Abstract
The structural and thermodynamic factors responsible for the singly and doubly occupied saturation states of the gramicidin channel are investigated with molecular dynamics simulations and free energy perturbation methods. The relative free energy of binding of all of the five common cations Li+, Na+, K+, Rb+, and Cs+ is calculated in the singly and doubly occupied channel and in bulk water. The atomic system, which includes the gramicidin channel, a model membrane made of neutral Lennard-Jones particles and 190 explicit water molecules to form the bulk region, is similar to the one used in previous work to calculate the free energy profile of a Na+ ion along the axis of the channel. In all of the calculations, the ions are positioned in the main binding sites located near the entrances of the channel. The calculations reveal that the doubly occupied state is relatively more favorable for the larger ions. Thermodynamic decomposition is used to show that the origin of the trend observed in the calculations is due to the loss of favorable interactions between the ion and the single file water molecules inside the channel. Small ions are better solvated by the internal water molecules in the singly occupied state than in the doubly occupied state; bigger ions are solvated almost as well in both occupation states. Water-channel interactions play a role in the channel response. The observed trends are related to general thermodynamical properties of electrolyte solutions.
Full text
PDF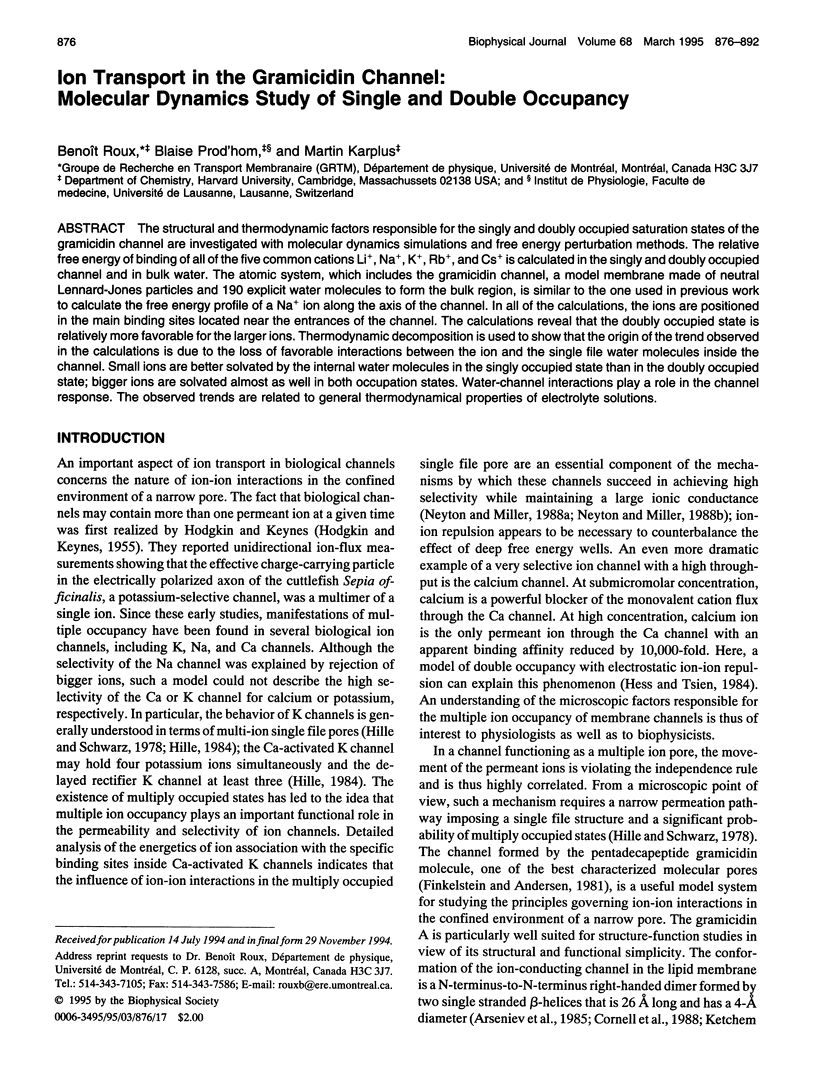
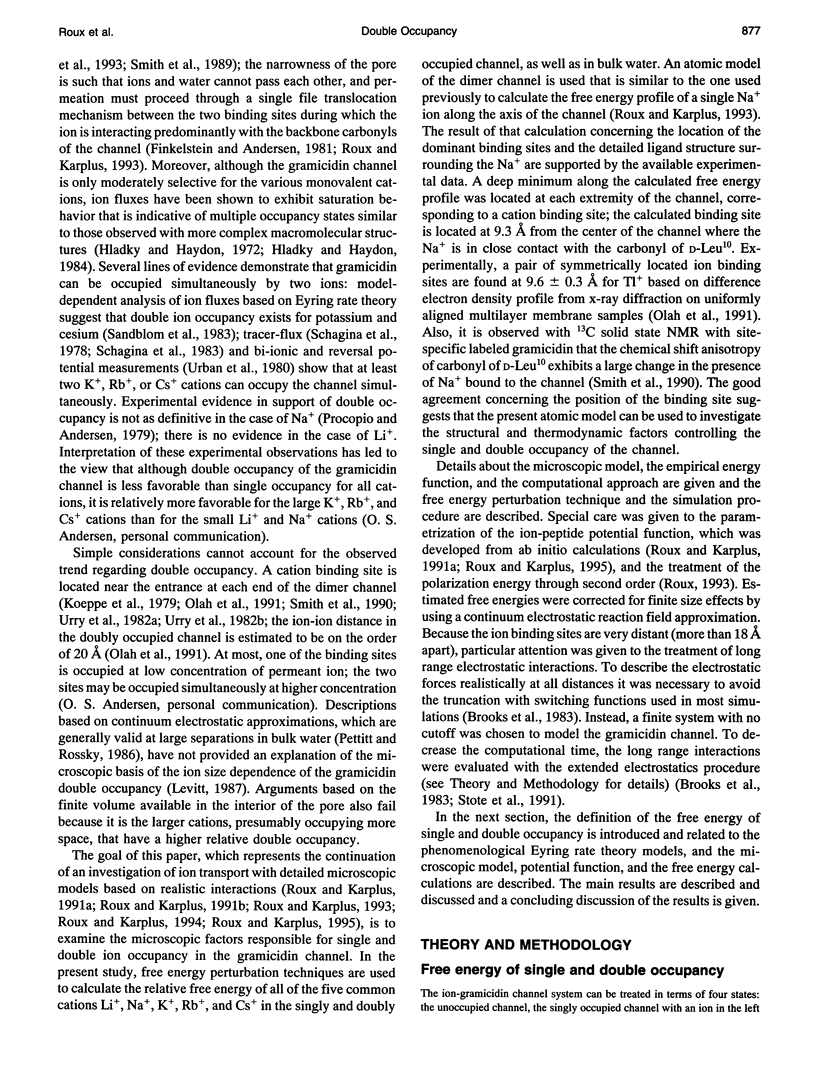
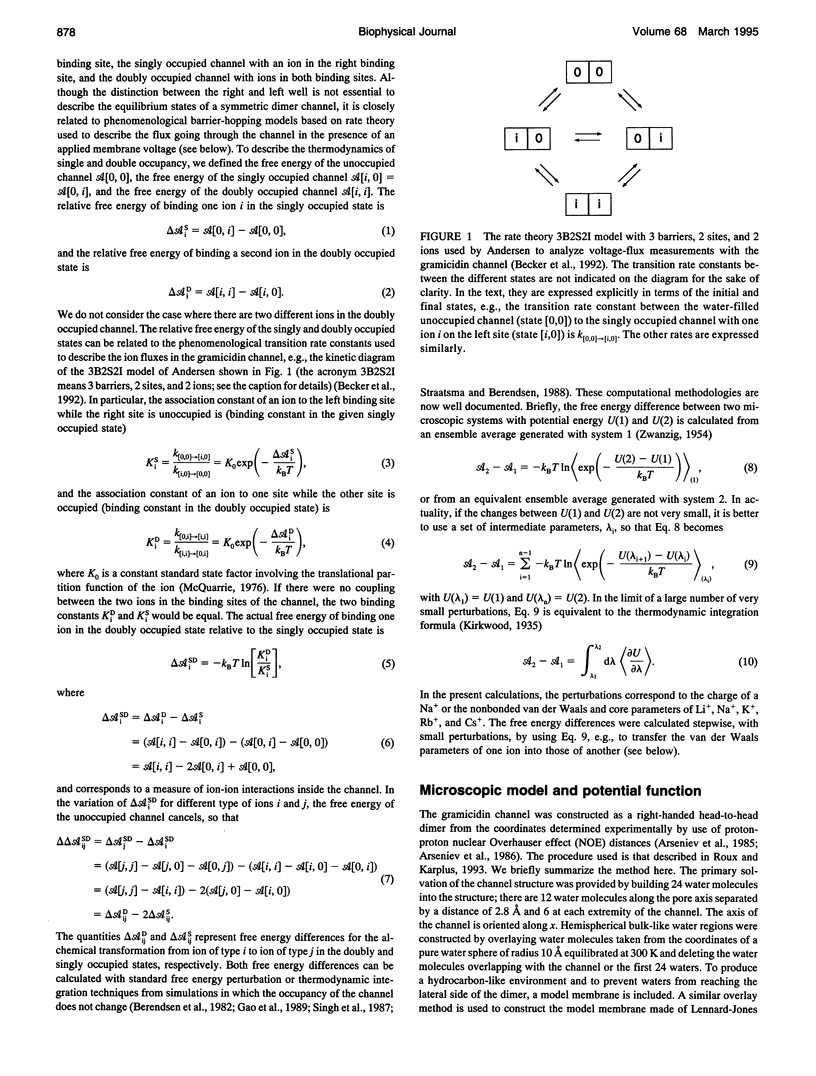
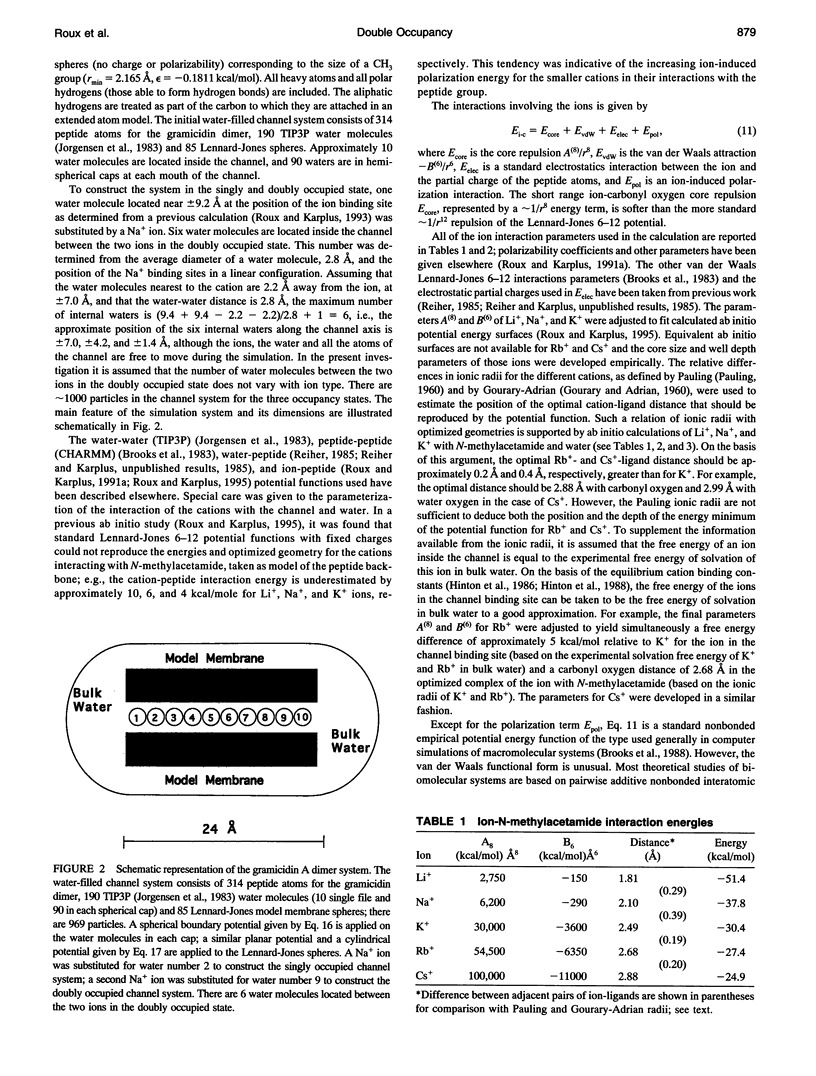
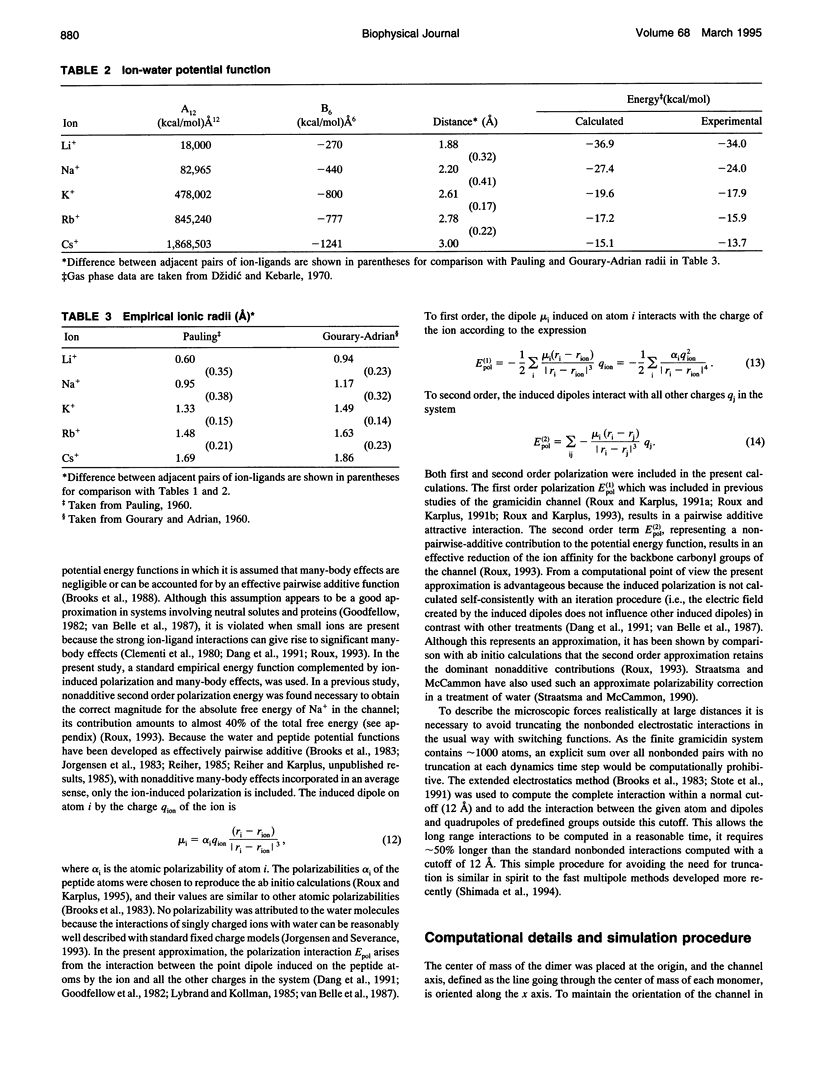
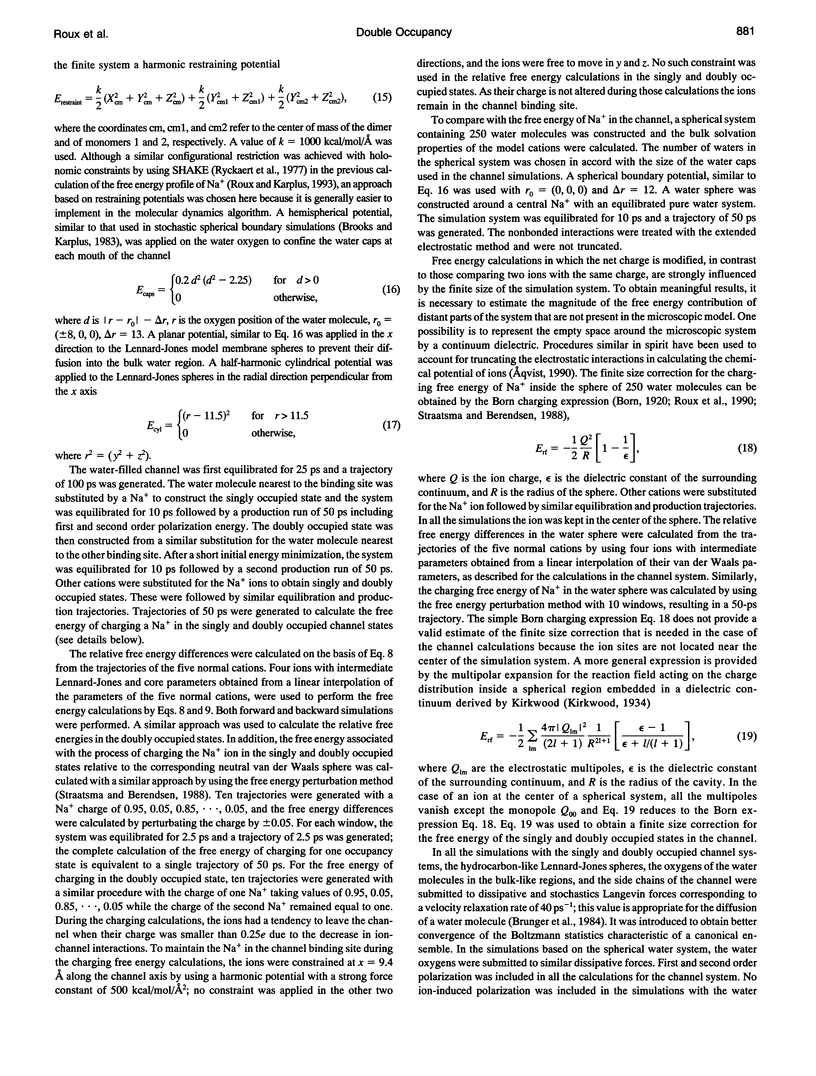
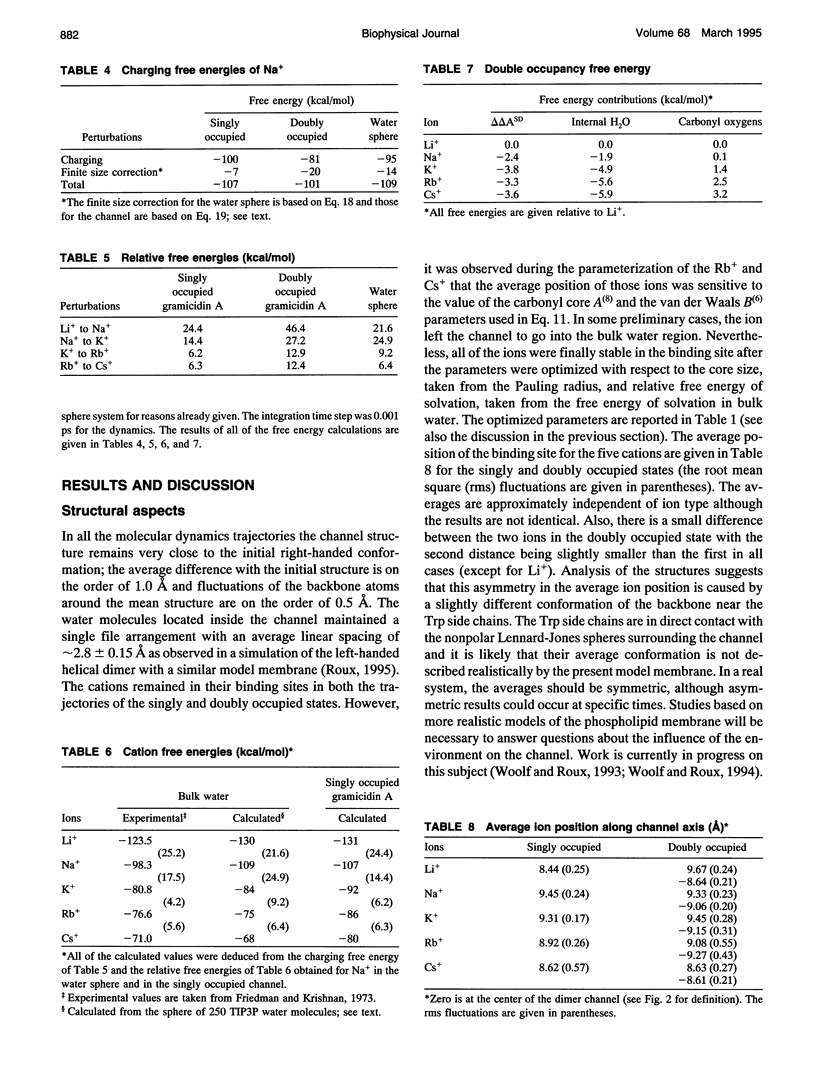
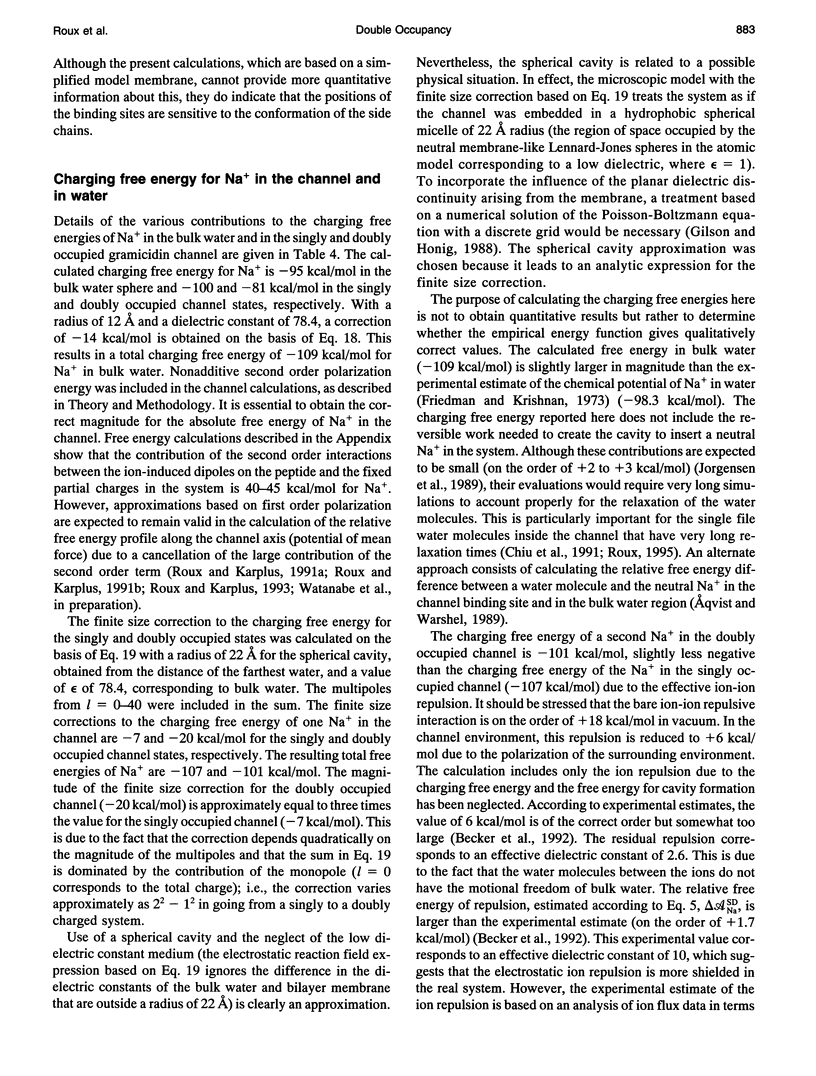
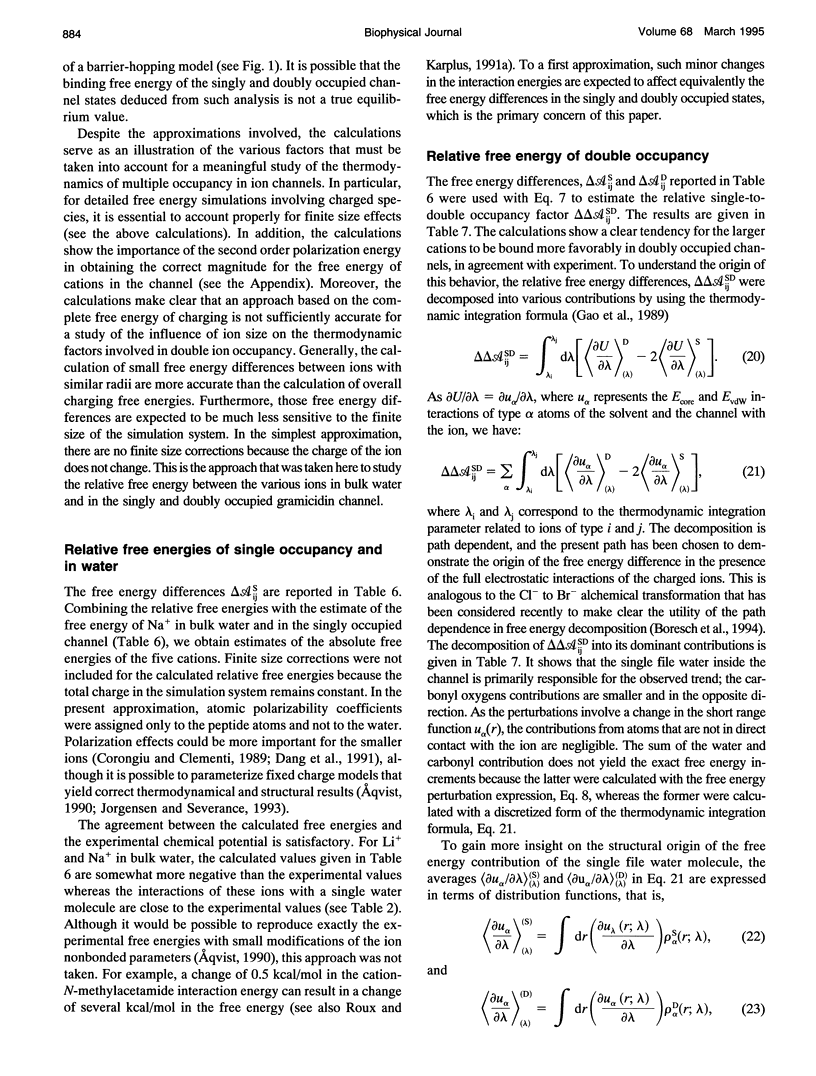
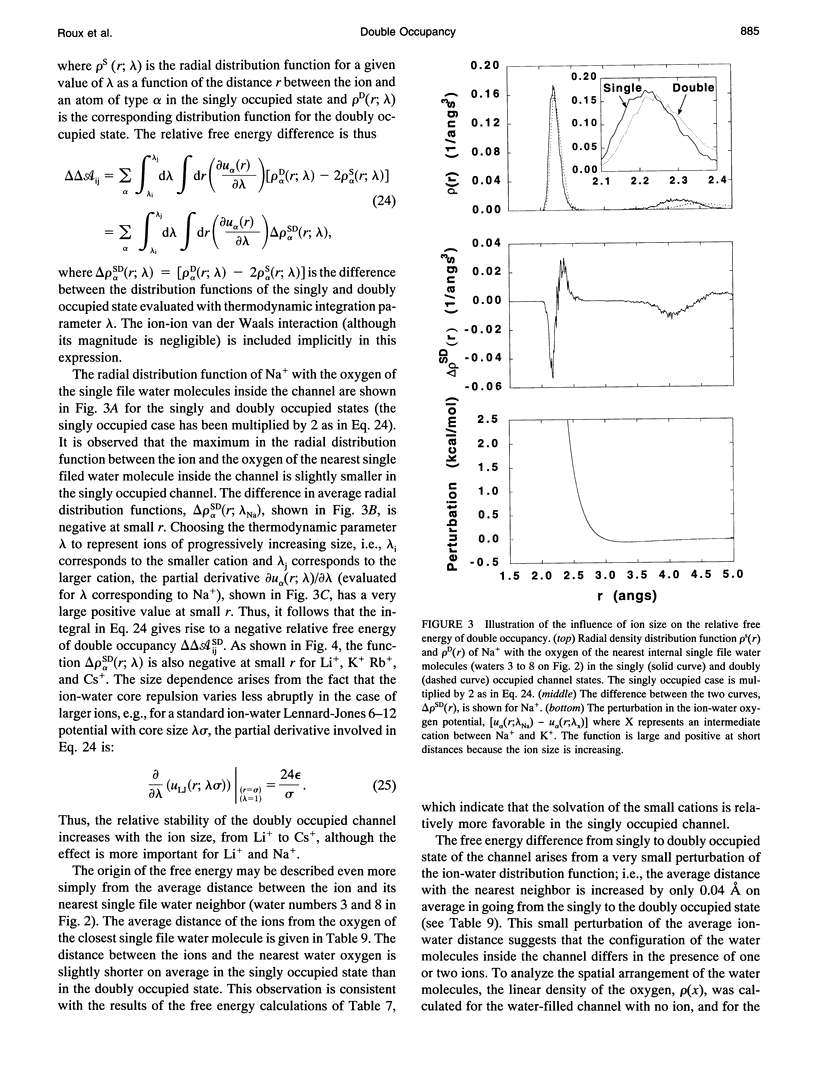
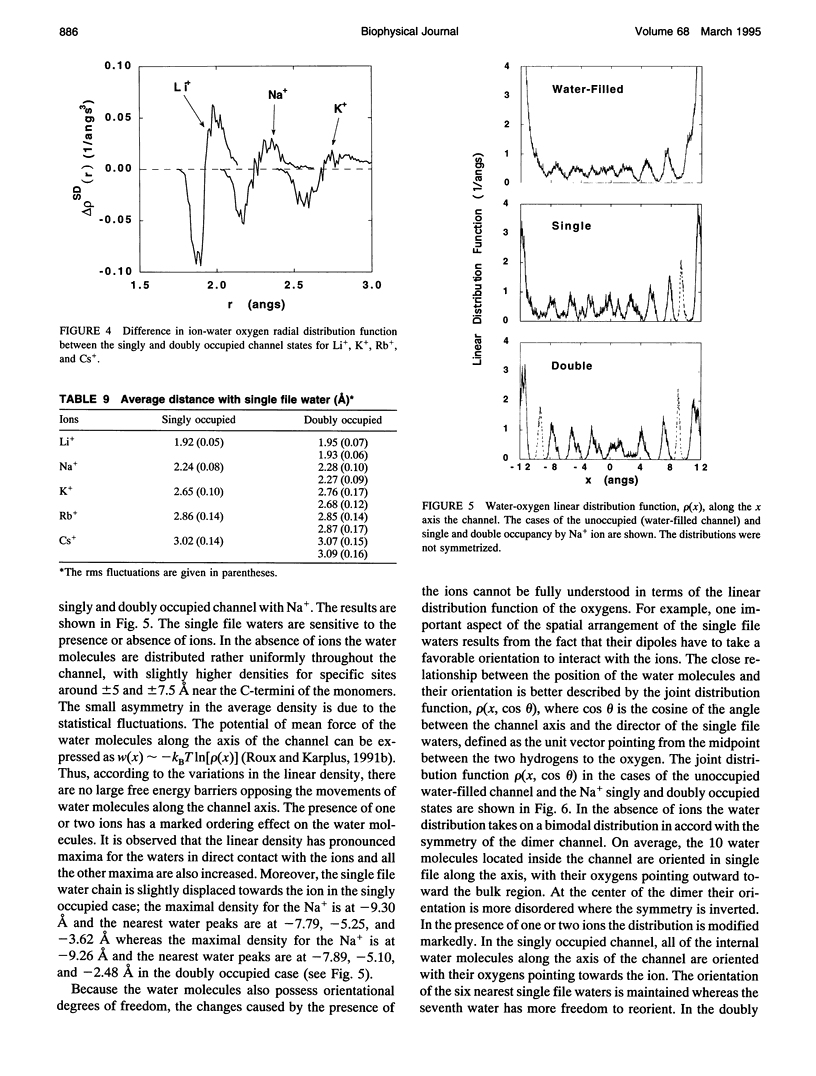
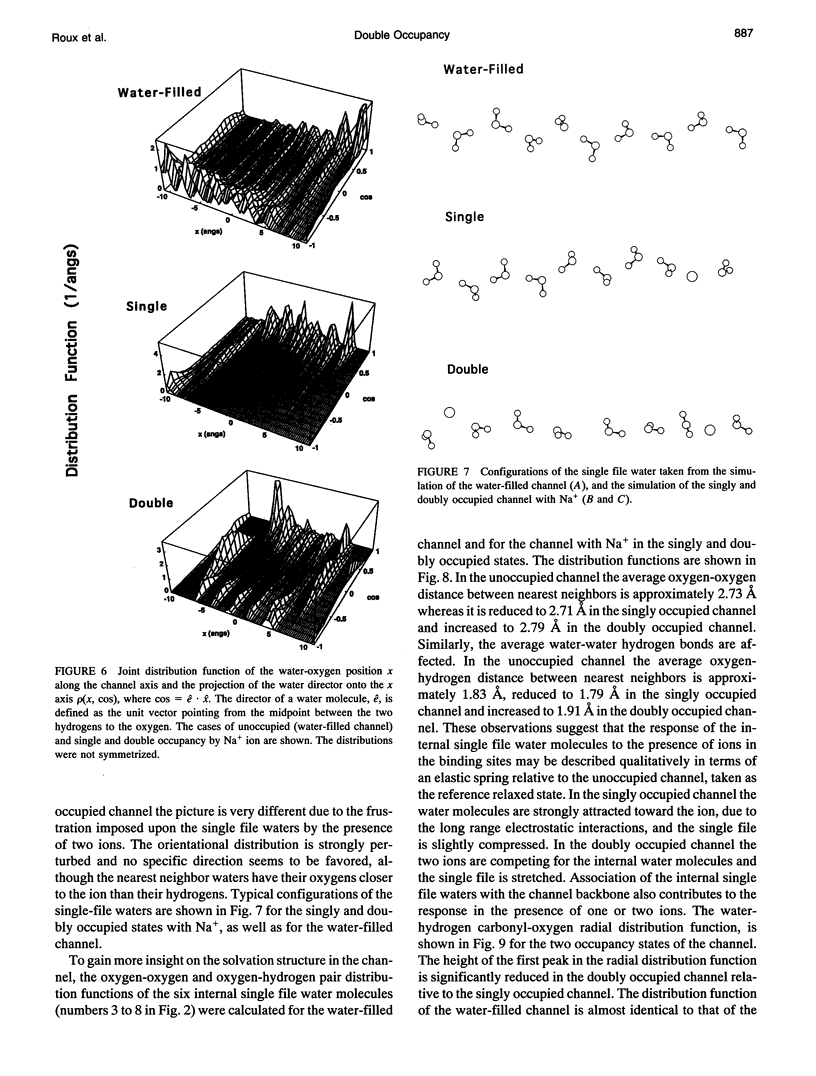
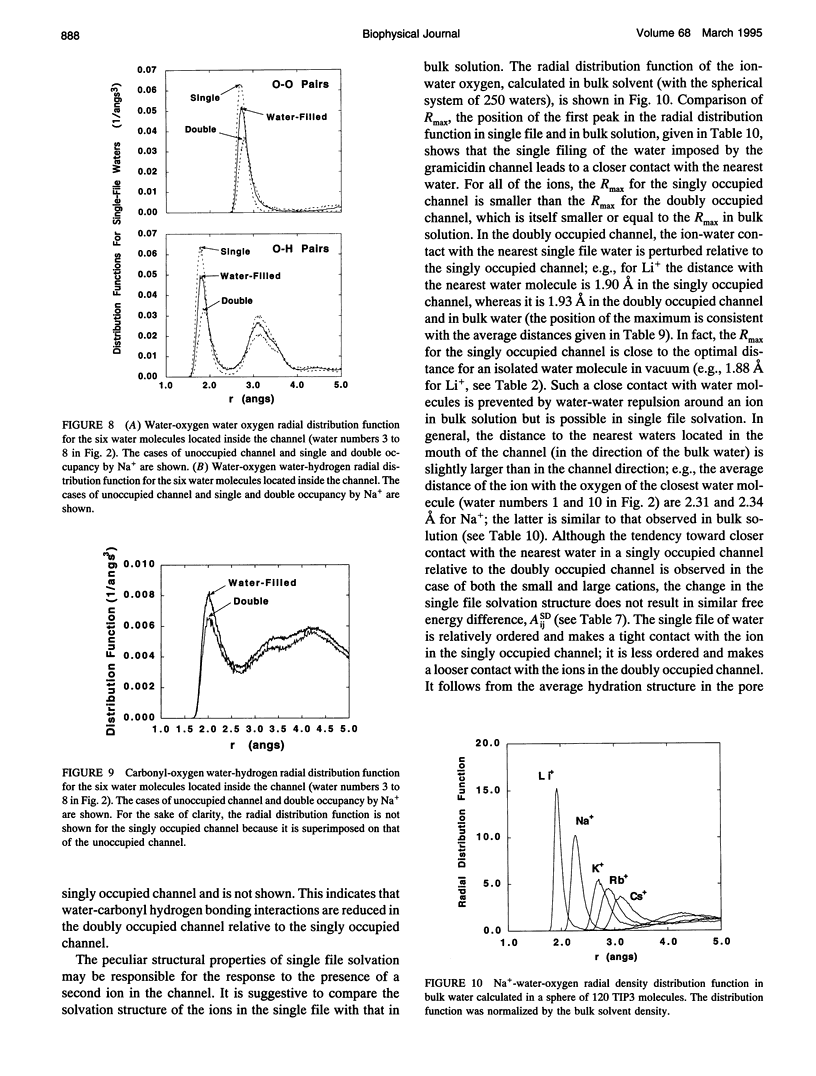
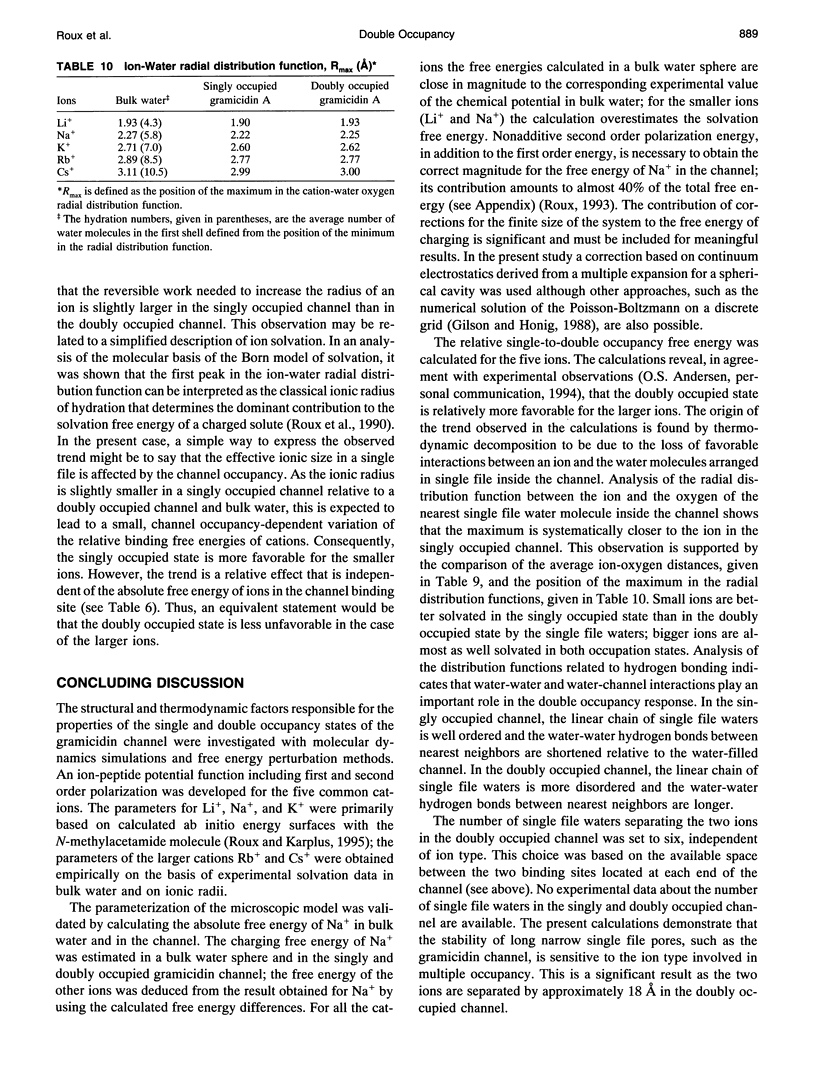
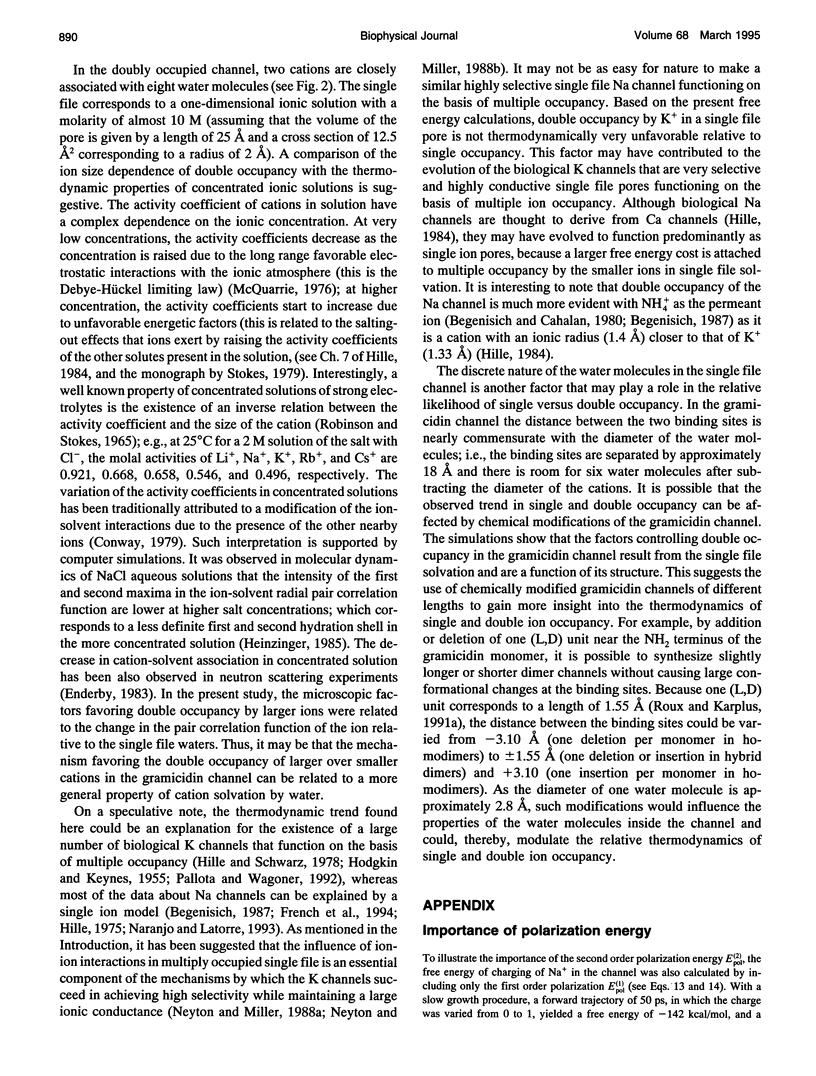
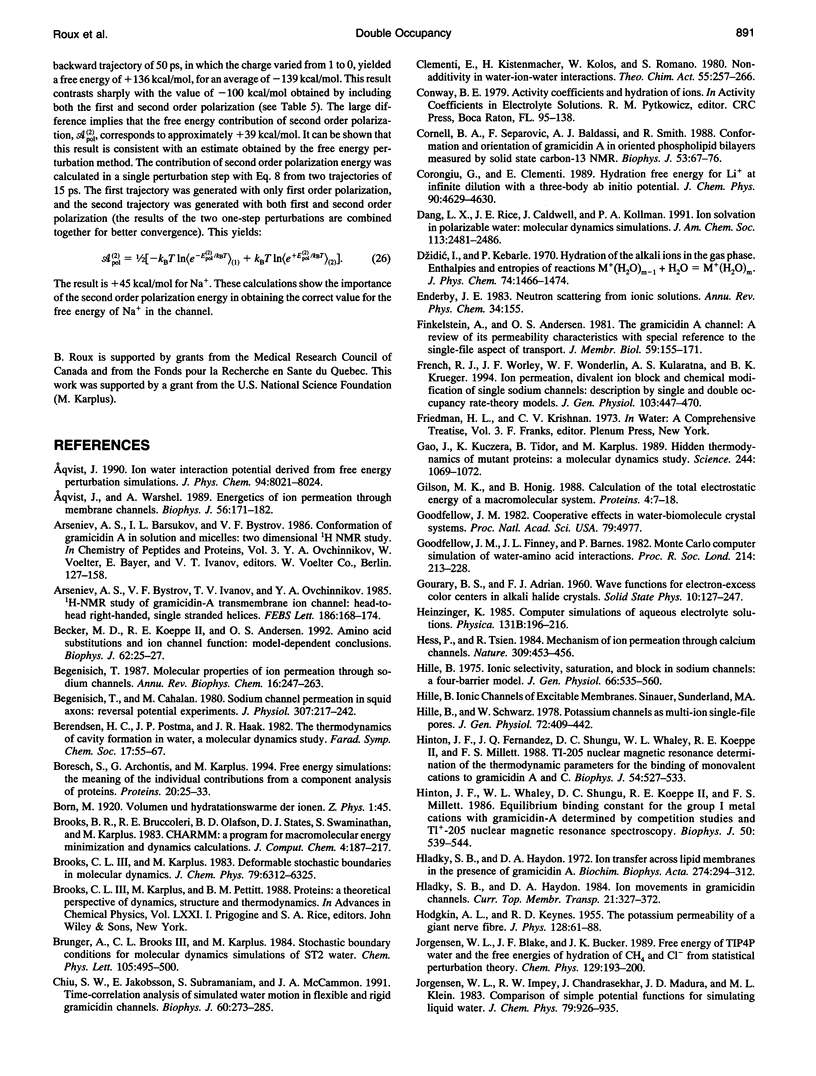
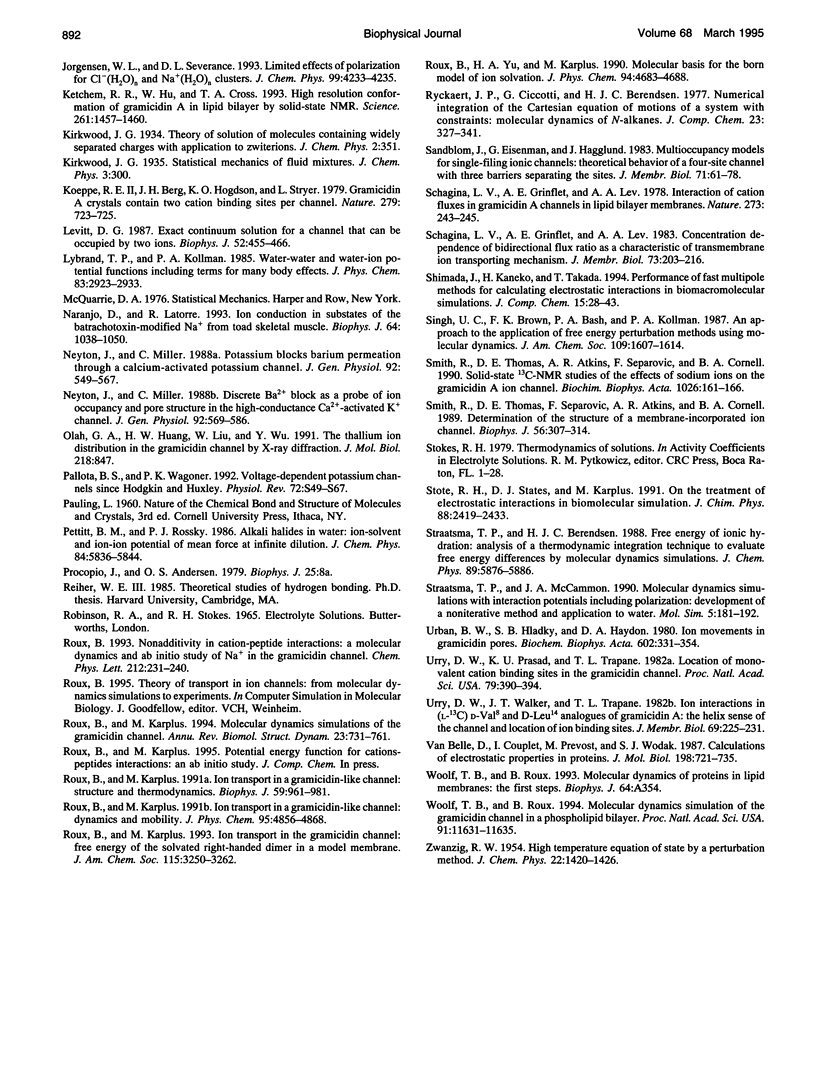
Images in this article
Selected References
These references are in PubMed. This may not be the complete list of references from this article.
- Aqvist J., Warshel A. Energetics of ion permeation through membrane channels. Solvation of Na+ by gramicidin A. Biophys J. 1989 Jul;56(1):171–182. doi: 10.1016/S0006-3495(89)82662-1. [DOI] [PMC free article] [PubMed] [Google Scholar]
- Arseniev A. S., Barsukov I. L., Bystrov V. F., Lomize A. L., Ovchinnikov YuA 1H-NMR study of gramicidin A transmembrane ion channel. Head-to-head right-handed, single-stranded helices. FEBS Lett. 1985 Jul 8;186(2):168–174. doi: 10.1016/0014-5793(85)80702-x. [DOI] [PubMed] [Google Scholar]
- Becker M. D., Koeppe R. E., 2nd, Andersen O. S. Amino acid substitutions and ion channel function. Model-dependent conclusions. Biophys J. 1992 Apr;62(1):25–27. doi: 10.1016/S0006-3495(92)81767-8. [DOI] [PMC free article] [PubMed] [Google Scholar]
