Abstract
1. The lengths of the thin filaments in amphibian and mammalian cardiac muscle have been determined from electron micrographs of serial transverse sections. Thin filament lengths in frog atrial trabeculae range from 0.8 to greater than 1.3 micrometers, with a maximum possible error of 0.14--0.15 micrometer. In rat atrial tissue the span is from 0.6 to more than 1.1 micrometer, whereas in rat papillary muscle the breadth of the distribution is much narrower, from 0.9 to greater than 1.1 micrometer. Double overlap of thin filaments should, therefore, exist over a wide range of sarcomere lenghts. Thin filaments from opposite halves of a sarcomere accommodate each other by flexing up to an angle of about 2 degrees and moving from the trigonal position among the thick filaments to the centre of the region between two thick filaments. Such rearrangement probably contributes to the internal resistance to shortening in the muscle. 2. Except for the variation in thin filament lengths, the over-all morphology of the cardiac sarcomere is generally similar to that found in skeletal muscle. Thick filaments in heart muscle are uniform in length, and their profiles change along their lengths. They are generally round in the M band, triangular adjacent to the M band, round again in the overlap region, and either round or triangular near the tapered tips. The M bridges in rat cardiac tissue link neighbouring thick filaments to form a symmetric hexagonal array, whereas in the frog atrium, the M bridge connexions are incomplete and often form isolated triangular clusters. 3. Computed sarcomere length-developed tension curves were calculated using the