Abstract
The folding of short alanine-based peptides with different numbers of lysine residues is simulated at constant temperature (274 K) using the rigid-element Monte Carlo method. The solvent-referenced potential has prevented the multiple-minima problem in helix folding. From various initial structures, the peptides with three lysine residues fold into helix-dominated conformations with the calculated average helicity in the range of 60-80%. The peptide with six lysine residues shows only 8-14% helicity. These results agree well with experimental observations. The intramolecular electrostatic interaction of the charged lysine side chains and their electrostatic hydration destabilize the helical conformations of the peptide with six lysine residues, whereas these effects on the peptides with three lysine residues are small. The simulations provide insight into the helix-folding mechanism, including the beta-bend intermediate in helix initiation, the (i, i + 3) hydrogen bonds, the asymmetrical helix propagation, and the asymmetrical helicities in the N- and C-terminal regions. These findings are consistent with previous studies.
Full text
PDF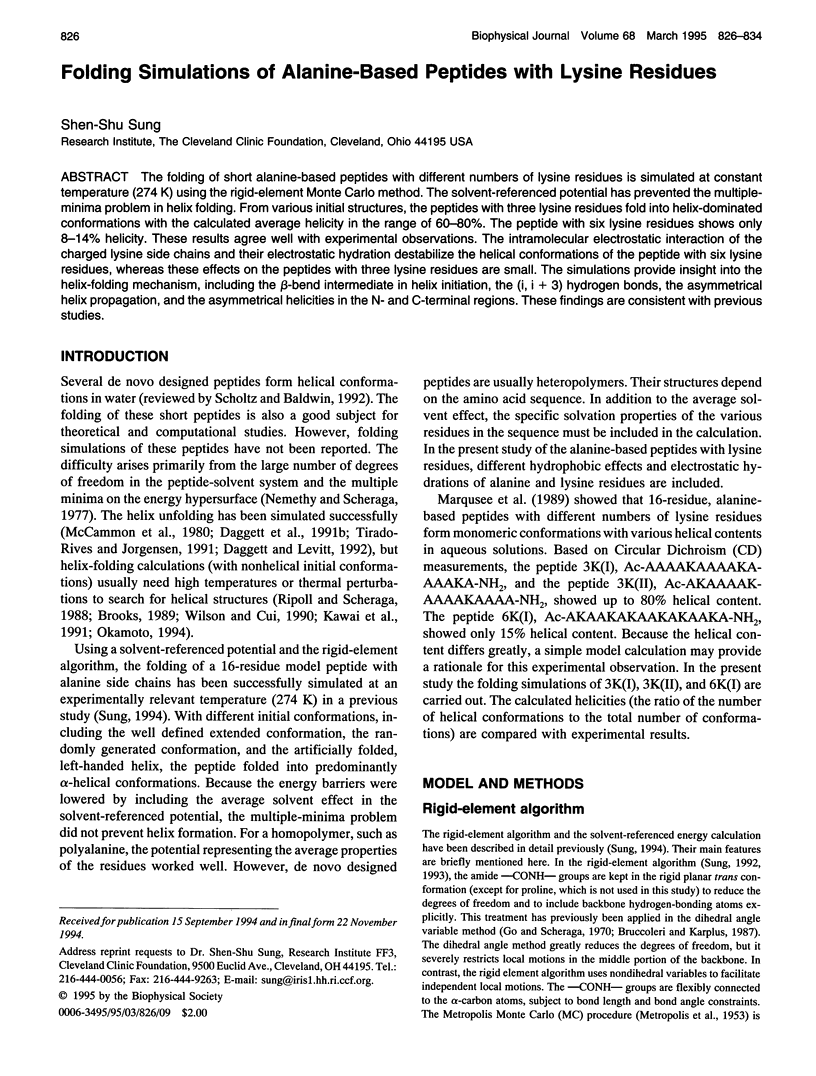
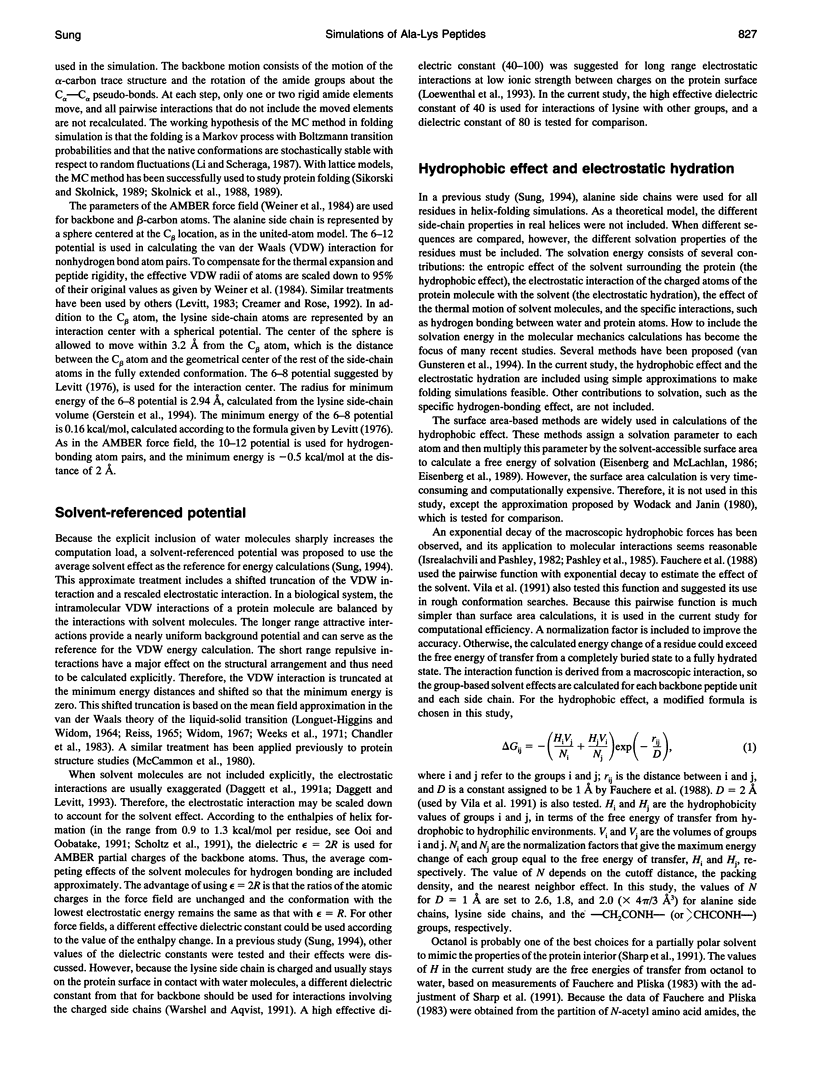
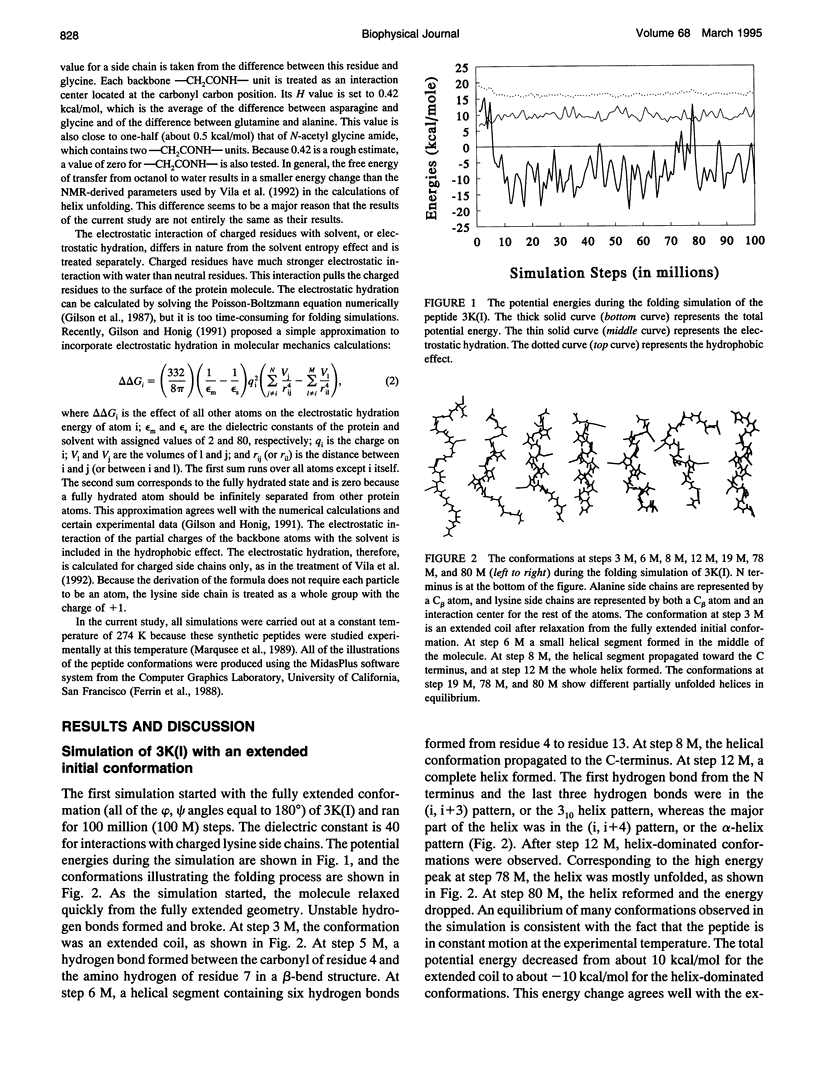
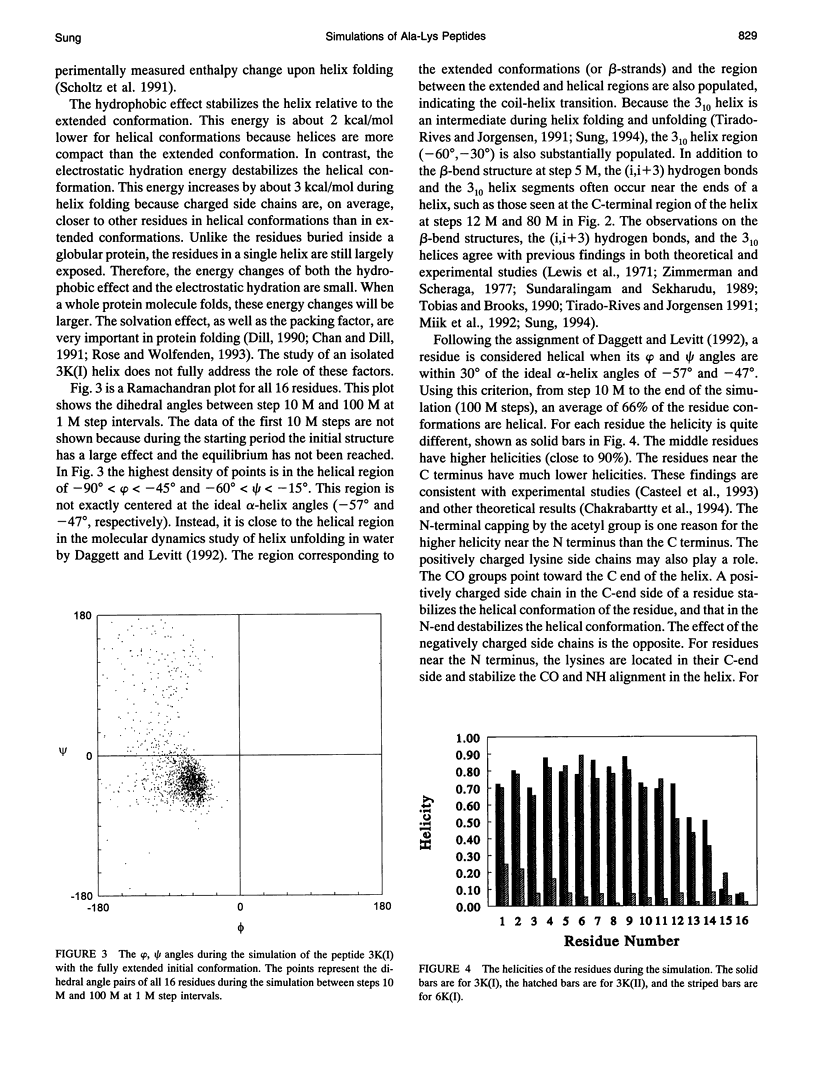
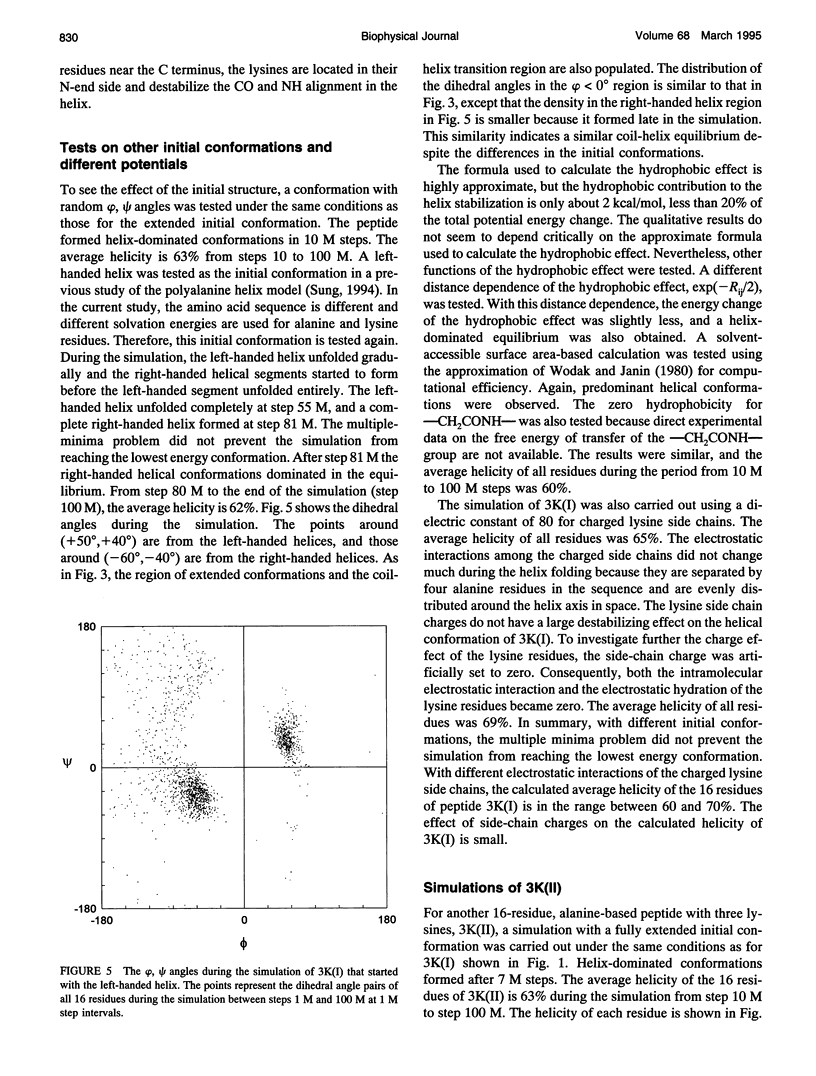
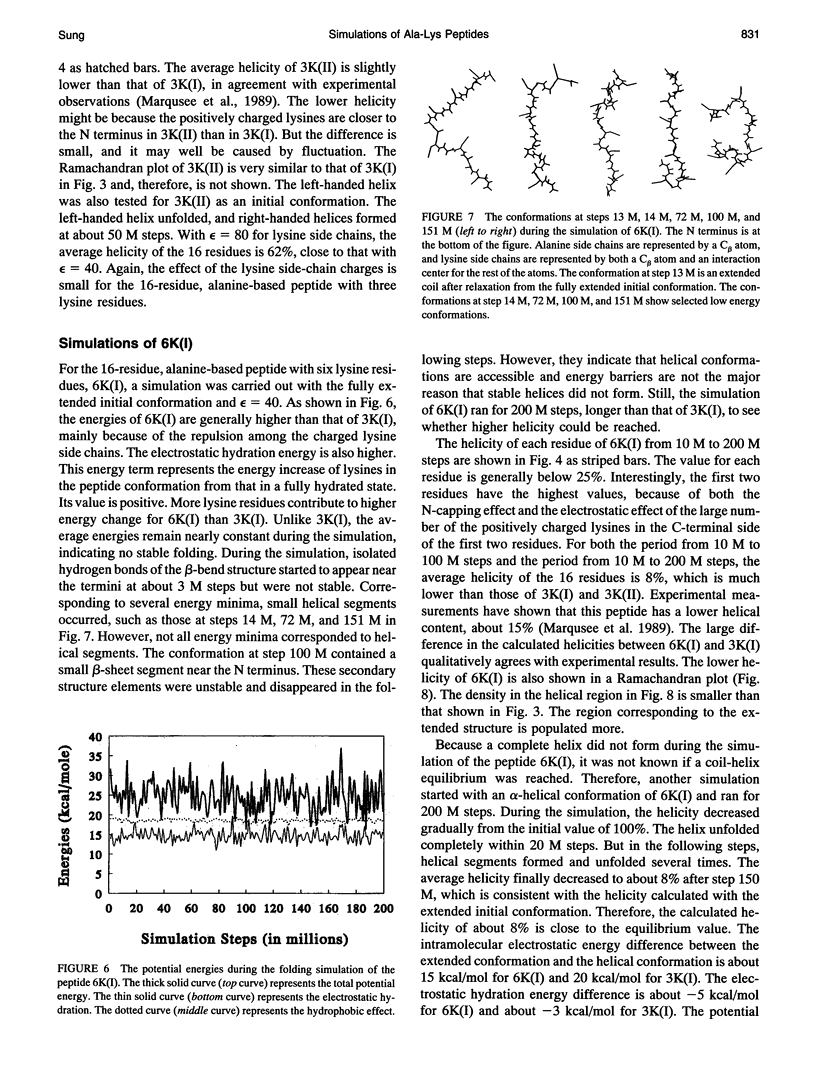
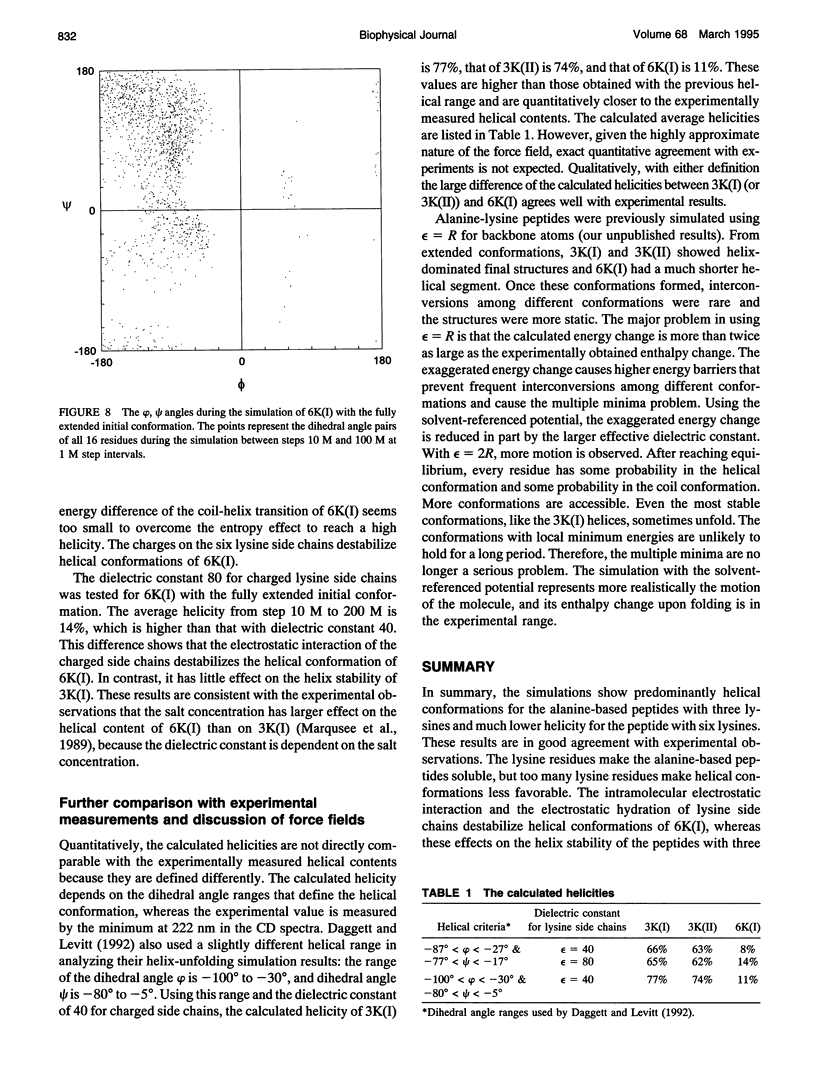
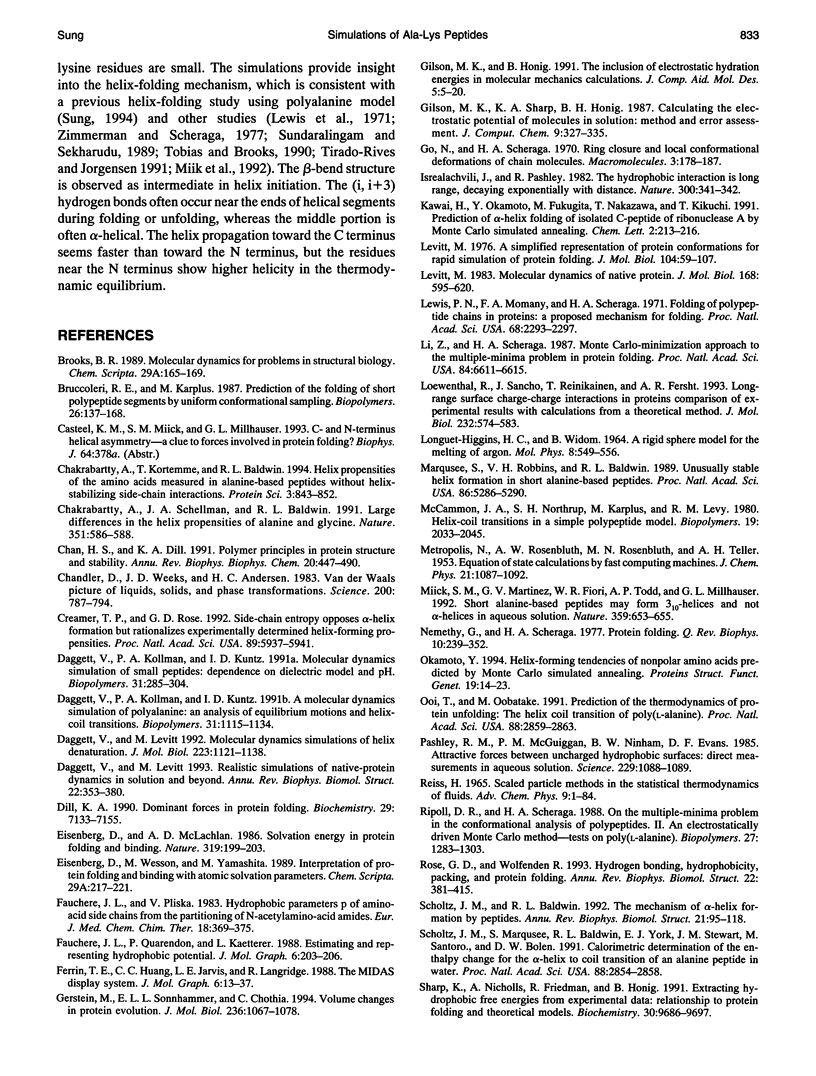
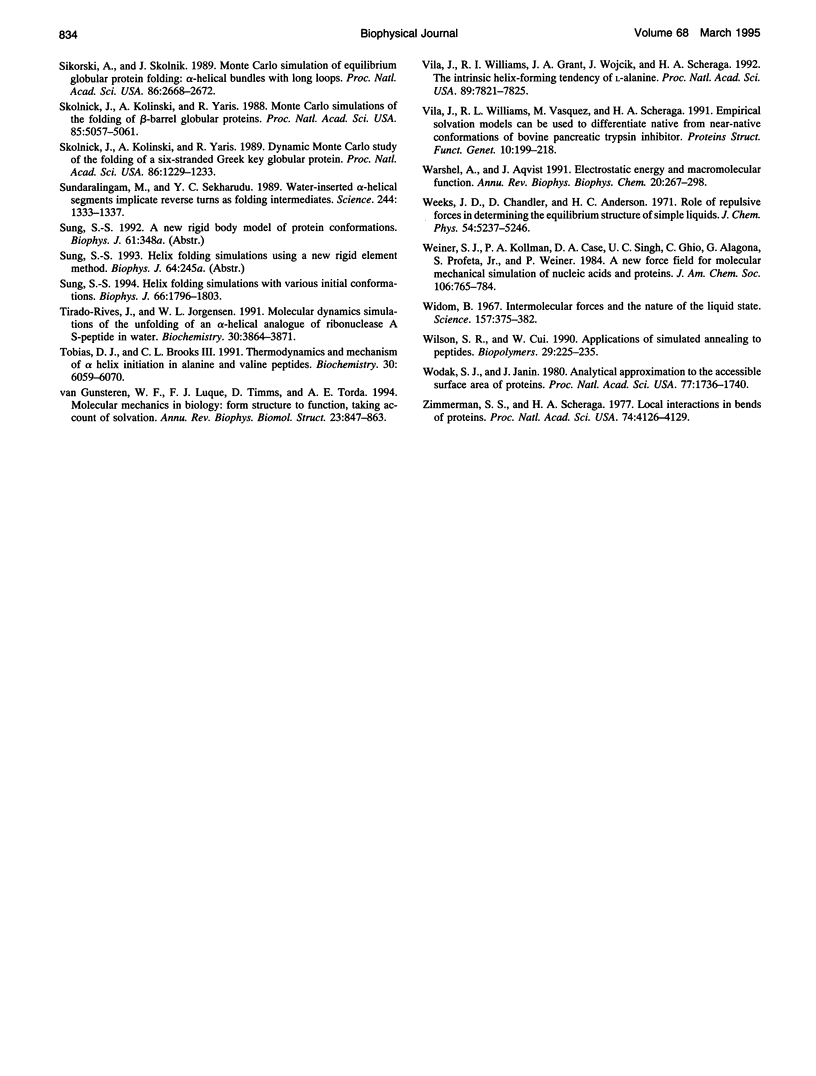
Selected References
