Abstract
1. The oxygen consumption and the movements of labelled phosphate were measured in garfish olfactory nerve at rest and during activity.
2. In solutions with 2·5 mM-K and 0·2 mM-phosphate the resting oxygen consumption was 0·206 m-mole/kg.min; activity at 2 sec-1 produced an extra oxygen consumption of 2·46 μmole/kg.impulse. The extra oxygen consumption declined exponentially with a time constant of 2·62 min at 22-26 °C.
3. The phosphate efflux, measured simultaneously, had a resting efflux rate constant of 1·24 × 10-3 min-1; activity at 2 sec-1 produced an extra fractional loss of 9·38 × 10-6 impulse-1. The increase in phosphate efflux followed almost the same time course as the increase in oxygen consumption.
4. Increasing the frequency of stimulation from 2 sec-1 to 3 or 5 sec-1 decreased both the extra oxygen consumption and the extra fractional loss of phosphate. When the frequency was decreased to 0·5 or 1 sec-1 the extra oxygen consumption per impulse increased, while the extra phosphate liberation was lowered.
5. Changing the phosphate concentration did not much affect the extra oxygen consumption; on the other hand, lowering or increasing the phosphate from the standard 0.2 mM decreased both the resting and the stimulated phosphate efflux.
6. Lowering the K from the standard 2·5 mM did not affect the extra oxygen consumption, but increased