Abstract
Recent studies have demonstrated a relationship between the activity of the Ca-ATPase of sarcoplasmic reticulum and its state of self-association. In the present study, the effects of thapsigargin (TG), a toxin that specifically inhibits the Ca-ATPase of rabbit skeletal muscle sarcoplasmic reticulum membrane, were studied by detecting the time-resolved phosphorescence anisotropy (TPA) decay of the Ca-ATPase that had been labeled with the phosphorescent probe erythrosin-isothiocyanate (ErITC). Anisotropy decays were fit to a function that consisted of three exponential decays plus a constant background, as well as to a function describing explicitly the uniaxial rotation of proteins in a membrane. In the absence of TG, the anisotropy was best-fit by a model representing the rotation of three populations, corresponding to different-sized oligomeric species in the membrane. The addition of stoichiometric amounts of TG to the Ca-ATPase promptly decreased the overall apparent rate of decay, indicating decreased rotational mobility. A detailed analysis showed that the principal change was not in the rates of rotation but rather in the population distribution of the Ca-ATPase molecules among the different-sized oligomers. TG decreased the proportion of small oligomers and increased the proportion of large ones. Preincubation of the ErITC-SR in 1 mM Ca2+, which stabilizes the E1 conformation relative to E2, was found to protect partially against the changes in the TPA associated with the presence of the inhibitor. These results are consistent with the hypothesis that TG