These references are in PubMed. This may not be the complete list of references from this article.
- Bruccoleri R. E., Karplus M. Prediction of the folding of short polypeptide segments by uniform conformational sampling. Biopolymers. 1987 Jan;26(1):137–168. doi: 10.1002/bip.360260114. [DOI] [PubMed] [Google Scholar]
- Chakrabartty A., Kortemme T., Baldwin R. L. Helix propensities of the amino acids measured in alanine-based peptides without helix-stabilizing side-chain interactions. Protein Sci. 1994 May;3(5):843–852. doi: 10.1002/pro.5560030514. [DOI] [PMC free article] [PubMed] [Google Scholar]
- Chakrabartty A., Schellman J. A., Baldwin R. L. Large differences in the helix propensities of alanine and glycine. Nature. 1991 Jun 13;351(6327):586–588. doi: 10.1038/351586a0. [DOI] [PubMed] [Google Scholar]
- Chan H. S., Dill K. A. Polymer principles in protein structure and stability. Annu Rev Biophys Biophys Chem. 1991;20:447–490. doi: 10.1146/annurev.bb.20.060191.002311. [DOI] [PubMed] [Google Scholar]
- Chandler D., Weeks J. D., Andersen H. C. Van der waals picture of liquids, solids, and phase transformations. Science. 1983 May 20;220(4599):787–794. doi: 10.1126/science.220.4599.787. [DOI] [PubMed] [Google Scholar]
- Creamer T. P., Rose G. D. Side-chain entropy opposes alpha-helix formation but rationalizes experimentally determined helix-forming propensities. Proc Natl Acad Sci U S A. 1992 Jul 1;89(13):5937–5941. doi: 10.1073/pnas.89.13.5937. [DOI] [PMC free article] [PubMed] [Google Scholar]