- Begenisich T. B., Cahalan M. D. Sodium channel permeation in squid axons. I: Reversal potential experiments. J Physiol. 1980 Oct;307:217–242. doi: 10.1113/jphysiol.1980.sp013432. [DOI] [PMC free article] [PubMed] [Google Scholar]
- Begenisich T. Molecular properties of ion permeation through sodium channels. Annu Rev Biophys Biophys Chem. 1987;16:247–263. doi: 10.1146/annurev.bb.16.060187.001335. [DOI] [PubMed] [Google Scholar]
- Boresch S., Archontis G., Karplus M. Free energy simulations: the meaning of the individual contributions from a component analysis. Proteins. 1994 Sep;20(1):25–33. doi: 10.1002/prot.340200105. [DOI] [PubMed] [Google Scholar]
- Chiu S. W., Jakobsson E., Subramaniam S., McCammon J. A. Time-correlation analysis of simulated water motion in flexible and rigid gramicidin channels. Biophys J. 1991 Jul;60(1):273–285. doi: 10.1016/S0006-3495(91)82049-5. [DOI] [PMC free article] [PubMed] [Google Scholar]
- Cornell B. A., Separovic F., Baldassi A. J., Smith R. Conformation and orientation of gramicidin a in oriented phospholipid bilayers measured by solid state carbon-13 NMR. Biophys J. 1988 Jan;53(1):67–76. doi: 10.1016/S0006-3495(88)83066-2. [DOI] [PMC free article] [PubMed] [Google Scholar]
- Finkelstein A., Andersen O. S. The gramicidin A channel: a review of its permeability characteristics with special reference to the single-file aspect of transport. J Membr Biol. 1981 Apr 30;59(3):155–171. doi: 10.1007/BF01875422. [DOI] [PubMed] [Google Scholar]