inhibits the Ca-ATPase by stabilizing it in an E2-like conformation, which promotes the formation of larger aggregates of the enzyme. When combined with the effects of other inhibitors on the Ca-ATPase, these results support a general model for the coupling of enzyme conformation and self-association in this system.
Full text
PDF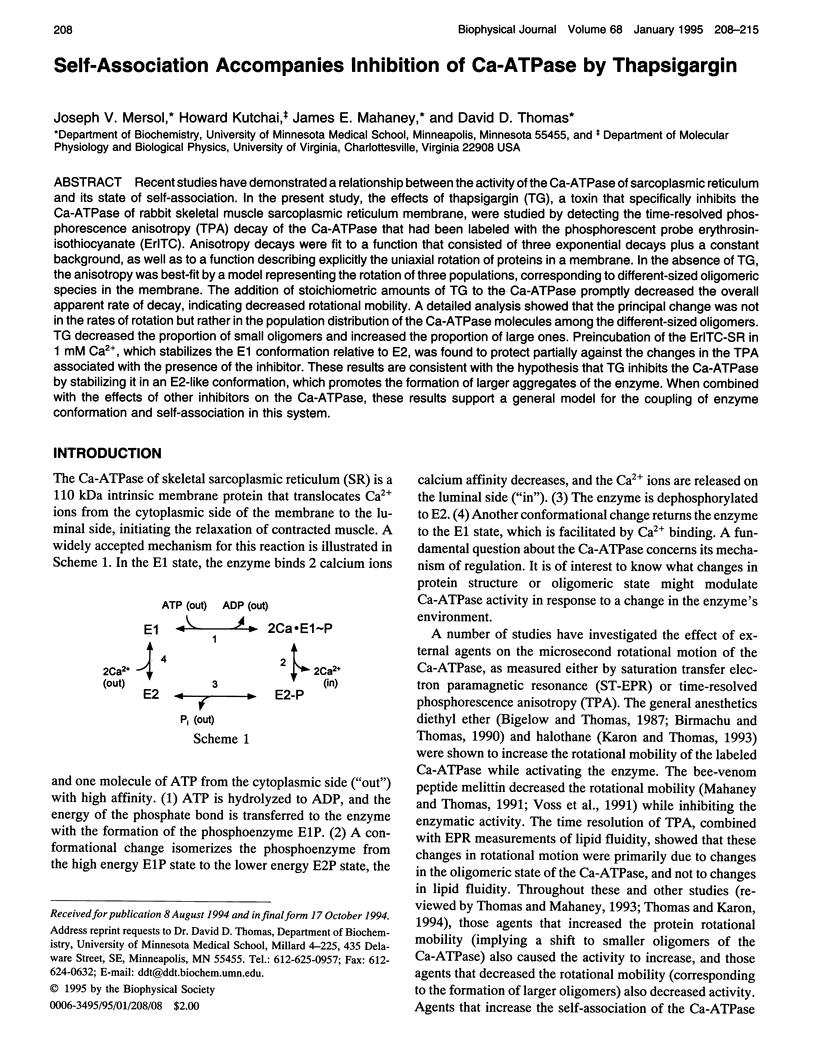
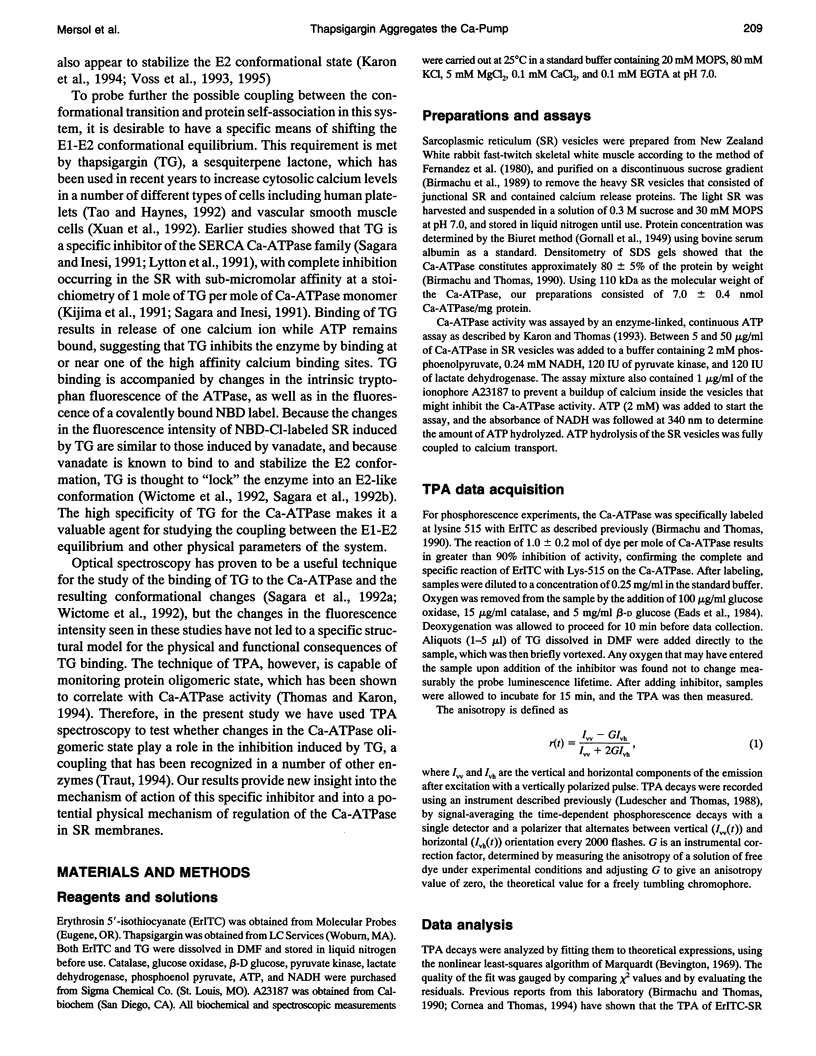
