Abstract
We have generalized the double nucleation mechanism of Ferrone et al. (Ferrone, F. A., J. Hofrichter, H. Sunshine, and W. A. Eaton. 1980. Biophys. J. 32:361-377; Ferrone, F. A., J. Hofrichter, and W. A. Eaton. 1985. J. Mol. Biol. 183:611-631) to describe the spatial dependence of the radial growth of polymer domains of sickle hemoglobin. Although this extended model requires the consideration of effects such as monomer diffusion, which are irrelevant to a spatially uniform description, no new adjustable parameters are required because diffusion constants are known independently. We find that monomer diffusion into the growing domain can keep the net unpolymerized monomer concentration approximately constant, and in that limit we present an analytic solution of the model. The model shows the features reported by Basak, S., F. A. Ferrone, and J. T. Wang (1988. Biophys J. 54:829-843) and provides a new means of determining the rate of polymer growth. When spatially integrated, the model exhibits the exponential growth seen in previous studies, although molecular parameters derived from analysis of the kinetics assuming uniformity must be modified in some cases to account for the spatially nonuniform growth. The model developed here can be easily adapted to any spatially dependent polymerization process.
Full text
PDF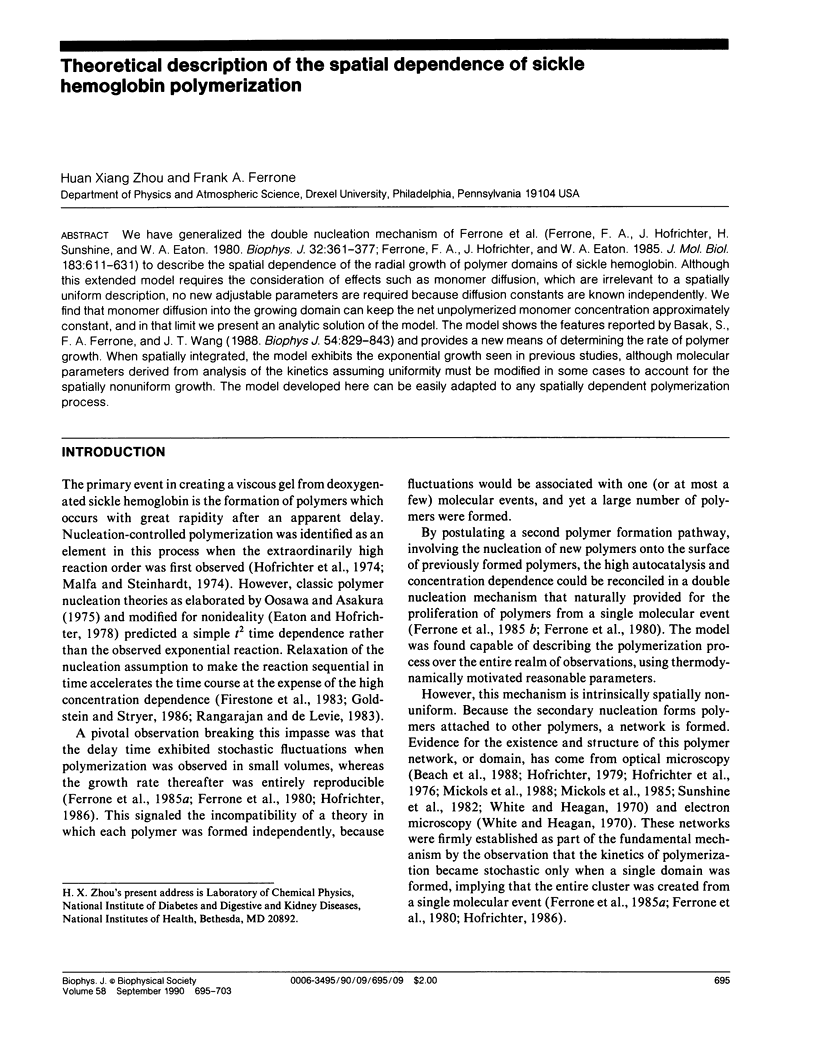
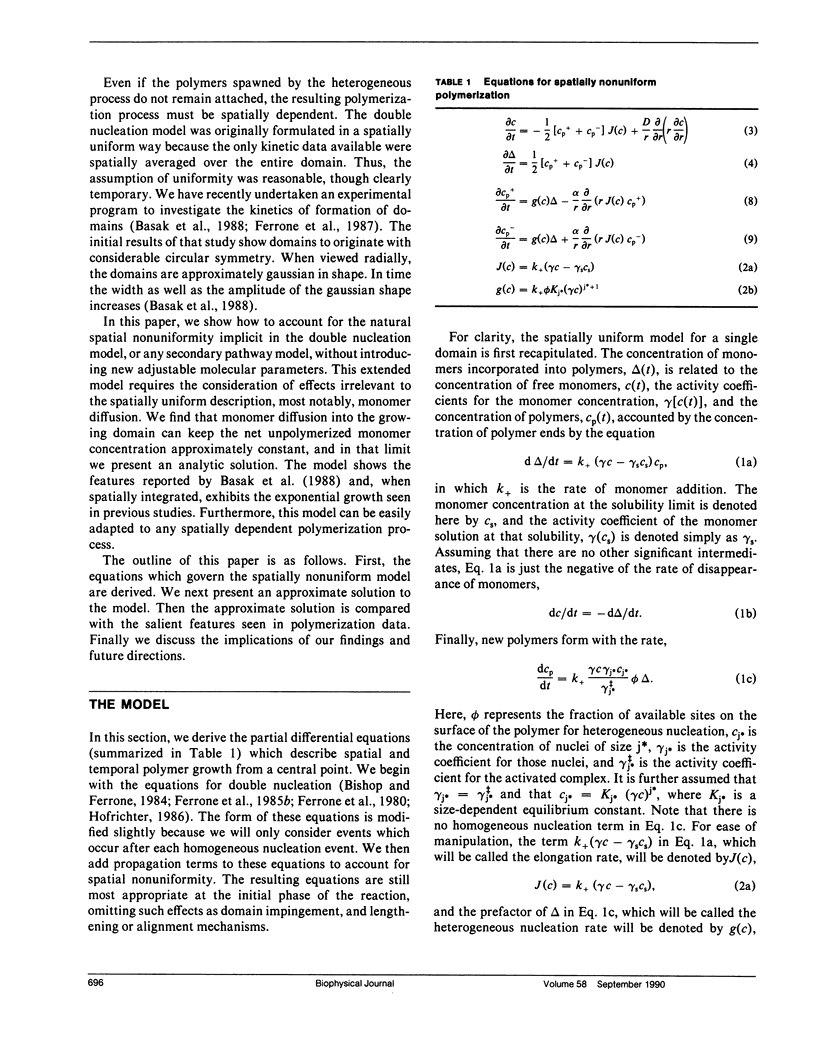
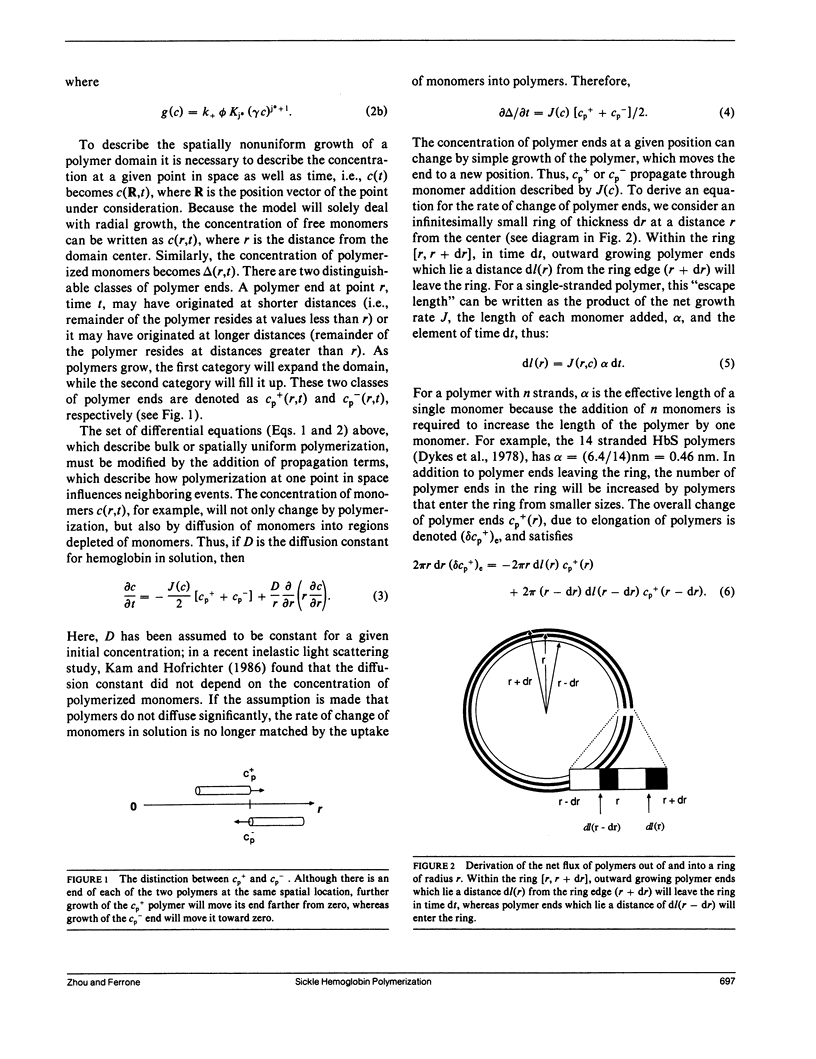
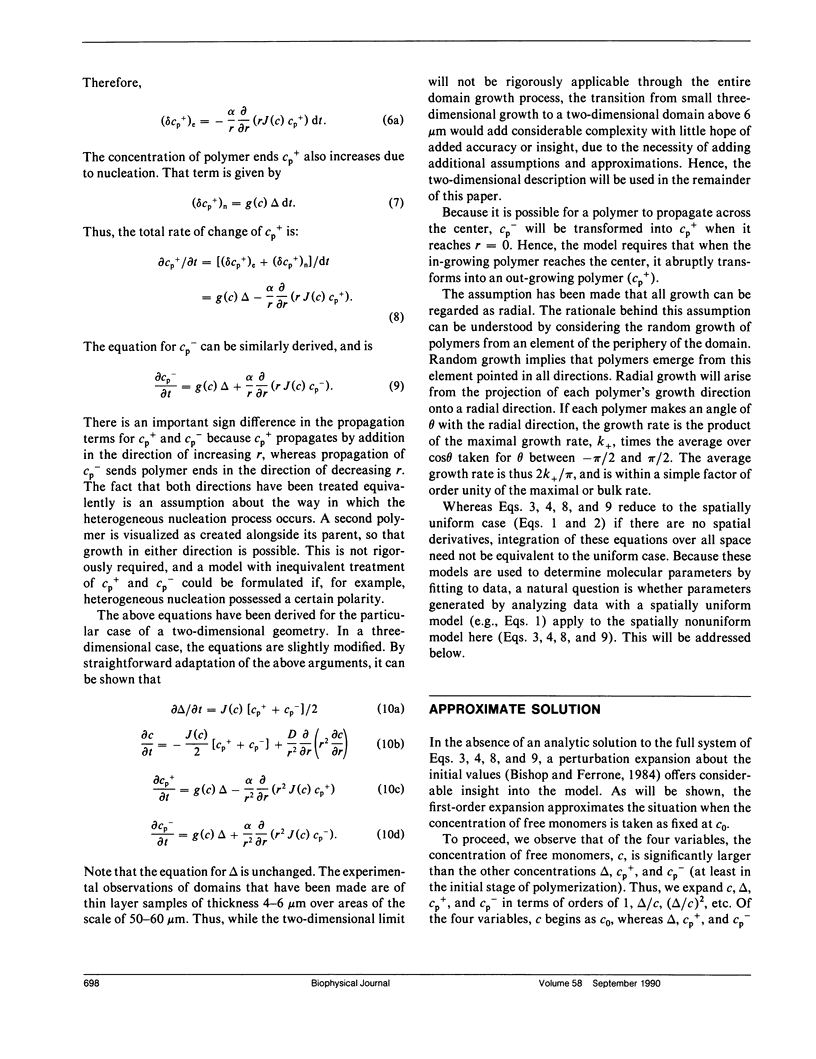
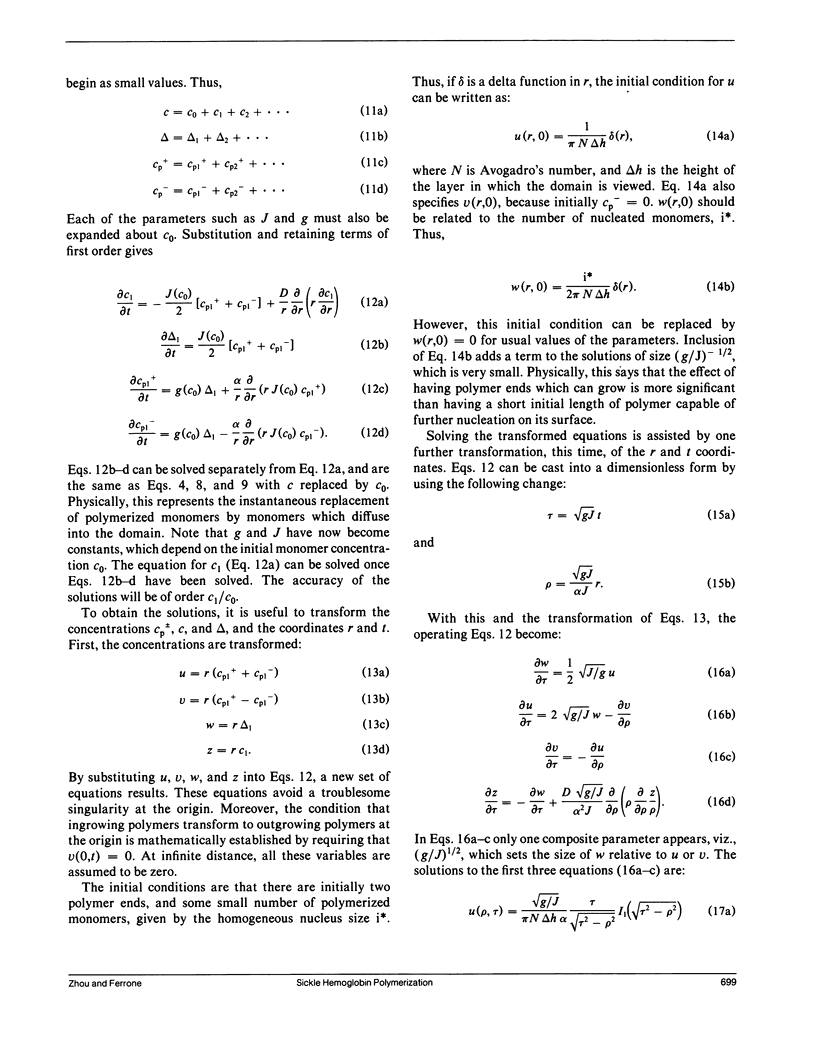
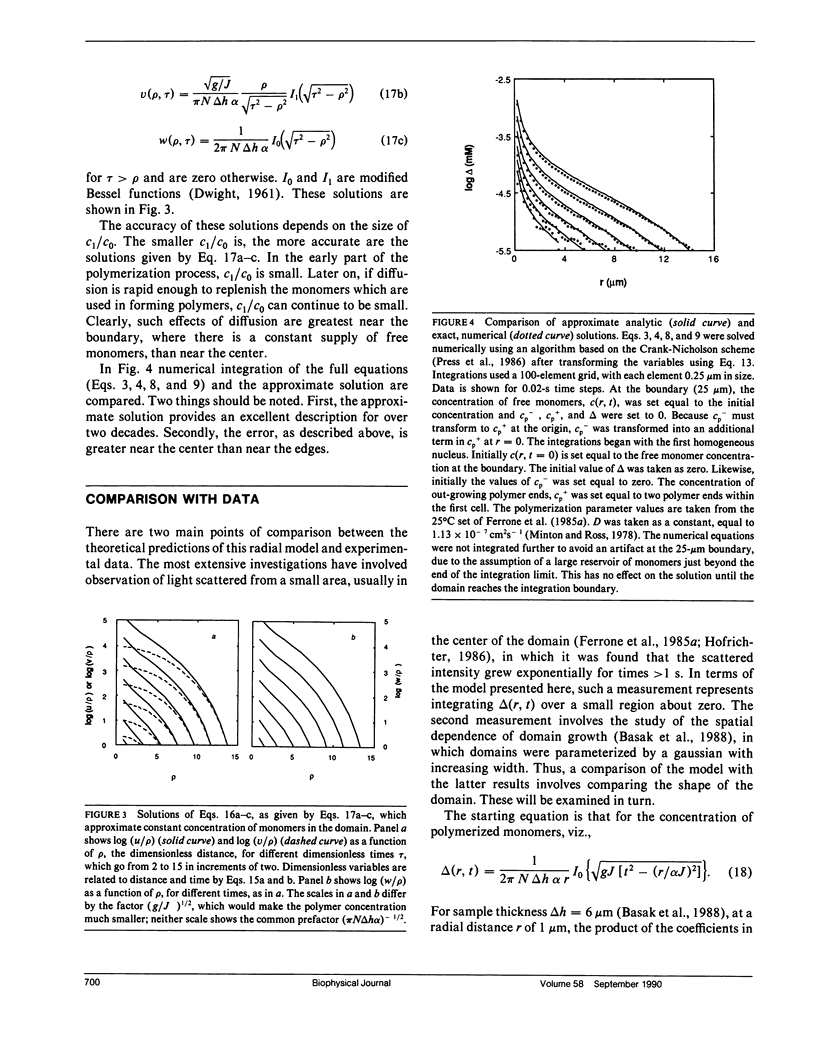
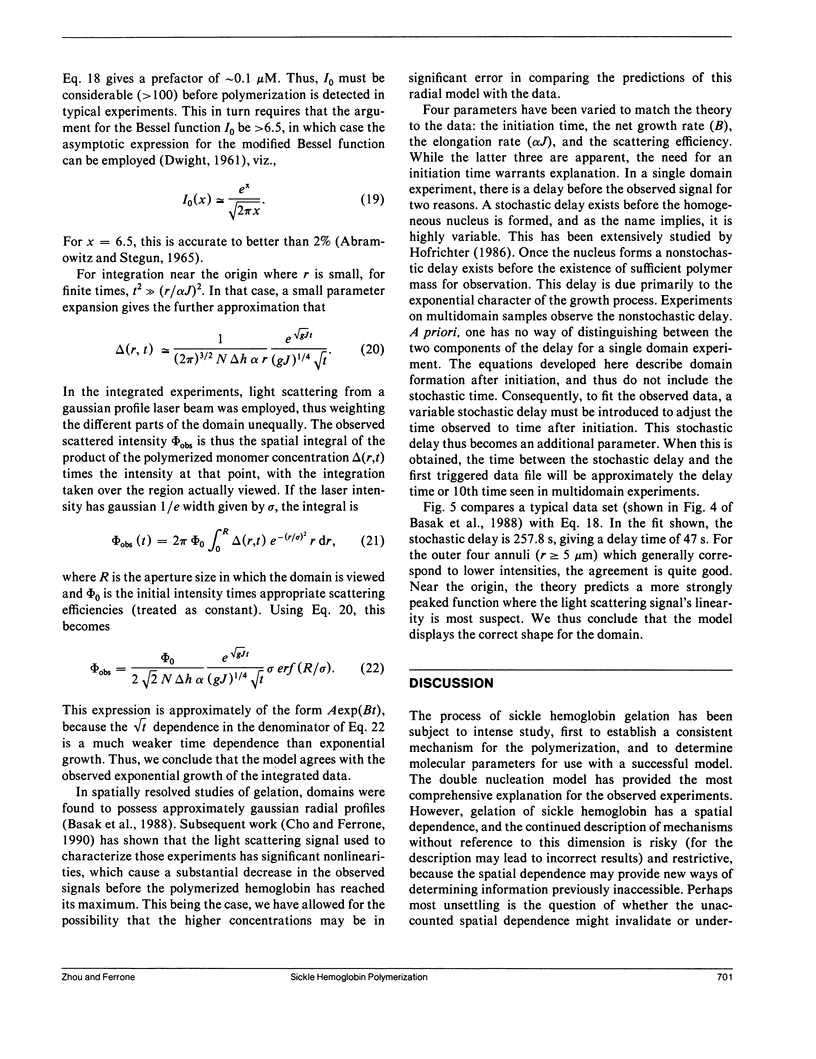
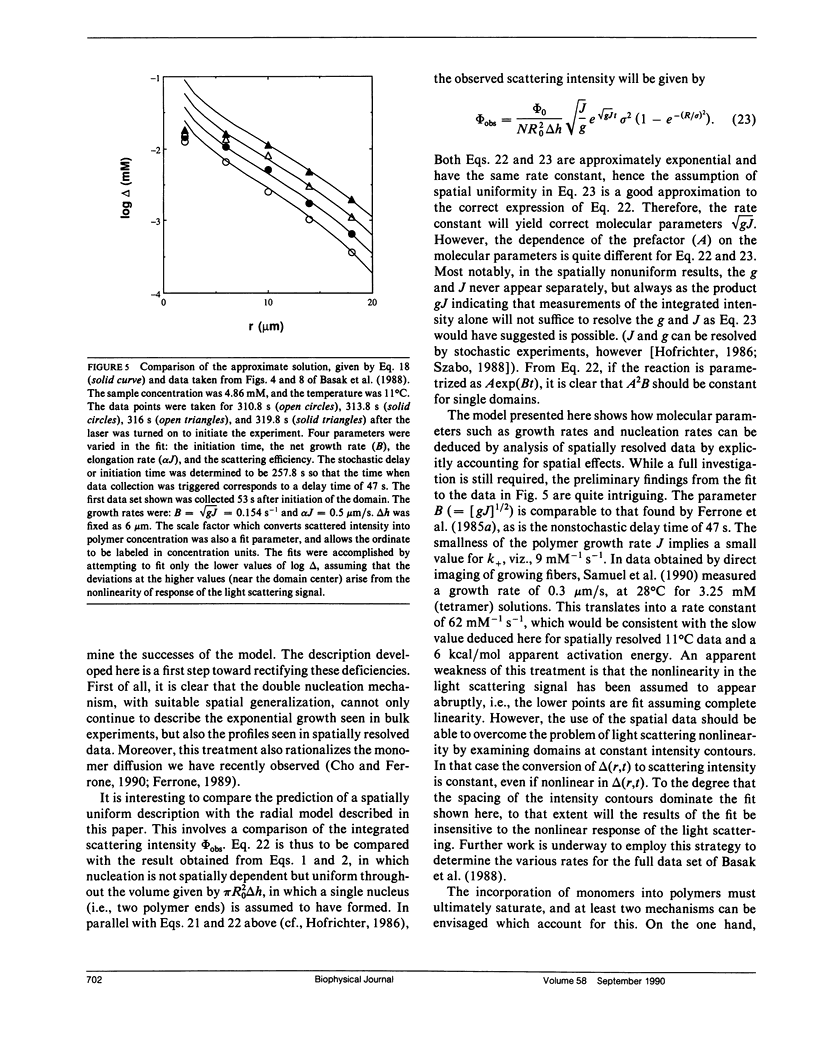
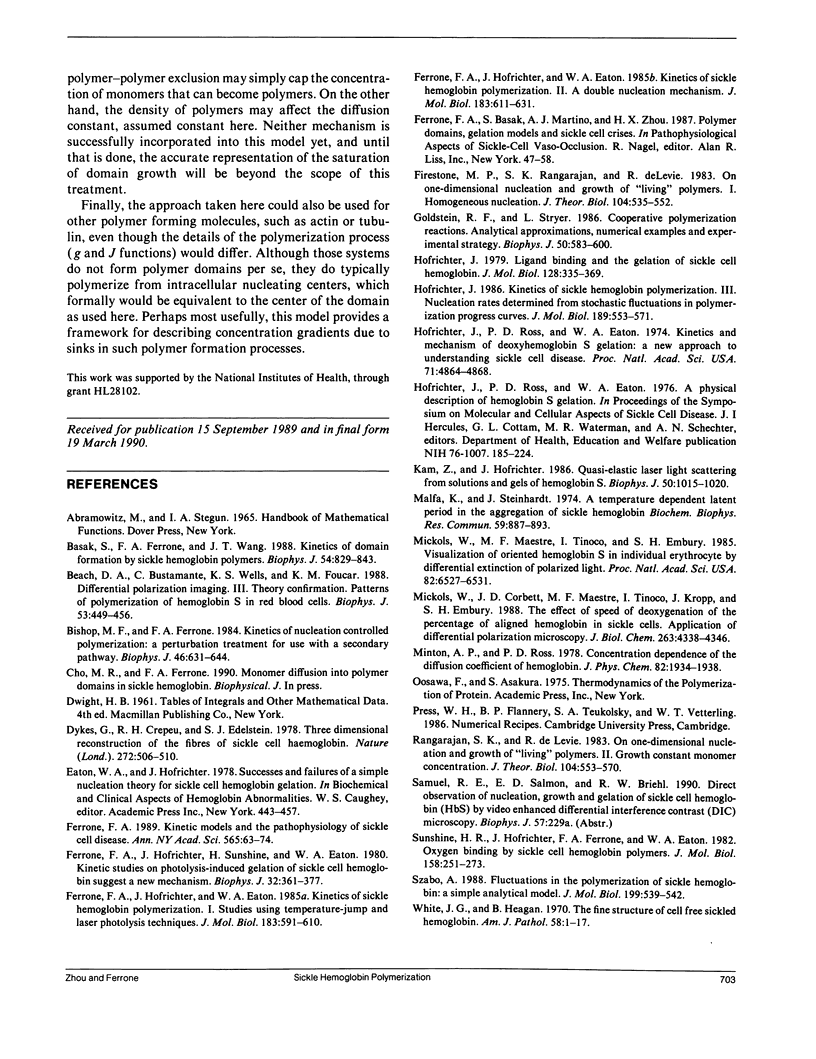
Selected References
