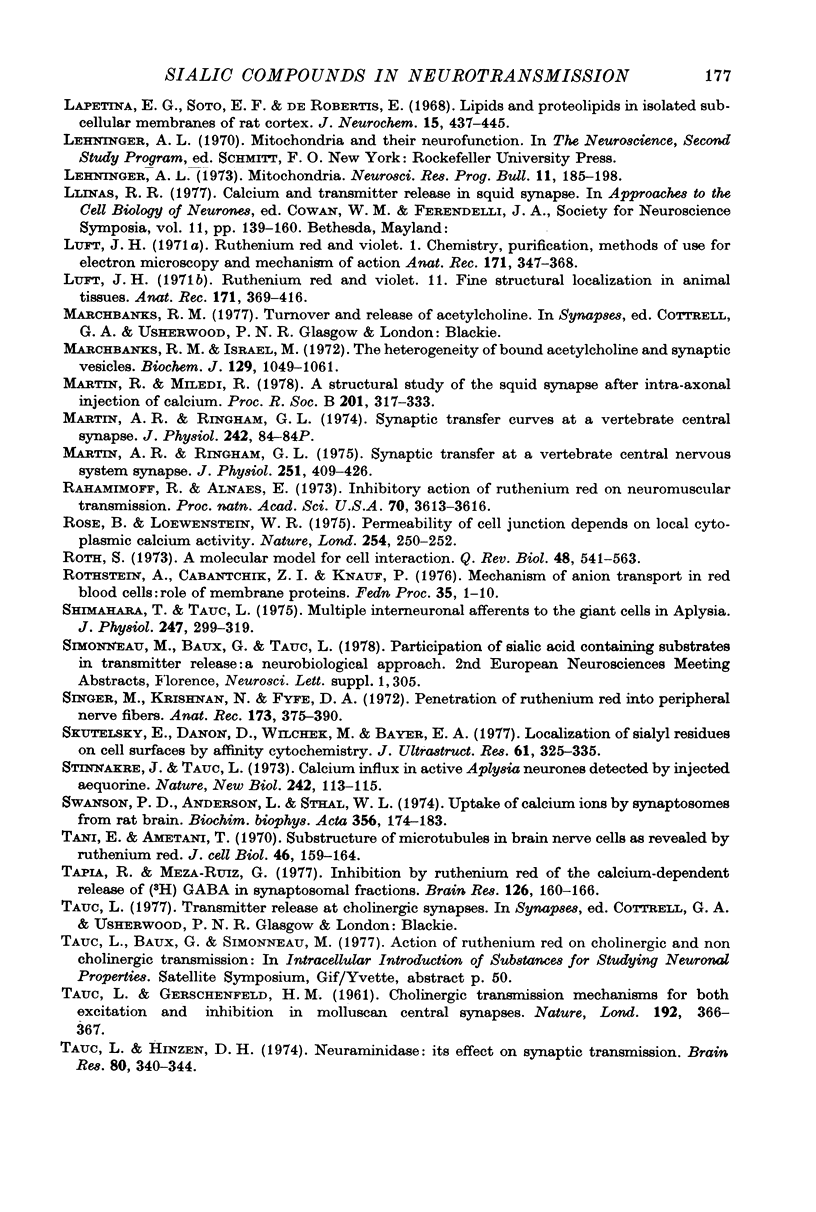
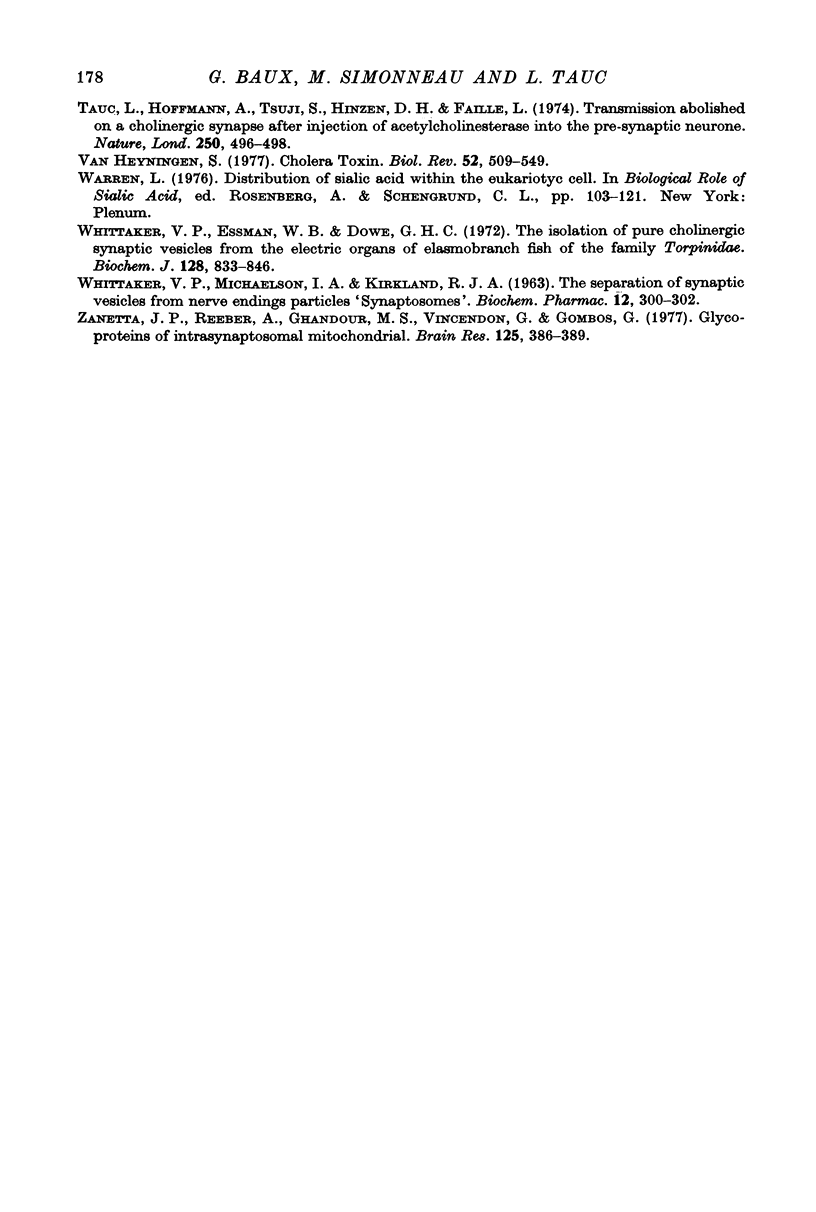
Selected References
These references are in PubMed. This may not be the complete list of references from this article.
- Alnaes E., Rahamimoff R. On the role of mitochondria in transmitter release from motor nerve terminals. J Physiol. 1975 Jun;248(2):285–306. doi: 10.1113/jphysiol.1975.sp010974. [DOI] [PMC free article] [PubMed] [Google Scholar]
- Baux G., Simonneau M., Tauc L. Blocking action of Ruthenium Red on cholinergic and non-cholinergic synapses: possible involvment of sialic acid-containing substrates in neurotransmission. Brain Res. 1978 Sep 8;152(3):633–638. doi: 10.1016/0006-8993(78)91122-8. [DOI] [PubMed] [Google Scholar]
- Bayer E. A., Skutelsky E., Wynne D., Wilchek M. Preparation of ferritin-avidin conjugates by reductive alkylation for use in electron microscopic cytochemistry. J Histochem Cytochem. 1976 Aug;24(8):933–939. doi: 10.1177/24.8.182877. [DOI] [PubMed] [Google Scholar]
- Bloedel J. R., Gage P. W., Llinás R., Quastel D. M. Transmission across the squid giant synapse in the presence of tetrodotoxin. J Physiol. 1967 Jan;188(2):52P–53P. [PubMed] [Google Scholar]
- Bloedel J., Gage P. W., Llinás R., Quastel D. M. Transmitter release at the squid giant synapse in the presence of tetrodotoxin. Nature. 1966 Oct 1;212(5057):49–50. doi: 10.1038/212049a0. [DOI] [PubMed] [Google Scholar]
- Brunngraber E. G., Dekirmenjian H., Brown B. D. The distribution of protein-bound N-acetylneuraminic acid in subcellular fractions of rat brain. Biochem J. 1967 Apr;103(1):73–78. doi: 10.1042/bj1030073. [DOI] [PMC free article] [PubMed] [Google Scholar]
- Dekirmenjian H., Brunngraber E. G. Distribution of protein-bound N-acetylneuraminic acid in subcellular particulate fractions prepared from rat whole brain. Biochim Biophys Acta. 1969 Feb 18;177(1):1–10. doi: 10.1016/0304-4165(69)90057-9. [DOI] [PubMed] [Google Scholar]
- Dreyfus P. Identification de l'acétate comme précurseur du radical acétyl de l'acétylcholine des jonctions neuro-musculaires du Rat. C R Acad Sci Hebd Seances Acad Sci D. 1975 Apr 28;280(16):1893–1894. [PubMed] [Google Scholar]
