Abstract
The early, rapid phase of tension recovery (phase 2) after a step change in sarcomere length is thought to reflect the force-generating transition of myosin bound to actin. We have measured the relation between the rate of tension redevelopment during phase 2 (r), estimated from the half-time of tension recovery during phase 2 (r = t0.5(-1)), and steady-state force at varying [Ca2+] in single fibers from rabbit psoas. Sarcomere length was monitored continuously by laser diffraction of fiber segments (length approximately 1.6 mm), and sarcomere homogeneity was maintained using periodic length release/restretch cycles at 13-15 degrees C. At lower [Ca2+] and forces, r was elevated relative to that at pCa 4.0 for both releases and stretches (between +/- 8 nm). For releases of -3.4 +/- 0.7 nm.hs-1 at pCa 6.6 (where force was 10-20% of maximum force at pCa 4.0), r was 3.3 +/- 1.0 ms-1 (mean +/- SD; N = 5), whereas the corresponding value of r at pCa 4.0 was 1.0 +/- 0.2 ms-1 for releases of -3.5 +/- 0.5 nm.hs-1 (mean +/- SD; N = 5). For stretches of 1.9 +/- 0.7 nm.hs-1, r was 1.0 +/- 0.3 ms-1 (mean +/- SD; N = 9) at pCa 6.6, whereas r was 0.4 +/- 0.1 ms-1 at pCa 4.0 for stretches of 1.9 +/- 0.5 (mean +/- SD; N = 14). Faster phase 2 transients at submaximal Ca(2+)-activation were not caused by changes in myofilament lattice spacing because 4% Dextran T-500, which minimizes lattice spacing changes, was