These references are in PubMed. This may not be the complete list of references from this article.
- Basak S., Ferrone F. A., Wang J. T. Kinetics of domain formation by sickle hemoglobin polymers. Biophys J. 1988 Nov;54(5):829–843. doi: 10.1016/S0006-3495(88)83020-0. [DOI] [PMC free article] [PubMed] [Google Scholar]
- Beach D. A., Bustamante C., Wells K. S., Foucar K. M. Differential polarization imaging. III. Theory confirmation. Patterns of polymerization of hemoglobin S in red blood sickle cells. Biophys J. 1988 Mar;53(3):449–456. doi: 10.1016/S0006-3495(88)83121-7. [DOI] [PMC free article] [PubMed] [Google Scholar]
- Bishop M. F., Ferrone F. A. Kinetics of nucleation-controlled polymerization. A perturbation treatment for use with a secondary pathway. Biophys J. 1984 Nov;46(5):631–644. doi: 10.1016/S0006-3495(84)84062-X. [DOI] [PMC free article] [PubMed] [Google Scholar]
- Dykes G., Crepeau R. H., Edelstein S. J. Three-dimensional reconstruction of the fibres of sickle cell haemoglobin. Nature. 1978 Apr 6;272(5653):506–510. doi: 10.1038/272506a0. [DOI] [PubMed] [Google Scholar]
- Ferrone F. A., Basak S., Martino A. J., Zhou H. X. Polymer domains, gelation models and sickle cell crises. Prog Clin Biol Res. 1987;240:47–58. [PubMed] [Google Scholar]
- Ferrone F. A., Hofrichter J., Eaton W. A. Kinetics of sickle hemoglobin polymerization. I. Studies using temperature-jump and laser photolysis techniques. J Mol Biol. 1985 Jun 25;183(4):591–610. doi: 10.1016/0022-2836(85)90174-3. [DOI] [PubMed] [Google Scholar]
- Ferrone F. A., Hofrichter J., Eaton W. A. Kinetics of sickle hemoglobin polymerization. II. A double nucleation mechanism. J Mol Biol. 1985 Jun 25;183(4):611–631. doi: 10.1016/0022-2836(85)90175-5. [DOI] [PubMed] [Google Scholar]