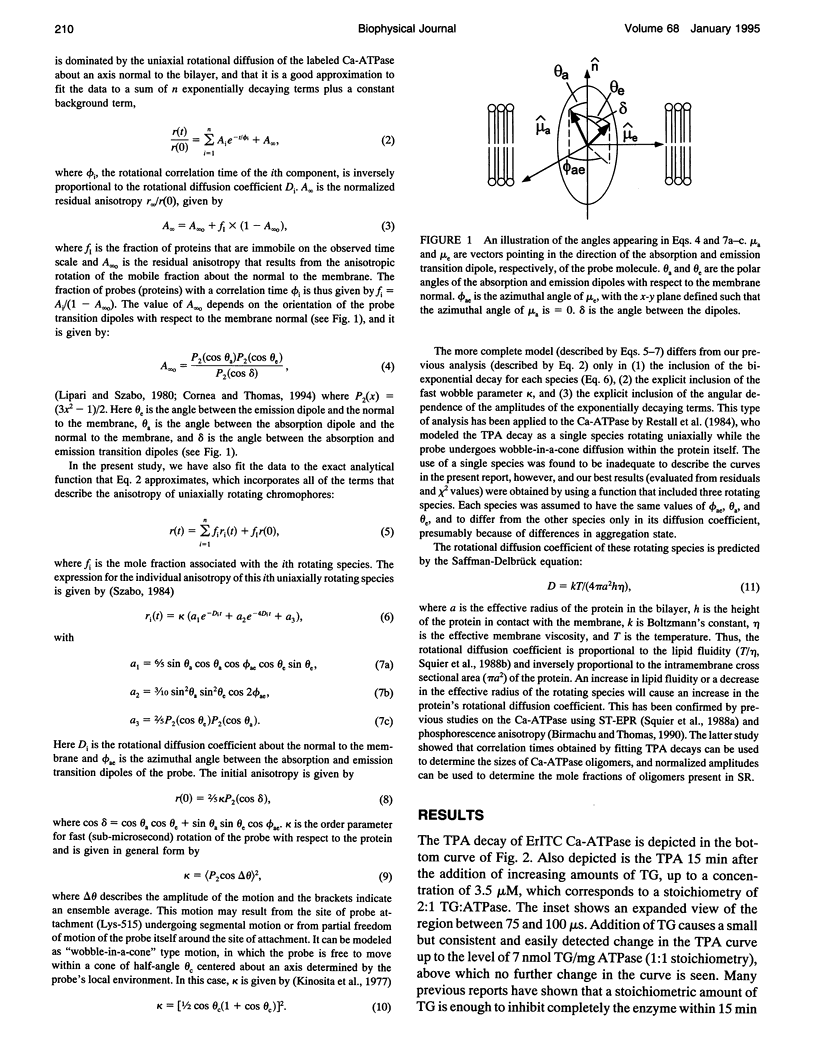
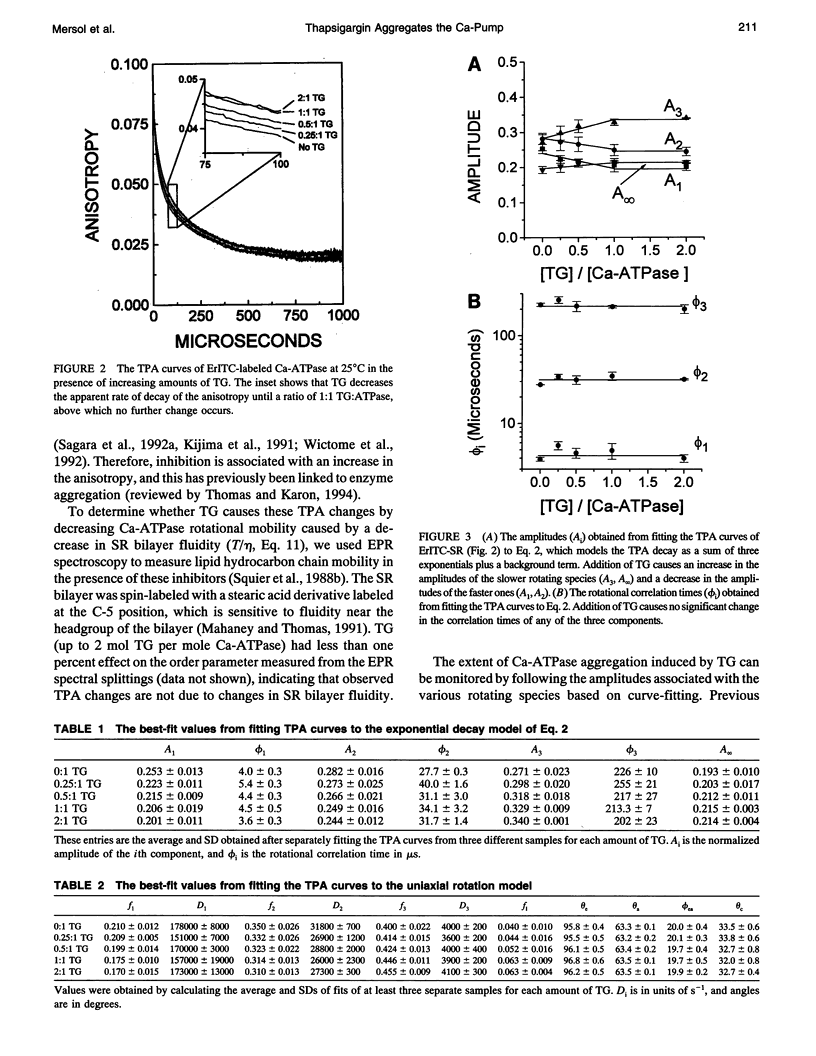
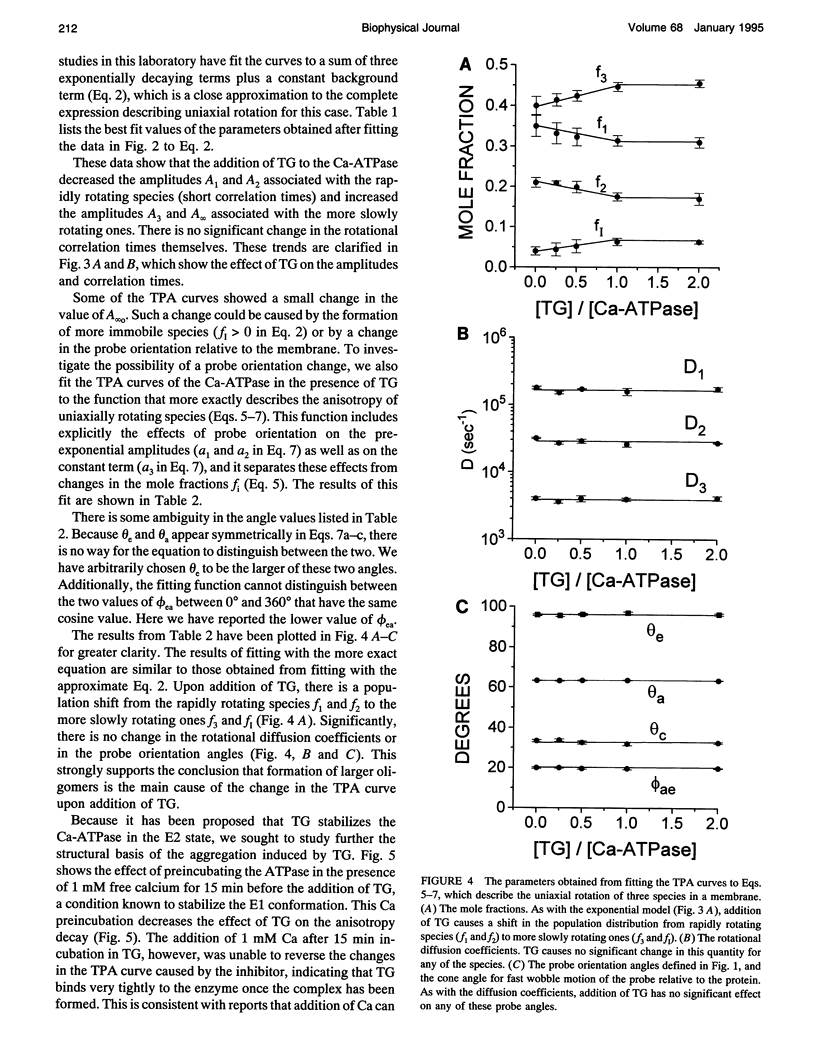
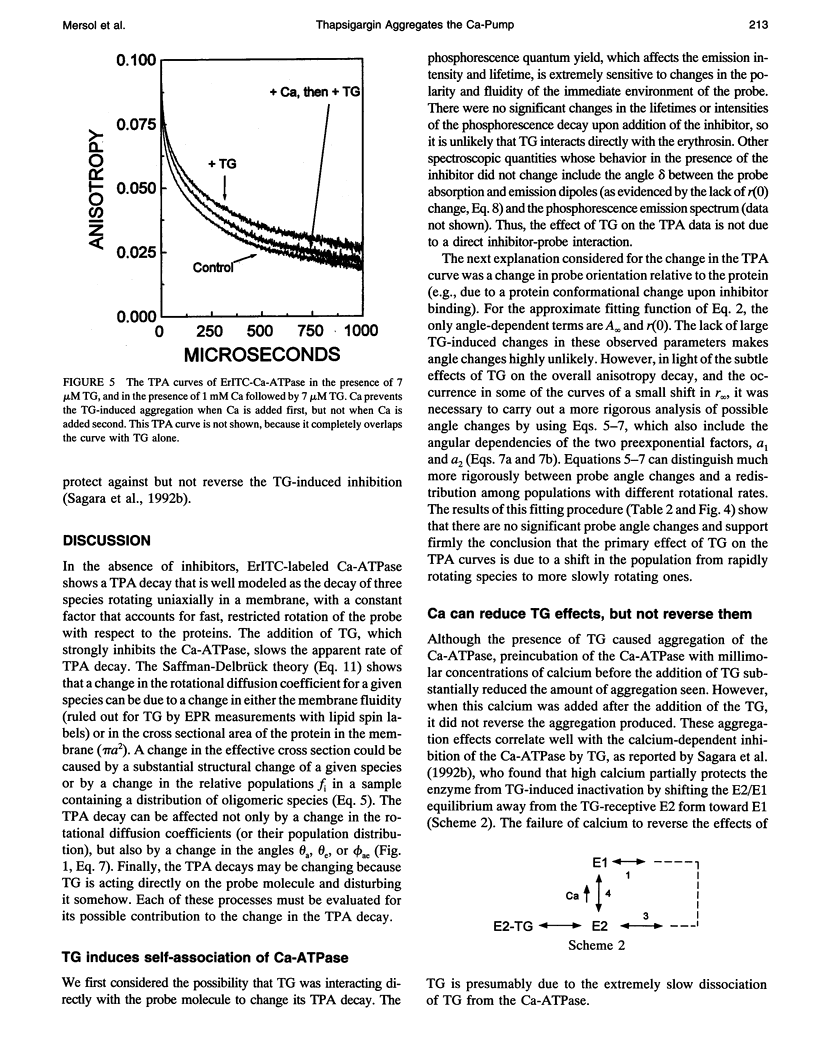
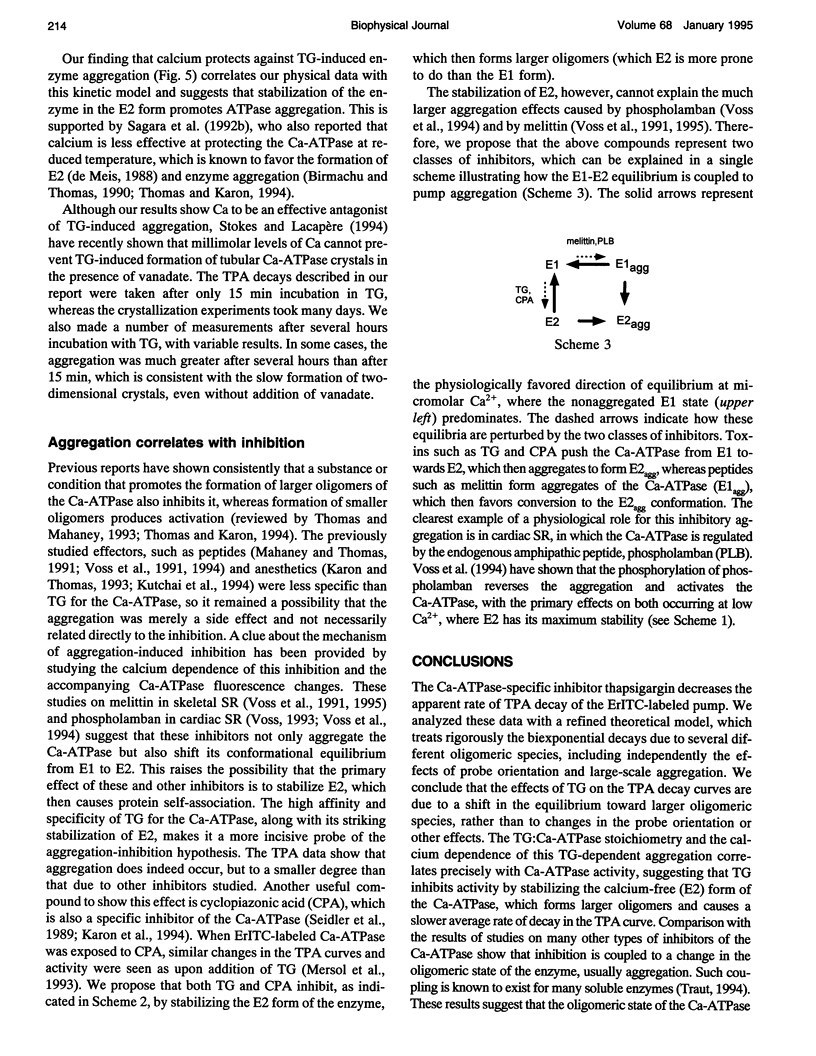
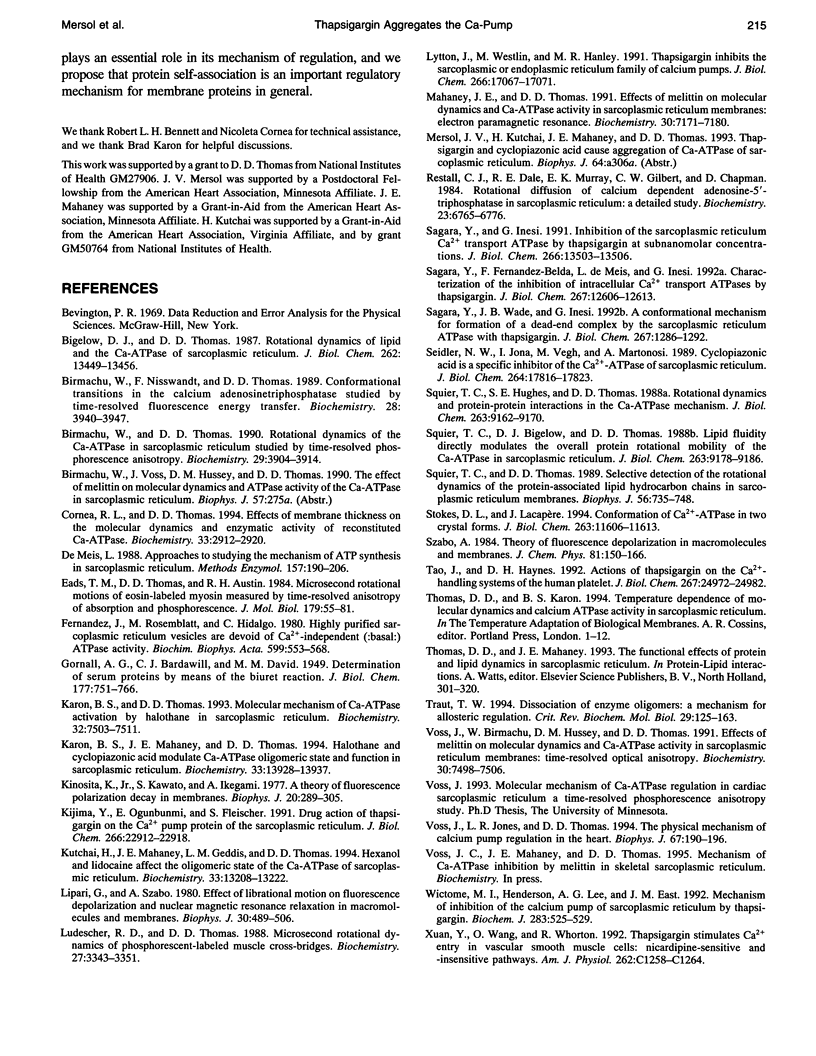
Images in this article
Selected References
These references are in PubMed. This may not be the complete list of references from this article.