both the resting and the extra loss of phosphate. At higher K concentrations the extra oxygen consumption and the extra fractional loss of phosphate decreased without much change in the resting phosphate efflux.
7. Application of 1-20 μM-strophanthidin produced a transient decrease in the resting phosphate efflux without much change in resting oxygen consumption. With 10 or 20 μM-strophanthidin the extra fractional loss of phosphate and the extra oxygen consumption were both lowered in approximately the same proportions.
8. The findings are consistent with the hypothesis that the increase in intracellular inorganic phosphate that results from increased break-down of ATP after activity, is the main cause for the increased phosphate efflux. A fraction of the increase in intracellular phosphate only appears to be liberated to the outside, the value of the fraction depending on the resting phosphate efflux before activity.
9. The initial increase in intracellular inorganic phosphate after an impulse, estimated from the oxygen consumption or the phosphate fluxes, appears to be about 12-19 μmole/kg nerve, remarkably close to the value known from chemical analysis.
Full text
PDF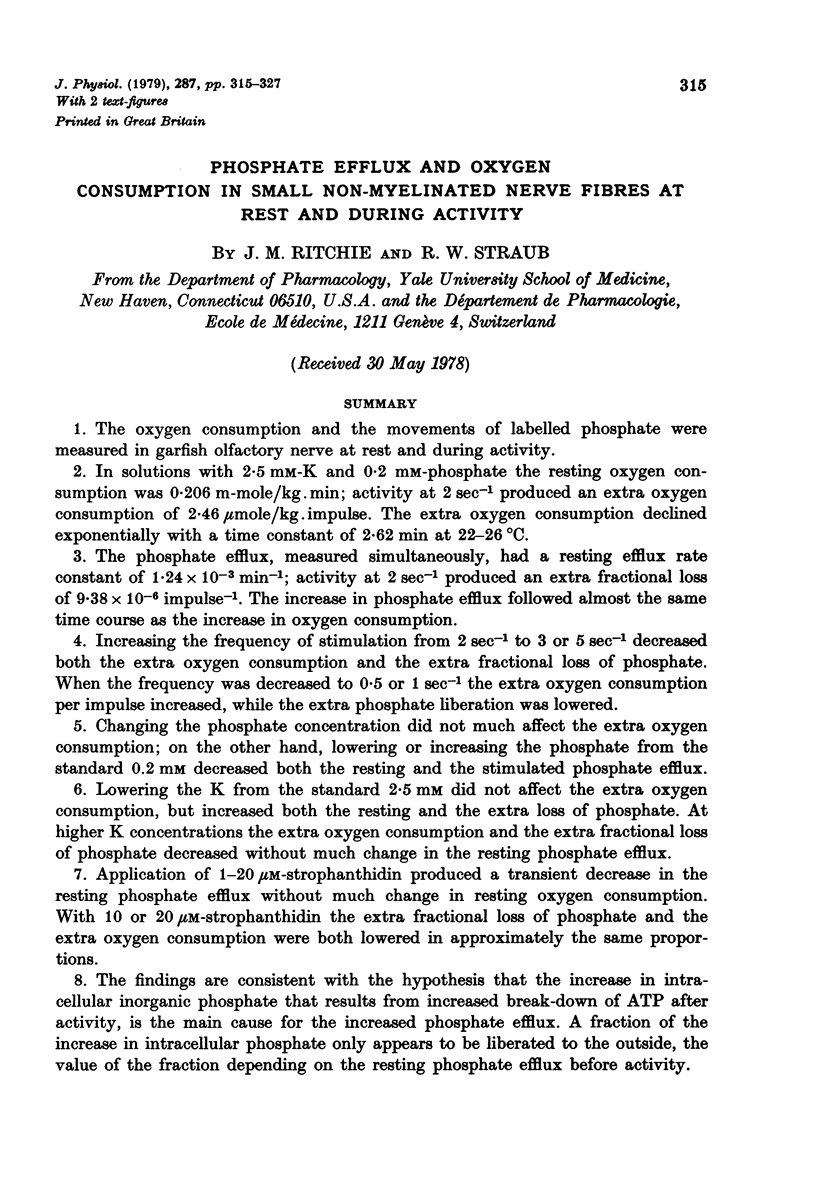
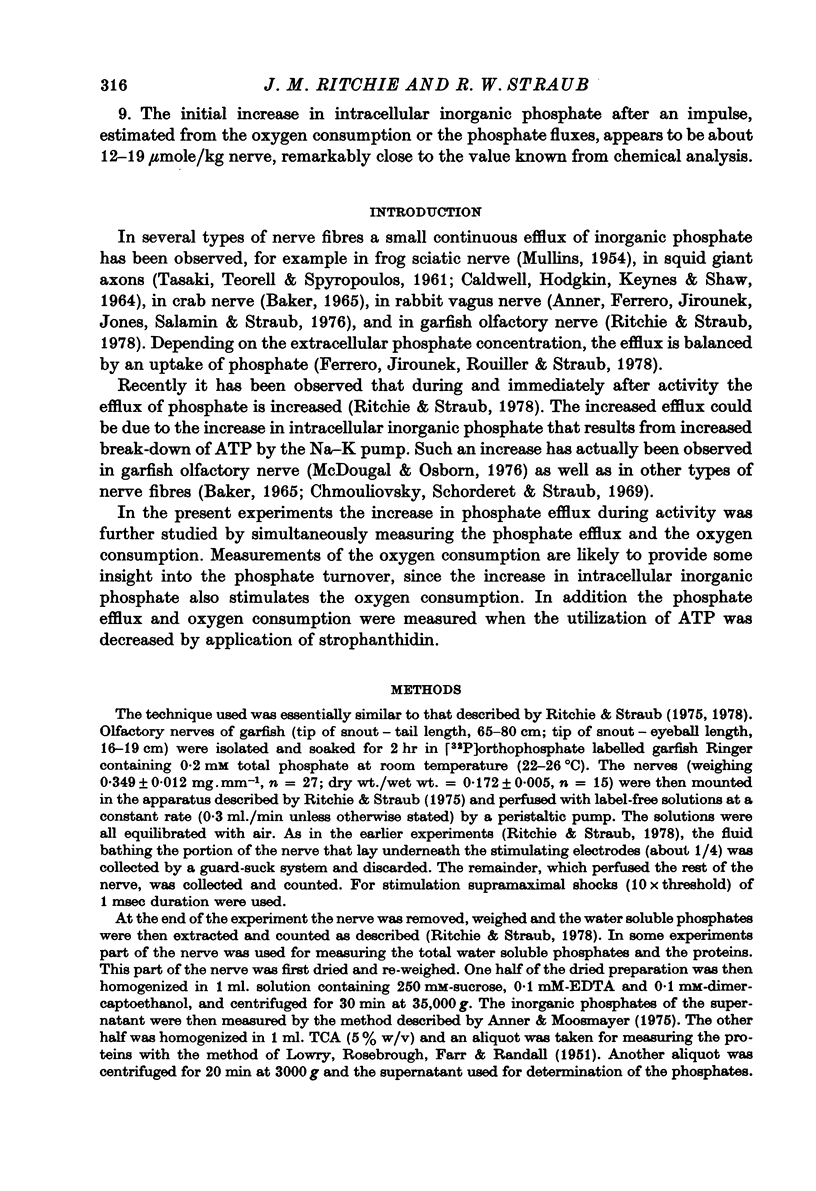
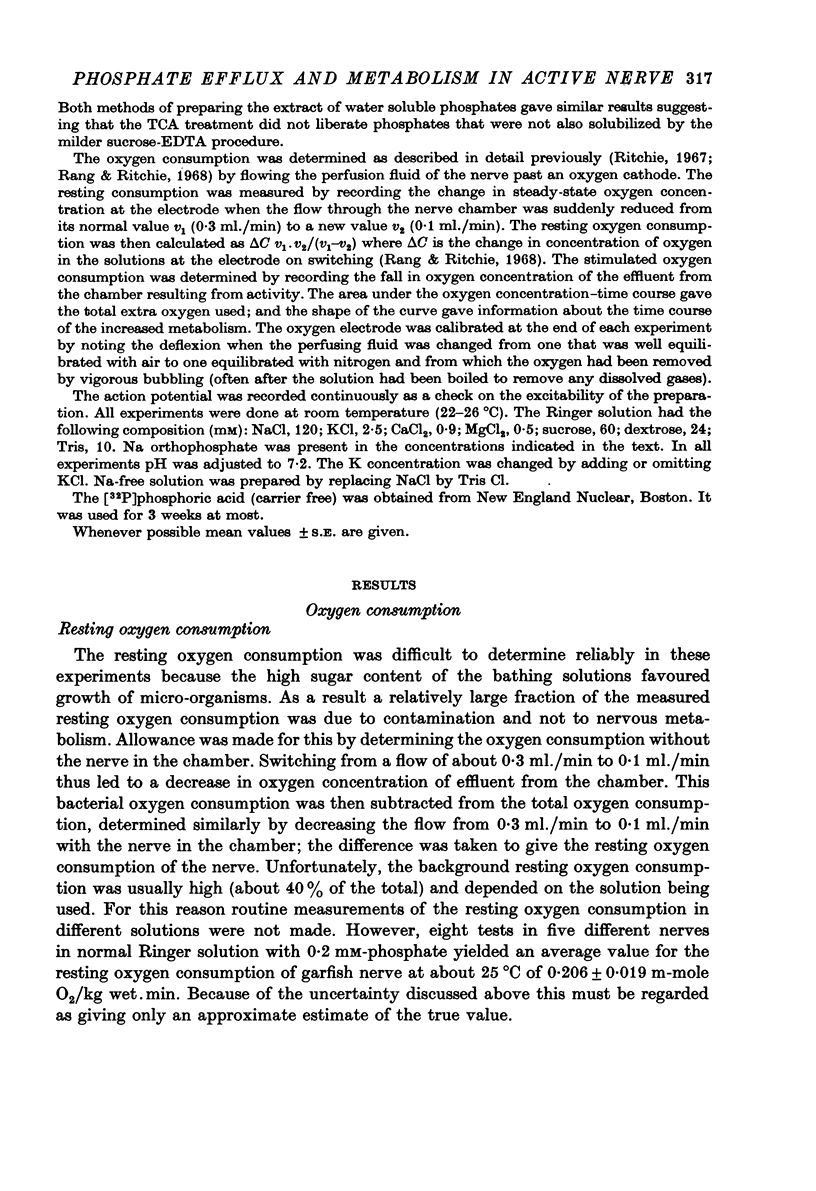
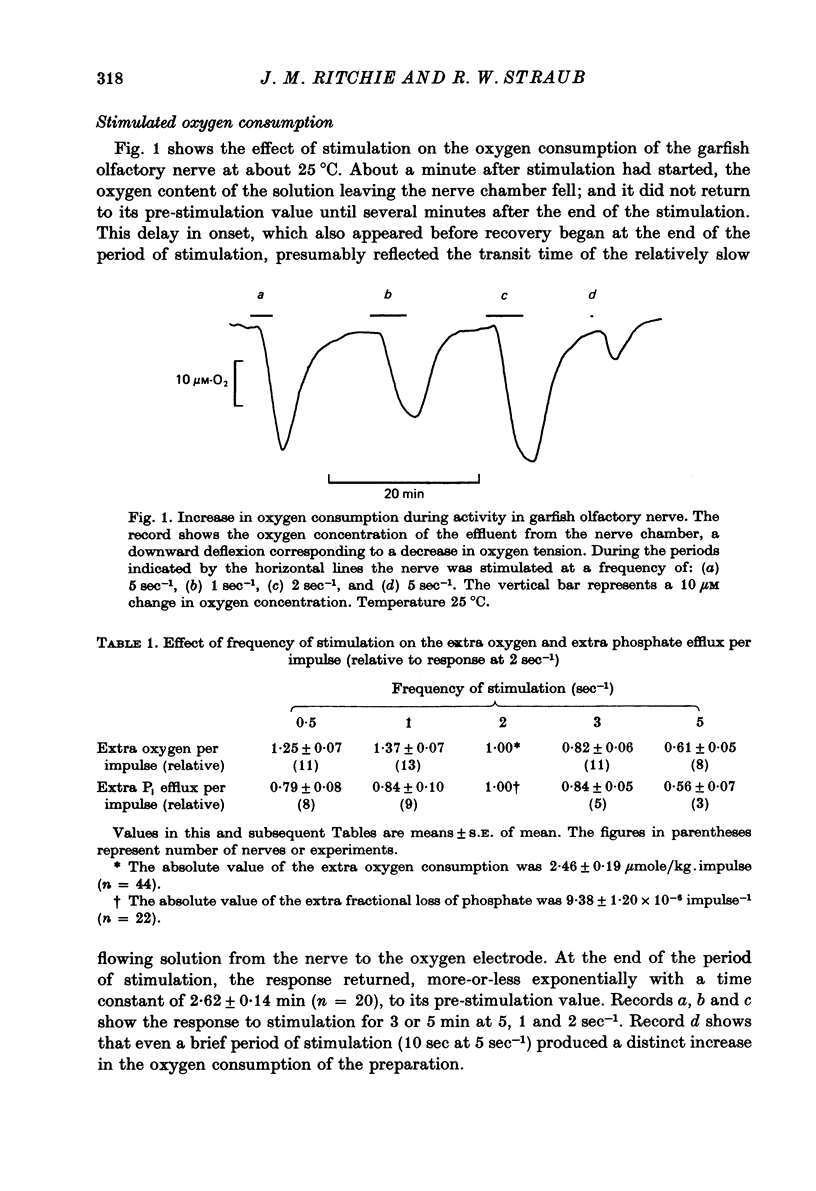
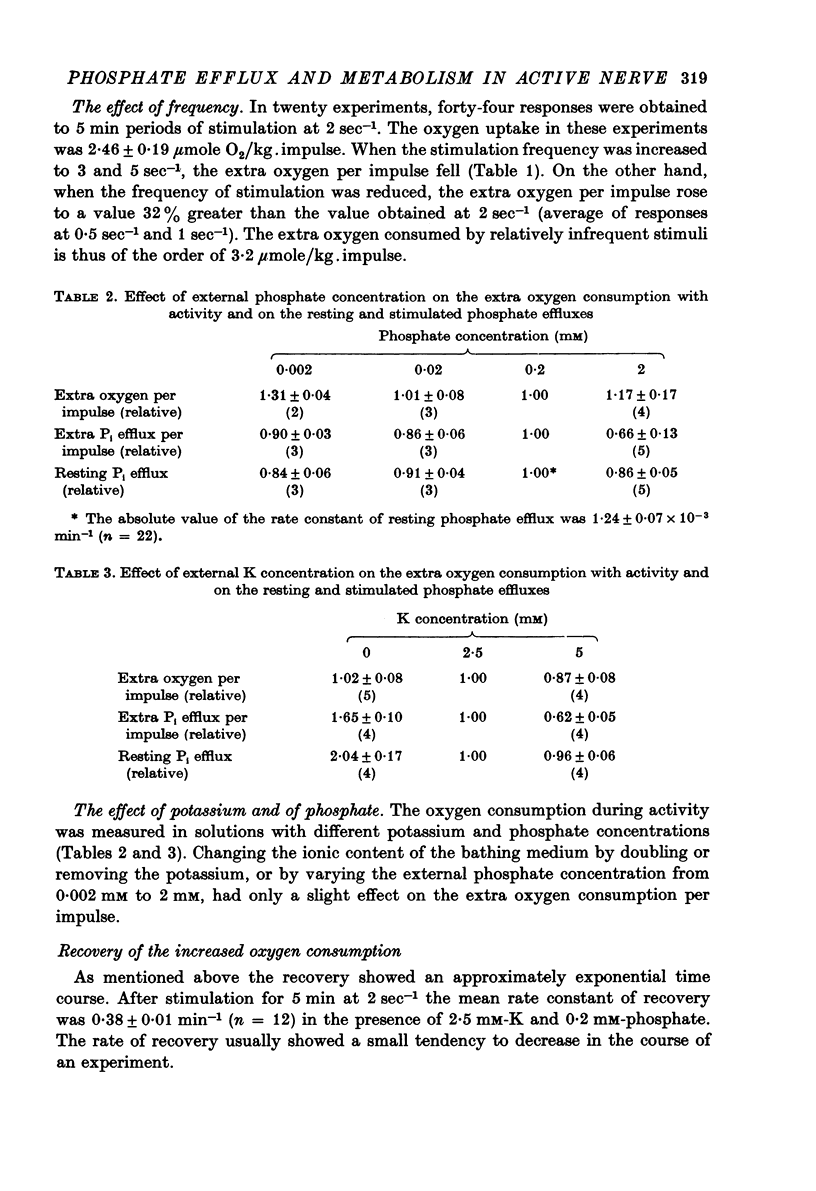