- French R. J., Worley J. F., 3rd, Wonderlin W. F., Kularatna A. S., Krueger B. K. Ion permeation, divalent ion block, and chemical modification of single sodium channels. Description by single- and double-occupancy rate-theory models. J Gen Physiol. 1994 Mar;103(3):447–470. doi: 10.1085/jgp.103.3.447. [DOI] [PMC free article] [PubMed] [Google Scholar]
- Gao J., Kuczera K., Tidor B., Karplus M. Hidden thermodynamics of mutant proteins: a molecular dynamics analysis. Science. 1989 Jun 2;244(4908):1069–1072. doi: 10.1126/science.2727695. [DOI] [PubMed] [Google Scholar]
- Gilson M. K., Honig B. Calculation of the total electrostatic energy of a macromolecular system: solvation energies, binding energies, and conformational analysis. Proteins. 1988;4(1):7–18. doi: 10.1002/prot.340040104. [DOI] [PubMed] [Google Scholar]
- Goodfellow J. M. Cooperative effects in water-biomolecule crystal systems. Proc Natl Acad Sci U S A. 1982 Aug;79(16):4977–4979. doi: 10.1073/pnas.79.16.4977. [DOI] [PMC free article] [PubMed] [Google Scholar]
- Goodfellow J. M., Finney J. L., Barnes P. Monte Carlo computer simulation of water-amino acid interactions. Proc R Soc Lond B Biol Sci. 1982 Jan 22;214(1195):213–228. doi: 10.1098/rspb.1982.0005. [DOI] [PubMed] [Google Scholar]
- HODGKIN A. L., KEYNES R. D. The potassium permeability of a giant nerve fibre. J Physiol. 1955 Apr 28;128(1):61–88. doi: 10.1113/jphysiol.1955.sp005291. [DOI] [PMC free article] [PubMed] [Google Scholar]