thin filament length distributions and the assumptions basic to the sliding filament theory of muscle contraction. The curves for atrial tissue have plateau regions approximately as wide as the one-half micron variation in thin filament length. 4. Work done against the internal loads during systole may be stored as potential energy and released during diastole to produce sarcomeric re-extension.
Full text
PDF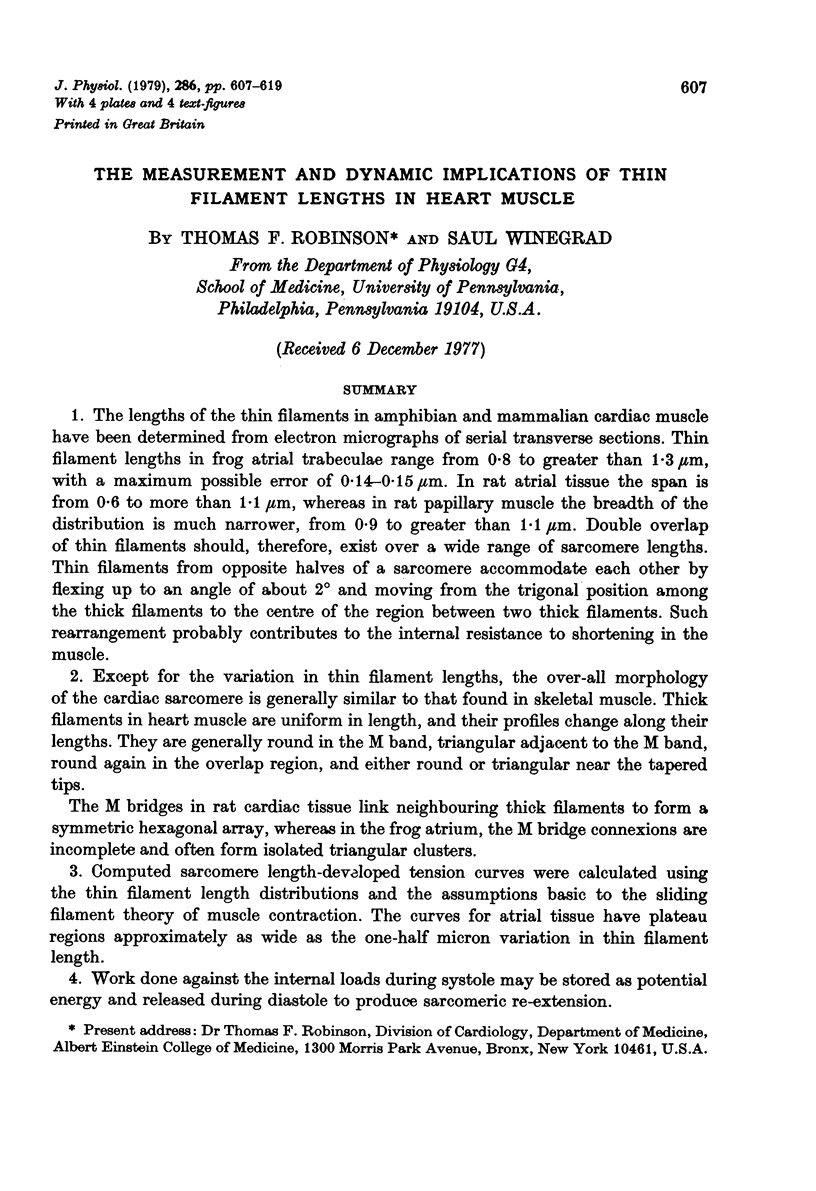
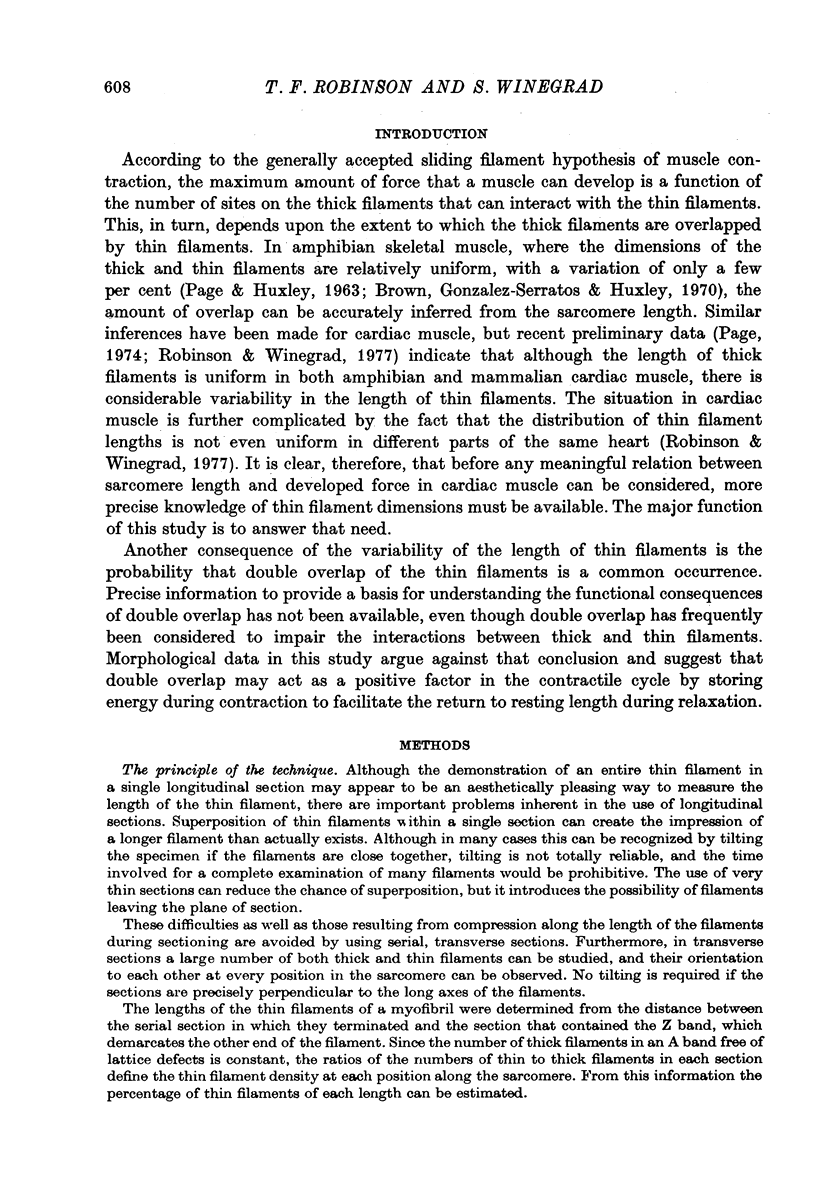
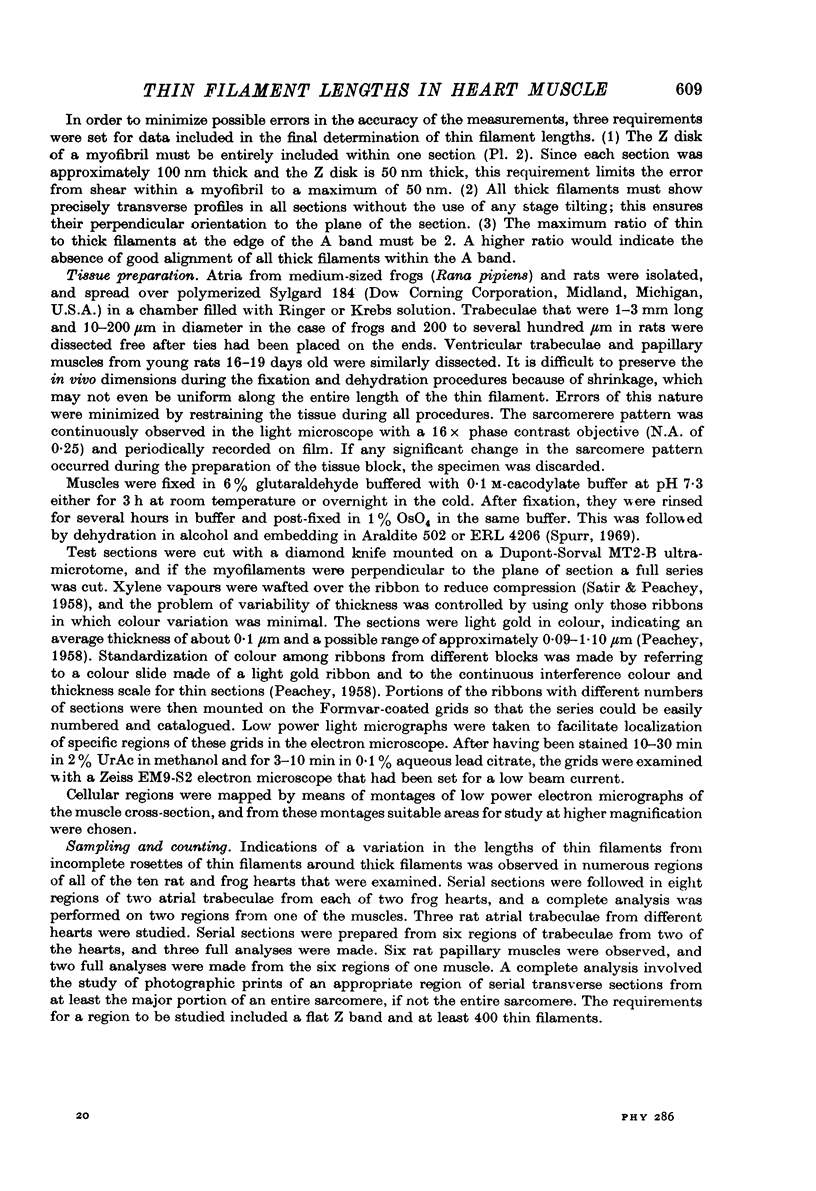
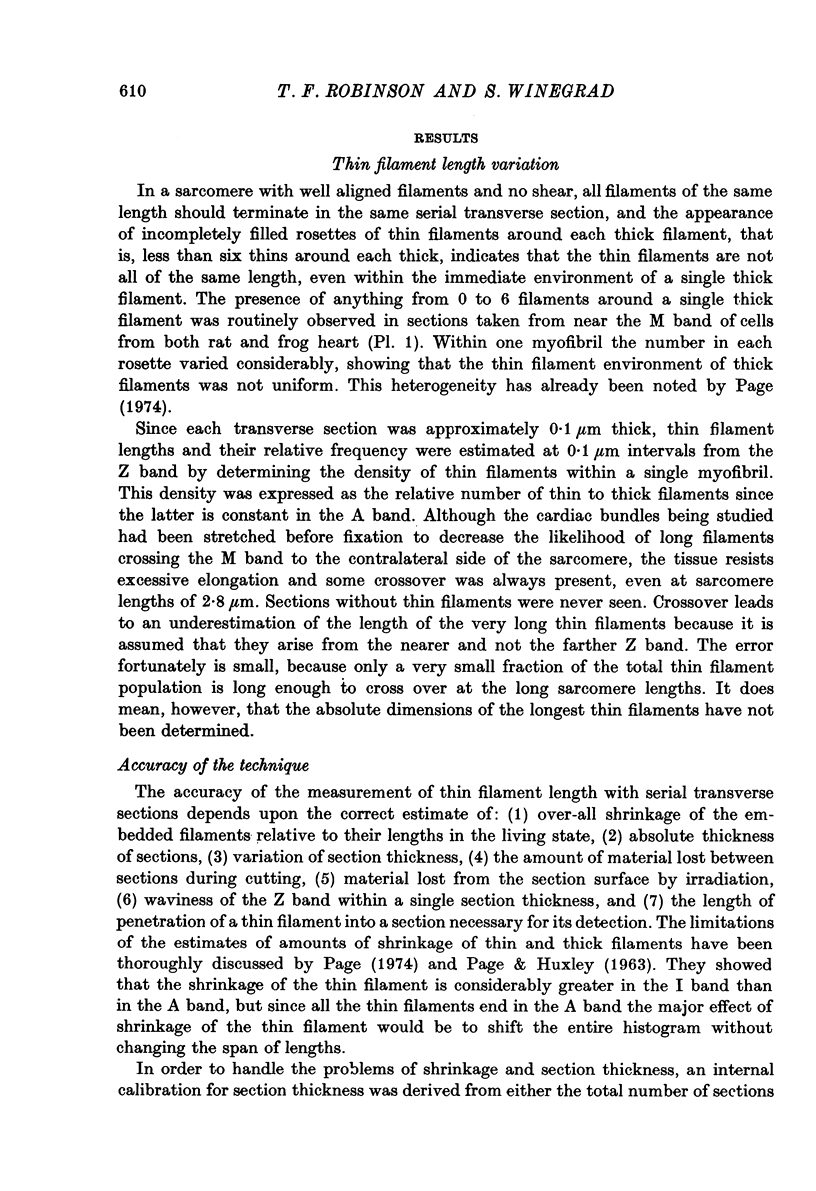
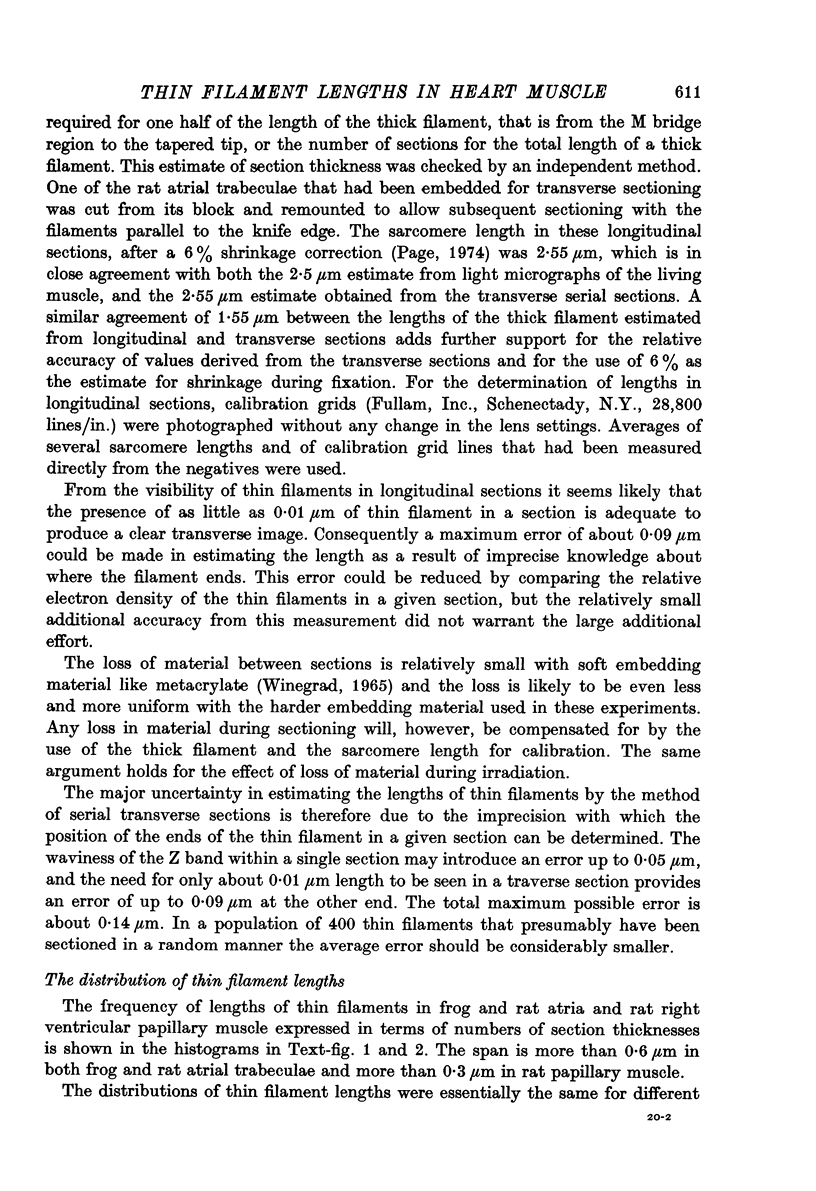
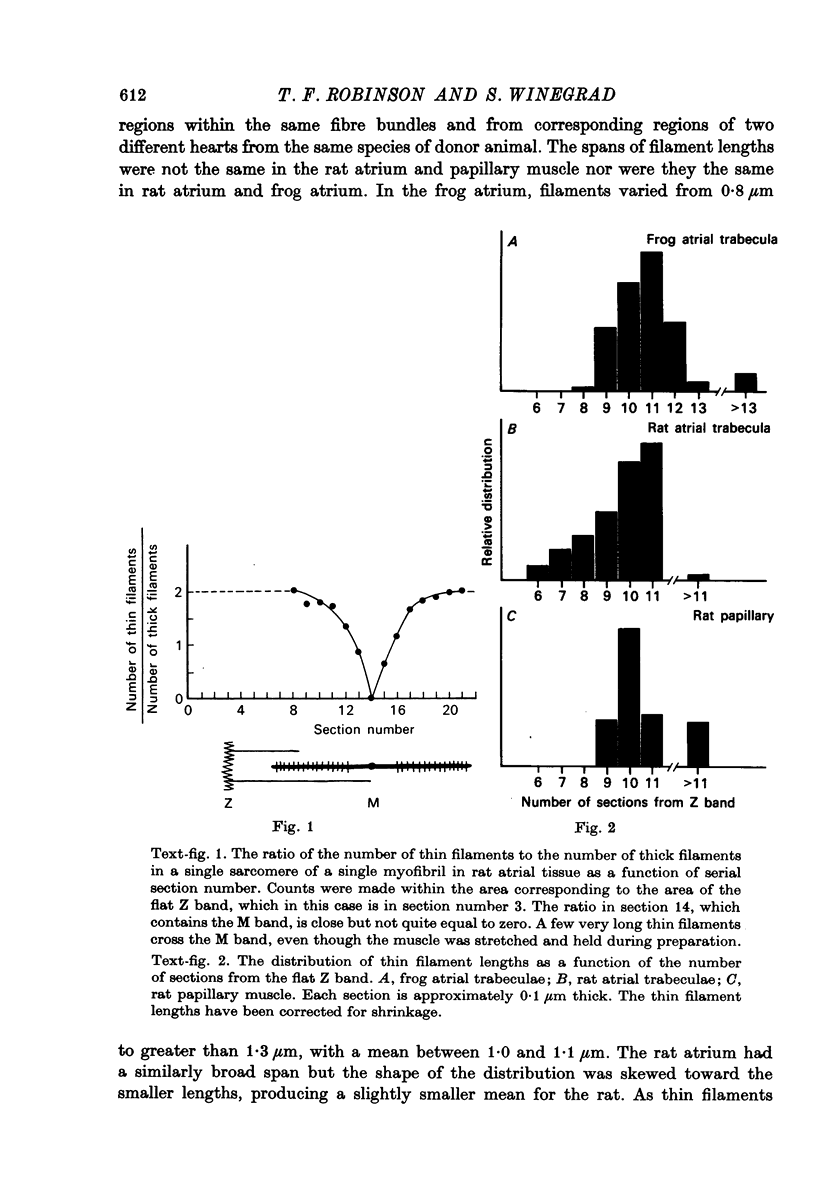
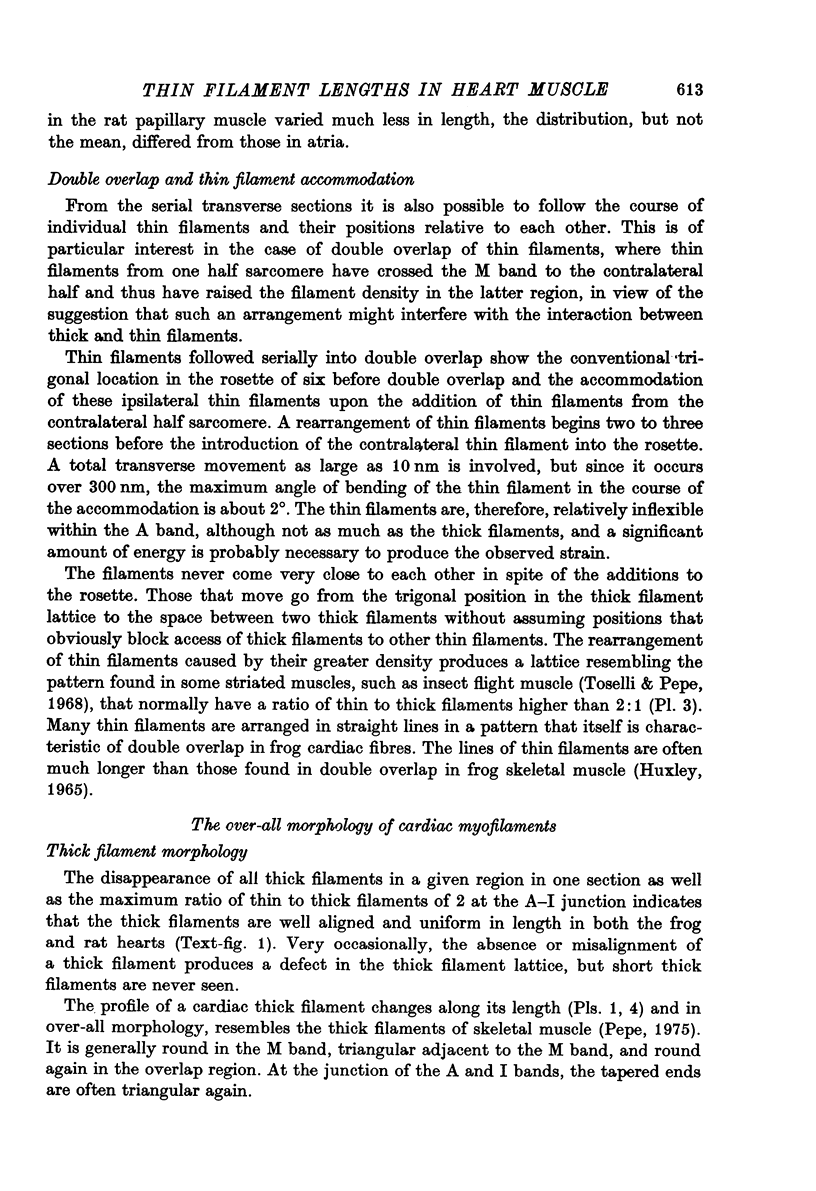
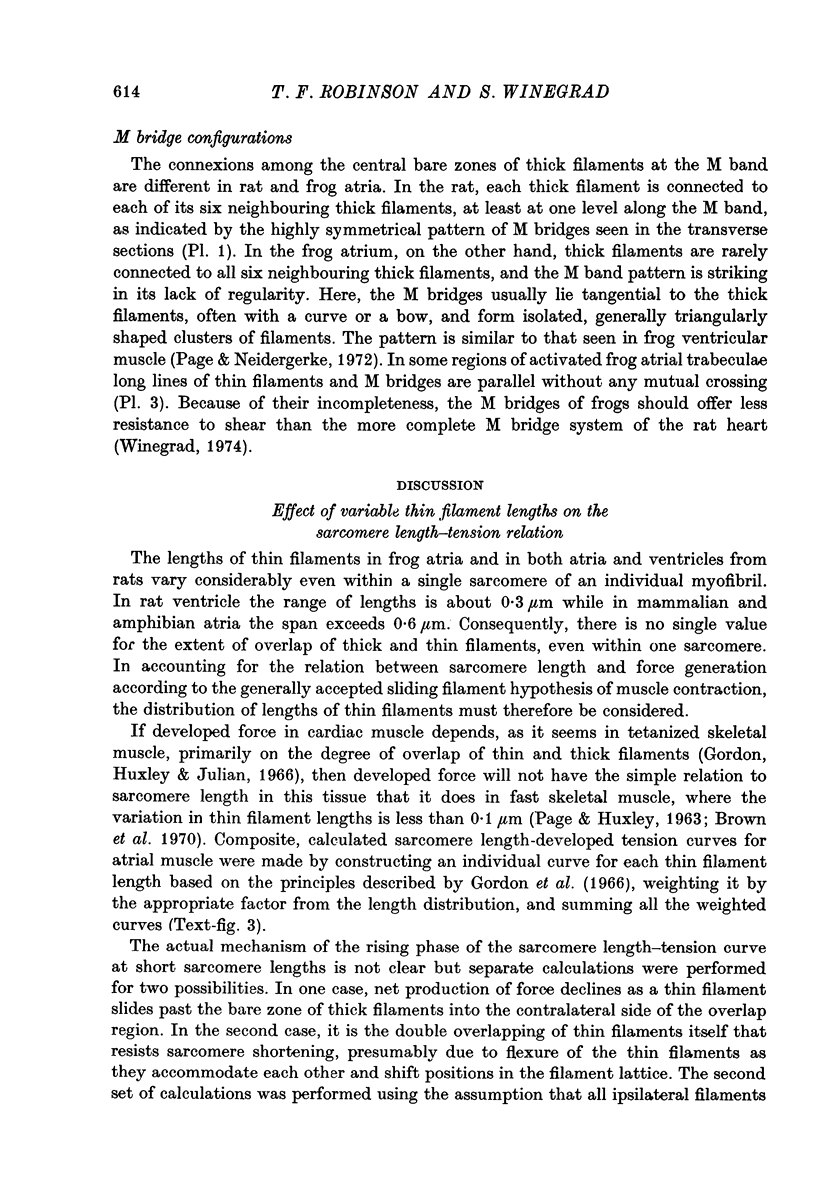
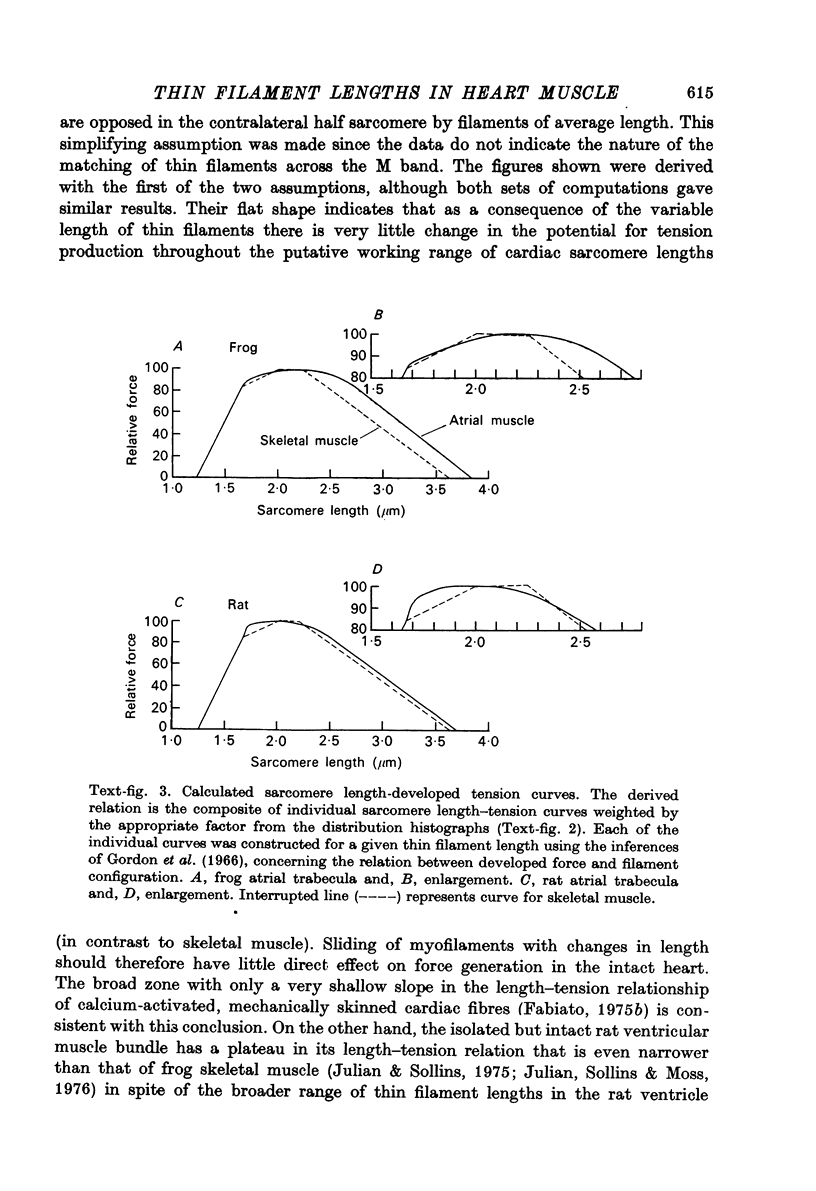
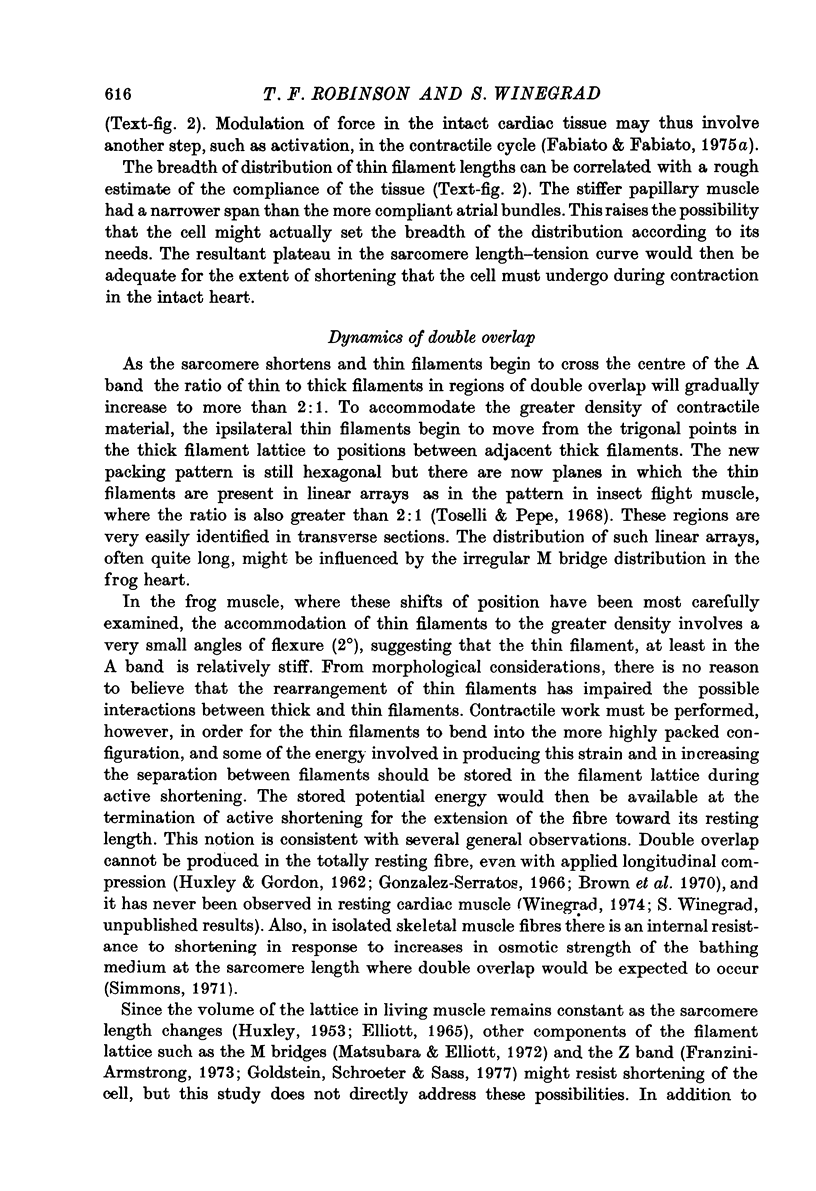