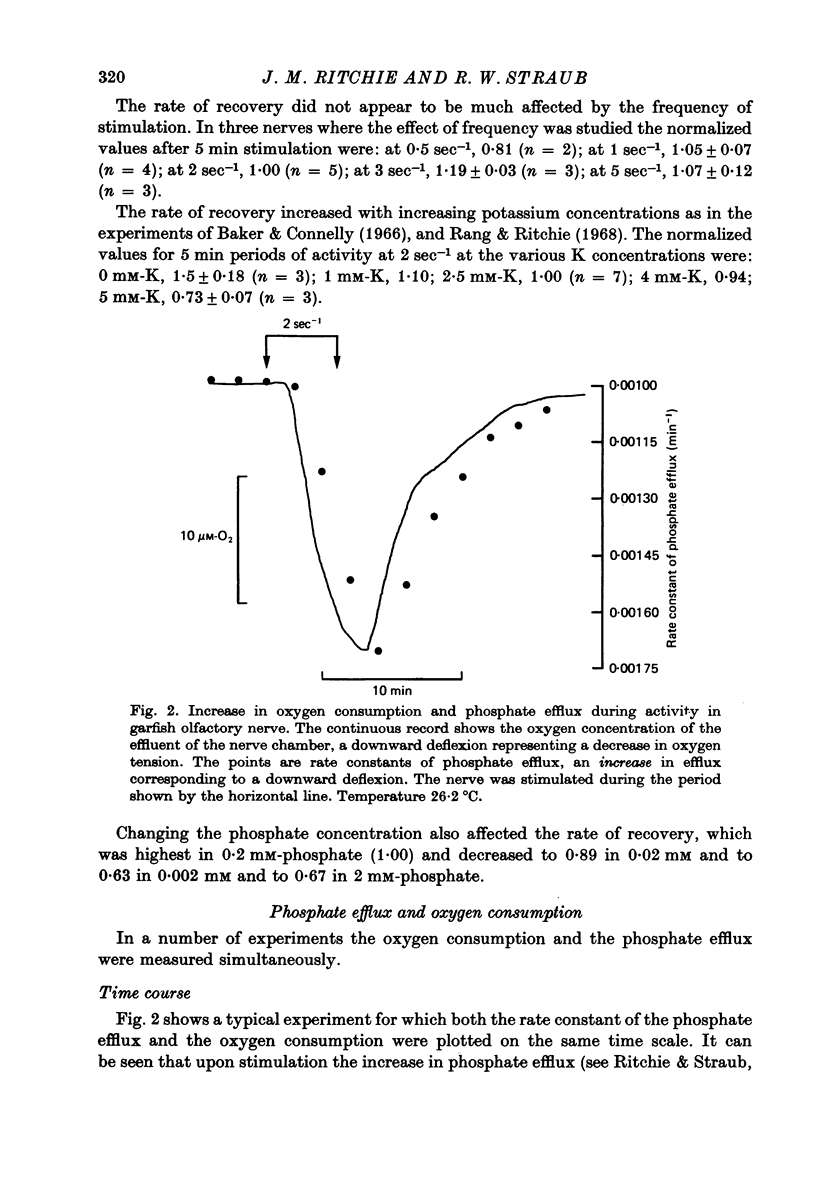
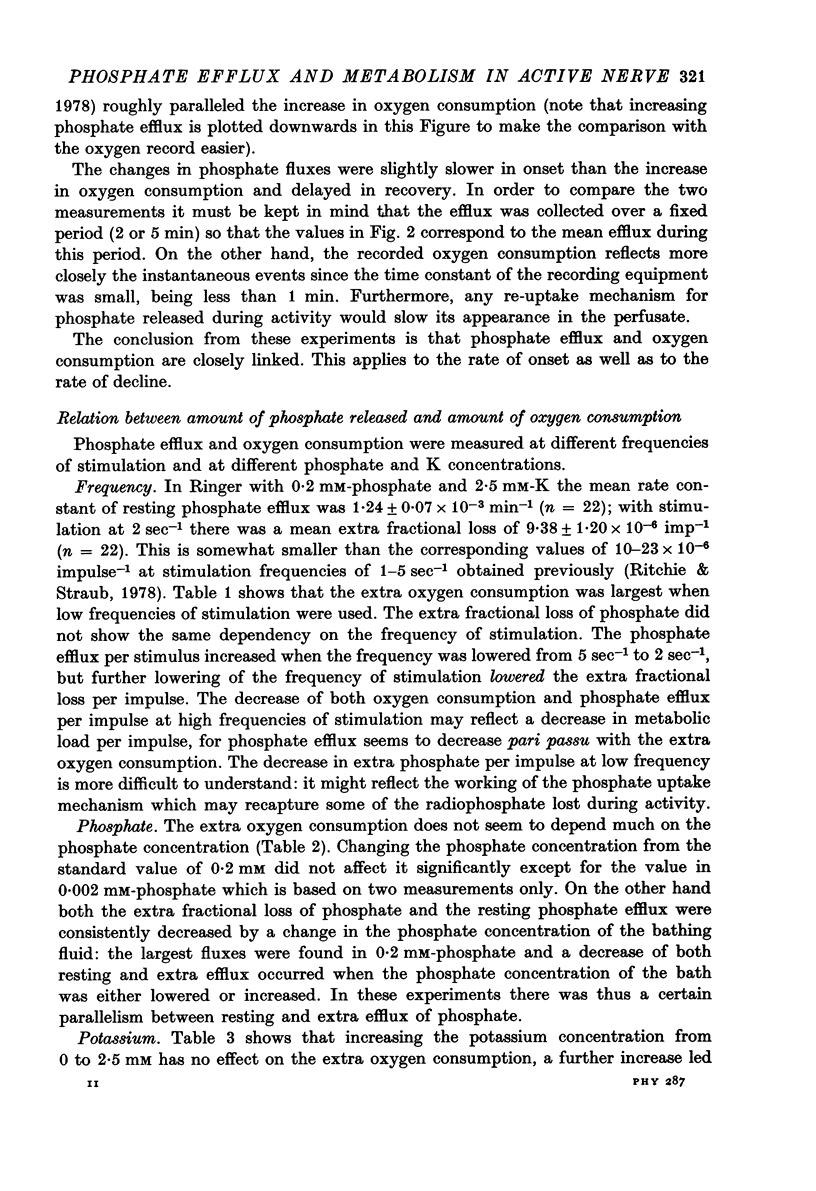
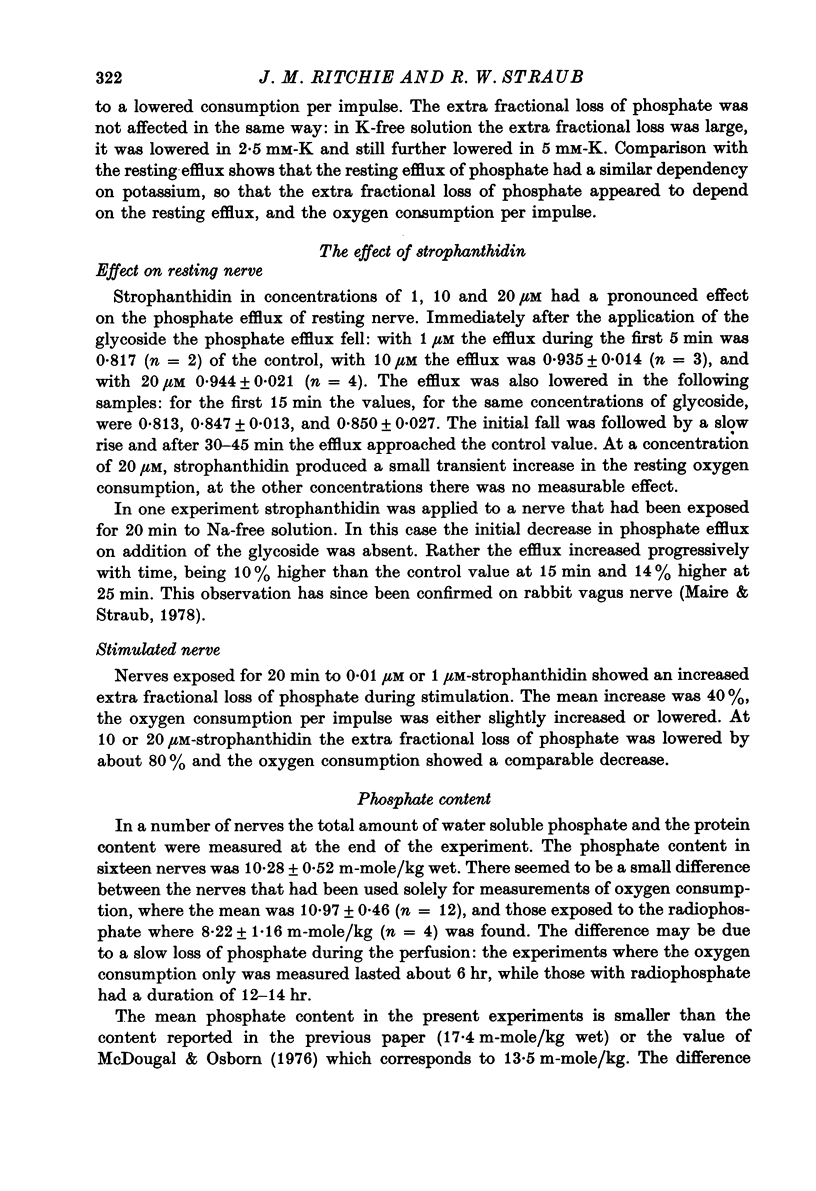
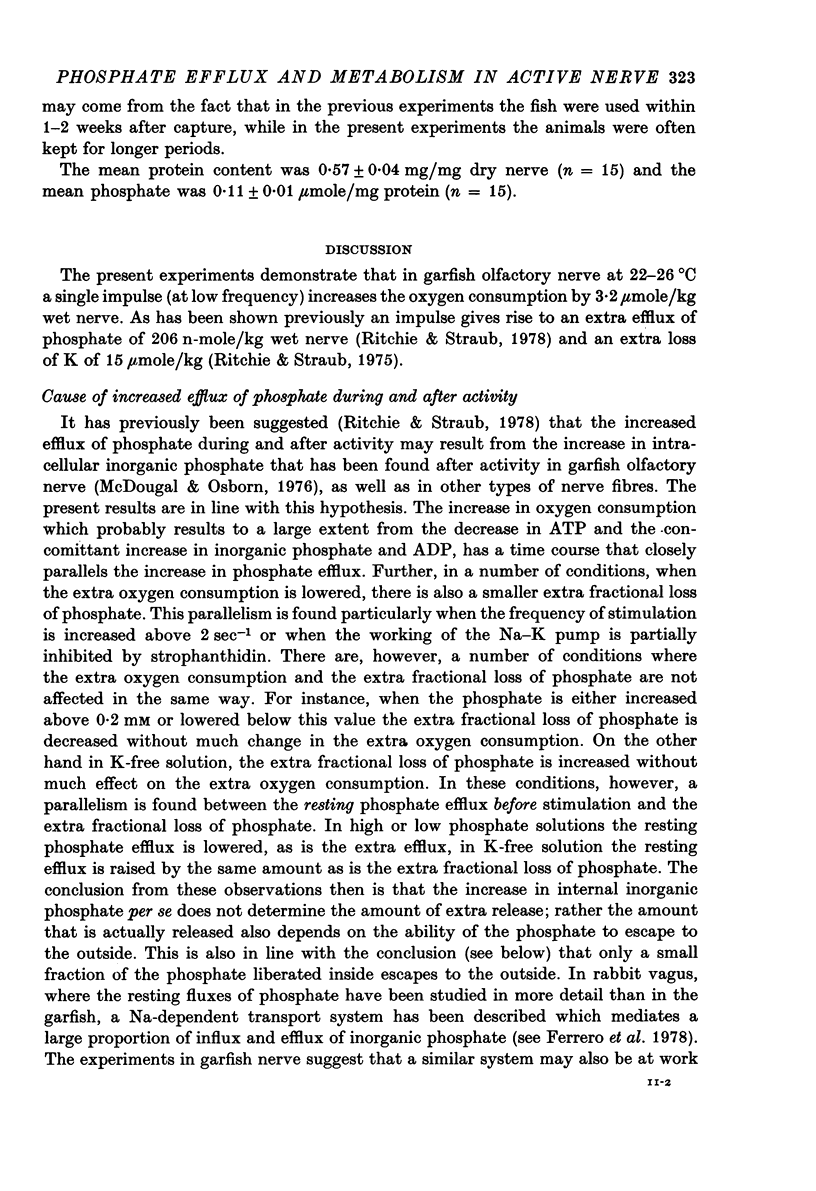
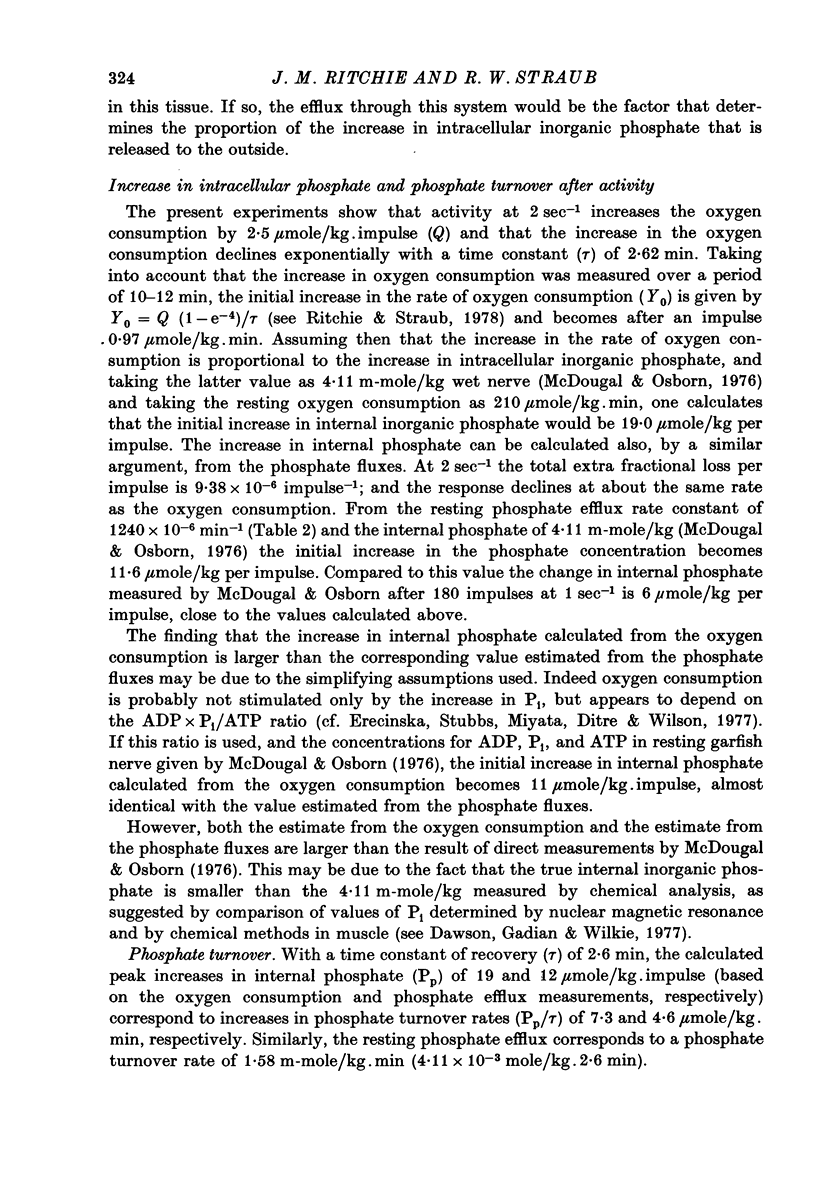
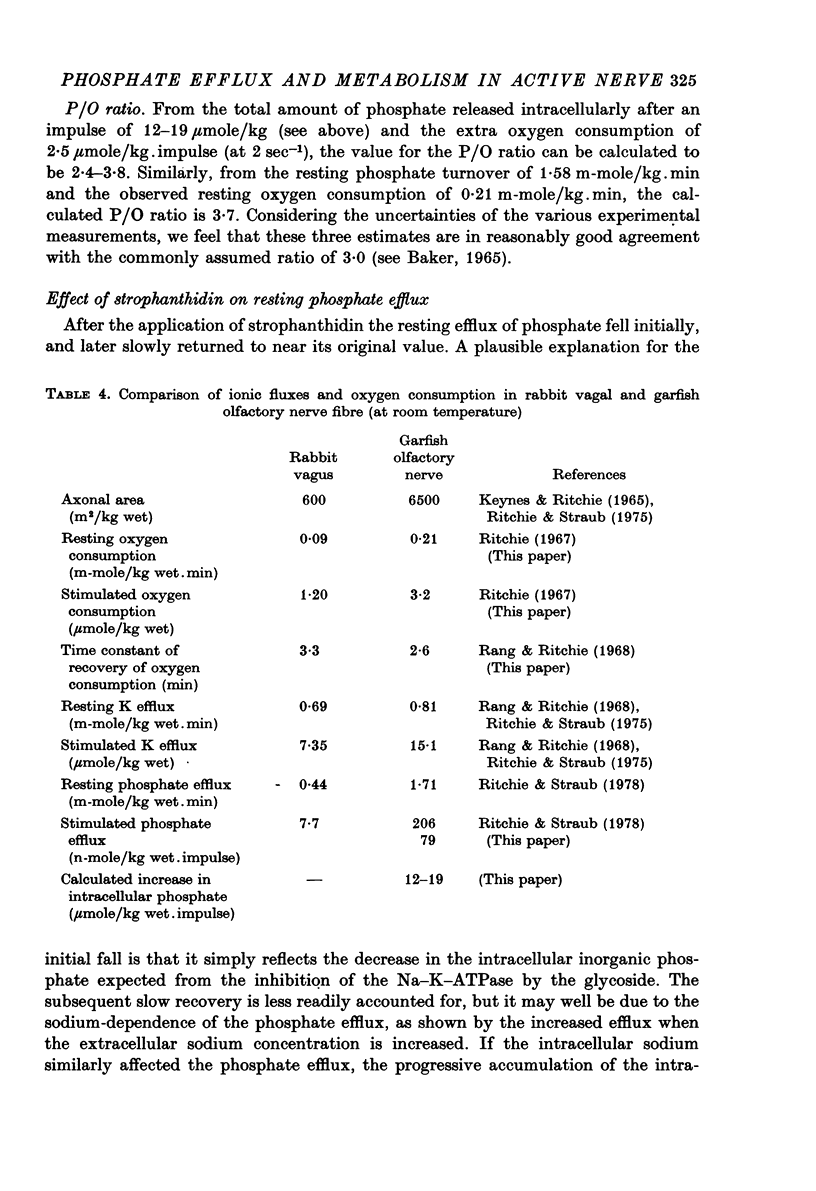
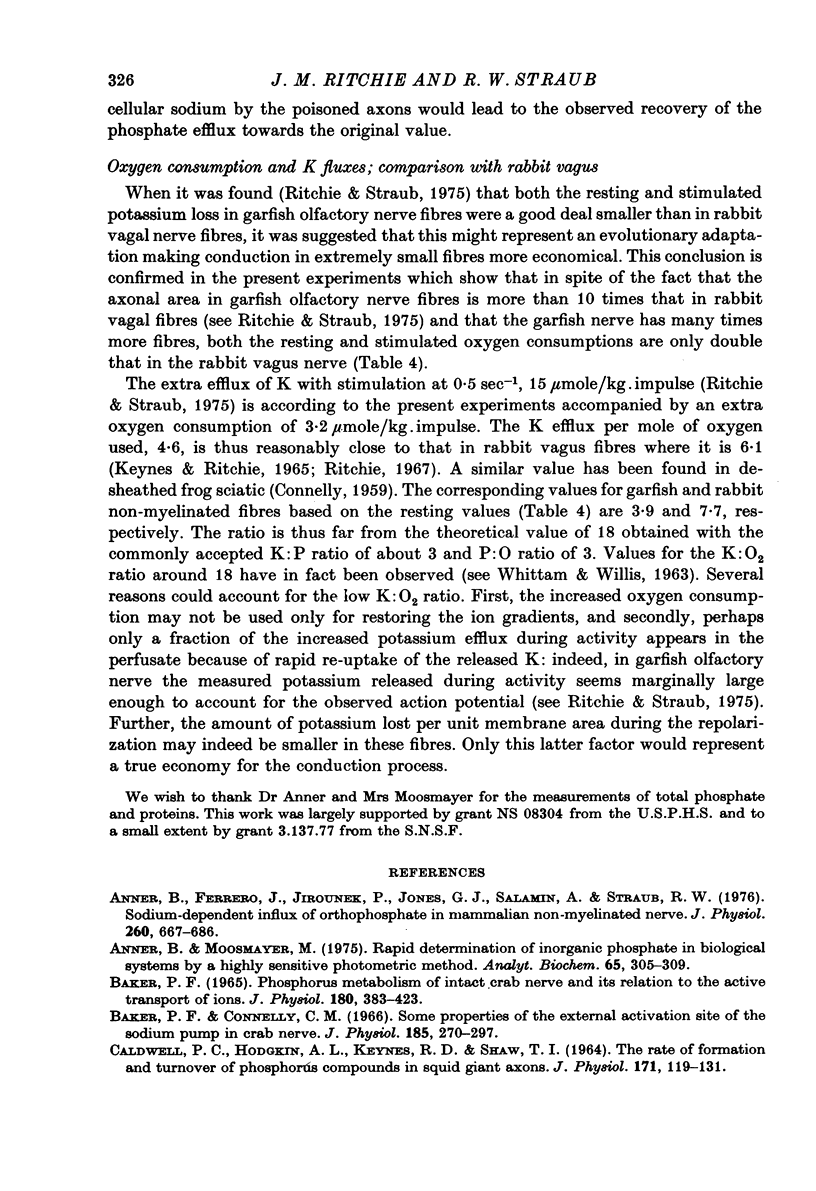
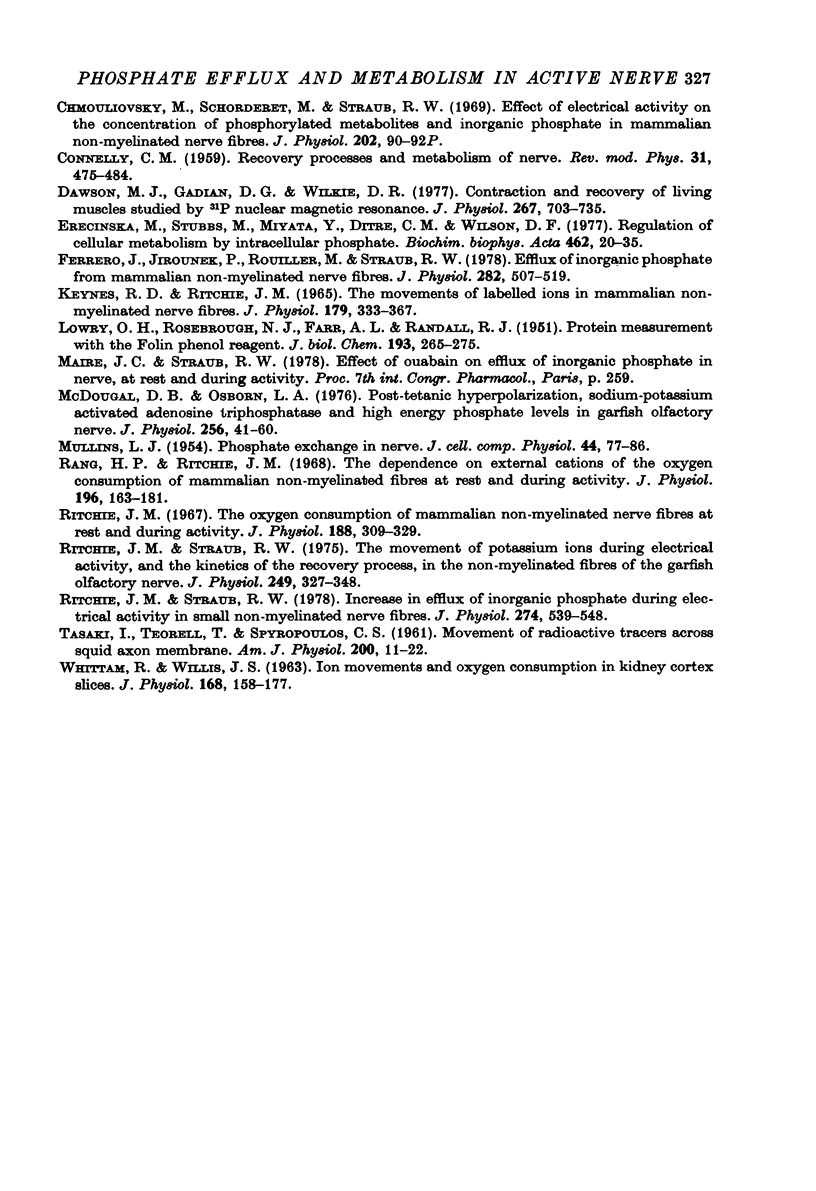
Selected References
These references are in PubMed. This may not be the complete list of references from this article.