- Daggett V., Kollman P. A., Kuntz I. D. A molecular dynamics simulation of polyalanine: an analysis of equilibrium motions and helix-coil transitions. Biopolymers. 1991 Aug;31(9):1115–1134. doi: 10.1002/bip.360310911. [DOI] [PubMed] [Google Scholar]
- Daggett V., Kollman P. A., Kuntz I. D. Molecular dynamics simulations of small peptides: dependence on dielectric model and pH. Biopolymers. 1991 Feb 15;31(3):285–304. doi: 10.1002/bip.360310304. [DOI] [PubMed] [Google Scholar]
- Daggett V., Levitt M. Molecular dynamics simulations of helix denaturation. J Mol Biol. 1992 Feb 20;223(4):1121–1138. doi: 10.1016/0022-2836(92)90264-k. [DOI] [PubMed] [Google Scholar]
- Daggett V., Levitt M. Realistic simulations of native-protein dynamics in solution and beyond. Annu Rev Biophys Biomol Struct. 1993;22:353–380. doi: 10.1146/annurev.bb.22.060193.002033. [DOI] [PubMed] [Google Scholar]
- Dill K. A. Dominant forces in protein folding. Biochemistry. 1990 Aug 7;29(31):7133–7155. doi: 10.1021/bi00483a001. [DOI] [PubMed] [Google Scholar]
- Eisenberg D., McLachlan A. D. Solvation energy in protein folding and binding. Nature. 1986 Jan 16;319(6050):199–203. doi: 10.1038/319199a0. [DOI] [PubMed] [Google Scholar]
- Gerstein M., Sonnhammer E. L., Chothia C. Volume changes in protein evolution. J Mol Biol. 1994 Mar 4;236(4):1067–1078. doi: 10.1016/0022-2836(94)90012-4. [DOI] [PubMed] [Google Scholar]
- Gilson M. K., Honig B. The inclusion of electrostatic hydration energies in molecular mechanics calculations. J Comput Aided Mol Des. 1991 Feb;5(1):5–20. doi: 10.1007/BF00173467. [DOI] [PubMed] [Google Scholar]
