Abstract
Phosphorus NMR spectroscopy was used to characterize the importance of electrostatic interactions in the lytic activity of melittin, a cationic peptide. The micellization induced by melittin has been characterized for several lipid mixtures composed of saturated phosphatidylcholine (PC) and a limited amount of charged lipid. For these systems, the thermal polymorphism is similar to the one observed for pure PC: small comicelles are stable in the gel phase and extended bilayers are formed in the liquid crystalline phase. Vesicle surface charge density influences strongly the micellization. Our results show that the presence of negatively charged lipids (phospholipid or unprotonated fatty acid) reduces the proportion of lysed vesicles. Conversely, the presence of positively charged lipids leads to a promotion of the lytic activity of the peptide. The modulation of the lytic effect is proposed to originate from the electrostatic interactions between the peptide and the bilayer surface. Attractive interactions anchor the peptide at the surface and, as a consequence, inhibit its lytic activity. Conversely, repulsive interactions favor the redistribution of melittin into the bilayer, causing enhanced lysis. A quantitative analysis of the interaction between melittin and negatively charged bilayers suggests that electroneutrality is reached at the surface, before micellization. The surface charge density of the lipid layer appears to be a determining factor for the lipid/peptide stoichiometry of the comicelles; a decrease in the lipid/peptide stoichiometry in the presence of negatively charged lipids appears to be a general consequence of the higher affinity of melittin for these membranes.
Full text
PDF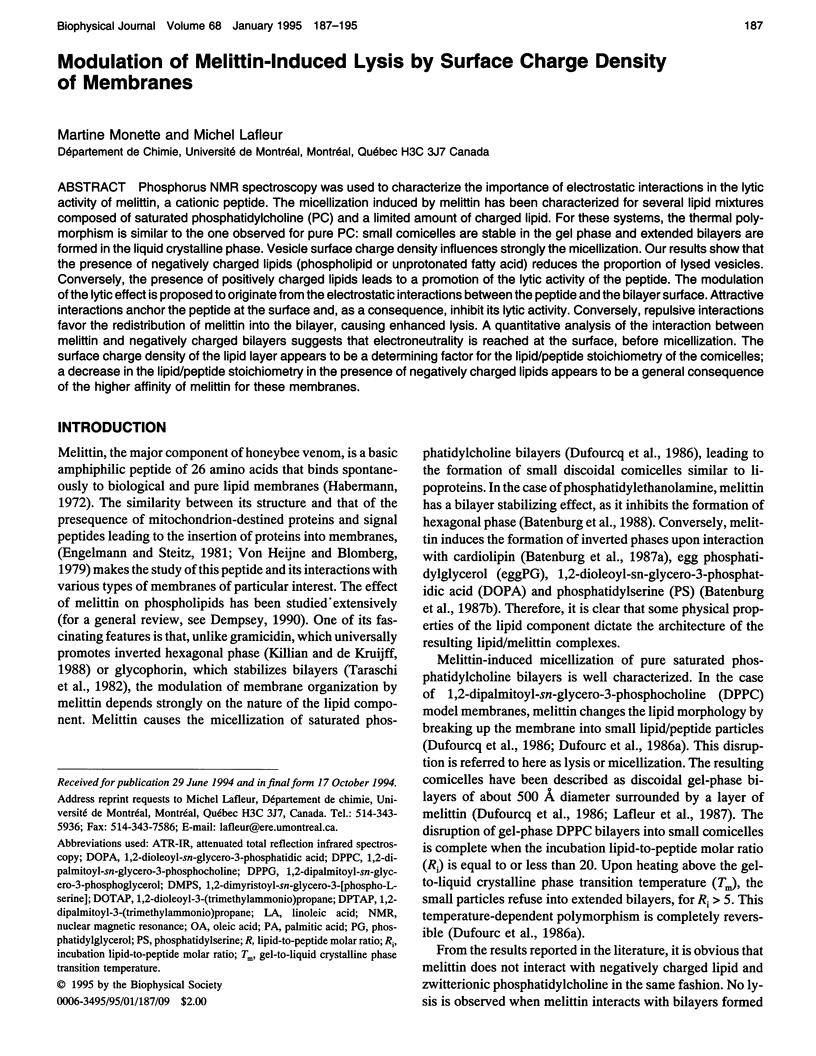
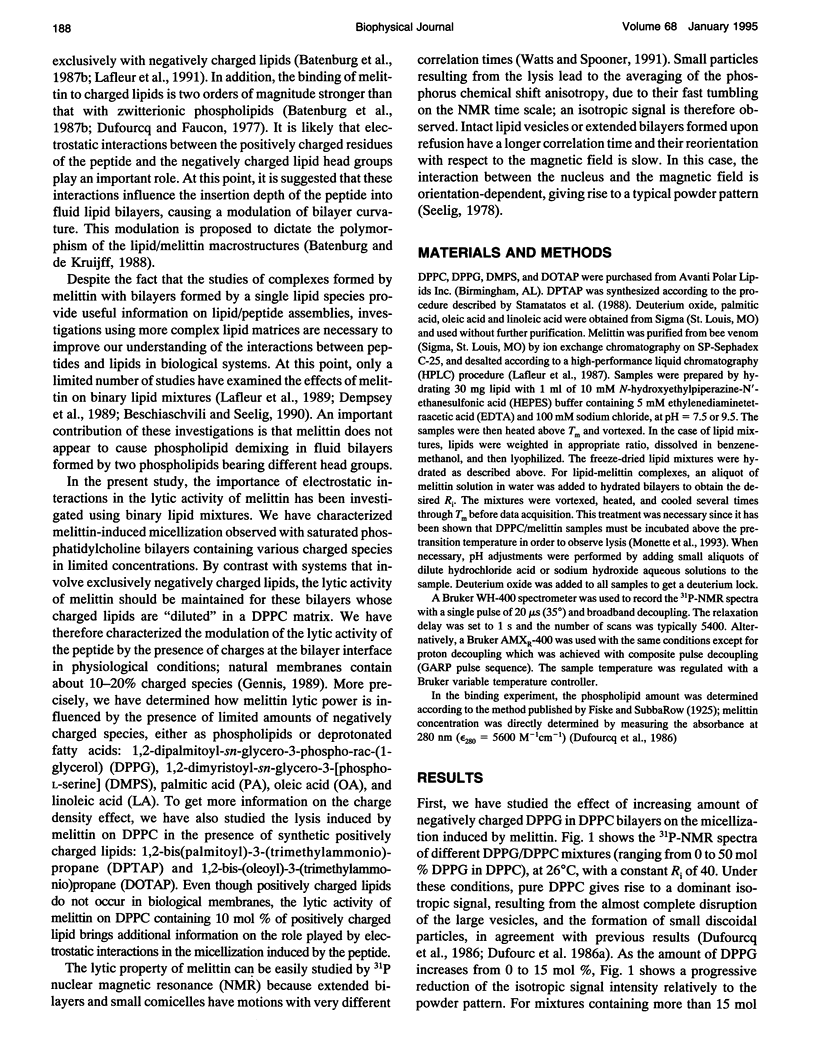
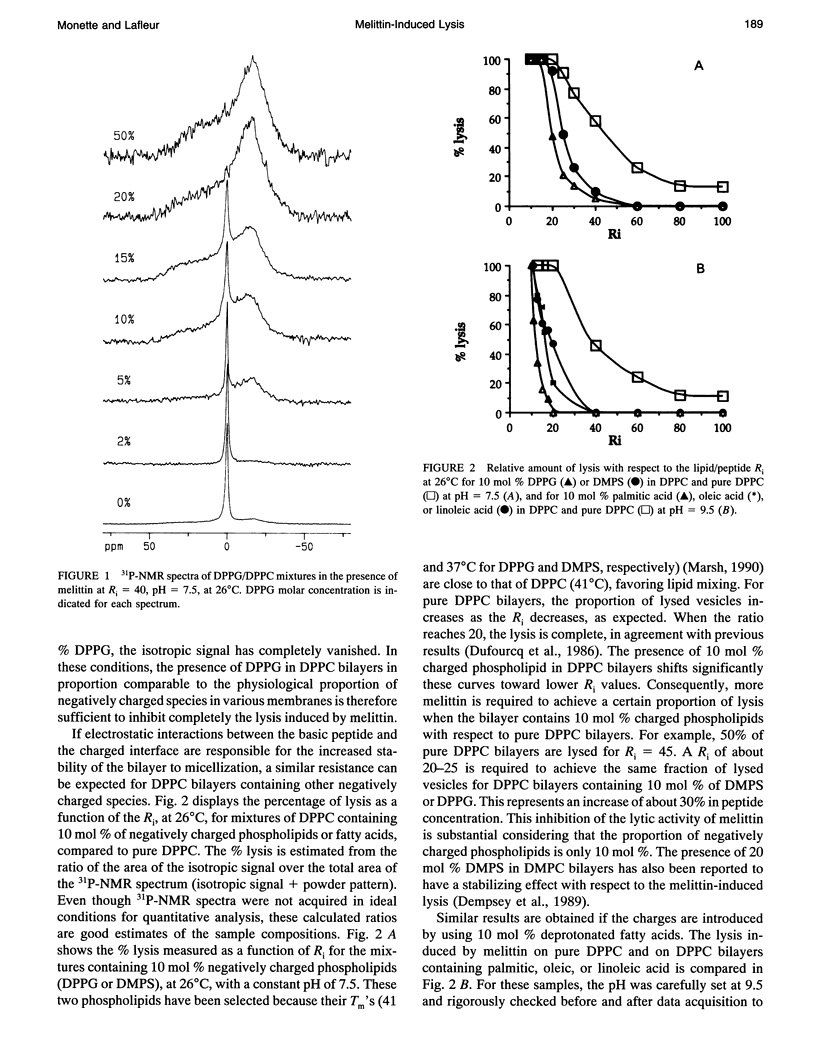
