Abstract
The "shell" model for Ca2(+)-inactivation of Ca2+ channels is based on the accumulation of Ca2+ in a macroscopic shell beneath the plasma membrane. The shell is filled by Ca2+ entering through open channels, with the elevated Ca2+ concentration inactivating both open and closed channels at a rate determined by how fast the shell is filled. In cells with low channel density, the high concentration Ca2+ "shell" degenerates into a collection of nonoverlapping "domains" localized near open channels. These domains form rapidly when channels open and disappear rapidly when channels close. We use this idea to develop a "domain" model for Ca2(+)-inactivation of Ca2+ channels. In this model the kinetics of formation of an inactivated state resulting from Ca2+ binding to open channels determines the inactivation rate, a mechanism identical with that which explains single-channel recordings on rabbit-mesenteric artery Ca2+ channels (Huang Y., J. M. Quayle, J. F. Worley, N. B. Standen, and M. T. Nelson. 1989. Biophys. J. 56:1023-1028). We show that the model correctly predicts five important features of the whole-cell Ca2(+)-inactivation for mouse pancreatic beta-cells (Plants, T. D. 1988. J. Physiol. 404:731-747) and that Ca2(+)-inactivation has only minor effects on the bursting electrical activity of these cells.
Full text
PDF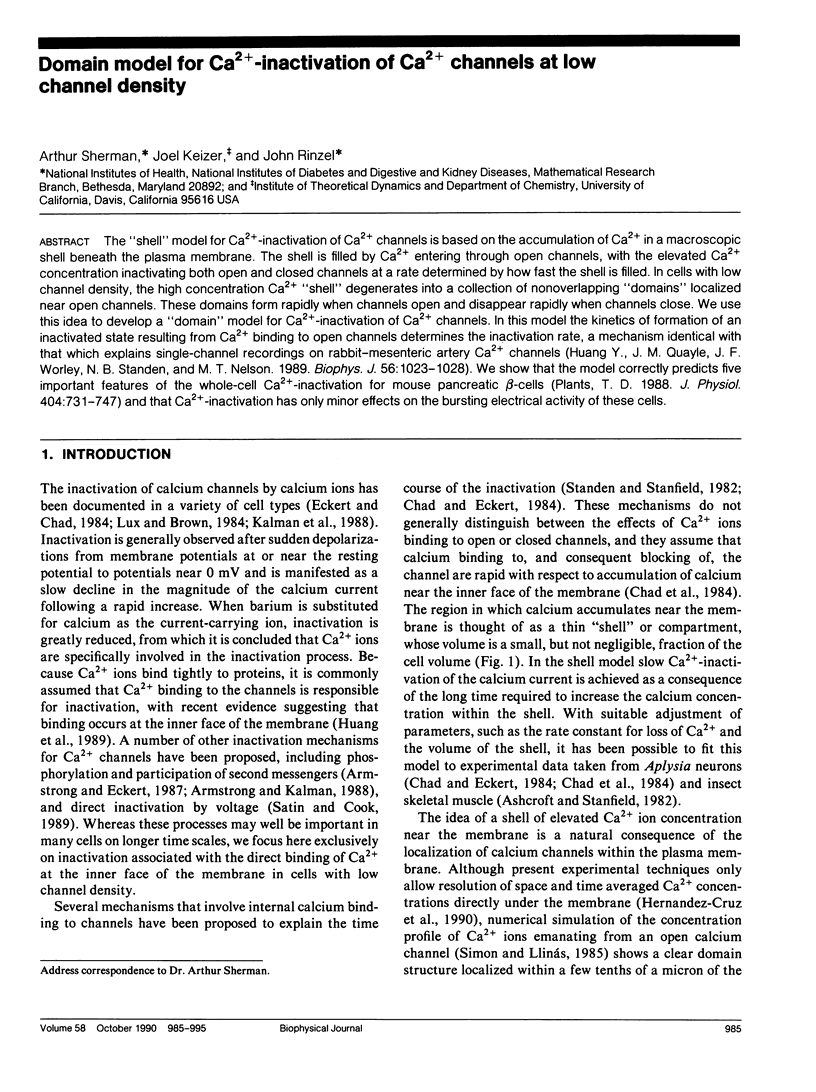
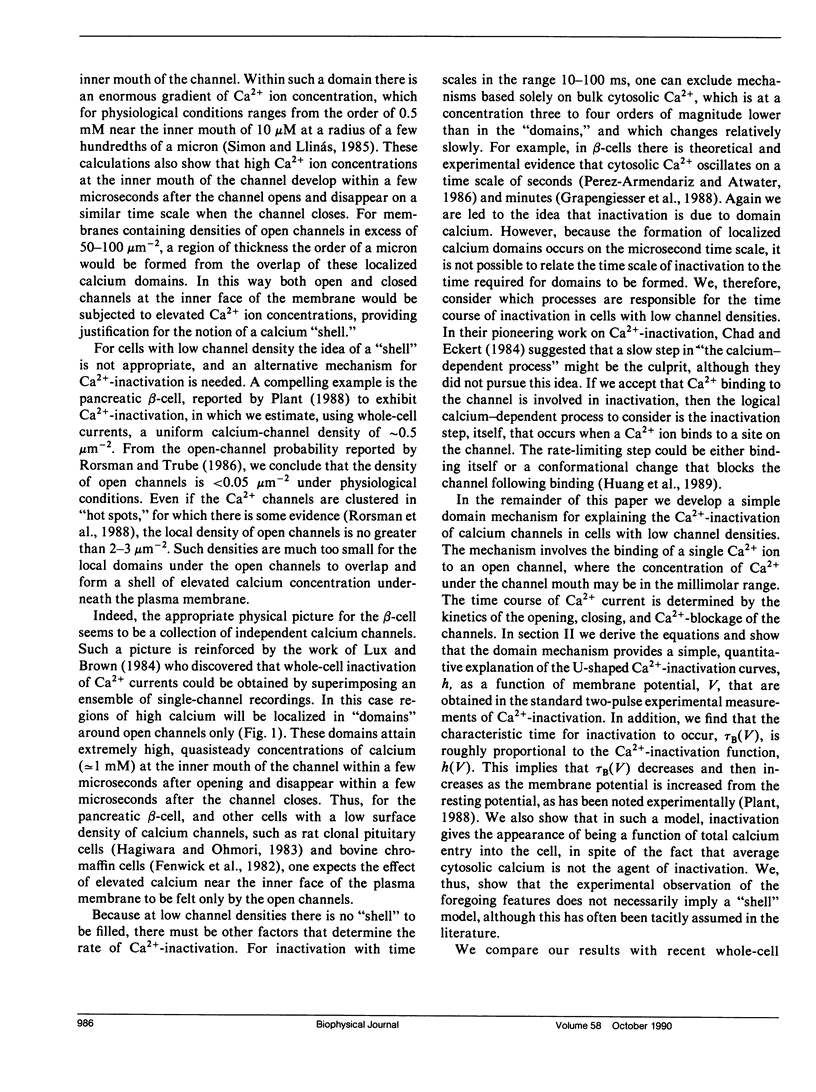
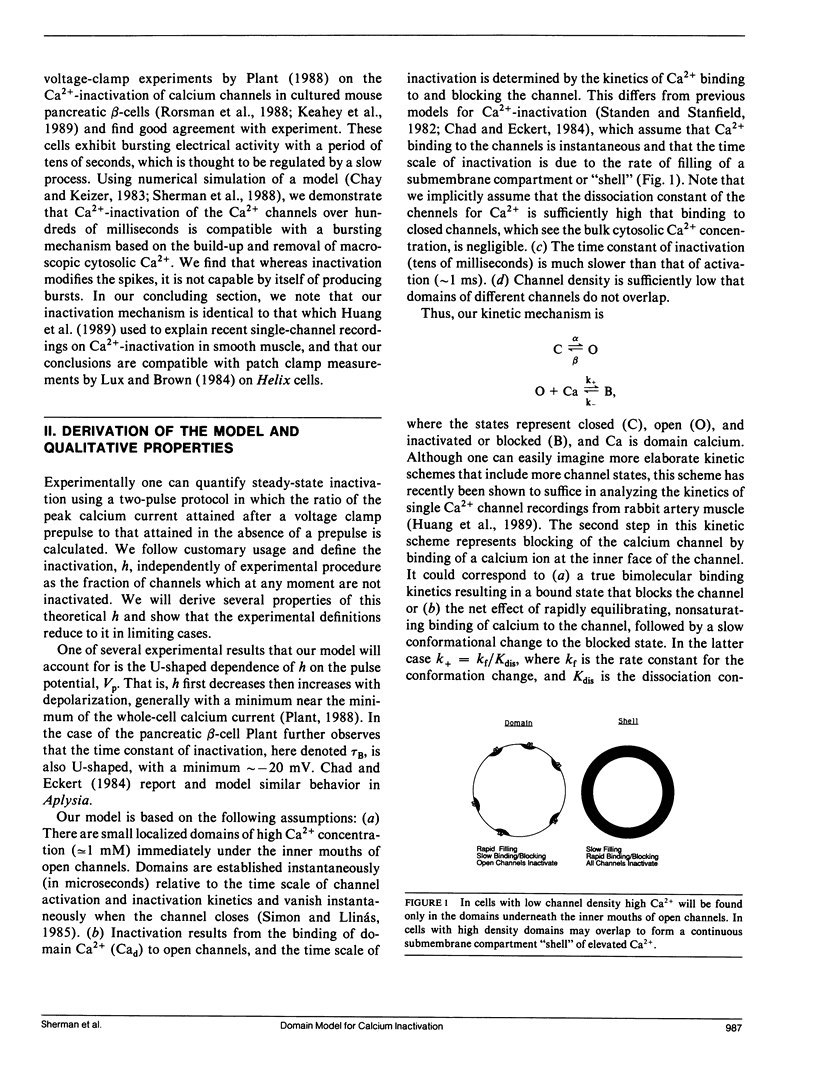
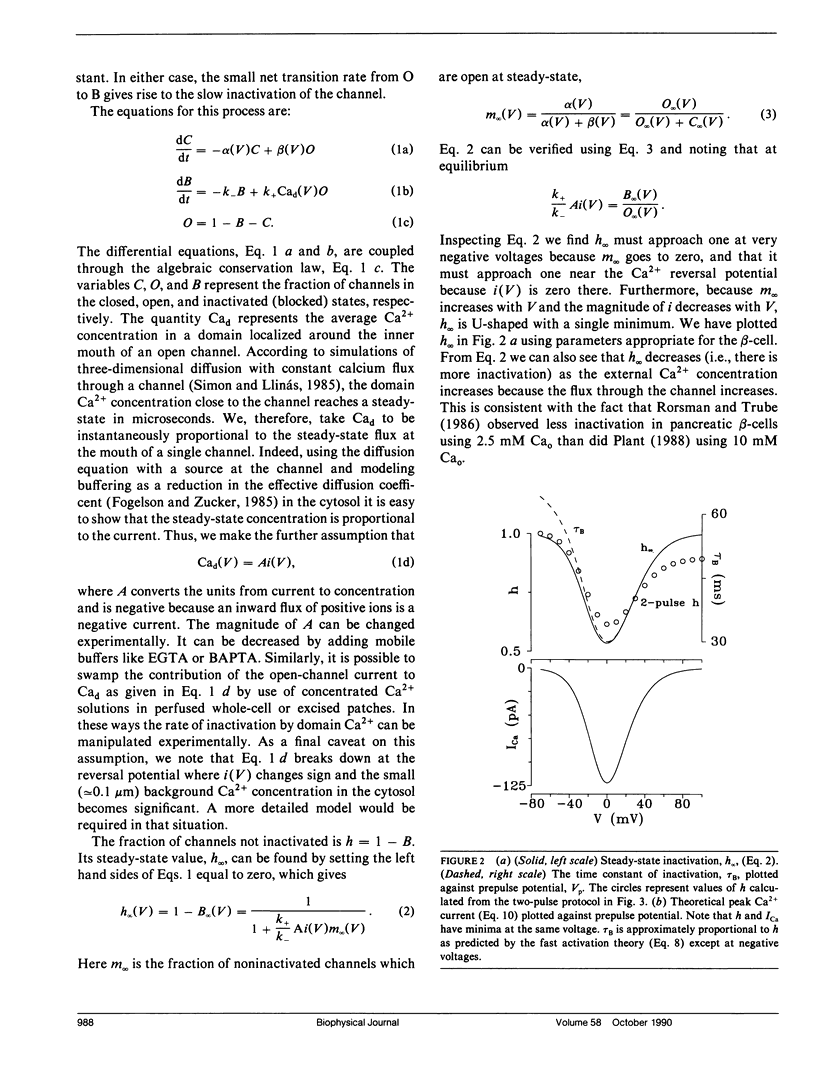
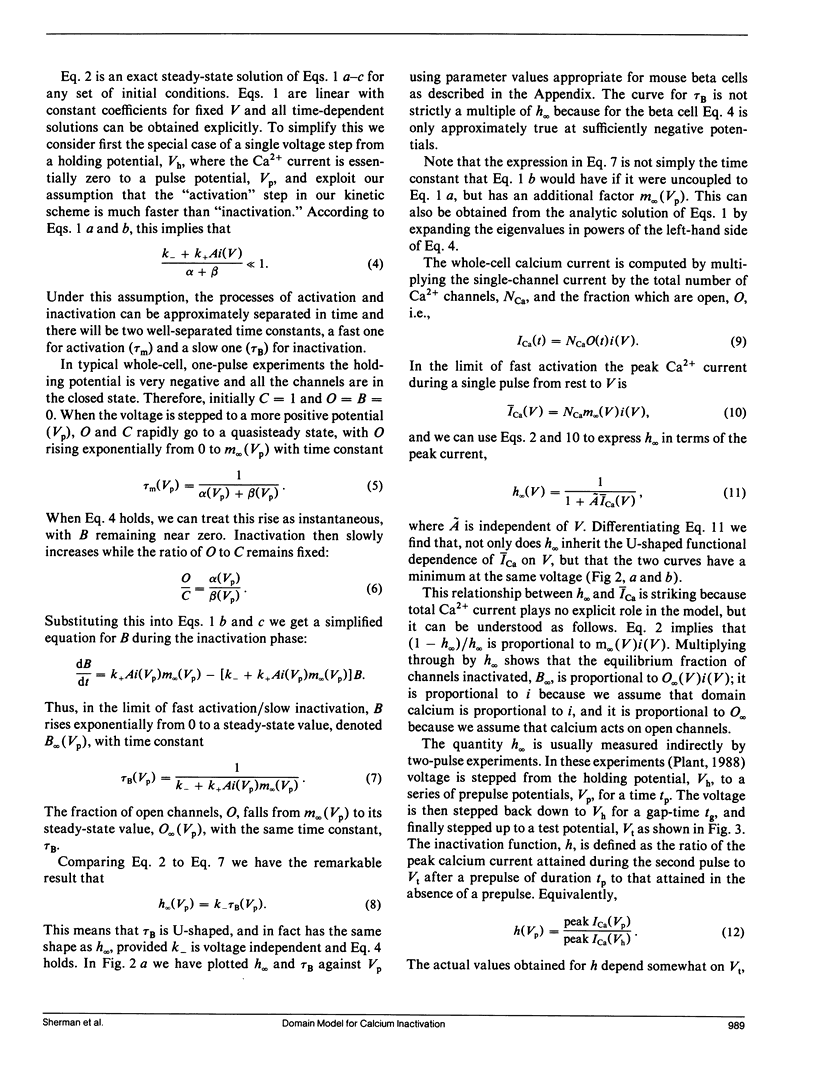
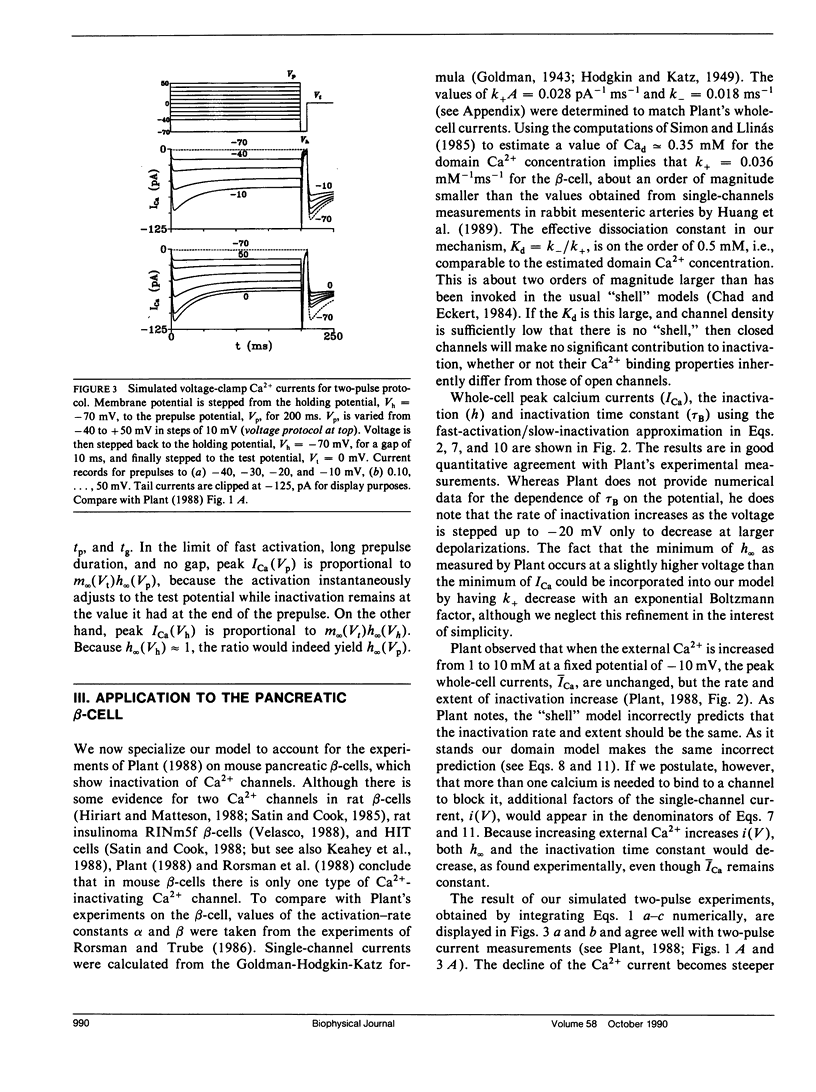