- Israelachvili J., Pashley R. The hydrophobic interaction is long range, decaying exponentially with distance. Nature. 1982 Nov 25;300(5890):341–342. doi: 10.1038/300341a0. [DOI] [PubMed] [Google Scholar]
- Komine S., Yoshida K., Yamashita H., Masaki Z. Voiding dysfunction in patients with human T-lymphotropic virus type-1-associated myelopathy (HAM). Paraplegia. 1989 Jun;27(3):217–221. doi: 10.1038/sc.1989.32. [DOI] [PubMed] [Google Scholar]
- Levitt M. A simplified representation of protein conformations for rapid simulation of protein folding. J Mol Biol. 1976 Jun 14;104(1):59–107. doi: 10.1016/0022-2836(76)90004-8. [DOI] [PubMed] [Google Scholar]
- Levitt M. Molecular dynamics of native protein. I. Computer simulation of trajectories. J Mol Biol. 1983 Aug 15;168(3):595–617. doi: 10.1016/s0022-2836(83)80304-0. [DOI] [PubMed] [Google Scholar]
- Lewis P. N., Momany F. A., Scheraga H. A. Folding of polypeptide chains in proteins: a proposed mechanism for folding. Proc Natl Acad Sci U S A. 1971 Sep;68(9):2293–2297. doi: 10.1073/pnas.68.9.2293. [DOI] [PMC free article] [PubMed] [Google Scholar]
- Li Z., Scheraga H. A. Monte Carlo-minimization approach to the multiple-minima problem in protein folding. Proc Natl Acad Sci U S A. 1987 Oct;84(19):6611–6615. doi: 10.1073/pnas.84.19.6611. [DOI] [PMC free article] [PubMed] [Google Scholar]
- Loewenthal R., Sancho J., Reinikainen T., Fersht A. R. Long-range surface charge-charge interactions in proteins. Comparison of experimental results with calculations from a theoretical method. J Mol Biol. 1993 Jul 20;232(2):574–583. doi: 10.1006/jmbi.1993.1412. [DOI] [PubMed] [Google Scholar]