- Dunant Y., Gautron J., Israël M., Lesbats B., Manaranche R. Evolution de la décharge de l'organe électrique de la Torpille et variations simultanées de l'acétylcholine au cours de la stimulation. J Neurochem. 1974 Oct;23(4):635–643. doi: 10.1111/j.1471-4159.1974.tb04386.x. [DOI] [PubMed] [Google Scholar]
- Dunant Y., Gautron J., Israël M., Lesbats B., Manaranche R. Les compartiments d'acetylcholine de l'organe electrique de la torpille et leurs modifications par la stimulation. J Neurochem. 1972 Aug;19(8):1987–2002. doi: 10.1111/j.1471-4159.1972.tb01488.x. [DOI] [PubMed] [Google Scholar]
- Eisenstadt M. L., Schwartz J. H. Metabolism of acetylcholine in the nervous system of Aplysia californica. III. Studies of an indentified cholinergic neuron. J Gen Physiol. 1975 Mar;65(3):293–213. doi: 10.1085/jgp.65.3.293. [DOI] [PMC free article] [PubMed] [Google Scholar]
- Fiore L., Meunier J. M. A network of synaptic relations in the buccal ganglia of Aplysia. Brain Res. 1975 Jul 11;92(2):336–340. doi: 10.1016/0006-8993(75)90280-2. [DOI] [PubMed] [Google Scholar]
- Fishman P. H., Brady R. O. Biosynthesis and function of gangliosides. Science. 1976 Nov 26;194(4268):906–915. doi: 10.1126/science.185697. [DOI] [PubMed] [Google Scholar]
- Fonnum F. Isolation of choline esters from aqueous solutions by extraction with sodium tetraphenylboron in organic solvents. Biochem J. 1969 Jun;113(2):291–298. doi: 10.1042/bj1130291. [DOI] [PMC free article] [PubMed] [Google Scholar]
- Fredman S. M., Jahan-Parwar B. Synaptic connections in the cerebral ganglion of Aplysia. Brain Res. 1975 Dec 12;100(1):209–214. doi: 10.1016/0006-8993(75)90262-0. [DOI] [PubMed] [Google Scholar]
- Gardner D. Bilateral symmetry and interneuronal organization in the buccal ganglia of Aplysia. Science. 1971 Aug 6;173(3996):550–553. doi: 10.1126/science.173.3996.550. [DOI] [PubMed] [Google Scholar]
- Gardner D. Interconnections of identified multiaction interneurons in buccal ganglia of Aplysia. J Neurophysiol. 1977 Mar;40(2):349–361. doi: 10.1152/jn.1977.40.2.349. [DOI] [PubMed] [Google Scholar]
- Gardner D., Kandel E. R. Physiological and kinetic properties of cholinergic receptors activated by multiaction interneurons in buccal ganglia of Aplysia. J Neurophysiol. 1977 Mar;40(2):333–348. doi: 10.1152/jn.1977.40.2.333. [DOI] [PubMed] [Google Scholar]