present in all solutions. The inverse relationship between phase 2 kinetics and force obtained during steady-state activation of skinned fibers appears to be qualitatively similar to observations on intact frog skeletal fibers during the development of tetanic force. The data are consistent with models that incorporate a direct effect of [Ca2+] on phase 2 kinetics of individual cross-bridges or, alternatively, in which phase 2 kinetics depend on cooperative interactions between cross-bridges.
Full text
PDF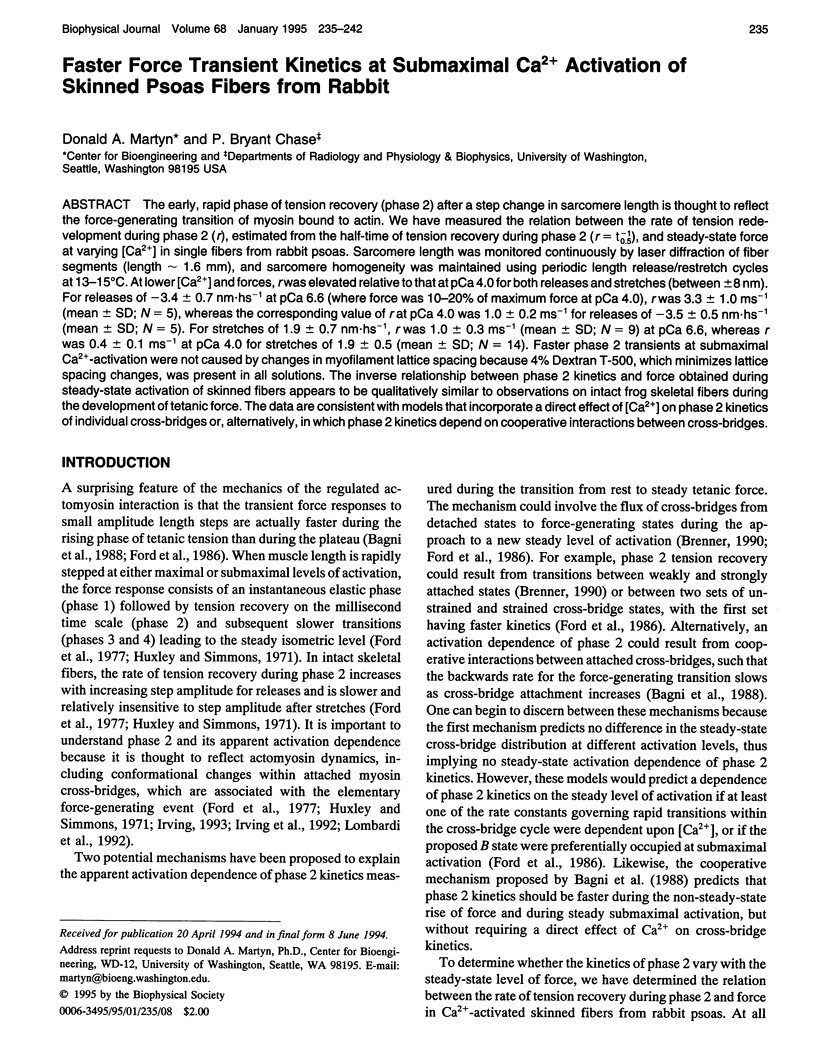
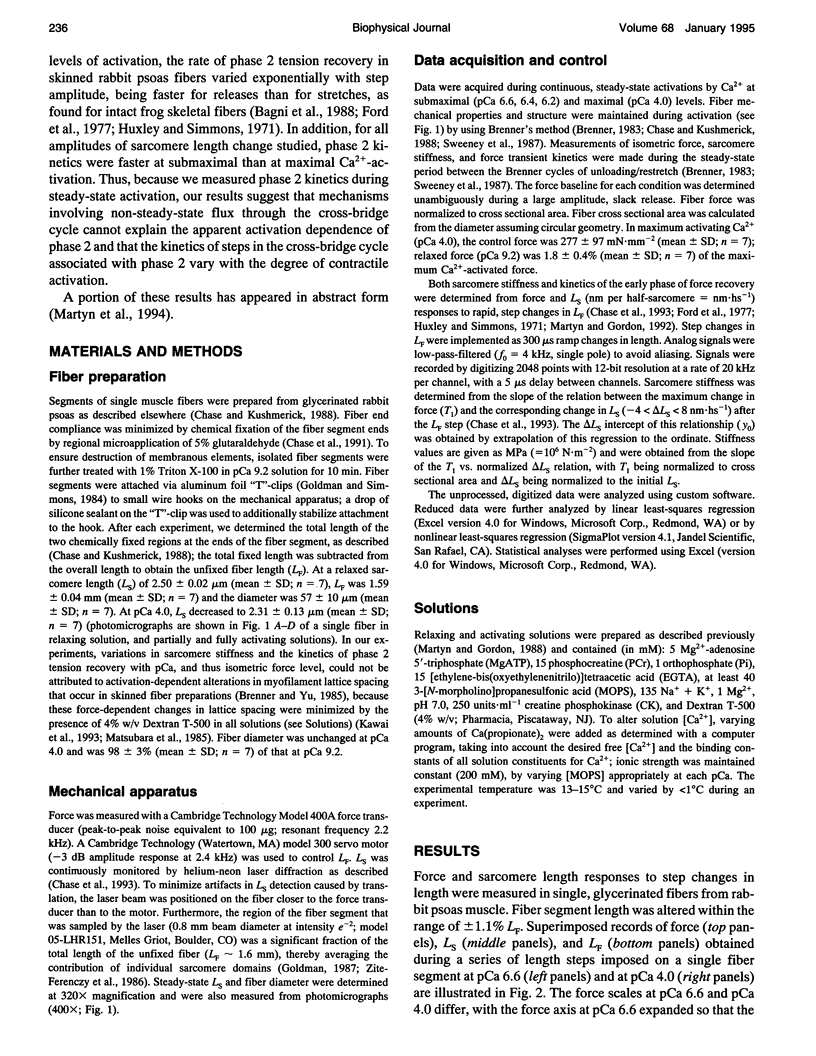
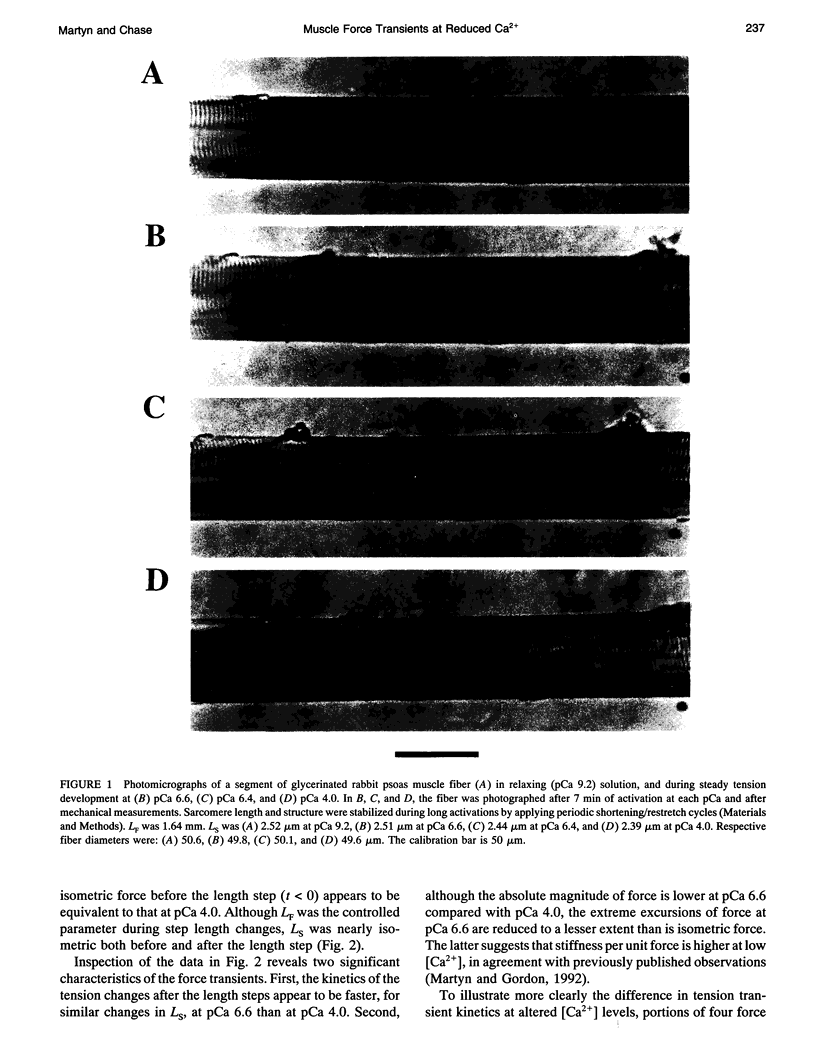
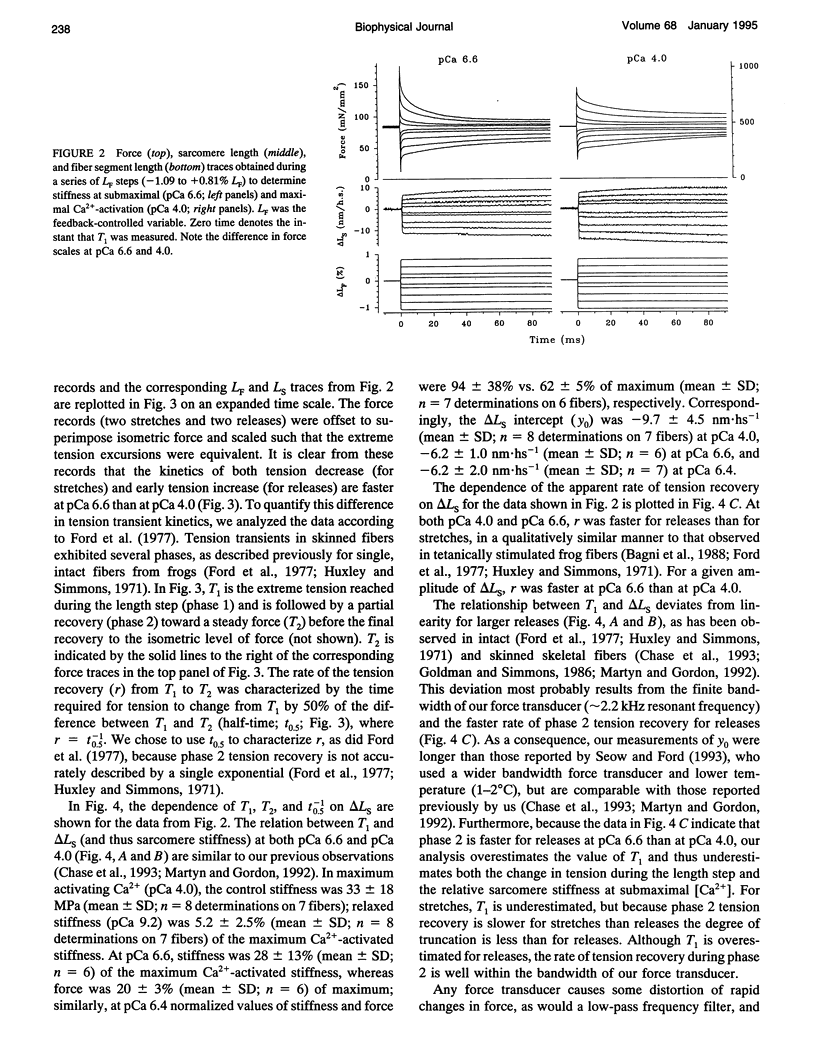
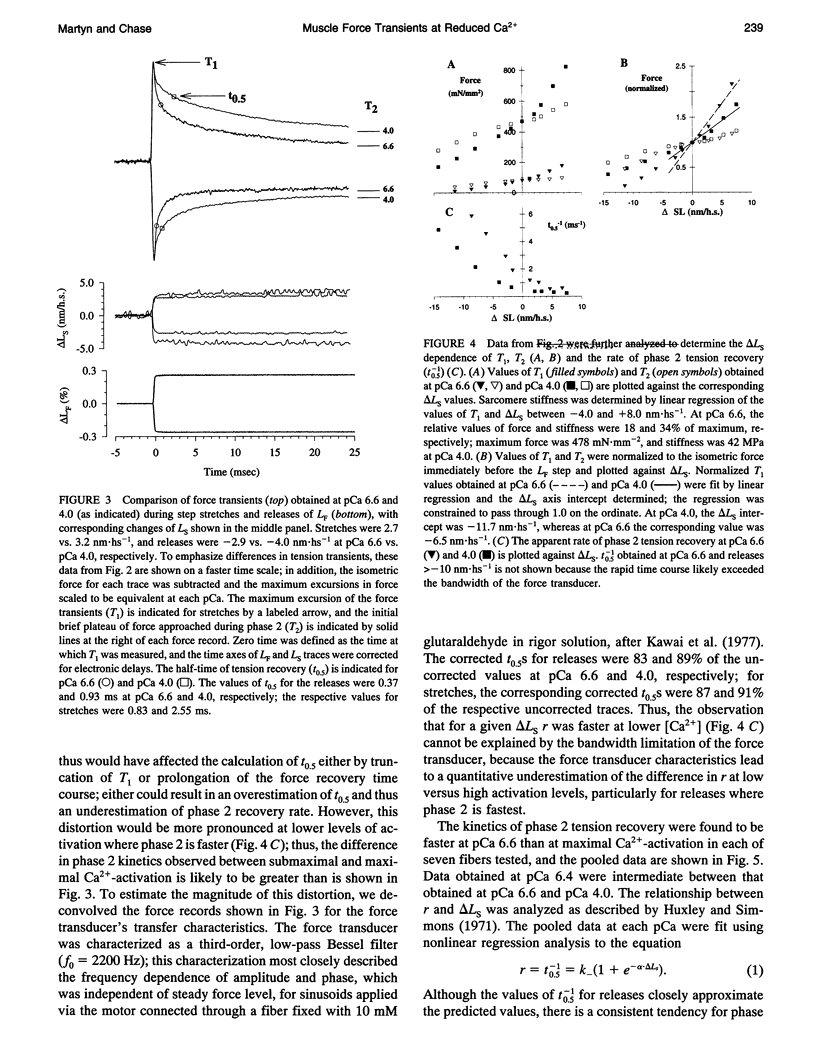
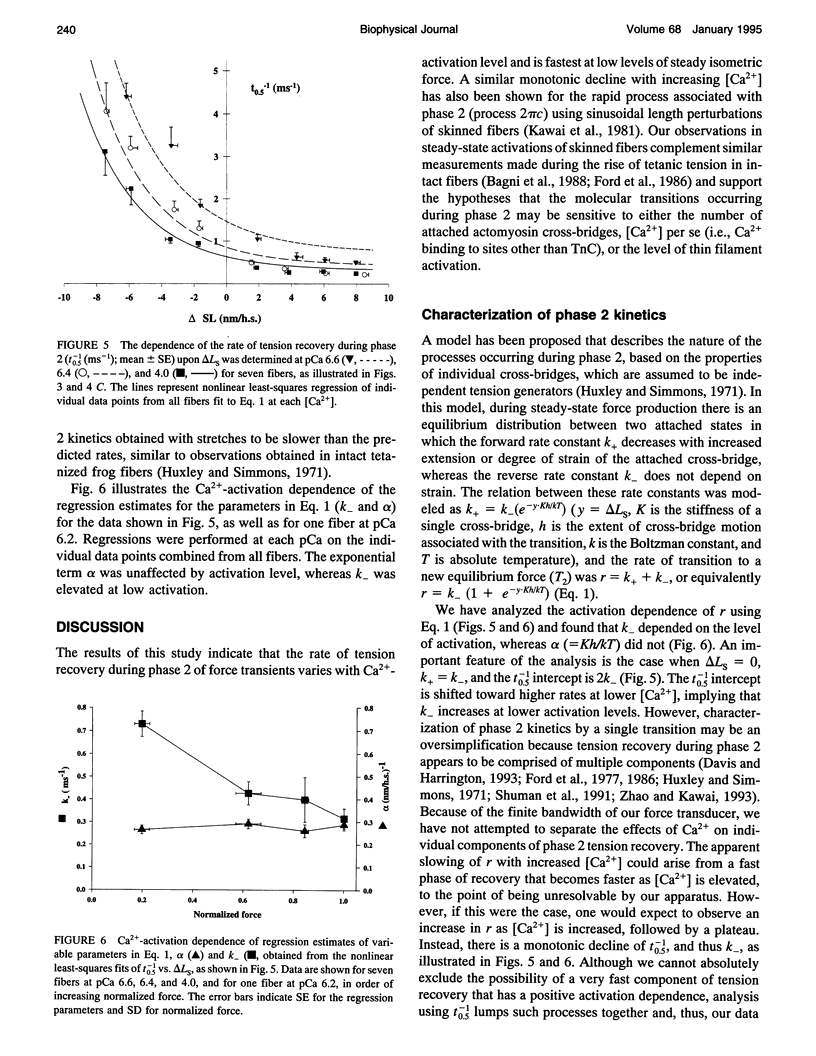
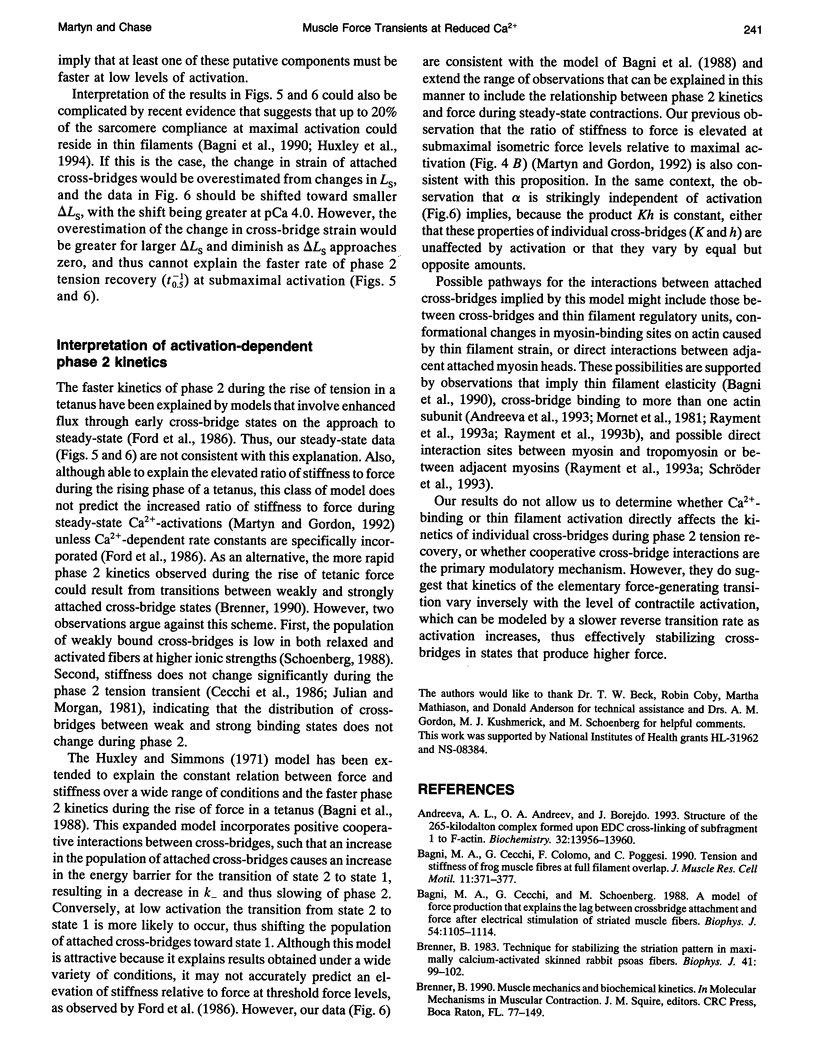
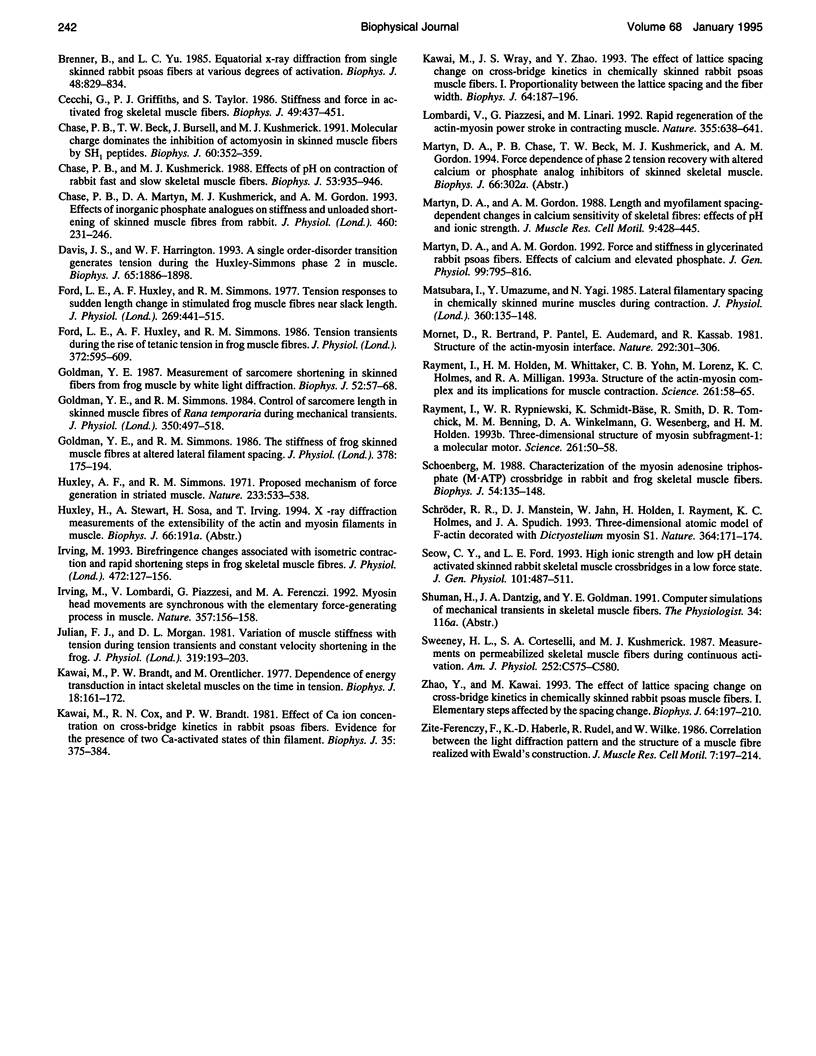
Images in this article
Selected References
These references are in PubMed. This may not be the complete list of references from this article.
- Andreeva A. L., Andreev O. A., Borejdo J. Structure of the 265-kilodalton complex formed upon EDC cross-linking of subfragment 1 to F-actin. Biochemistry. 1993 Dec 21;32(50):13956–13960. doi: 10.1021/bi00213a027. [DOI] [PubMed] [Google Scholar]
- Bagni M. A., Cecchi G., Colomo F., Poggesi C. Tension and stiffness of frog muscle fibres at full filament overlap. J Muscle Res Cell Motil. 1990 Oct;11(5):371–377. doi: 10.1007/BF01739758. [DOI] [PubMed] [Google Scholar]