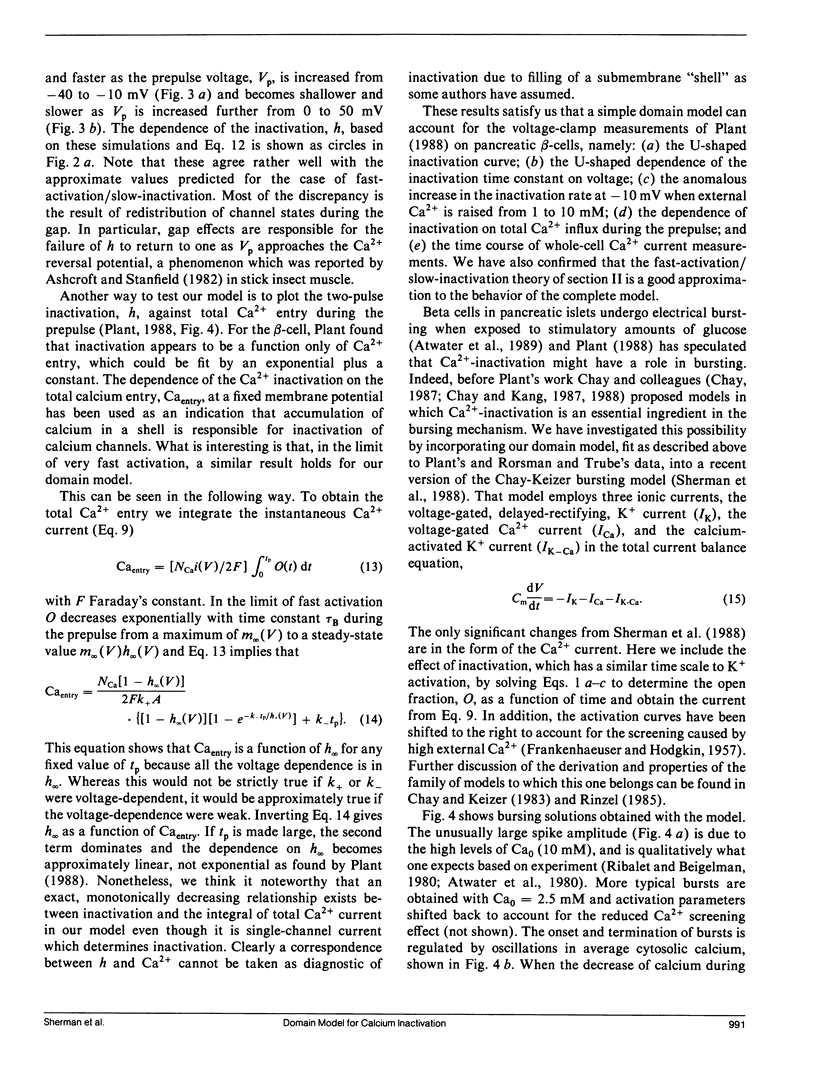
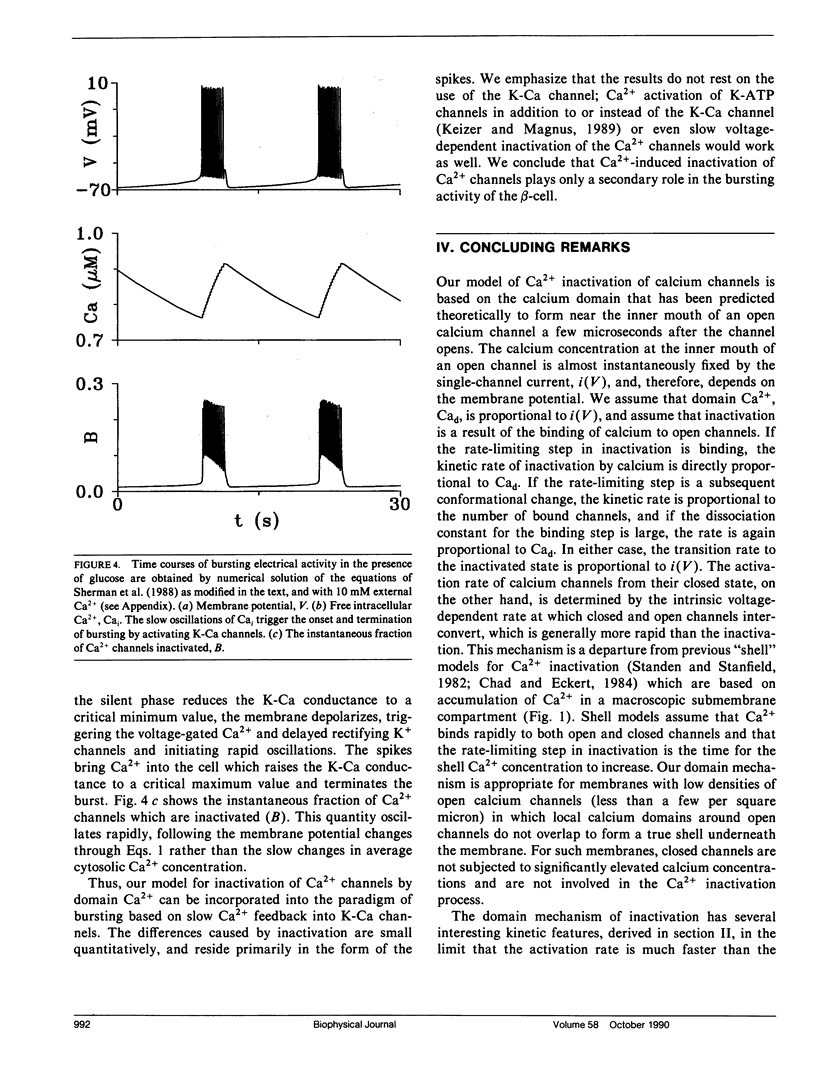
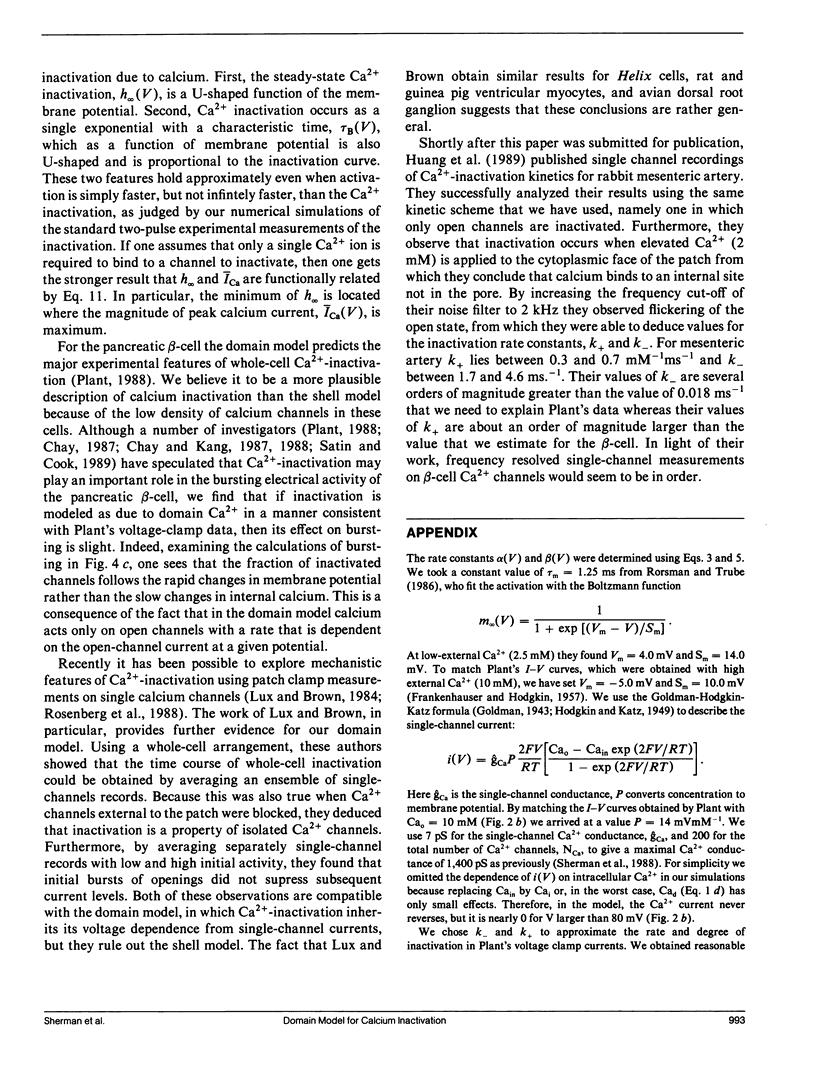
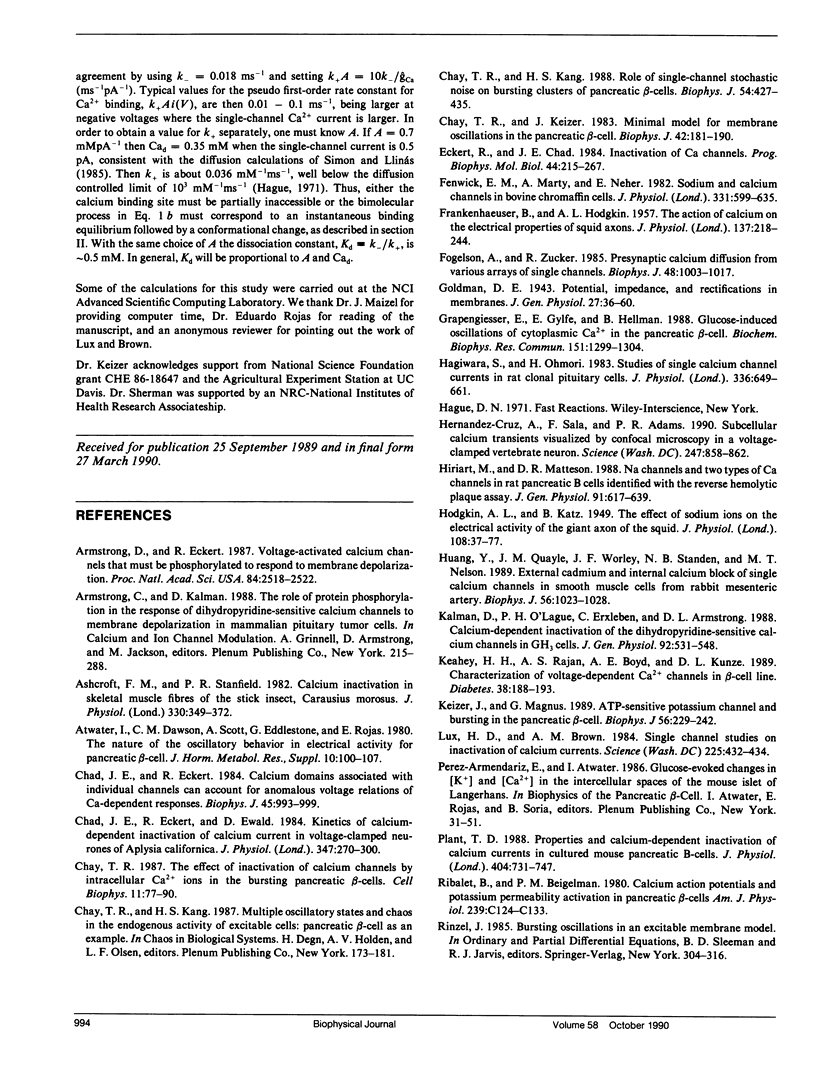
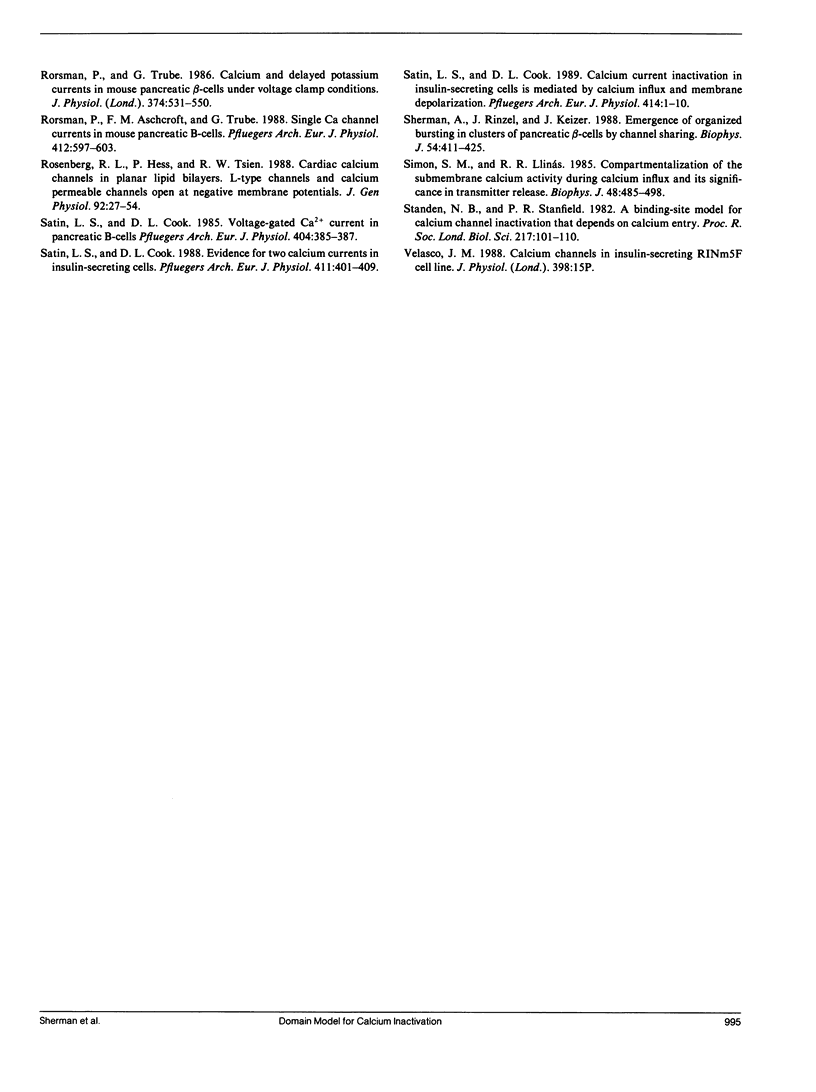
Selected References
These references are in PubMed. This may not be the complete list of references from this article.