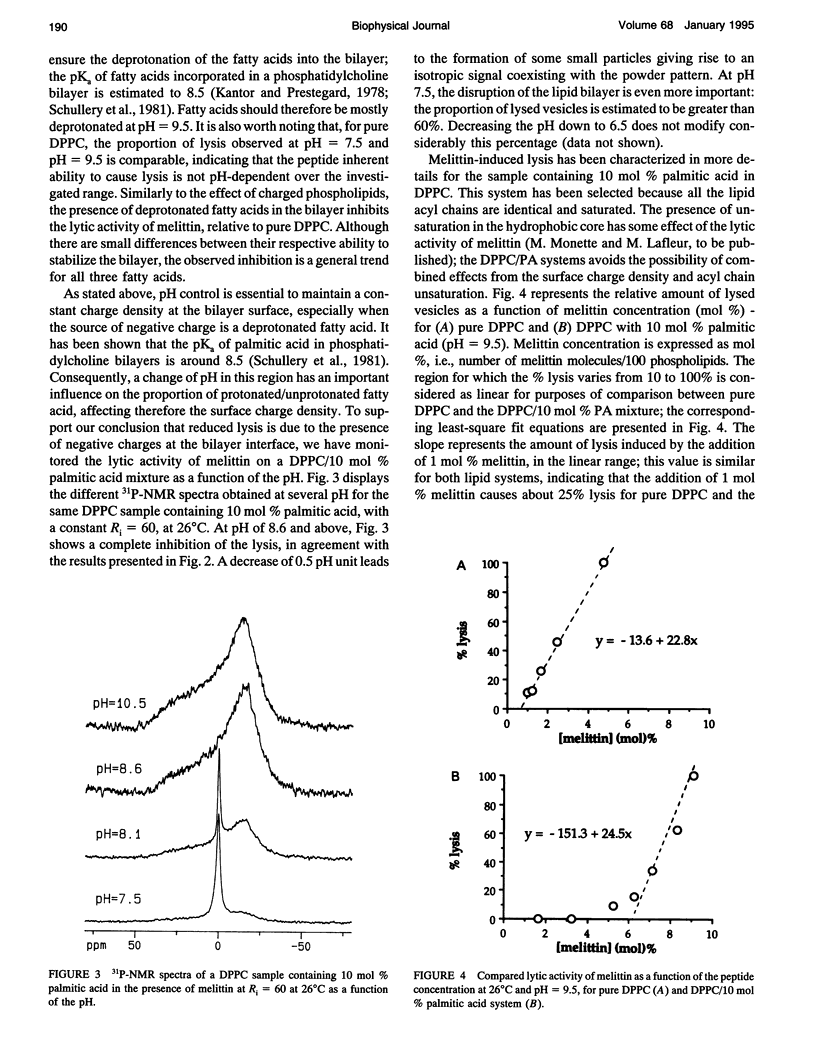
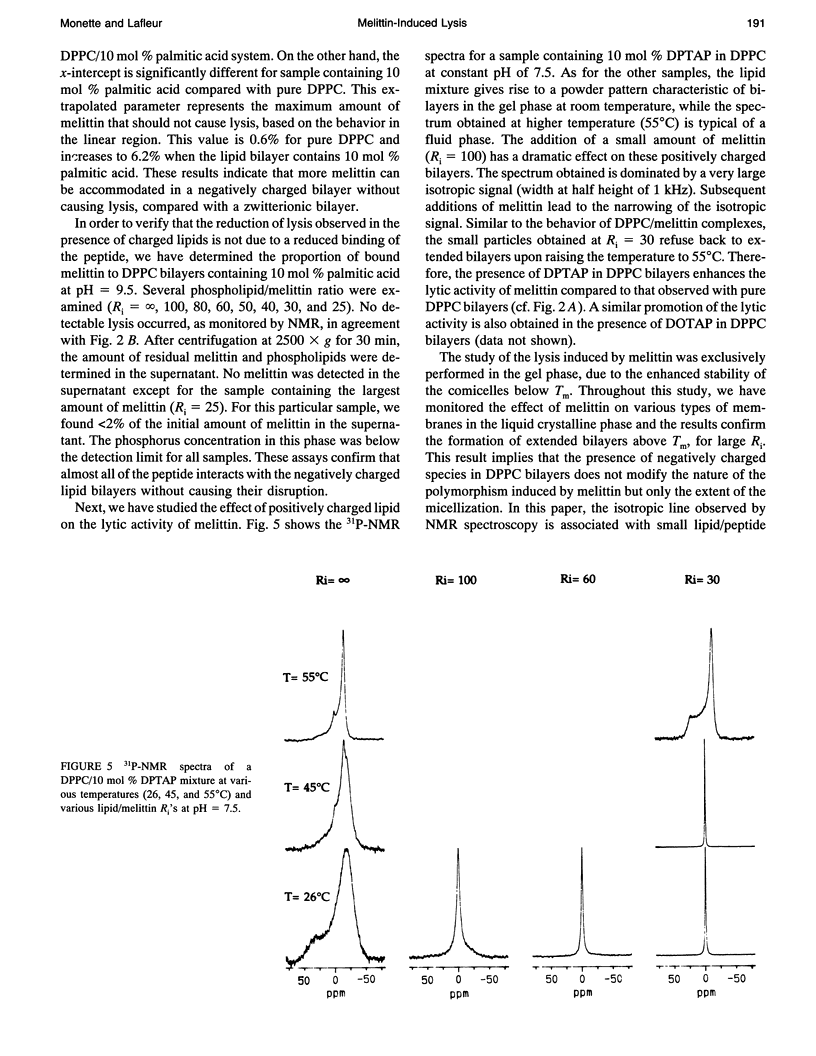
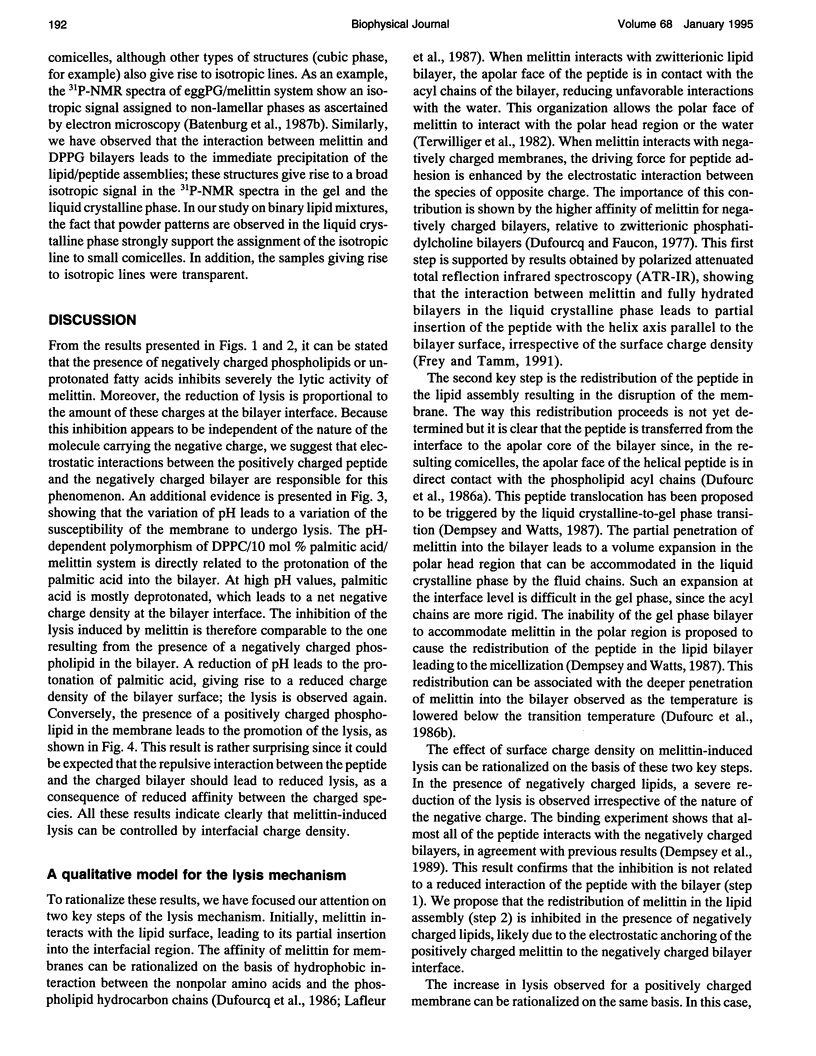
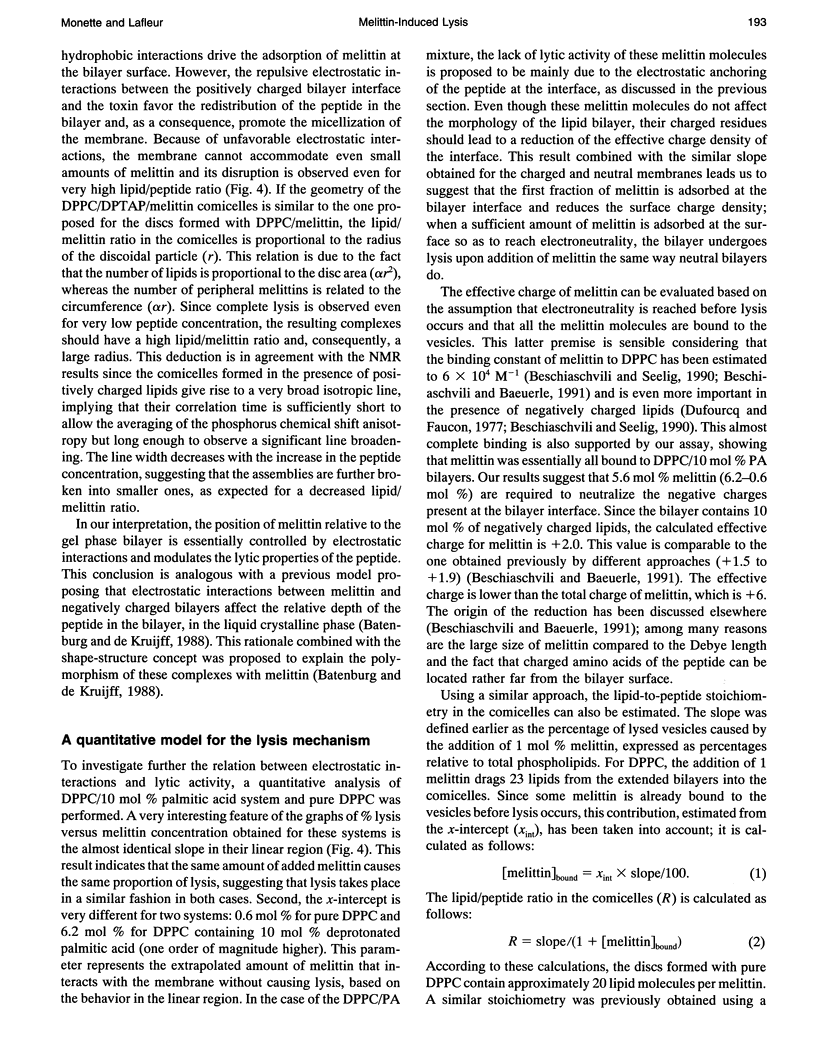
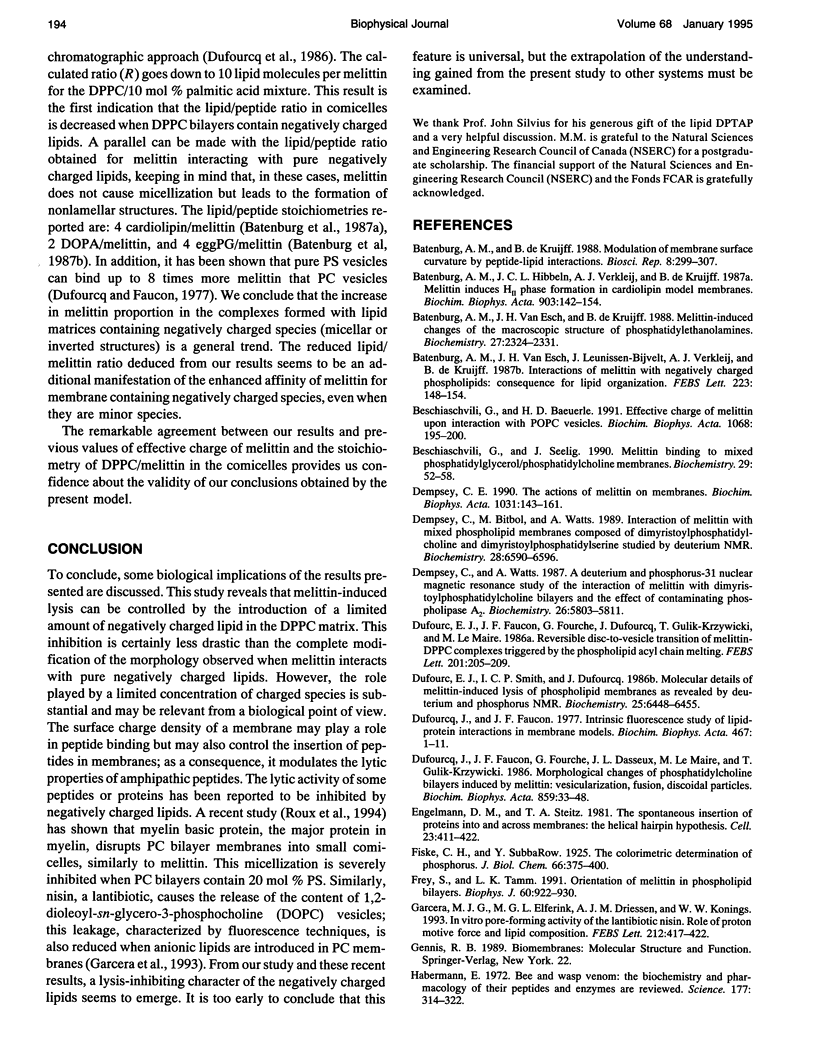
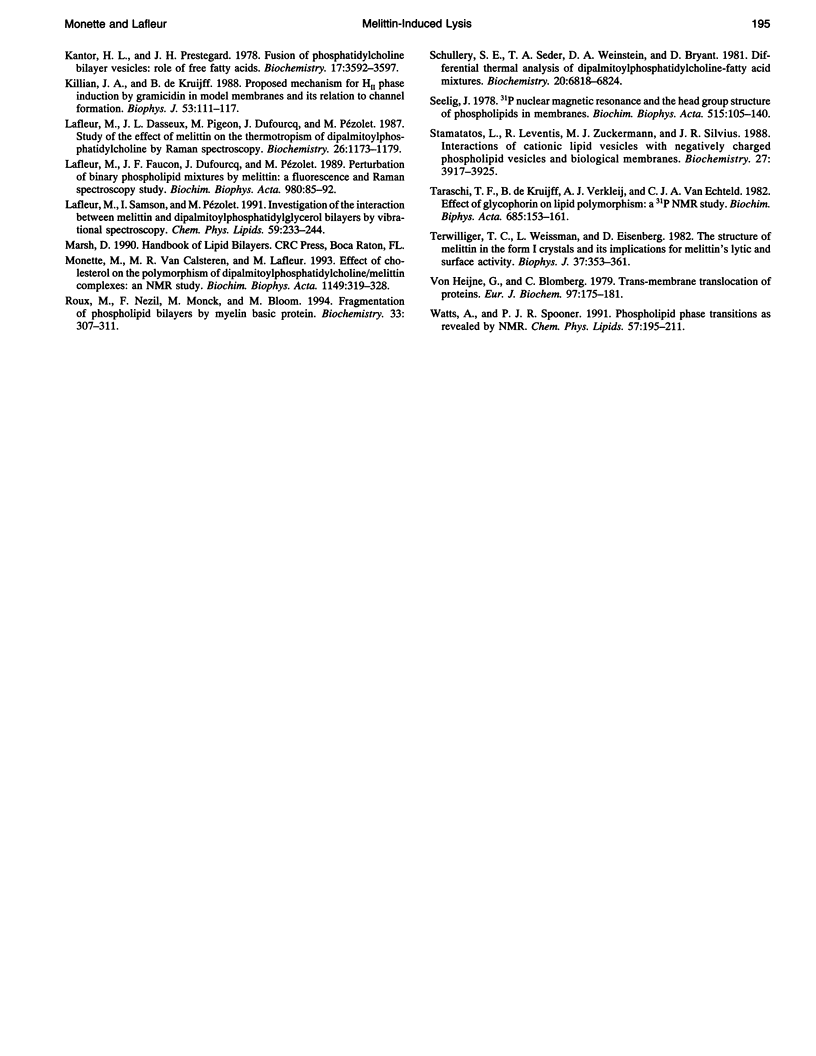
Selected References
These references are in PubMed. This may not be the complete list of references from this article.