- Hess P., Tsien R. W. Mechanism of ion permeation through calcium channels. 1984 May 31-Jun 6Nature. 309(5967):453–456. doi: 10.1038/309453a0. [DOI] [PubMed] [Google Scholar]
- Hille B. Ionic selectivity, saturation, and block in sodium channels. A four-barrier model. J Gen Physiol. 1975 Nov;66(5):535–560. doi: 10.1085/jgp.66.5.535. [DOI] [PMC free article] [PubMed] [Google Scholar]
- Hille B., Schwarz W. Potassium channels as multi-ion single-file pores. J Gen Physiol. 1978 Oct;72(4):409–442. doi: 10.1085/jgp.72.4.409. [DOI] [PMC free article] [PubMed] [Google Scholar]
- Hinton J. F., Fernandez J. Q., Shungu D. C., Whaley W. L., Koeppe R. E., 2nd, Millett F. S. TI-205 nuclear magnetic resonance determination of the thermodynamic parameters for the binding of monovalent cations to gramicidins A and C. Biophys J. 1988 Sep;54(3):527–533. doi: 10.1016/S0006-3495(88)82985-0. [DOI] [PMC free article] [PubMed] [Google Scholar]
- Hinton J. F., Whaley W. L., Shungu D., Koeppe R. E., 2nd, Millett F. S. Equilibrium binding constants for the group I metal cations with gramicidin-A determined by competition studies and T1+-205 nuclear magnetic resonance spectroscopy. Biophys J. 1986 Sep;50(3):539–544. doi: 10.1016/S0006-3495(86)83492-0. [DOI] [PMC free article] [PubMed] [Google Scholar]
- Hladky S. B., Haydon D. A. Ion transfer across lipid membranes in the presence of gramicidin A. I. Studies of the unit conductance channel. Biochim Biophys Acta. 1972 Aug 9;274(2):294–312. doi: 10.1016/0005-2736(72)90178-2. [DOI] [PubMed] [Google Scholar]