- Bagni M. A., Cecchi G., Schoenberg M. A model of force production that explains the lag between crossbridge attachment and force after electrical stimulation of striated muscle fibers. Biophys J. 1988 Dec;54(6):1105–1114. doi: 10.1016/S0006-3495(88)83046-7. [DOI] [PMC free article] [PubMed] [Google Scholar]
- Brenner B. Technique for stabilizing the striation pattern in maximally calcium-activated skinned rabbit psoas fibers. Biophys J. 1983 Jan;41(1):99–102. doi: 10.1016/S0006-3495(83)84411-7. [DOI] [PMC free article] [PubMed] [Google Scholar]
- Brenner B., Yu L. C. Equatorial x-ray diffraction from single skinned rabbit psoas fibers at various degrees of activation. Changes in intensities and lattice spacing. Biophys J. 1985 Nov;48(5):829–834. doi: 10.1016/S0006-3495(85)83841-8. [DOI] [PMC free article] [PubMed] [Google Scholar]
- Cecchi G., Griffiths P. J., Taylor S. Stiffness and force in activated frog skeletal muscle fibers. Biophys J. 1986 Feb;49(2):437–451. doi: 10.1016/S0006-3495(86)83653-0. [DOI] [PMC free article] [PubMed] [Google Scholar]
- Chase P. B., Beck T. W., Bursell J., Kushmerick M. J. Molecular charge dominates the inhibition of actomyosin in skinned muscle fibers by SH1 peptides. Biophys J. 1991 Aug;60(2):352–359. doi: 10.1016/S0006-3495(91)82060-4. [DOI] [PMC free article] [PubMed] [Google Scholar]