- Armstrong D., Eckert R. Voltage-activated calcium channels that must be phosphorylated to respond to membrane depolarization. Proc Natl Acad Sci U S A. 1987 Apr;84(8):2518–2522. doi: 10.1073/pnas.84.8.2518. [DOI] [PMC free article] [PubMed] [Google Scholar]
- Ashcroft F. M., Stanfield P. R. Calcium inactivation in skeletal muscle fibres of the stick insect, Carausius morosus. J Physiol. 1982 Sep;330:349–372. doi: 10.1113/jphysiol.1982.sp014345. [DOI] [PMC free article] [PubMed] [Google Scholar]
- Atwater I., Dawson C. M., Scott A., Eddlestone G., Rojas E. The nature of the oscillatory behaviour in electrical activity from pancreatic beta-cell. Horm Metab Res Suppl. 1980;Suppl 10:100–107. [PubMed] [Google Scholar]
- Chad J. E., Eckert R. Calcium domains associated with individual channels can account for anomalous voltage relations of CA-dependent responses. Biophys J. 1984 May;45(5):993–999. doi: 10.1016/S0006-3495(84)84244-7. [DOI] [PMC free article] [PubMed] [Google Scholar]
- Chad J., Eckert R., Ewald D. Kinetics of calcium-dependent inactivation of calcium current in voltage-clamped neurones of Aplysia californica. J Physiol. 1984 Feb;347:279–300. doi: 10.1113/jphysiol.1984.sp015066. [DOI] [PMC free article] [PubMed] [Google Scholar]
- Chay T. R., Kang H. S. Role of single-channel stochastic noise on bursting clusters of pancreatic beta-cells. Biophys J. 1988 Sep;54(3):427–435. doi: 10.1016/S0006-3495(88)82976-X. [DOI] [PMC free article] [PubMed] [Google Scholar]
- Chay T. R., Keizer J. Minimal model for membrane oscillations in the pancreatic beta-cell. Biophys J. 1983 May;42(2):181–190. doi: 10.1016/S0006-3495(83)84384-7. [DOI] [PMC free article] [PubMed] [Google Scholar]
- Chay T. R. The effect of inactivation of calcium channels by intracellular Ca2+ ions in the bursting pancreatic beta-cells. Cell Biophys. 1987 Dec;11:77–90. doi: 10.1007/BF02797114. [DOI] [PubMed] [Google Scholar]
- Eckert R., Chad J. E. Inactivation of Ca channels. Prog Biophys Mol Biol. 1984;44(3):215–267. doi: 10.1016/0079-6107(84)90009-9. [DOI] [PubMed] [Google Scholar]
- FRANKENHAEUSER B., HODGKIN A. L. The action of calcium on the electrical properties of squid axons. J Physiol. 1957 Jul 11;137(2):218–244. doi: 10.1113/jphysiol.1957.sp005808. [DOI] [PMC free article] [PubMed] [Google Scholar]
- Fenwick E. M., Marty A., Neher E. Sodium and calcium channels in bovine chromaffin cells. J Physiol. 1982 Oct;331:599–635. doi: 10.1113/jphysiol.1982.sp014394. [DOI] [PMC free article] [PubMed] [Google Scholar]
- Fogelson A. L., Zucker R. S. Presynaptic calcium diffusion from various arrays of single channels. Implications for transmitter release and synaptic facilitation. Biophys J. 1985 Dec;48(6):1003–1017. doi: 10.1016/S0006-3495(85)83863-7. [DOI] [PMC free article] [PubMed] [Google Scholar]
- Goldman D. E. POTENTIAL, IMPEDANCE, AND RECTIFICATION IN MEMBRANES. J Gen Physiol. 1943 Sep 20;27(1):37–60. doi: 10.1085/jgp.27.1.37. [DOI] [PMC free article] [PubMed] [Google Scholar]
- Grapengiesser E., Gylfe E., Hellman B. Glucose-induced oscillations of cytoplasmic Ca2+ in the pancreatic beta-cell. Biochem Biophys Res Commun. 1988 Mar 30;151(3):1299–1304. doi: 10.1016/s0006-291x(88)80503-5. [DOI] [PubMed] [Google Scholar]
- HODGKIN A. L., KATZ B. The effect of sodium ions on the electrical activity of giant axon of the squid. J Physiol. 1949 Mar 1;108(1):37–77. doi: 10.1113/jphysiol.1949.sp004310. [DOI] [PMC free article] [PubMed] [Google Scholar]
- Hagiwara S., Ohmori H. Studies of single calcium channel currents in rat clonal pituitary cells. J Physiol. 1983 Mar;336:649–661. doi: 10.1113/jphysiol.1983.sp014603. [DOI] [PMC free article] [PubMed] [Google Scholar]
- Hernández-Cruz A., Sala F., Adams P. R. Subcellular calcium transients visualized by confocal microscopy in a voltage-clamped vertebrate neuron. Science. 1990 Feb 16;247(4944):858–862. doi: 10.1126/science.2154851. [DOI] [PubMed] [Google Scholar]
- Hiriart M., Matteson D. R. Na channels and two types of Ca channels in rat pancreatic B cells identified with the reverse hemolytic plaque assay. J Gen Physiol. 1988 May;91(5):617–639. doi: 10.1085/jgp.91.5.617. [DOI] [PMC free article] [PubMed] [Google Scholar]