- Batenburg A. M., Hibbeln J. C., Verkleij A. J., de Kruijff B. Melittin induces HII phase formation in cardiolipin model membranes. Biochim Biophys Acta. 1987 Sep 18;903(1):142–154. doi: 10.1016/0005-2736(87)90164-7. [DOI] [PubMed] [Google Scholar]
- Batenburg A. M., de Kruijff B. Modulation of membrane surface curvature by peptide-lipid interactions. Biosci Rep. 1988 Aug;8(4):299–307. doi: 10.1007/BF01115220. [DOI] [PubMed] [Google Scholar]
- Batenburg A. M., van Esch J. H., Leunissen-Bijvelt J., Verkleij A. J., de Kruijff B. Interaction of melittin with negatively charged phospholipids: consequences for lipid organization. FEBS Lett. 1987 Oct 19;223(1):148–154. doi: 10.1016/0014-5793(87)80526-4. [DOI] [PubMed] [Google Scholar]
- Batenburg A. M., van Esch J. H., de Kruijff B. Melittin-induced changes of the macroscopic structure of phosphatidylethanolamines. Biochemistry. 1988 Apr 5;27(7):2324–2331. doi: 10.1021/bi00407a013. [DOI] [PubMed] [Google Scholar]
- Beschiaschvili G., Baeuerle H. D. Effective charge of melittin upon interaction with POPC vesicles. Biochim Biophys Acta. 1991 Sep 30;1068(2):195–200. doi: 10.1016/0005-2736(91)90210-y. [DOI] [PubMed] [Google Scholar]
- Beschiaschvili G., Seelig J. Melittin binding to mixed phosphatidylglycerol/phosphatidylcholine membranes. Biochemistry. 1990 Jan 9;29(1):52–58. doi: 10.1021/bi00453a007. [DOI] [PubMed] [Google Scholar]
- Dempsey C. E. The actions of melittin on membranes. Biochim Biophys Acta. 1990 May 7;1031(2):143–161. doi: 10.1016/0304-4157(90)90006-x. [DOI] [PubMed] [Google Scholar]
- Dempsey C. E., Watts A. A deuterium and phosphorus-31 nuclear magnetic resonance study of the interaction of melittin with dimyristoylphosphatidylcholine bilayers and the effects of contaminating phospholipase A2. Biochemistry. 1987 Sep 8;26(18):5803–5811. doi: 10.1021/bi00392a033. [DOI] [PubMed] [Google Scholar]
- Dufourc E. J., Smith I. C., Dufourcq J. Molecular details of melittin-induced lysis of phospholipid membranes as revealed by deuterium and phosphorus NMR. Biochemistry. 1986 Oct 21;25(21):6448–6455. doi: 10.1021/bi00369a016. [DOI] [PubMed] [Google Scholar]
- Dufourcq J., Faucon J. F., Fourche G., Dasseux J. L., Le Maire M., Gulik-Krzywicki T. Morphological changes of phosphatidylcholine bilayers induced by melittin: vesicularization, fusion, discoidal particles. Biochim Biophys Acta. 1986 Jul 10;859(1):33–48. doi: 10.1016/0005-2736(86)90315-9. [DOI] [PubMed] [Google Scholar]
- Dufourcq J., Faucon J. F. Intrinsic fluorescence study of lipid-protein interactions in membrane models. Binding of melittin, an amphipathic peptide, to phospholipid vesicles. Biochim Biophys Acta. 1977 May 16;467(1):1–11. doi: 10.1016/0005-2736(77)90236-x. [DOI] [PubMed] [Google Scholar]
- Engelman D. M., Steitz T. A. The spontaneous insertion of proteins into and across membranes: the helical hairpin hypothesis. Cell. 1981 Feb;23(2):411–422. doi: 10.1016/0092-8674(81)90136-7. [DOI] [PubMed] [Google Scholar]
- Frey S., Tamm L. K. Orientation of melittin in phospholipid bilayers. A polarized attenuated total reflection infrared study. Biophys J. 1991 Oct;60(4):922–930. doi: 10.1016/S0006-3495(91)82126-9. [DOI] [PMC free article] [PubMed] [Google Scholar]
- Garcerá M. J., Elferink M. G., Driessen A. J., Konings W. N. In vitro pore-forming activity of the lantibiotic nisin. Role of protonmotive force and lipid composition. Eur J Biochem. 1993 Mar 1;212(2):417–422. doi: 10.1111/j.1432-1033.1993.tb17677.x. [DOI] [PubMed] [Google Scholar]