- Chase P. B., Kushmerick M. J. Effects of pH on contraction of rabbit fast and slow skeletal muscle fibers. Biophys J. 1988 Jun;53(6):935–946. doi: 10.1016/S0006-3495(88)83174-6. [DOI] [PMC free article] [PubMed] [Google Scholar]
- Chase P. B., Martyn D. A., Kushmerick M. J., Gordon A. M. Effects of inorganic phosphate analogues on stiffness and unloaded shortening of skinned muscle fibres from rabbit. J Physiol. 1993 Jan;460:231–246. doi: 10.1113/jphysiol.1993.sp019469. [DOI] [PMC free article] [PubMed] [Google Scholar]
- Davis J. S., Harrington W. F. A single order-disorder transition generates tension during the Huxley-Simmons phase 2 in muscle. Biophys J. 1993 Nov;65(5):1886–1898. doi: 10.1016/S0006-3495(93)81259-1. [DOI] [PMC free article] [PubMed] [Google Scholar]
- Ford L. E., Huxley A. F., Simmons R. M. Tension responses to sudden length change in stimulated frog muscle fibres near slack length. J Physiol. 1977 Jul;269(2):441–515. doi: 10.1113/jphysiol.1977.sp011911. [DOI] [PMC free article] [PubMed] [Google Scholar]
- Ford L. E., Huxley A. F., Simmons R. M. Tension transients during the rise of tetanic tension in frog muscle fibres. J Physiol. 1986 Mar;372:595–609. doi: 10.1113/jphysiol.1986.sp016027. [DOI] [PMC free article] [PubMed] [Google Scholar]
- Goldman Y. E. Measurement of sarcomere shortening in skinned fibers from frog muscle by white light diffraction. Biophys J. 1987 Jul;52(1):57–68. doi: 10.1016/S0006-3495(87)83188-0. [DOI] [PMC free article] [PubMed] [Google Scholar]