- Ferrone F. A., Hofrichter J., Sunshine H. R., Eaton W. A. Kinetic studies on photolysis-induced gelation of sickle cell hemoglobin suggest a new mechanism. Biophys J. 1980 Oct;32(1):361–380. doi: 10.1016/S0006-3495(80)84962-9. [DOI] [PMC free article] [PubMed] [Google Scholar]
- Ferrone F. A. Kinetic models and the pathophysiology of sickle cell disease. Ann N Y Acad Sci. 1989;565:63–74. doi: 10.1111/j.1749-6632.1989.tb24151.x. [DOI] [PubMed] [Google Scholar]
- Firestone M. P., de Levie R., Rangarajan S. K. On one-dimensional nucleation and growth of "living" polymers I. Homogeneous nucleation. J Theor Biol. 1983 Oct 21;104(4):535–552. doi: 10.1016/0022-5193(83)90244-8. [DOI] [PubMed] [Google Scholar]
- Goldstein R. F., Stryer L. Cooperative polymerization reactions. Analytical approximations, numerical examples, and experimental strategy. Biophys J. 1986 Oct;50(4):583–599. doi: 10.1016/S0006-3495(86)83498-1. [DOI] [PMC free article] [PubMed] [Google Scholar]
- Hofrichter J. Kinetics of sickle hemoglobin polymerization. III. Nucleation rates determined from stochastic fluctuations in polymerization progress curves. J Mol Biol. 1986 Jun 5;189(3):553–571. doi: 10.1016/0022-2836(86)90324-4. [DOI] [PubMed] [Google Scholar]
- Hofrichter J. Ligand binding and the gelation of sickle cell hemoglobin. J Mol Biol. 1979 Mar 5;128(3):335–369. doi: 10.1016/0022-2836(79)90092-5. [DOI] [PubMed] [Google Scholar]
- Hofrichter J., Ross P. D., Eaton W. A. Kinetics and mechanism of deoxyhemoglobin S gelation: a new approach to understanding sickle cell disease. Proc Natl Acad Sci U S A. 1974 Dec;71(12):4864–4868. doi: 10.1073/pnas.71.12.4864. [DOI] [PMC free article] [PubMed] [Google Scholar]
- Kam Z., Hofrichter J. Quasi-elastic laser light scattering from solutions and gels of hemoglobin S. Biophys J. 1986 Nov;50(5):1015–1020. doi: 10.1016/S0006-3495(86)83544-5. [DOI] [PMC free article] [PubMed] [Google Scholar]