- Gardner D. Voltage-clamp analysis of a self-inhibitory synaptic potential in the buccal ganglia of Aplysia. J Physiol. 1977 Jan;264(3):893–920. doi: 10.1113/jphysiol.1977.sp011701. [DOI] [PMC free article] [PubMed] [Google Scholar]
- Geduldig D., Junge D. Sodium and calcium components of action potentials in the Aplysia giant neurone. J Physiol. 1968 Dec;199(2):347–365. doi: 10.1113/jphysiol.1968.sp008657. [DOI] [PMC free article] [PubMed] [Google Scholar]
- Gomez-Puyou A., De Gomez-Puyou M. T., Becker G., Lehninger A. L. An insoluble Ca 2+ -binding factor from rat liver mitochondria. Biochem Biophys Res Commun. 1972 May 26;47(4):814–819. doi: 10.1016/0006-291x(72)90565-7. [DOI] [PubMed] [Google Scholar]
- Hinzen D. H., Davies M. A. Synaptic connexions and related postsynaptic pharmacology studied in the cerebral ganglion of Aplysia. Brain Res. 1978 Apr 7;144(1):49–62. doi: 10.1016/0006-8993(78)90434-1. [DOI] [PubMed] [Google Scholar]
- Hinzen D. H., Tauc L. Membrane properties of Aplysia neurones intracellularly injected with phospholipases A and C. J Physiol. 1977 Jun;268(1):21–34. doi: 10.1113/jphysiol.1977.sp011844. [DOI] [PMC free article] [PubMed] [Google Scholar]
- Israel M., Gautron J., Lesbats B. Isolement des vésicules synaptiques de l'organe électrique de la Torpille et localisation de l'acétylcholine à leur niveau. C R Acad Sci Hebd Seances Acad Sci D. 1968 Jan 15;266(3):273–275. [PubMed] [Google Scholar]
- Israël M., Gautron J., Lesbats B. Fractionnement de l'organe electrique de la torpille: localisation subcellulaire de l'acetylcholine. J Neurochem. 1970 Oct;17(10):1441–1450. doi: 10.1111/j.1471-4159.1970.tb00511.x. [DOI] [PubMed] [Google Scholar]
- Israël M., Tucek S. Utilization of acetate and pyruvate for the synthesis of 'total', 'bound' and 'free' acetylcholine in the electric organ of Torpedo. J Neurochem. 1974 Apr;22(4):487–491. doi: 10.1111/j.1471-4159.1974.tb06883.x. [DOI] [PubMed] [Google Scholar]
- KRNJEVIC K., MILEDI R. Presynaptic failure of neuromuscular propagation in rats. J Physiol. 1959 Dec;149:1–22. doi: 10.1113/jphysiol.1959.sp006321. [DOI] [PMC free article] [PubMed] [Google Scholar]