- Marqusee S., Robbins V. H., Baldwin R. L. Unusually stable helix formation in short alanine-based peptides. Proc Natl Acad Sci U S A. 1989 Jul;86(14):5286–5290. doi: 10.1073/pnas.86.14.5286. [DOI] [PMC free article] [PubMed] [Google Scholar]
- Miick S. M., Martinez G. V., Fiori W. R., Todd A. P., Millhauser G. L. Short alanine-based peptides may form 3(10)-helices and not alpha-helices in aqueous solution. Nature. 1992 Oct 15;359(6396):653–655. doi: 10.1038/359653a0. [DOI] [PubMed] [Google Scholar]
- Némethy G., Scheraga H. A. Protein folding. Q Rev Biophys. 1977 Aug;10(3):239–252. doi: 10.1017/s0033583500002936. [DOI] [PubMed] [Google Scholar]
- Okamoto Y. Helix-forming tendencies of nonpolar amino acids predicted by Monte Carlo simulated annealing. Proteins. 1994 May;19(1):14–23. doi: 10.1002/prot.340190104. [DOI] [PubMed] [Google Scholar]
- Ooi T., Oobatake M. Prediction of the thermodynamics of protein unfolding: the helix-coil transition of poly(L-alanine). Proc Natl Acad Sci U S A. 1991 Apr 1;88(7):2859–2863. doi: 10.1073/pnas.88.7.2859. [DOI] [PMC free article] [PubMed] [Google Scholar]
- Pashley R. M., McGuiggan P. M., Ninham B. W., Evans D. F. Attractive forces between uncharged hydrophobic surfaces: direct measurements in aqueous solution. Science. 1985 Sep 13;229(4718):1088–1089. doi: 10.1126/science.4035349. [DOI] [PubMed] [Google Scholar]
- Ripoll D. R., Scheraga H. A. On the multiple-minima problem in the conformational analysis of polypeptides. II. An electrostatically driven Monte Carlo method--tests on poly(L-alanine). Biopolymers. 1988 Aug;27(8):1283–1303. doi: 10.1002/bip.360270808. [DOI] [PubMed] [Google Scholar]