- Huang Y., Quayle J. M., Worley J. F., Standen N. B., Nelson M. T. External cadmium and internal calcium block of single calcium channels in smooth muscle cells from rabbit mesenteric artery. Biophys J. 1989 Nov;56(5):1023–1028. doi: 10.1016/S0006-3495(89)82747-X. [DOI] [PMC free article] [PubMed] [Google Scholar]
- Kalman D., O'Lague P. H., Erxleben C., Armstrong D. L. Calcium-dependent inactivation of the dihydropyridine-sensitive calcium channels in GH3 cells. J Gen Physiol. 1988 Oct;92(4):531–548. doi: 10.1085/jgp.92.4.531. [DOI] [PMC free article] [PubMed] [Google Scholar]
- Keahey H. H., Rajan A. S., Boyd A. E., 3rd, Kunze D. L. Characterization of voltage-dependent Ca2+ channels in beta-cell line. Diabetes. 1989 Feb;38(2):188–193. doi: 10.2337/diab.38.2.188. [DOI] [PubMed] [Google Scholar]
- Keizer J., Magnus G. ATP-sensitive potassium channel and bursting in the pancreatic beta cell. A theoretical study. Biophys J. 1989 Aug;56(2):229–242. doi: 10.1016/S0006-3495(89)82669-4. [DOI] [PMC free article] [PubMed] [Google Scholar]
- Lux H. D., Brown A. M. Single channel studies on inactivation of calcium currents. Science. 1984 Jul 27;225(4660):432–434. doi: 10.1126/science.6330896. [DOI] [PubMed] [Google Scholar]
- Perez-Armendariz E., Atwater I. Glucose-evoked changes in [K+] and [Ca2+] in the intercellular spaces of the mouse islet of Langerhans. Adv Exp Med Biol. 1986;211:31–51. doi: 10.1007/978-1-4684-5314-0_3. [DOI] [PubMed] [Google Scholar]
- Plant T. D. Properties and calcium-dependent inactivation of calcium currents in cultured mouse pancreatic B-cells. J Physiol. 1988 Oct;404:731–747. doi: 10.1113/jphysiol.1988.sp017316. [DOI] [PMC free article] [PubMed] [Google Scholar]
- Ribalet B., Beigelman P. M. Calcium action potentials and potassium permeability activation in pancreatic beta-cells. Am J Physiol. 1980 Sep;239(3):C124–C133. doi: 10.1152/ajpcell.1980.239.3.C124. [DOI] [PubMed] [Google Scholar]
- Rorsman P., Ashcroft F. M., Trube G. Single Ca channel currents in mouse pancreatic B-cells. Pflugers Arch. 1988 Oct;412(6):597–603. doi: 10.1007/BF00583760. [DOI] [PubMed] [Google Scholar]
- Rorsman P., Trube G. Calcium and delayed potassium currents in mouse pancreatic beta-cells under voltage-clamp conditions. J Physiol. 1986 May;374:531–550. doi: 10.1113/jphysiol.1986.sp016096. [DOI] [PMC free article] [PubMed] [Google Scholar]
- Rosenberg R. L., Hess P., Tsien R. W. Cardiac calcium channels in planar lipid bilayers. L-type channels and calcium-permeable channels open at negative membrane potentials. J Gen Physiol. 1988 Jul;92(1):27–54. doi: 10.1085/jgp.92.1.27. [DOI] [PMC free article] [PubMed] [Google Scholar]
- Satin L. S., Cook D. L. Calcium current inactivation in insulin-secreting cells is mediated by calcium influx and membrane depolarization. Pflugers Arch. 1989 May;414(1):1–10. doi: 10.1007/BF00585619. [DOI] [PubMed] [Google Scholar]
- Satin L. S., Cook D. L. Evidence for two calcium currents in insulin-secreting cells. Pflugers Arch. 1988 Apr;411(4):401–409. doi: 10.1007/BF00587719. [DOI] [PubMed] [Google Scholar]
- Satin L. S., Cook D. L. Voltage-gated Ca2+ current in pancreatic B-cells. Pflugers Arch. 1985 Aug;404(4):385–387. doi: 10.1007/BF00585354. [DOI] [PubMed] [Google Scholar]
- Sherman A., Rinzel J., Keizer J. Emergence of organized bursting in clusters of pancreatic beta-cells by channel sharing. Biophys J. 1988 Sep;54(3):411–425. doi: 10.1016/S0006-3495(88)82975-8. [DOI] [PMC free article] [PubMed] [Google Scholar]
- Simon S. M., Llinás R. R. Compartmentalization of the submembrane calcium activity during calcium influx and its significance in transmitter release. Biophys J. 1985 Sep;48(3):485–498. doi: 10.1016/S0006-3495(85)83804-2. [DOI] [PMC free article] [PubMed] [Google Scholar]
- Standen N. B., Stanfield P. R. A binding-site model for calcium channel inactivation that depends on calcium entry. Proc R Soc Lond B Biol Sci. 1982 Dec 22;217(1206):101–110. doi: 10.1098/rspb.1982.0097. [DOI] [PubMed] [Google Scholar]