- Goldman Y. E., Simmons R. M. Control of sarcomere length in skinned muscle fibres of Rana temporaria during mechanical transients. J Physiol. 1984 May;350:497–518. doi: 10.1113/jphysiol.1984.sp015215. [DOI] [PMC free article] [PubMed] [Google Scholar]
- Goldman Y. E., Simmons R. M. The stiffness of frog skinned muscle fibres at altered lateral filament spacing. J Physiol. 1986 Sep;378:175–194. doi: 10.1113/jphysiol.1986.sp016213. [DOI] [PMC free article] [PubMed] [Google Scholar]
- Huxley A. F., Simmons R. M. Proposed mechanism of force generation in striated muscle. Nature. 1971 Oct 22;233(5321):533–538. doi: 10.1038/233533a0. [DOI] [PubMed] [Google Scholar]
- Irving M. Birefringence changes associated with isometric contraction and rapid shortening steps in frog skeletal muscle fibres. J Physiol. 1993 Dec;472:127–156. doi: 10.1113/jphysiol.1993.sp019940. [DOI] [PMC free article] [PubMed] [Google Scholar]
- Irving M., Lombardi V., Piazzesi G., Ferenczi M. A. Myosin head movements are synchronous with the elementary force-generating process in muscle. Nature. 1992 May 14;357(6374):156–158. doi: 10.1038/357156a0. [DOI] [PubMed] [Google Scholar]
- Julian F. J., Morgan D. L. Variation of muscle stiffness with tension during tension transients and constant velocity shortening in the frog. J Physiol. 1981;319:193–203. doi: 10.1113/jphysiol.1981.sp013901. [DOI] [PMC free article] [PubMed] [Google Scholar]