- Ketchem R. R., Hu W., Cross T. A. High-resolution conformation of gramicidin A in a lipid bilayer by solid-state NMR. Science. 1993 Sep 10;261(5127):1457–1460. doi: 10.1126/science.7690158. [DOI] [PubMed] [Google Scholar]
- Koeppe R. E., 2nd, Berg J. M., Hodgson K. O., Stryer L. Gramicidin A crystals contain two cation binding sites per channel. Nature. 1979 Jun 21;279(5715):723–725. doi: 10.1038/279723a0. [DOI] [PubMed] [Google Scholar]
- Levitt D. G. Exact continuum solution for a channel that can be occupied by two ions. Biophys J. 1987 Sep;52(3):455–466. doi: 10.1016/S0006-3495(87)83234-4. [DOI] [PMC free article] [PubMed] [Google Scholar]
- Naranjo D., Latorre R. Ion conduction in substates of the batrachotoxin-modified Na+ channel from toad skeletal muscle. Biophys J. 1993 Apr;64(4):1038–1050. doi: 10.1016/S0006-3495(93)81469-3. [DOI] [PMC free article] [PubMed] [Google Scholar]
- Neyton J., Miller C. Discrete Ba2+ block as a probe of ion occupancy and pore structure in the high-conductance Ca2+ -activated K+ channel. J Gen Physiol. 1988 Nov;92(5):569–586. doi: 10.1085/jgp.92.5.569. [DOI] [PMC free article] [PubMed] [Google Scholar]
- Neyton J., Miller C. Potassium blocks barium permeation through a calcium-activated potassium channel. J Gen Physiol. 1988 Nov;92(5):549–567. doi: 10.1085/jgp.92.5.549. [DOI] [PMC free article] [PubMed] [Google Scholar]
- Olah G. A., Huang H. W., Liu W. H., Wu Y. L. Location of ion-binding sites in the gramicidin channel by X-ray diffraction. J Mol Biol. 1991 Apr 20;218(4):847–858. doi: 10.1016/0022-2836(91)90272-8. [DOI] [PubMed] [Google Scholar]