- Kahane I., Polliack A., Rachmilewitz E. A., Bayer E. A., Skutelsky E. Distribution of sialic acids on the red blood cell membrane in beta thalassaemia. Nature. 1978 Feb 16;271(5646):674–675. doi: 10.1038/271674a0. [DOI] [PubMed] [Google Scholar]
- Katz B., Miledi R. A study of synaptic transmission in the absence of nerve impulses. J Physiol. 1967 Sep;192(2):407–436. doi: 10.1113/jphysiol.1967.sp008307. [DOI] [PMC free article] [PubMed] [Google Scholar]
- Katz B., Miledi R. Tetrodotoxin and neuromuscular transmission. Proc R Soc Lond B Biol Sci. 1967 Jan 31;167(1006):8–22. doi: 10.1098/rspb.1967.0010. [DOI] [PubMed] [Google Scholar]
- Katz B., Miledi R. The release of acetylcholine from nerve endings by graded electric pulses. Proc R Soc Lond B Biol Sci. 1967 Jan 31;167(1006):23–38. doi: 10.1098/rspb.1967.0011. [DOI] [PubMed] [Google Scholar]
- Katz B., Miledi R. Transmitter leakage from motor nerve endings. Proc R Soc Lond B Biol Sci. 1977 Feb 11;196(1122):59–72. doi: 10.1098/rspb.1977.0029. [DOI] [PubMed] [Google Scholar]
- Koike H., Dandel E. R., Schwartz J. H. Synaptic release of radioactivity after intrasomatic injection of choline-3H into an identified cholinergic interneuron in abdominal ganglion of Aplysia californica. J Neurophysiol. 1974 Jul;37(4):815–827. doi: 10.1152/jn.1974.37.4.815. [DOI] [PubMed] [Google Scholar]
- Kusano K. Influence of ionic environment on the relationship between pre- and postsynaptic potentials. J Neurobiol. 1970;1(4):435–437. doi: 10.1002/neu.480010407. [DOI] [PubMed] [Google Scholar]
- Lapetina E. G., Soto E. F., De Robertis E. Lipids and proteolipids in isolated subcellular membranes of rat brain cortex. J Neurochem. 1968 Jun;15(6):437–445. doi: 10.1111/j.1471-4159.1968.tb08939.x. [DOI] [PubMed] [Google Scholar]
- Lapetina E. G., Soto E. F., de Robertis E. Gangliosides and acetylcholinesterase in isolated membranes of the rat-brain cortex. Biochim Biophys Acta. 1967 Feb 1;135(1):33–43. doi: 10.1016/0005-2736(67)90005-3. [DOI] [PubMed] [Google Scholar]
- Luft J. H. Ruthenium red and violet. I. Chemistry, purification, methods of use for electron microscopy and mechanism of action. Anat Rec. 1971 Nov;171(3):347–368. doi: 10.1002/ar.1091710302. [DOI] [PubMed] [Google Scholar]
- Marchbanks R. M., Israël M. The heterogeneity of bound acetylcholine and synaptic vesicles. Biochem J. 1972 Oct;129(5):1049–1061. doi: 10.1042/bj1291049. [DOI] [PMC free article] [PubMed] [Google Scholar]