- Habermann E. Bee and wasp venoms. Science. 1972 Jul 28;177(4046):314–322. doi: 10.1126/science.177.4046.314. [DOI] [PubMed] [Google Scholar]
- Kantor H. L., Prestegard J. H. Fusion of phosphatidylcholine bilayer vesicles: role of free fatty acid. Biochemistry. 1978 Aug 22;17(17):3592–3597. doi: 10.1021/bi00610a027. [DOI] [PubMed] [Google Scholar]
- Killian J. A., de Kruijff B. Proposed Mechanism for H(II) Phase Induction by Gramicidin in Model Membranes and Its Relation to Channel Formation. Biophys J. 1988 Jan;53(1):111–117. doi: 10.1016/s0006-3495(88)83072-8. [DOI] [PMC free article] [PubMed] [Google Scholar]
- Lafleur M., Dasseux J. L., Pigeon M., Dufourcq J., Pézolet M. Study of the effect of melittin on the thermotropism of dipalmitoylphosphatidylcholine by Raman spectroscopy. Biochemistry. 1987 Feb 24;26(4):1173–1179. doi: 10.1021/bi00378a027. [DOI] [PubMed] [Google Scholar]
- Lafleur M., Faucon J. F., Dufourcq J., Pézolet M. Perturbation of binary phospholipid mixtures by melittin: a fluorescence and raman spectroscopy study. Biochim Biophys Acta. 1989 Mar 27;980(1):85–92. doi: 10.1016/0005-2736(89)90203-4. [DOI] [PubMed] [Google Scholar]
- Lafleur M., Samson I., Pézolet M. Investigation of the interaction between melittin and dipalmitoylphosphatidylglycerol bilayers by vibrational spectroscopy. Chem Phys Lipids. 1991 Oct;59(3):233–244. doi: 10.1016/0009-3084(91)90023-5. [DOI] [PubMed] [Google Scholar]
- Monette M., Van Calsteren M. R., Lafleur M. Effect of cholesterol on the polymorphism of dipalmitoylphosphatidylcholine/melittin complexes: an NMR study. Biochim Biophys Acta. 1993 Jul 4;1149(2):319–328. doi: 10.1016/0005-2736(93)90217-n. [DOI] [PubMed] [Google Scholar]
- Roux M., Nezil F. A., Monck M., Bloom M. Fragmentation of phospholipid bilayers by myelin basic protein. Biochemistry. 1994 Jan 11;33(1):307–311. doi: 10.1021/bi00167a040. [DOI] [PubMed] [Google Scholar]
- Schullery S. E., Seder T. A., Weinstein D. A., Bryant D. A. Differential thermal analysis of dipalmitoylphosphatidylcholine--fatty acid mixtures. Biochemistry. 1981 Nov 24;20(24):6818–6824. doi: 10.1021/bi00527a012. [DOI] [PubMed] [Google Scholar]
- Seelig J. 31P nuclear magnetic resonance and the head group structure of phospholipids in membranes. Biochim Biophys Acta. 1978 Jul 31;515(2):105–140. doi: 10.1016/0304-4157(78)90001-1. [DOI] [PubMed] [Google Scholar]
- Stamatatos L., Leventis R., Zuckermann M. J., Silvius J. R. Interactions of cationic lipid vesicles with negatively charged phospholipid vesicles and biological membranes. Biochemistry. 1988 May 31;27(11):3917–3925. doi: 10.1021/bi00411a005. [DOI] [PubMed] [Google Scholar]
- Taraschi T. F., De Kruijff B., Verkleij A., Van Echteld C. J. Effect of glycophorin on lipid polymorphism. A 31P-NMR study. Biochim Biophys Acta. 1982 Feb 23;685(2):153–161. doi: 10.1016/0005-2736(82)90092-x. [DOI] [PubMed] [Google Scholar]
- Terwilliger T. C., Weissman L., Eisenberg D. The structure of melittin in the form I crystals and its implication for melittin's lytic and surface activities. Biophys J. 1982 Jan;37(1):353–361. doi: 10.1016/S0006-3495(82)84683-3. [DOI] [PMC free article] [PubMed] [Google Scholar]
- Watts A., Spooner P. J. Phospholipid phase transitions as revealed by NMR. Chem Phys Lipids. 1991 Mar;57(2-3):195–211. doi: 10.1016/0009-3084(91)90076-n. [DOI] [PubMed] [Google Scholar]
- von Heijne G., Blomberg C. Trans-membrane translocation of proteins. The direct transfer model. Eur J Biochem. 1979 Jun;97(1):175–181. doi: 10.1111/j.1432-1033.1979.tb13100.x. [DOI] [PubMed] [Google Scholar]