- Pallotta B. S., Wagoner P. K. Voltage-dependent potassium channels since Hodgkin and Huxley. Physiol Rev. 1992 Oct;72(4 Suppl):S49–S67. doi: 10.1152/physrev.1992.72.suppl_4.S49. [DOI] [PubMed] [Google Scholar]
- Roux B., Karplus M. Ion transport in a model gramicidin channel. Structure and thermodynamics. Biophys J. 1991 May;59(5):961–981. doi: 10.1016/S0006-3495(91)82311-6. [DOI] [PMC free article] [PubMed] [Google Scholar]
- Roux B., Karplus M. Molecular dynamics simulations of the gramicidin channel. Annu Rev Biophys Biomol Struct. 1994;23:731–761. doi: 10.1146/annurev.bb.23.060194.003503. [DOI] [PubMed] [Google Scholar]
- Sandblom J., Eisenman G., Hägglund J. Multioccupancy models for single filing ionic channels: theoretical behavior of a four-site channel with three barriers separating the sites. J Membr Biol. 1983;71(1-2):61–78. doi: 10.1007/BF01870675. [DOI] [PubMed] [Google Scholar]
- Schagina L. V., Grinfeldt A. E., Lev A. A. Interaction of cation fluxes in gramicidin A channels in lipid bilayer membranes. Nature. 1978 May 18;273(5659):243–245. doi: 10.1038/273243a0. [DOI] [PubMed] [Google Scholar]
- Smith R., Thomas D. E., Atkins A. R., Separovic F., Cornell B. A. Solid-state 13C-NMR studies of the effects of sodium ions on the gramicidin A ion channel. Biochim Biophys Acta. 1990 Jul 24;1026(2):161–166. doi: 10.1016/0005-2736(90)90059-w. [DOI] [PubMed] [Google Scholar]