- Martin A. R., Ringham G. L. Synaptic transfer at a vertebrate central nervous system synapse. J Physiol. 1975 Oct;251(2):409–426. doi: 10.1113/jphysiol.1975.sp011101. [DOI] [PMC free article] [PubMed] [Google Scholar]
- Martin A. R., Ringham G. Proceedings: Synaptic transfer curves at a vertebrate central synapse. J Physiol. 1974 Oct;242(2):84P–86P. [PubMed] [Google Scholar]
- Martin R., Miledi R. A structural study of the squid synapse after intraaxonal injection of calcium. Proc R Soc Lond B Biol Sci. 1978 Jun 5;201(1145):317–333. doi: 10.1098/rspb.1978.0049. [DOI] [PubMed] [Google Scholar]
- Rahamimoff R., Alnaes E. Inhibitory action of Ruthenium red on neuromuscular transmission. Proc Natl Acad Sci U S A. 1973 Dec;70(12):3613–3616. doi: 10.1073/pnas.70.12.3613. [DOI] [PMC free article] [PubMed] [Google Scholar]
- Rose B., Loewenstein W. R. Permeability of cell junction depends on local cytoplasmic calcium activity. Nature. 1975 Mar 20;254(5497):250–252. doi: 10.1038/254250a0. [DOI] [PubMed] [Google Scholar]
- Roth S. A molecular model for cell interactions. Q Rev Biol. 1973 Dec;48(4):541–563. doi: 10.1086/407816. [DOI] [PubMed] [Google Scholar]
- Rothstein A., Cabantchik Z. I., Knauf P. Mechanism of anion transport in red blood cells: role of membrane proteins. Fed Proc. 1976 Jan;35(1):3–10. [PubMed] [Google Scholar]
- Shimahara T., Tauc L. Multiple interneuronal afferents to the giant cells in Aplysia. J Physiol. 1975 May;247(2):299–319. doi: 10.1113/jphysiol.1975.sp010933. [DOI] [PMC free article] [PubMed] [Google Scholar]
- Singer M., Krishnan N., Fyfe D. A. Penetration of ruthenium red into peripheral nerve fibers. Anat Rec. 1972 Aug;173(4):375–389. doi: 10.1002/ar.1091730401. [DOI] [PubMed] [Google Scholar]
- Skutelsky E., Danon D., Wilchek M., Bayer E. A. Localization of sialyl residues on cell surfaces by affinity cytochemistry. J Ultrastruct Res. 1977 Dec;61(3):325–335. doi: 10.1016/s0022-5320(77)80057-9. [DOI] [PubMed] [Google Scholar]
- Stinnakre J., Tauc L. Calcium influx in active Aplysia neurones detected by injected aequorin. Nat New Biol. 1973 Mar 28;242(117):113–115. doi: 10.1038/newbio242113b0. [DOI] [PubMed] [Google Scholar]