- Rose G. D., Wolfenden R. Hydrogen bonding, hydrophobicity, packing, and protein folding. Annu Rev Biophys Biomol Struct. 1993;22:381–415. doi: 10.1146/annurev.bb.22.060193.002121. [DOI] [PubMed] [Google Scholar]
- Scholtz J. M., Baldwin R. L. The mechanism of alpha-helix formation by peptides. Annu Rev Biophys Biomol Struct. 1992;21:95–118. doi: 10.1146/annurev.bb.21.060192.000523. [DOI] [PubMed] [Google Scholar]
- Scholtz J. M., Marqusee S., Baldwin R. L., York E. J., Stewart J. M., Santoro M., Bolen D. W. Calorimetric determination of the enthalpy change for the alpha-helix to coil transition of an alanine peptide in water. Proc Natl Acad Sci U S A. 1991 Apr 1;88(7):2854–2858. doi: 10.1073/pnas.88.7.2854. [DOI] [PMC free article] [PubMed] [Google Scholar]
- Sharp K. A., Nicholls A., Friedman R., Honig B. Extracting hydrophobic free energies from experimental data: relationship to protein folding and theoretical models. Biochemistry. 1991 Oct 8;30(40):9686–9697. doi: 10.1021/bi00104a017. [DOI] [PubMed] [Google Scholar]
- Sikorski A., Skolnick J. Monte Carlo simulation of equilibrium globular protein folding: alpha-helical bundles with long loops. Proc Natl Acad Sci U S A. 1989 Apr;86(8):2668–2672. doi: 10.1073/pnas.86.8.2668. [DOI] [PMC free article] [PubMed] [Google Scholar]
- Skolnick J., Kolinski A., Yaris R. Dynamic Monte Carlo study of the folding of a six-stranded Greek key globular protein. Proc Natl Acad Sci U S A. 1989 Feb;86(4):1229–1233. doi: 10.1073/pnas.86.4.1229. [DOI] [PMC free article] [PubMed] [Google Scholar]