- Anner B., Ferrero J., Jirounek P., Jones G. J., Salamin A., Straub R. W. Sodium-dependent influx of orthophosphate in mammalian non-myelinated nerve. J Physiol. 1976 Sep;260(3):667–686. doi: 10.1113/jphysiol.1976.sp011538. [DOI] [PMC free article] [PubMed] [Google Scholar]
- Baker P. F., Connelly C. M. Some properties of the external activation site of the sodium pump in crab nerve. J Physiol. 1966 Jul;185(2):270–297. doi: 10.1113/jphysiol.1966.sp007987. [DOI] [PMC free article] [PubMed] [Google Scholar]
- CALDWELL P. C., HODGKIN A. L., KEYNES R. D., SHAW T. I. THE RATE OF FORMATION AND TURNOVER OF PHOSPHORUS COMPOUNDS IN SQUID GIANT AXONS. J Physiol. 1964 May;171:119–131. doi: 10.1113/jphysiol.1964.sp007366. [DOI] [PMC free article] [PubMed] [Google Scholar]
- Chmouliovsky M., Schorderet M., Straub R. W. Effect of electrical activity on the concentration of phosphorylated metabolites and inorganic phosphate in mammalian non-myelinated nerve fibres. J Physiol. 1969 Jun;202(2):90P–92P. [PubMed] [Google Scholar]
- Dawson M. J., Gadian D. G., Wilkie D. R. Contraction and recovery of living muscles studies by 31P nuclear magnetic resonance. J Physiol. 1977 Jun;267(3):703–735. doi: 10.1113/jphysiol.1977.sp011835. [DOI] [PMC free article] [PubMed] [Google Scholar]
- Erecińska M., Stubbs M., Miyata Y., Ditre C. M. Regulation of cellular metabolism by intracellular phosphate. Biochim Biophys Acta. 1977 Oct 12;462(1):20–35. doi: 10.1016/0005-2728(77)90186-4. [DOI] [PubMed] [Google Scholar]
- Ferrero J., Jirounek P., Rouiller M., Straub R. W. Efflux of inorganic phosphate from mammalian non-myelinated nerve fibres. J Physiol. 1978 Sep;282:507–519. doi: 10.1113/jphysiol.1978.sp012478. [DOI] [PMC free article] [PubMed] [Google Scholar]
- Keynes R. D., Ritchie J. M. The movements of labelled ions in mammalian non-myelinated nerve fibres. J Physiol. 1965 Jul;179(2):333–367. doi: 10.1113/jphysiol.1965.sp007666. [DOI] [PMC free article] [PubMed] [Google Scholar]
- LOWRY O. H., ROSEBROUGH N. J., FARR A. L., RANDALL R. J. Protein measurement with the Folin phenol reagent. J Biol Chem. 1951 Nov;193(1):265–275. [PubMed] [Google Scholar]
- McDougal D. B., Osborn L. A. Post-tetanic hyperpolarization, sodium-potassium-activated adenosine triphosphatase and high energy phosphate levels in garfish olfactory nerve. J Physiol. 1976 Mar;256(1):41–60. doi: 10.1113/jphysiol.1976.sp011310. [DOI] [PMC free article] [PubMed] [Google Scholar]
- Rang H. P., Ritchie J. M. The dependence on external cations of the oxygen consumption of mammalian non-myelinated fibres at rest and during activity. J Physiol. 1968 May;196(1):163–181. doi: 10.1113/jphysiol.1968.sp008501. [DOI] [PMC free article] [PubMed] [Google Scholar]
- Ritchie J. M., Straub R. W. Increase in efflux of inorganic phosphate during electrical activity in small non-myelinated nerve fibres. J Physiol. 1978 Jan;274:539–548. doi: 10.1113/jphysiol.1978.sp012165. [DOI] [PMC free article] [PubMed] [Google Scholar]
- Ritchie J. M., Straub R. W. The movement of potassium ions during electrical activity, and the kinetics of the recovery process, in the non-myelinated fibres of the garfish olfactory nerve. J Physiol. 1975 Jul;249(2):327–348. doi: 10.1113/jphysiol.1975.sp011018. [DOI] [PMC free article] [PubMed] [Google Scholar]
- Ritchie J. M. The oxygen consumption of mammalian non-myelinated nerve fibres at rest and during activity. J Physiol. 1967 Feb;188(3):309–329. doi: 10.1113/jphysiol.1967.sp008141. [DOI] [PMC free article] [PubMed] [Google Scholar]
- TASAKI I., TEORELL T., SPYROPOULOS C. S. Movement of radioactive tracers across squid axon membrane. Am J Physiol. 1961 Jan;200:11–22. doi: 10.1152/ajplegacy.1961.200.1.11. [DOI] [PubMed] [Google Scholar]
- WHITTAM R., WILLIS J. S. ION MOVEMENTS AND OXYGEN CONSUMPTION IN KIDNEY CORTEX SLICES. J Physiol. 1963 Aug;168:158–177. doi: 10.1113/jphysiol.1963.sp007184. [DOI] [PMC free article] [PubMed] [Google Scholar]