- Kawai M., Brandt P., Orentlicher M. Dependence of energy transduction in intact skeletal muscles on the time in tension. Biophys J. 1977 May;18(2):161–172. doi: 10.1016/S0006-3495(77)85605-1. [DOI] [PMC free article] [PubMed] [Google Scholar]
- Kawai M., Cox R. N., Brandt P. W. Effect of Ca ion concentration on cross-bridge kinetics in rabbit psoas fibers. Evidence for the presence of two Ca-activated states of thin filament. Biophys J. 1981 Aug;35(2):375–384. doi: 10.1016/S0006-3495(81)84796-0. [DOI] [PMC free article] [PubMed] [Google Scholar]
- Kawai M., Wray J. S., Zhao Y. The effect of lattice spacing change on cross-bridge kinetics in chemically skinned rabbit psoas muscle fibers. I. Proportionality between the lattice spacing and the fiber width. Biophys J. 1993 Jan;64(1):187–196. doi: 10.1016/S0006-3495(93)81356-0. [DOI] [PMC free article] [PubMed] [Google Scholar]
- Lombardi V., Piazzesi G., Linari M. Rapid regeneration of the actin-myosin power stroke in contracting muscle. Nature. 1992 Feb 13;355(6361):638–641. doi: 10.1038/355638a0. [DOI] [PubMed] [Google Scholar]
- Martyn D. A., Gordon A. M. Force and stiffness in glycerinated rabbit psoas fibers. Effects of calcium and elevated phosphate. J Gen Physiol. 1992 May;99(5):795–816. doi: 10.1085/jgp.99.5.795. [DOI] [PMC free article] [PubMed] [Google Scholar]