- Malfa R., Steinhardt J. A temperature-dependent latent-period in the aggregation of sickle-cell deoxyhemoglobin. Biochem Biophys Res Commun. 1974 Aug 5;59(3):887–893. doi: 10.1016/s0006-291x(74)80062-8. [DOI] [PubMed] [Google Scholar]
- Mickols W. E., Corbett J. D., Maestre M. F., Tinoco I., Jr, Kropp J., Embury S. H. The effect of speed of deoxygenation on the percentage of aligned hemoglobin in sickle cells. Application of differential polarization microscopy. J Biol Chem. 1988 Mar 25;263(9):4338–4346. [PubMed] [Google Scholar]
- Mickols W., Maestre M. F., Tinoco I., Jr, Embury S. H. Visualization of oriented hemoglobin S in individual erythrocytes by differential extinction of polarized light. Proc Natl Acad Sci U S A. 1985 Oct;82(19):6527–6531. doi: 10.1073/pnas.82.19.6527. [DOI] [PMC free article] [PubMed] [Google Scholar]
- Rangarajan S. K., de Levie R. On one-dimensional nucleation and growth of "living" polymers. II. Growth at constant monomer concentration. J Theor Biol. 1983 Oct 21;104(4):553–570. doi: 10.1016/0022-5193(83)90245-x. [DOI] [PubMed] [Google Scholar]
- Sunshine H. R., Hofrichter J., Ferrone F. A., Eaton W. A. Oxygen binding by sickle cell hemoglobin polymers. J Mol Biol. 1982 Jun 25;158(2):251–273. doi: 10.1016/0022-2836(82)90432-6. [DOI] [PubMed] [Google Scholar]
- Szabo A. Fluctuations in the polymerization of sickle hemoglobin. A simple analytic model. J Mol Biol. 1988 Feb 5;199(3):539–542. doi: 10.1016/0022-2836(88)90624-9. [DOI] [PubMed] [Google Scholar]
- White J. G., Heagan B. The fine structure of cell-free sickled hemoglobin. Am J Pathol. 1970 Jan;58(1):1–17. [PMC free article] [PubMed] [Google Scholar]