- Swanson P. D., Anderson L., Stahl W. L. Uptake of calcium ions by synaptosomes from rat brain. Biochim Biophys Acta. 1974 Jul 31;356(2):174–183. doi: 10.1016/0005-2736(74)90281-8. [DOI] [PubMed] [Google Scholar]
- TAUC L., GERSCHENFELD H. M. Cholinergic transmission mechanisms for both excitation and inhibition in molluscan central synapses. Nature. 1961 Oct 28;192:366–367. doi: 10.1038/192366a0. [DOI] [PubMed] [Google Scholar]
- Tani E., Ametani T. Substructure of microtubules in brain nerve cells as revealed by ruthenium red. J Cell Biol. 1970 Jul;46(1):159–165. doi: 10.1083/jcb.46.1.159. [DOI] [PMC free article] [PubMed] [Google Scholar]
- Tapia R., Meza-Ruíz G. Inhibition by ruthenium red of the calcium-dependent release of [3H]GABA in synaptosomal fractions. Brain Res. 1977 Apr 22;126(1):160–166. doi: 10.1016/0006-8993(77)90223-2. [DOI] [PubMed] [Google Scholar]
- Tauc L., Hinzen D. H. Neuraminidase: its effect on synaptic transmission. Brain Res. 1974 Nov 15;80(2):340–344. doi: 10.1016/0006-8993(74)90697-0. [DOI] [PubMed] [Google Scholar]
- Tauc L., Hoffmann A., Tsuji S., Hinzen D. H., Faille L. Transmission abolished on a cholinergic synapse after injection of acetylcholinesterase into the presynaptic neurone. Nature. 1974 Aug 9;250(5466):496–498. doi: 10.1038/250496a0. [DOI] [PubMed] [Google Scholar]
- WHITTAKER V. P., MICHAELSON I. A., KIRKLAND R. J. The separation of synaptic vesicles from disrupted nervending particles. Biochem Pharmacol. 1963 Mar;12:300–302. doi: 10.1016/0006-2952(63)90156-4. [DOI] [PubMed] [Google Scholar]
- Whittaker V. P., Essman W. B., Dowe G. H. The isolation of pure cholinergic synaptic vesicles from the electric organs of elasmobranch fish of the family Torpedinidae. Biochem J. 1972 Jul;128(4):833–845. doi: 10.1042/bj1280833. [DOI] [PMC free article] [PubMed] [Google Scholar]
- Zanetta J. P., Reeber A., Ghandour M. S., Vincendon G., Gombos G. Glycoproteins of intrasynaptosomal mitochondria. Brain Res. 1977 Apr 15;125(2):386–389. doi: 10.1016/0006-8993(77)90635-7. [DOI] [PubMed] [Google Scholar]
- van Heyningen S. Cholera toxin. Biol Rev Camb Philos Soc. 1977 Nov;52(4):509–509. doi: 10.1111/j.1469-185x.1977.tb00858.x. [DOI] [PubMed] [Google Scholar]