- Martyn D. A., Gordon A. M. Length and myofilament spacing-dependent changes in calcium sensitivity of skeletal fibres: effects of pH and ionic strength. J Muscle Res Cell Motil. 1988 Oct;9(5):428–445. doi: 10.1007/BF01774069. [DOI] [PubMed] [Google Scholar]
- Matsubara I., Umazume Y., Yagi N. Lateral filamentary spacing in chemically skinned murine muscles during contraction. J Physiol. 1985 Mar;360:135–148. doi: 10.1113/jphysiol.1985.sp015608. [DOI] [PMC free article] [PubMed] [Google Scholar]
- Mornet D., Bertrand R., Pantel P., Audemard E., Kassab R. Structure of the actin-myosin interface. Nature. 1981 Jul 23;292(5821):301–306. doi: 10.1038/292301a0. [DOI] [PubMed] [Google Scholar]
- Rayment I., Holden H. M., Whittaker M., Yohn C. B., Lorenz M., Holmes K. C., Milligan R. A. Structure of the actin-myosin complex and its implications for muscle contraction. Science. 1993 Jul 2;261(5117):58–65. doi: 10.1126/science.8316858. [DOI] [PubMed] [Google Scholar]
- Rayment I., Rypniewski W. R., Schmidt-Bäse K., Smith R., Tomchick D. R., Benning M. M., Winkelmann D. A., Wesenberg G., Holden H. M. Three-dimensional structure of myosin subfragment-1: a molecular motor. Science. 1993 Jul 2;261(5117):50–58. doi: 10.1126/science.8316857. [DOI] [PubMed] [Google Scholar]
- Schoenberg M. Characterization of the myosin adenosine triphosphate (M.ATP) crossbridge in rabbit and frog skeletal muscle fibers. Biophys J. 1988 Jul;54(1):135–148. doi: 10.1016/S0006-3495(88)82938-2. [DOI] [PMC free article] [PubMed] [Google Scholar]