- Bigelow D. J., Thomas D. D. Rotational dynamics of lipid and the Ca-ATPase in sarcoplasmic reticulum. The molecular basis of activation by diethyl ether. J Biol Chem. 1987 Oct 5;262(28):13449–13456. [PubMed] [Google Scholar]
- Birmachu W., Nisswandt F. L., Thomas D. D. Conformational transitions in the calcium adenosinetriphosphatase studied by time-resolved fluorescence resonance energy transfer. Biochemistry. 1989 May 2;28(9):3940–3947. doi: 10.1021/bi00435a047. [DOI] [PubMed] [Google Scholar]
- Birmachu W., Thomas D. D. Rotational dynamics of the Ca-ATPase in sarcoplasmic reticulum studied by time-resolved phosphorescence anisotropy. Biochemistry. 1990 Apr 24;29(16):3904–3914. doi: 10.1021/bi00468a017. [DOI] [PubMed] [Google Scholar]
- Cornea R. L., Thomas D. D. Effects of membrane thickness on the molecular dynamics and enzymatic activity of reconstituted Ca-ATPase. Biochemistry. 1994 Mar 15;33(10):2912–2920. doi: 10.1021/bi00176a022. [DOI] [PubMed] [Google Scholar]
- Eads T. M., Thomas D. D., Austin R. H. Microsecond rotational motions of eosin-labeled myosin measured by time-resolved anisotropy of absorption and phosphorescence. J Mol Biol. 1984 Oct 15;179(1):55–81. doi: 10.1016/0022-2836(84)90306-1. [DOI] [PubMed] [Google Scholar]
- Fernandez J. L., Rosemblatt M., Hidalgo C. Highly purified sarcoplasmic reticulum vesicles are devoid of Ca2+-independent ('basal') ATPase activity. Biochim Biophys Acta. 1980 Jul;599(2):552–568. doi: 10.1016/0005-2736(80)90199-6. [DOI] [PubMed] [Google Scholar]
- Karon B. S., Mahaney J. E., Thomas D. D. Halothane and cyclopiazonic acid modulate Ca-ATPase oligomeric state and function in sarcoplasmic reticulum. Biochemistry. 1994 Nov 22;33(46):13928–13937. doi: 10.1021/bi00250a048. [DOI] [PubMed] [Google Scholar]
- Karon B. S., Thomas D. D. Molecular mechanism of Ca-ATPase activation by halothane in sarcoplasmic reticulum. Biochemistry. 1993 Jul 27;32(29):7503–7511. doi: 10.1021/bi00080a023. [DOI] [PubMed] [Google Scholar]
- Kijima Y., Ogunbunmi E., Fleischer S. Drug action of thapsigargin on the Ca2+ pump protein of sarcoplasmic reticulum. J Biol Chem. 1991 Dec 5;266(34):22912–22918. [PubMed] [Google Scholar]
- Kinosita K., Jr, Kawato S., Ikegami A. A theory of fluorescence polarization decay in membranes. Biophys J. 1977 Dec;20(3):289–305. doi: 10.1016/S0006-3495(77)85550-1. [DOI] [PMC free article] [PubMed] [Google Scholar]
- Kutchai H., Mahaney J. E., Geddis L. M., Thomas D. D. Hexanol and lidocaine affect the oligomeric state of the Ca-ATPase of sarcoplasmic reticulum. Biochemistry. 1994 Nov 15;33(45):13208–13222. doi: 10.1021/bi00249a007. [DOI] [PubMed] [Google Scholar]
- Lipari G., Szabo A. Effect of librational motion on fluorescence depolarization and nuclear magnetic resonance relaxation in macromolecules and membranes. Biophys J. 1980 Jun;30(3):489–506. doi: 10.1016/S0006-3495(80)85109-5. [DOI] [PMC free article] [PubMed] [Google Scholar]
- Ludescher R. D., Thomas D. D. Microsecond rotational dynamics of phosphorescent-labeled muscle cross-bridges. Biochemistry. 1988 May 3;27(9):3343–3351. doi: 10.1021/bi00409a034. [DOI] [PubMed] [Google Scholar]
- Lytton J., Westlin M., Hanley M. R. Thapsigargin inhibits the sarcoplasmic or endoplasmic reticulum Ca-ATPase family of calcium pumps. J Biol Chem. 1991 Sep 15;266(26):17067–17071. [PubMed] [Google Scholar]
- Mahaney J. E., Thomas D. D. Effects of melittin on molecular dynamics and Ca-ATPase activity in sarcoplasmic reticulum membranes: electron paramagnetic resonance. Biochemistry. 1991 Jul 23;30(29):7171–7180. doi: 10.1021/bi00243a019. [DOI] [PubMed] [Google Scholar]
- Restall C. J., Dale R. E., Murray E. K., Gilbert C. W., Chapman D. Rotational diffusion of calcium-dependent adenosine-5'-triphosphatase in sarcoplasmic reticulum: a detailed study. Biochemistry. 1984 Dec 18;23(26):6765–6776. doi: 10.1021/bi00321a075. [DOI] [PubMed] [Google Scholar]