- Smith R., Thomas D. E., Separovic F., Atkins A. R., Cornell B. A. Determination of the structure of a membrane-incorporated ion channel. Solid-state nuclear magnetic resonance studies of gramicidin A. Biophys J. 1989 Aug;56(2):307–314. doi: 10.1016/S0006-3495(89)82677-3. [DOI] [PMC free article] [PubMed] [Google Scholar]
- Urban B. W., Hladky S. B., Haydon D. A. Ion movements in gramicidin pores. An example of single-file transport. Biochim Biophys Acta. 1980 Nov 4;602(2):331–354. doi: 10.1016/0005-2736(80)90316-8. [DOI] [PubMed] [Google Scholar]
- Urry D. W., Prasad K. U., Trapane T. L. Location of monovalent cation binding sites in the gramicidin channel. Proc Natl Acad Sci U S A. 1982 Jan;79(2):390–394. doi: 10.1073/pnas.79.2.390. [DOI] [PMC free article] [PubMed] [Google Scholar]
- Urry D. W., Walker J. T., Trapane T. L. Ion interactions in (1-13C)D-Val8 and D-Leu14 analogs of gramicidin A, the helix sense of the channel and location of ion binding sites. J Membr Biol. 1982;69(3):225–231. doi: 10.1007/BF01870401. [DOI] [PubMed] [Google Scholar]
- Van Belle D., Couplet I., Prevost M., Wodak S. J. Calculations of electrostatic properties in proteins. Analysis of contributions from induced protein dipoles. J Mol Biol. 1987 Dec 20;198(4):721–735. doi: 10.1016/0022-2836(87)90213-0. [DOI] [PubMed] [Google Scholar]
- Woolf T. B., Roux B. Molecular dynamics simulation of the gramicidin channel in a phospholipid bilayer. Proc Natl Acad Sci U S A. 1994 Nov 22;91(24):11631–11635. doi: 10.1073/pnas.91.24.11631. [DOI] [PMC free article] [PubMed] [Google Scholar]