- Schröder R. R., Manstein D. J., Jahn W., Holden H., Rayment I., Holmes K. C., Spudich J. A. Three-dimensional atomic model of F-actin decorated with Dictyostelium myosin S1. Nature. 1993 Jul 8;364(6433):171–174. doi: 10.1038/364171a0. [DOI] [PubMed] [Google Scholar]
- Seow C. Y., Ford L. E. High ionic strength and low pH detain activated skinned rabbit skeletal muscle crossbridges in a low force state. J Gen Physiol. 1993 Apr;101(4):487–511. doi: 10.1085/jgp.101.4.487. [DOI] [PMC free article] [PubMed] [Google Scholar]
- Sweeney H. L., Corteselli S. A., Kushmerick M. J. Measurements on permeabilized skeletal muscle fibers during continuous activation. Am J Physiol. 1987 May;252(5 Pt 1):C575–C580. doi: 10.1152/ajpcell.1987.252.5.C575. [DOI] [PubMed] [Google Scholar]
- Zhao Y., Kawai M. The effect of the lattice spacing change on cross-bridge kinetics in chemically skinned rabbit psoas muscle fibers. II. Elementary steps affected by the spacing change. Biophys J. 1993 Jan;64(1):197–210. doi: 10.1016/S0006-3495(93)81357-2. [DOI] [PMC free article] [PubMed] [Google Scholar]
- Zite-Ferenczy F., Häberle K. D., Rüdel R., Wilke W. Correlation between the light diffraction pattern and the structure of a muscle fibre realized with Ewald's construction. J Muscle Res Cell Motil. 1986 Jun;7(3):197–214. doi: 10.1007/BF01753553. [DOI] [PubMed] [Google Scholar]