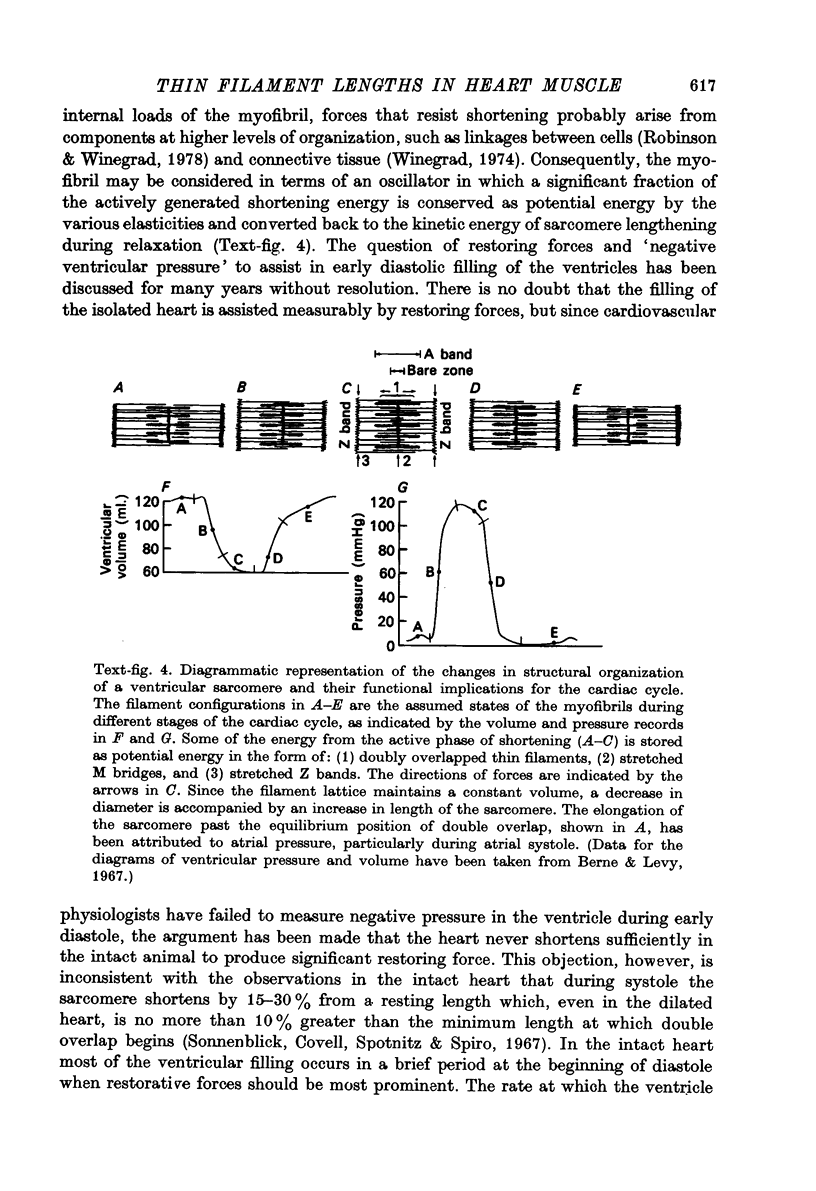
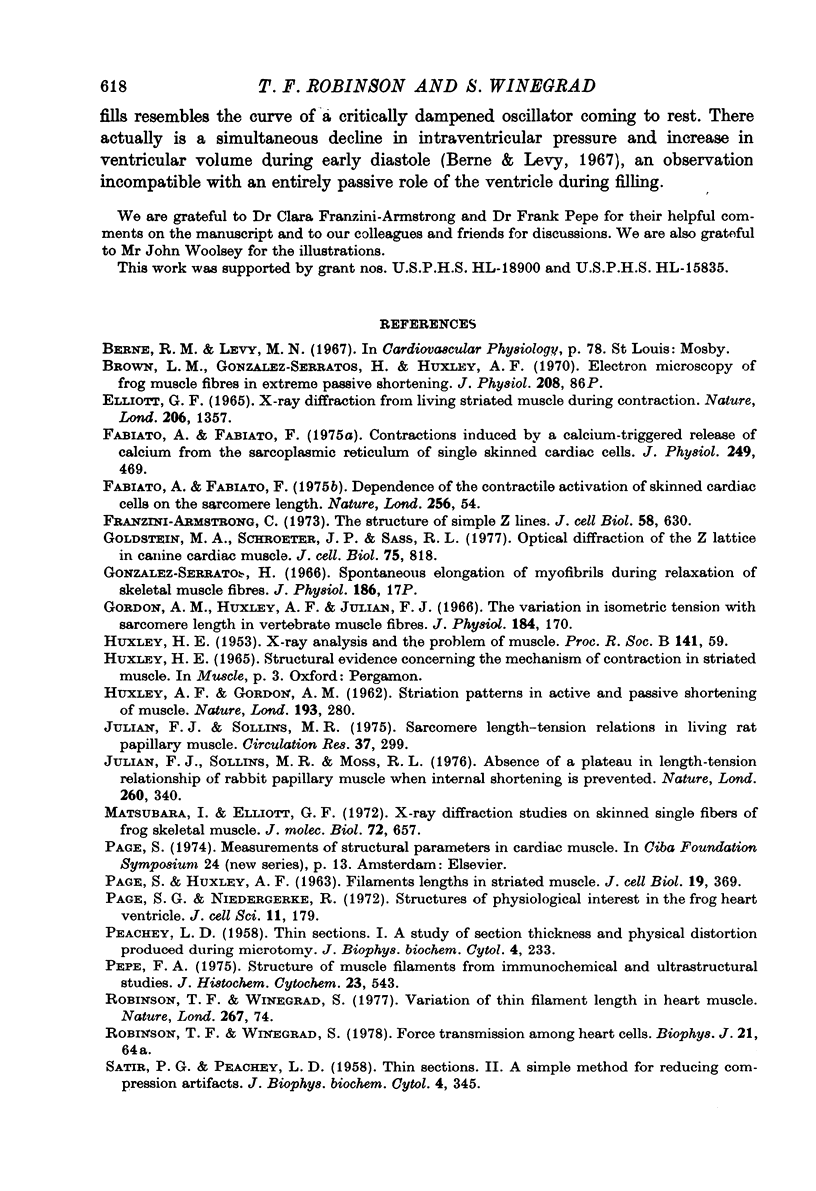
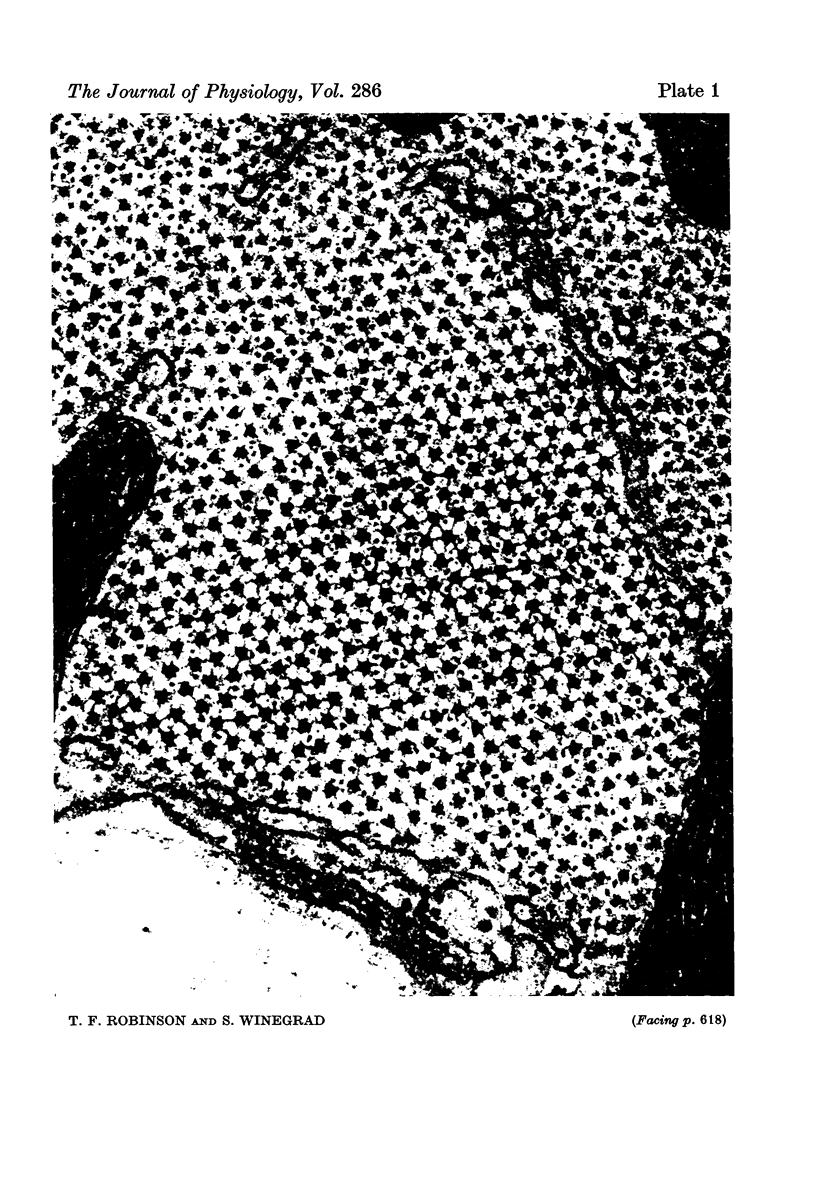
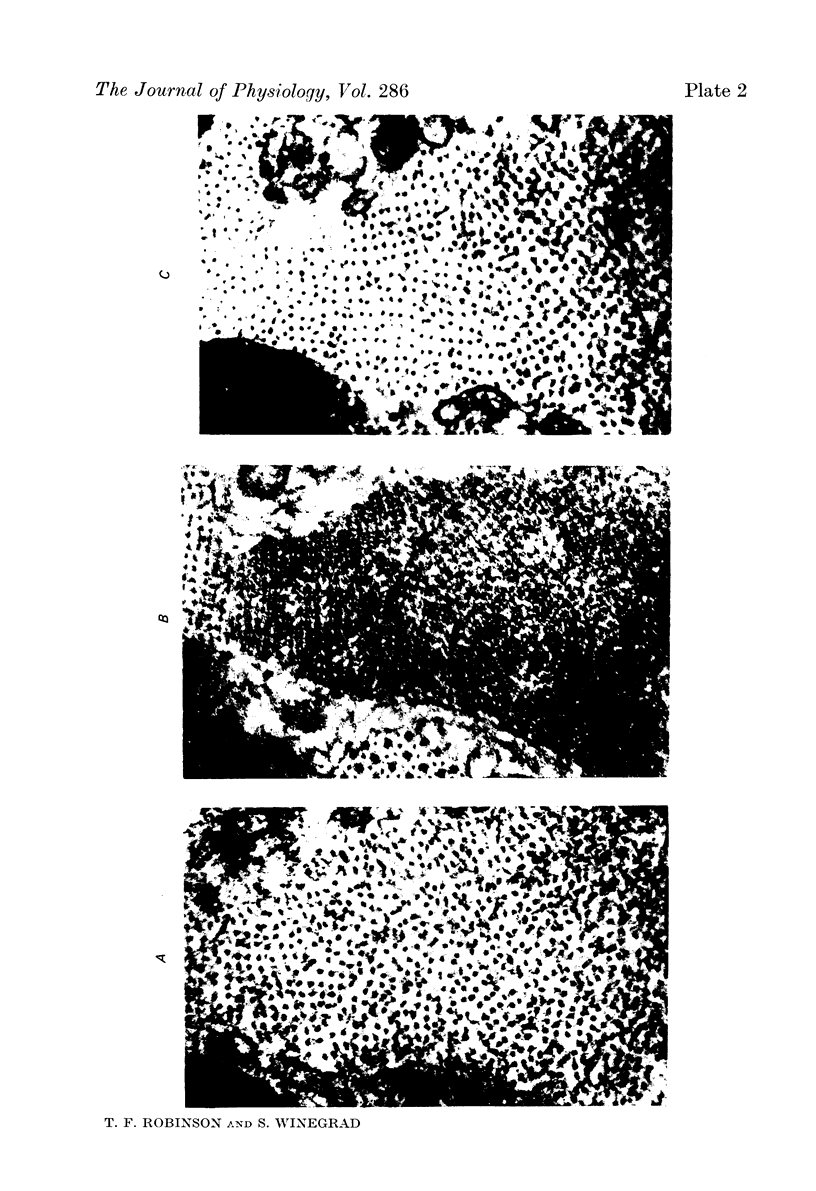
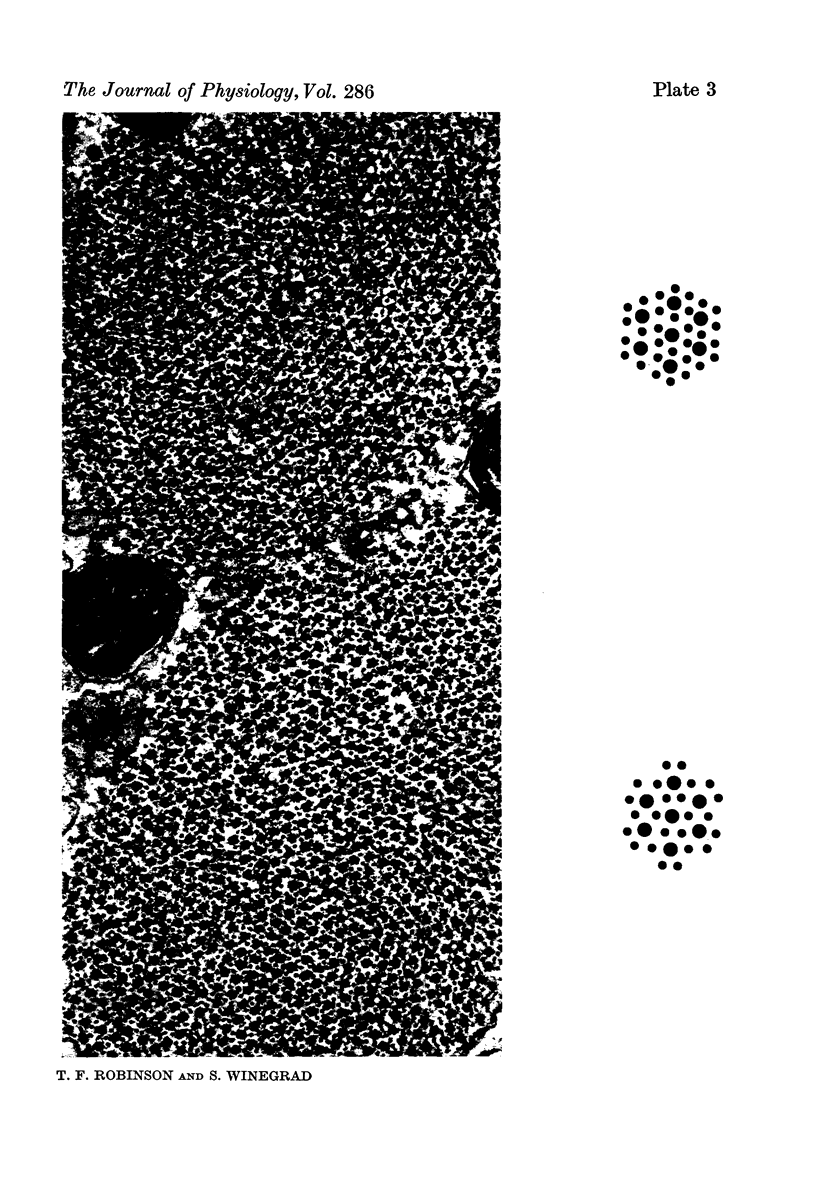
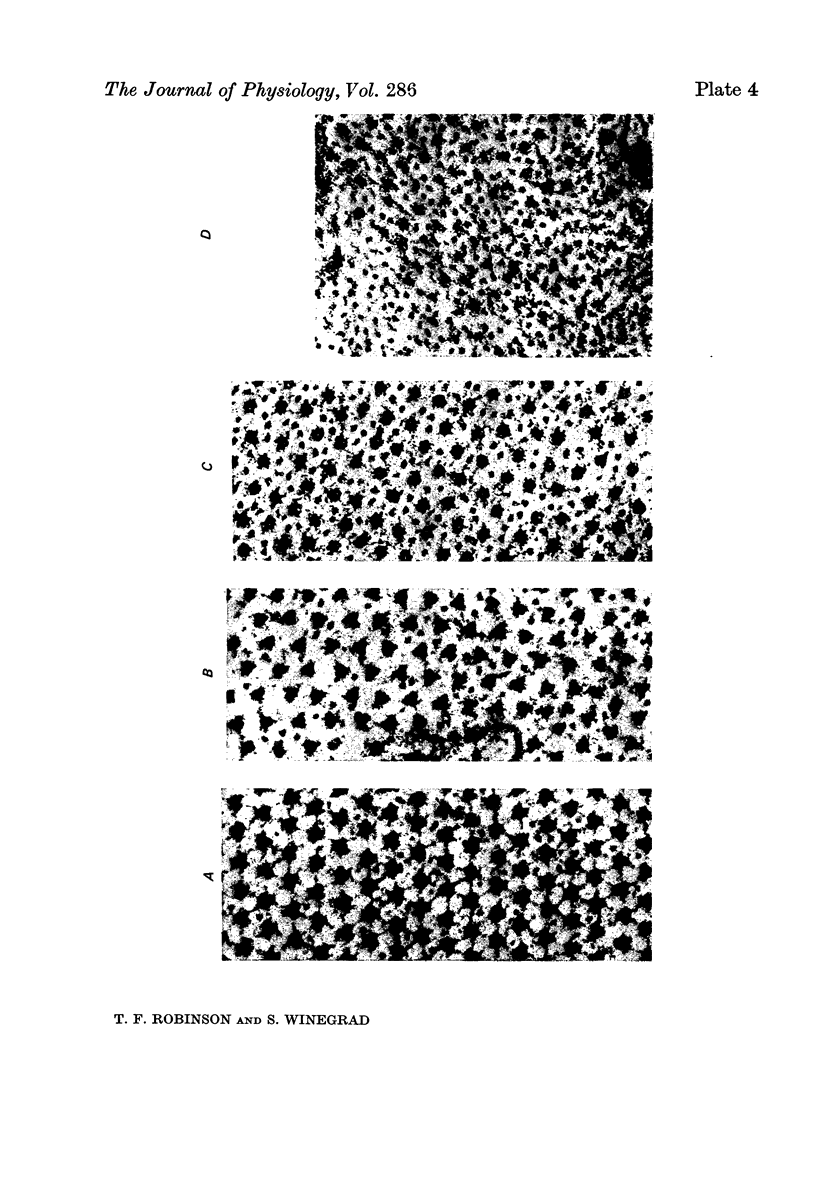
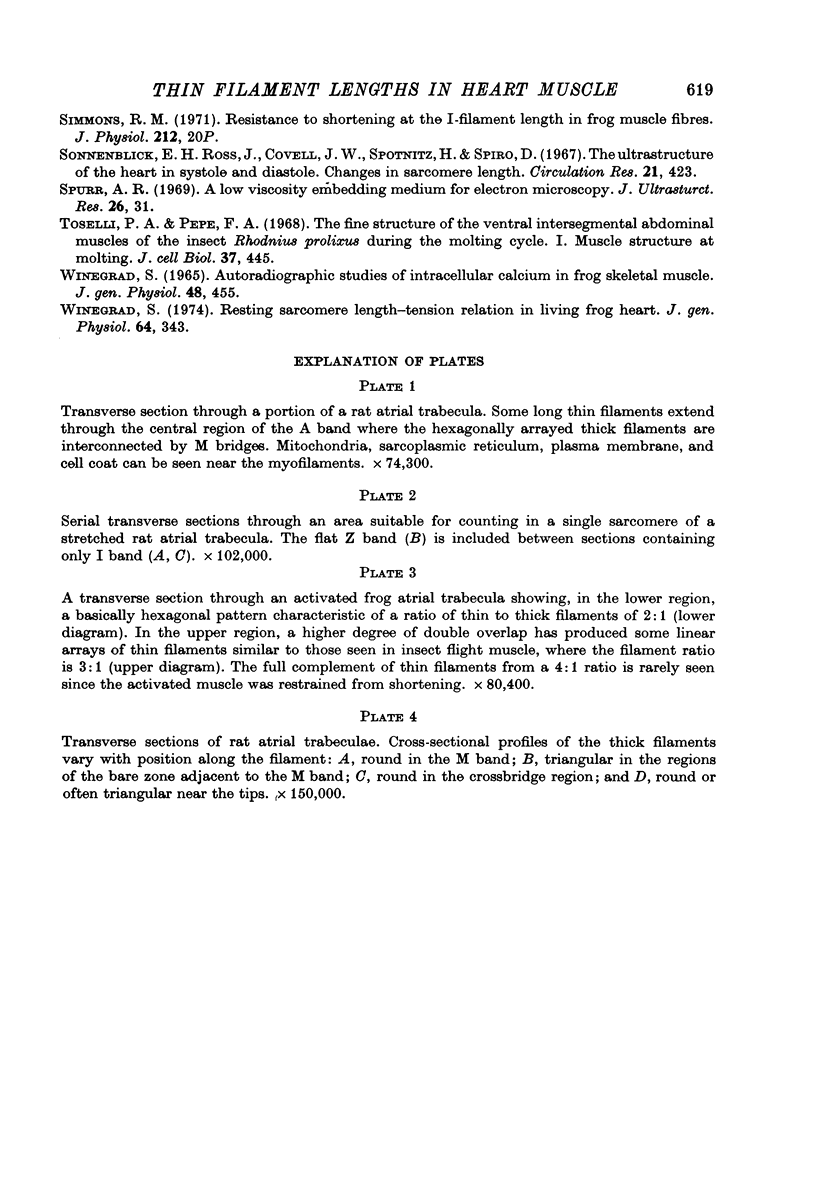
Images in this article
Selected References
These references are in PubMed. This may not be the complete list of references from this article.