- Skolnick J., Kolinski A., Yaris R. Monte Carlo simulations of the folding of beta-barrel globular proteins. Proc Natl Acad Sci U S A. 1988 Jul;85(14):5057–5061. doi: 10.1073/pnas.85.14.5057. [DOI] [PMC free article] [PubMed] [Google Scholar]
- Sundaralingam M., Sekharudu Y. C. Water-inserted alpha-helical segments implicate reverse turns as folding intermediates. Science. 1989 Jun 16;244(4910):1333–1337. doi: 10.1126/science.2734612. [DOI] [PubMed] [Google Scholar]
- Sung S. S. Helix folding simulations with various initial conformations. Biophys J. 1994 Jun;66(6):1796–1803. doi: 10.1016/S0006-3495(94)80973-7. [DOI] [PMC free article] [PubMed] [Google Scholar]
- Tirado-Rives J., Jorgensen W. L. Molecular dynamics simulations of the unfolding of an alpha-helical analogue of ribonuclease A S-peptide in water. Biochemistry. 1991 Apr 23;30(16):3864–3871. doi: 10.1021/bi00230a009. [DOI] [PubMed] [Google Scholar]
- Tobias D. J., Brooks C. L., 3rd Thermodynamics and mechanism of alpha helix initiation in alanine and valine peptides. Biochemistry. 1991 Jun 18;30(24):6059–6070. doi: 10.1021/bi00238a033. [DOI] [PubMed] [Google Scholar]
- Vila J., Williams R. L., Grant J. A., Wójcik J., Scheraga H. A. The intrinsic helix-forming tendency of L-alanine. Proc Natl Acad Sci U S A. 1992 Aug 15;89(16):7821–7825. doi: 10.1073/pnas.89.16.7821. [DOI] [PMC free article] [PubMed] [Google Scholar]