- Sagara Y., Fernandez-Belda F., de Meis L., Inesi G. Characterization of the inhibition of intracellular Ca2+ transport ATPases by thapsigargin. J Biol Chem. 1992 Jun 25;267(18):12606–12613. [PubMed] [Google Scholar]
- Sagara Y., Inesi G. Inhibition of the sarcoplasmic reticulum Ca2+ transport ATPase by thapsigargin at subnanomolar concentrations. J Biol Chem. 1991 Jul 25;266(21):13503–13506. [PubMed] [Google Scholar]
- Sagara Y., Wade J. B., Inesi G. A conformational mechanism for formation of a dead-end complex by the sarcoplasmic reticulum ATPase with thapsigargin. J Biol Chem. 1992 Jan 15;267(2):1286–1292. [PubMed] [Google Scholar]
- Seidler N. W., Jona I., Vegh M., Martonosi A. Cyclopiazonic acid is a specific inhibitor of the Ca2+-ATPase of sarcoplasmic reticulum. J Biol Chem. 1989 Oct 25;264(30):17816–17823. [PubMed] [Google Scholar]
- Squier T. C., Bigelow D. J., Thomas D. D. Lipid fluidity directly modulates the overall protein rotational mobility of the Ca-ATPase in sarcoplasmic reticulum. J Biol Chem. 1988 Jul 5;263(19):9178–9186. [PubMed] [Google Scholar]
- Squier T. C., Hughes S. E., Thomas D. D. Rotational dynamics and protein-protein interactions in the Ca-ATPase mechanism. J Biol Chem. 1988 Jul 5;263(19):9162–9170. [PubMed] [Google Scholar]
- Squier T. C., Thomas D. D. Selective detection of the rotational dynamics of the protein-associated lipid hydrocarbon chains in sarcoplasmic reticulum membranes. Biophys J. 1989 Oct;56(4):735–748. doi: 10.1016/S0006-3495(89)82721-3. [DOI] [PMC free article] [PubMed] [Google Scholar]
- Stokes D. L., Lacapère J. J. Conformation of Ca(2+)-ATPase in two crystal forms. Effects of Ca2+, thapsigargin, adenosine 5'-(beta, gamma-methylene)triphosphate), and chromium(III)-ATP on crystallization. J Biol Chem. 1994 Apr 15;269(15):11606–11613. [PubMed] [Google Scholar]
- Tao J., Haynes D. H. Actions of thapsigargin on the Ca(2+)-handling systems of the human platelet. Incomplete inhibition of the dense tubular Ca2+ uptake, partial inhibition of the Ca2+ extrusion pump, increase in plasma membrane Ca2+ permeability, and consequent elevation of resting cytoplasmic Ca2+. J Biol Chem. 1992 Dec 15;267(35):24972–24982. [PubMed] [Google Scholar]
- Traut T. W. Dissociation of enzyme oligomers: a mechanism for allosteric regulation. Crit Rev Biochem Mol Biol. 1994;29(2):125–163. doi: 10.3109/10409239409086799. [DOI] [PubMed] [Google Scholar]
- Voss J., Birmachu W., Hussey D. M., Thomas D. D. Effects of melittin on molecular dynamics and Ca-ATPase activity in sarcoplasmic reticulum membranes: time-resolved optical anisotropy. Biochemistry. 1991 Jul 30;30(30):7498–7506. doi: 10.1021/bi00244a019. [DOI] [PubMed] [Google Scholar]
- Voss J., Jones L. R., Thomas D. D. The physical mechanism of calcium pump regulation in the heart. Biophys J. 1994 Jul;67(1):190–196. doi: 10.1016/S0006-3495(94)80469-2. [DOI] [PMC free article] [PubMed] [Google Scholar]
- Wictome M., Henderson I., Lee A. G., East J. M. Mechanism of inhibition of the calcium pump of sarcoplasmic reticulum by thapsigargin. Biochem J. 1992 Apr 15;283(Pt 2):525–529. doi: 10.1042/bj2830525. [DOI] [PMC free article] [PubMed] [Google Scholar]
- Xuan Y. T., Wang O. L., Whorton A. R. Thapsigargin stimulates Ca2+ entry in vascular smooth muscle cells: nicardipine-sensitive and -insensitive pathways. Am J Physiol. 1992 May;262(5 Pt 1):C1258–C1265. doi: 10.1152/ajpcell.1992.262.5.C1258. [DOI] [PubMed] [Google Scholar]
- de Meis L. Approaches to studying the mechanisms of ATP synthesis in sarcoplasmic reticulum. Methods Enzymol. 1988;157:190–206. doi: 10.1016/0076-6879(88)57075-1. [DOI] [PubMed] [Google Scholar]