- Elliott G. F., Lowy J., Millman B. M. X-ray diffraction from living striated muscle during contraction. Nature. 1965 Jun 26;206(991):1357–1358. doi: 10.1038/2061357a0. [DOI] [PubMed] [Google Scholar]
- Fabiato A., Fabiato F. Contractions induced by a calcium-triggered release of calcium from the sarcoplasmic reticulum of single skinned cardiac cells. J Physiol. 1975 Aug;249(3):469–495. doi: 10.1113/jphysiol.1975.sp011026. [DOI] [PMC free article] [PubMed] [Google Scholar]
- Fabiato A., Fabiato F. Dependence of the contractile activation of skinned cardiac cells on the sarcomere length. Nature. 1975 Jul 3;256(5512):54–56. doi: 10.1038/256054a0. [DOI] [PubMed] [Google Scholar]
- Franzini-Armstrong C. The structure of a simple Z line. J Cell Biol. 1973 Sep;58(3):630–642. doi: 10.1083/jcb.58.3.630. [DOI] [PMC free article] [PubMed] [Google Scholar]
- Goldstein M. A., Schroeter J. P., Sass R. L. Optical diffraction of the Z lattice in canine cardiac muscle. J Cell Biol. 1977 Dec;75(3):818–836. doi: 10.1083/jcb.75.3.818. [DOI] [PMC free article] [PubMed] [Google Scholar]
- Gordon A. M., Huxley A. F., Julian F. J. The variation in isometric tension with sarcomere length in vertebrate muscle fibres. J Physiol. 1966 May;184(1):170–192. doi: 10.1113/jphysiol.1966.sp007909. [DOI] [PMC free article] [PubMed] [Google Scholar]
- HUXLEY A. F., GORDON A. M. Striation patterns in active and passive shortening of muscle. Nature. 1962 Jan 20;193:280–281. doi: 10.1038/193280b0. [DOI] [PubMed] [Google Scholar]
- HUXLEY H. E. X-ray analysis and the problem of muscle. Proc R Soc Lond B Biol Sci. 1953 Mar 11;141(902):59–62. doi: 10.1098/rspb.1953.0017. [DOI] [PubMed] [Google Scholar]
- Julian F. J., Sollins M. R., Moss R. L. Absence of a plateau in length-tension relationship of rabbit papillary muscle when internal shortening is prevented. Nature. 1976 Mar 25;260(5549):340–342. doi: 10.1038/260340a0. [DOI] [PubMed] [Google Scholar]
- Julian F. J., Sollins M. R. Sarcomere length-tension relations in living rat papillary muscle. Circ Res. 1975 Sep;37(3):299–308. doi: 10.1161/01.res.37.3.299. [DOI] [PubMed] [Google Scholar]
- Matsubara I., Elliott G. F. X-ray diffraction studies on skinned single fibres of frog skeletal muscle. J Mol Biol. 1972 Dec 30;72(3):657–669. doi: 10.1016/0022-2836(72)90183-0. [DOI] [PubMed] [Google Scholar]
- PAGE S. G., HUXLEY H. E. FILAMENT LENGTHS IN STRIATED MUSCLE. J Cell Biol. 1963 Nov;19:369–390. doi: 10.1083/jcb.19.2.369. [DOI] [PMC free article] [PubMed] [Google Scholar]
- PEACHEY L. D. Thin sections. I. A study of section thickness and physical distortion produced during microtomy. J Biophys Biochem Cytol. 1958 May 25;4(3):233–242. doi: 10.1083/jcb.4.3.233. [DOI] [PMC free article] [PubMed] [Google Scholar]
- Page S. G., Niedergerke R. Structures of physiological interest in the frog heart ventricle. J Cell Sci. 1972 Jul;11(1):179–203. doi: 10.1242/jcs.11.1.179. [DOI] [PubMed] [Google Scholar]
- Pepe F. A. Structure of muscle filaments from immunohistochemical and ultrastructural studies. J Histochem Cytochem. 1975 Jul;23(7):543–562. doi: 10.1177/23.7.1095653. [DOI] [PubMed] [Google Scholar]
- Robinson T. F., Winegrad S. Variation of thin filament length in heart muscles. Nature. 1977 May 5;267(5606):74–75. doi: 10.1038/267074a0. [DOI] [PubMed] [Google Scholar]
- SATIR P. G., PEACHEY L. D. Thin sections. II. A simple method for reducing compression artifacts. J Biophys Biochem Cytol. 1958 May 25;4(3):345–348. doi: 10.1083/jcb.4.3.345. [DOI] [PMC free article] [PubMed] [Google Scholar]
- Simmons R. M. Resistance to shortening at the I-filament length in frog muscle fibres. J Physiol. 1971 Jan;212(2):20P–22P. [PubMed] [Google Scholar]
- Sonnenblick E. H., Ross J., Jr, Covell J. W., Spotnitz H. M., Spiro D. The ultrastructure of the heart in systole and diastole. Chantes in sarcomere length. Circ Res. 1967 Oct;21(4):423–431. doi: 10.1161/01.res.21.4.423. [DOI] [PubMed] [Google Scholar]
- Spurr A. R. A low-viscosity epoxy resin embedding medium for electron microscopy. J Ultrastruct Res. 1969 Jan;26(1):31–43. doi: 10.1016/s0022-5320(69)90033-1. [DOI] [PubMed] [Google Scholar]
- Toselli P. A., Pepe F. A. The fine structure of the ventral intersegmental abdominal muscles of the insect Rhodnius prolixus during the molting cycle. I. Muscle structure at molting. J Cell Biol. 1968 May;37(2):445–461. doi: 10.1083/jcb.37.2.445. [DOI] [PMC free article] [PubMed] [Google Scholar]
- WINEGRAD S. AUTORADIOGRAPHIC STUDIES OF INTRACELLULAR CALCIUM IN FROG SKELETAL MUSCLE. J Gen Physiol. 1965 Jan;48:455–479. doi: 10.1085/jgp.48.3.455. [DOI] [PMC free article] [PubMed] [Google Scholar]
- Winegrad S. Resting sarcomere length-tension relation in living frog heart. J Gen Physiol. 1974 Sep;64(3):343–355. doi: 10.1085/jgp.64.3.343. [DOI] [PMC free article] [PubMed] [Google Scholar]