- Vila J., Williams R. L., Vásquez M., Scheraga H. A. Empirical solvation models can be used to differentiate native from near-native conformations of bovine pancreatic trypsin inhibitor. Proteins. 1991;10(3):199–218. doi: 10.1002/prot.340100305. [DOI] [PubMed] [Google Scholar]
- Warshel A., Aqvist J. Electrostatic energy and macromolecular function. Annu Rev Biophys Biophys Chem. 1991;20:267–298. doi: 10.1146/annurev.bb.20.060191.001411. [DOI] [PubMed] [Google Scholar]
- Widom B. Intermolecular Forces and the Nature of the Liquid State: Liquids reflect in their bulk properties the attractions and repulsions of their constituent molecules. Science. 1967 Jul 28;157(3787):375–382. doi: 10.1126/science.157.3787.375. [DOI] [PubMed] [Google Scholar]
- Wilson S. R., Cui W. L. Applications of simulated annealing to peptides. Biopolymers. 1990 Jan;29(1):225–235. doi: 10.1002/bip.360290127. [DOI] [PubMed] [Google Scholar]
- Wodak S. J., Janin J. Analytical approximation to the accessible surface area of proteins. Proc Natl Acad Sci U S A. 1980 Apr;77(4):1736–1740. doi: 10.1073/pnas.77.4.1736. [DOI] [PMC free article] [PubMed] [Google Scholar]
- Zimmerman S. S., Scheraga H. A. Local interactions in bends of proteins. Proc Natl Acad Sci U S A. 1977 Oct;74(10):4126–4129. doi: 10.1073/pnas.74.10.4126. [DOI] [PMC free article] [PubMed] [Google Scholar]
- van Gunsteren W. F., Luque F. J., Timms D., Torda A. E. Molecular mechanics in biology: from structure to function, taking account of solvation. Annu Rev Biophys Biomol Struct. 1994;23:847–863. doi: 10.1146/annurev.bb.23.060194.004215. [DOI] [PubMed] [Google Scholar]