Abstract
Inward barium current (IBa) through voltage-gated calcium channels was recorded from chick cochlear hair cells using the whole-cell clamp technique. IBa was sensitive to dihydropyridines and insensitive to the peptide toxins omega-agatoxin IVa, omega-conotoxin GVIa, and omega-conotoxin MVIIC. Changing the holding potential over a -40 to -80 mV range had no effect on the time course or magnitude of IBa nor did it reveal any inactivating inward currents. The activation of IBa was modeled with Hodgkin-Huxley m2 kinetics. The time constant of activation, tau m, was 550 microseconds at -30 mV and gradually decreased to 100 microseconds at +50 mV. A Boltzmann fit to the activation curve, m infinity, yielded a half activation voltage of -15 mV and a steepness factor of 7.8 mV. Opening and closing rate constants, alpha m and beta m, were calculated from tau m and m infinity, then fit with modified exponential functions. The H-H model derived by evaluating the exponential functions for alpha m and beta m not only provided an excellent fit to the time course of IBa activation, but was predictive of the time course and magnitude of the IBa tail current. No differences in kinetics or voltage dependence of activation of IBa were found between tall and short hair cells. We conclude that both tall and short hair cells of the chick cochlea predominantly, if not exclusively, express noninactivating L-type calcium channels. These channels are therefore responsible for processes requiring voltage-dependent calcium entry through the basolateral cell membrane, such as transmitter release and activation of Ca(2+)-dependent K+ channels.
Full text
PDF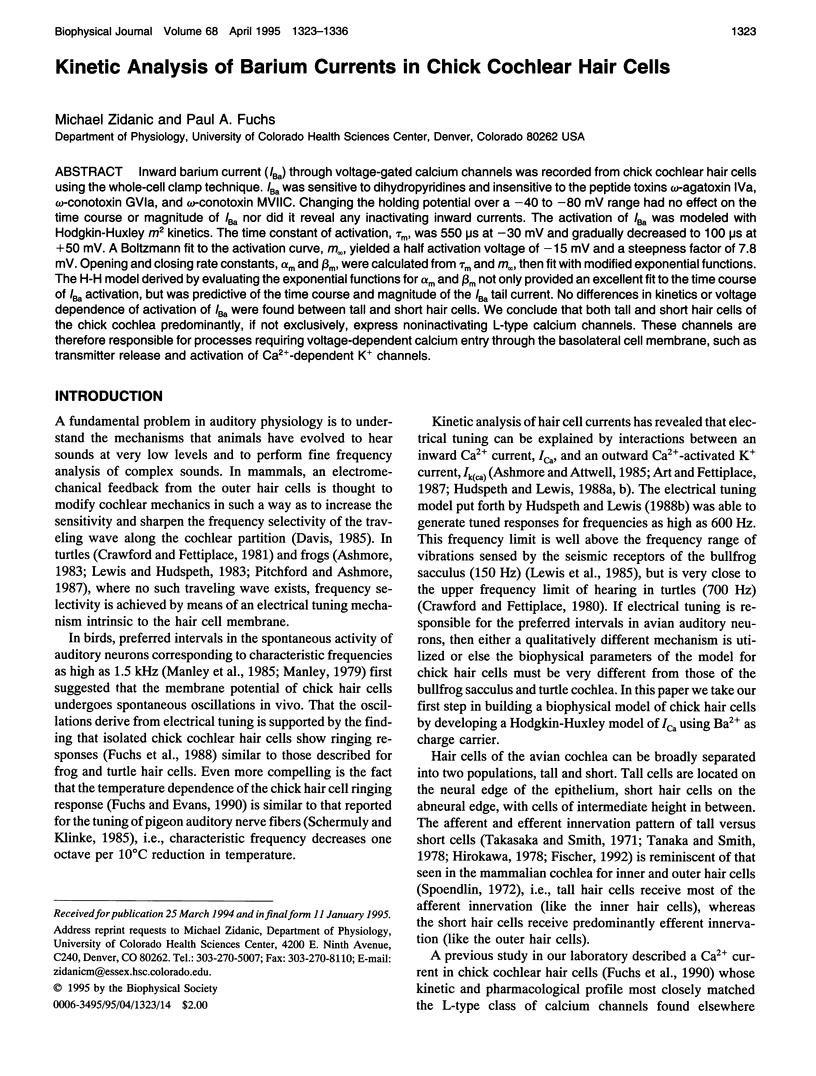
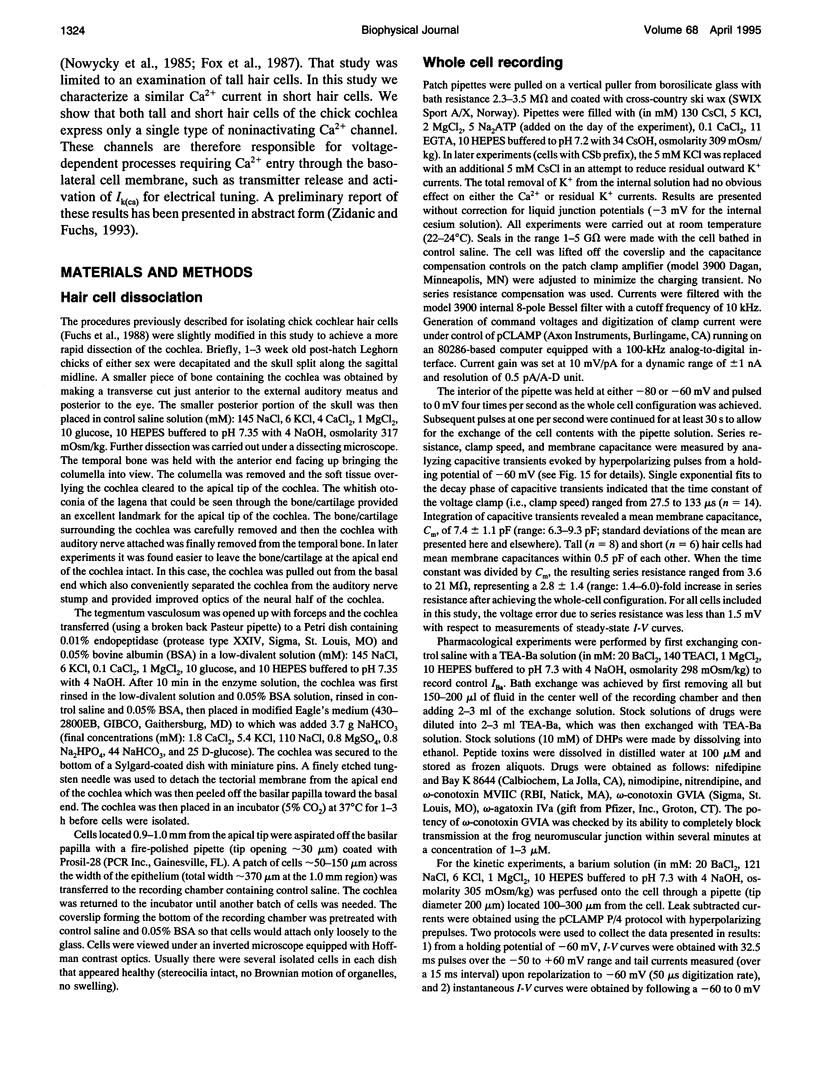
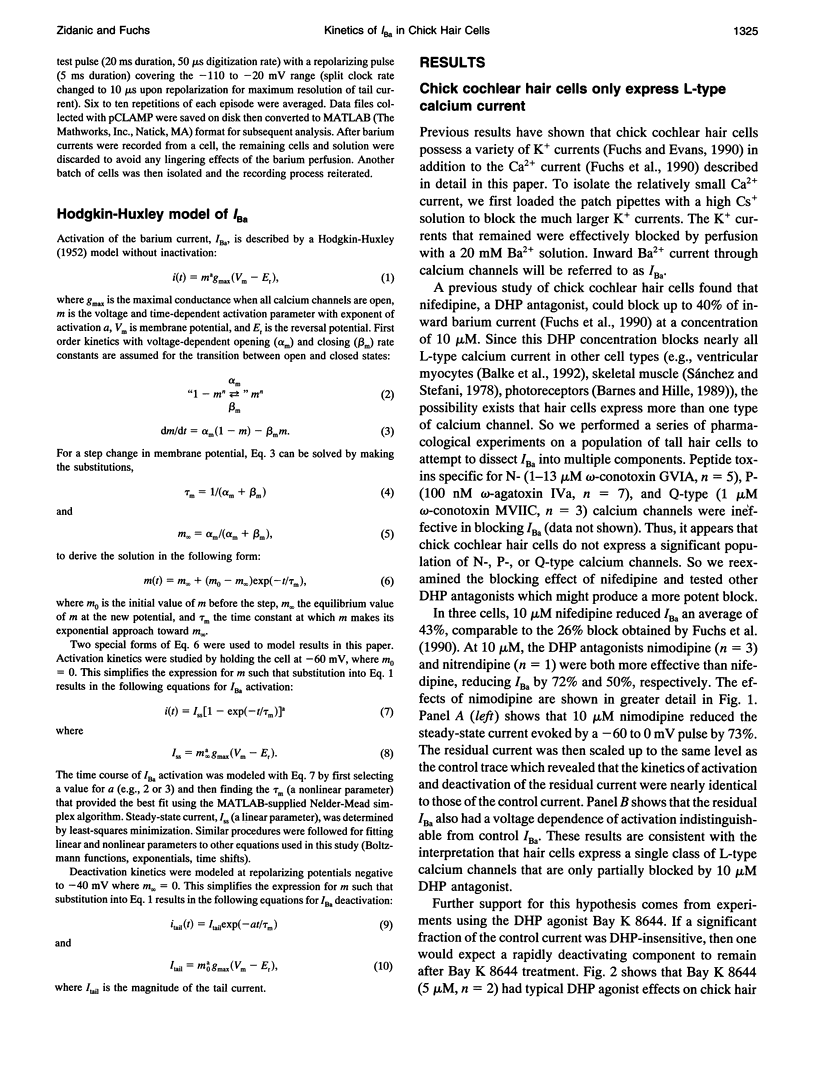
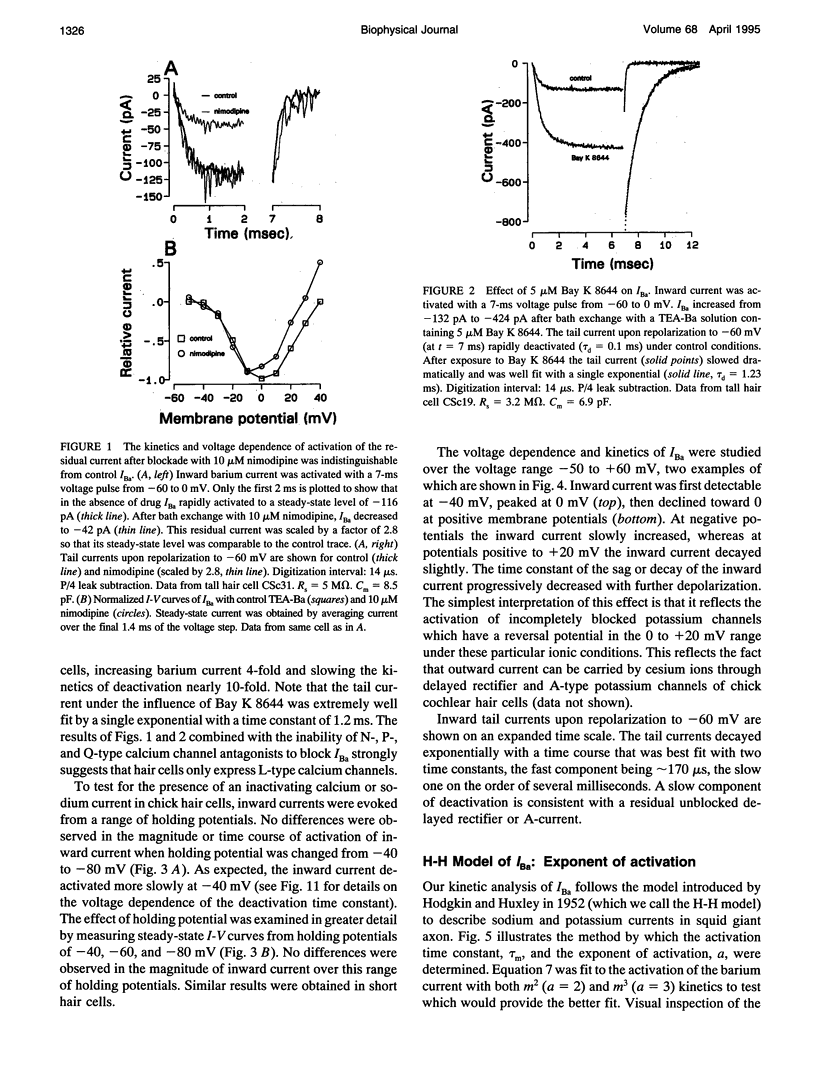
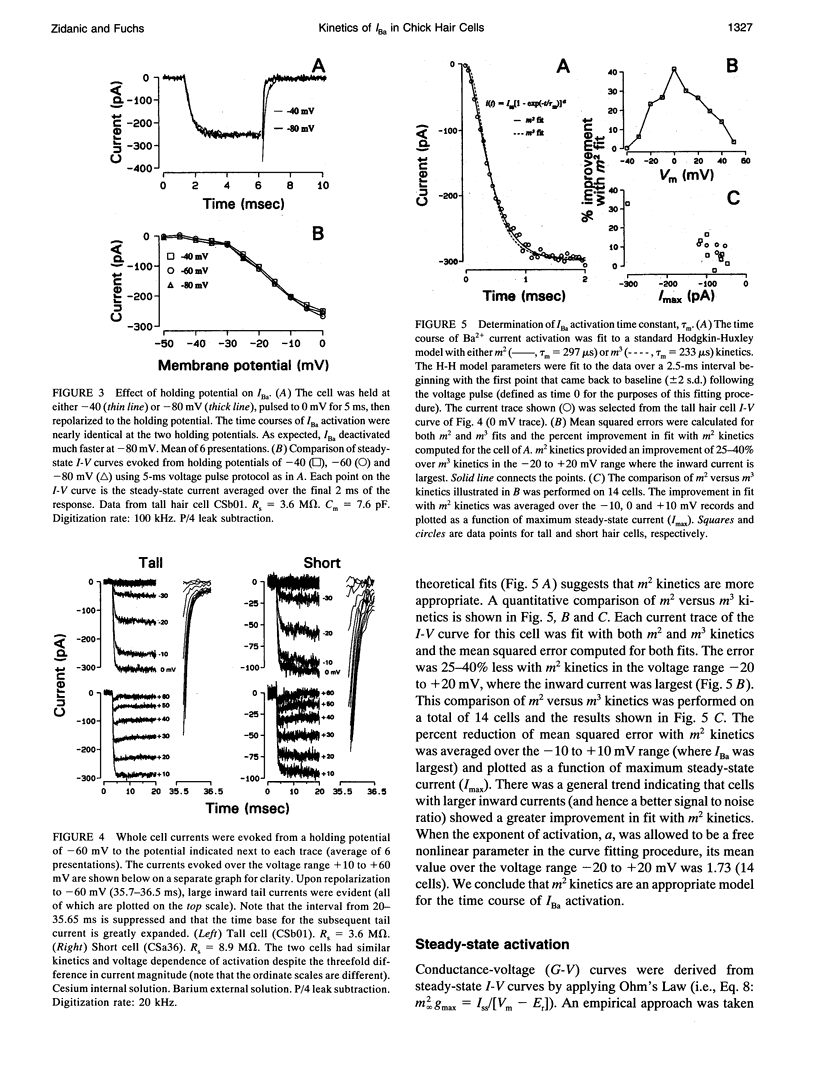
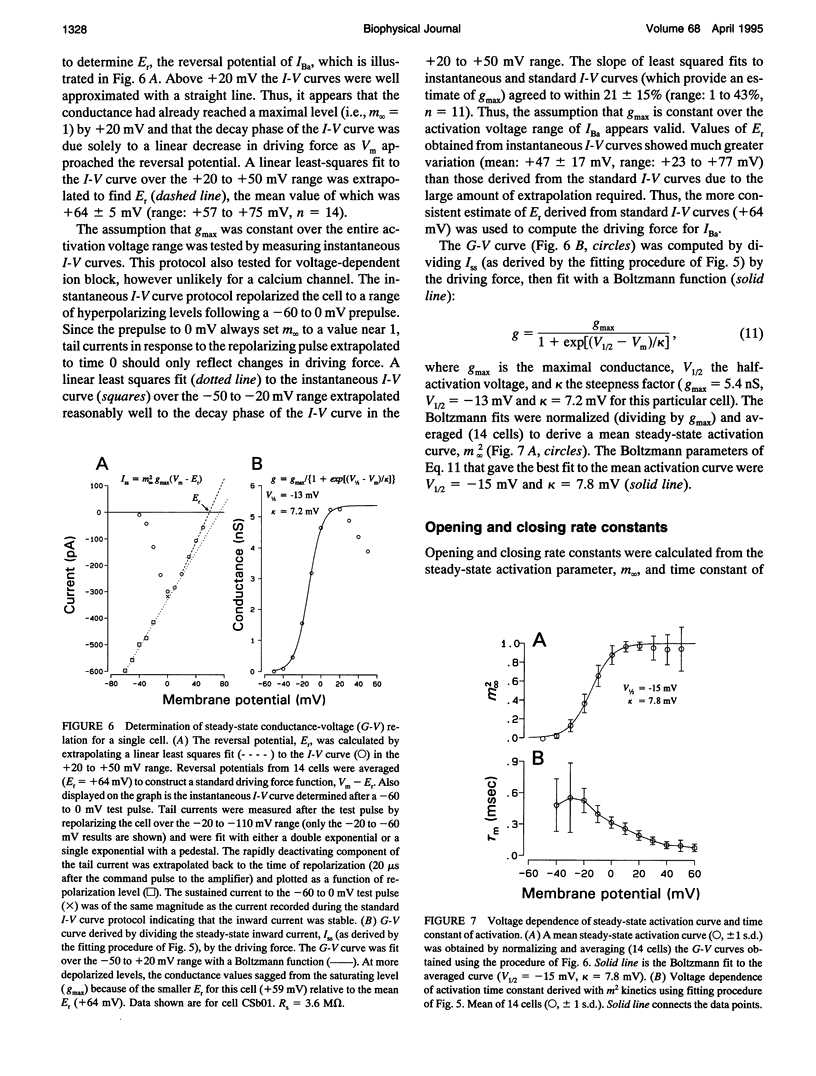
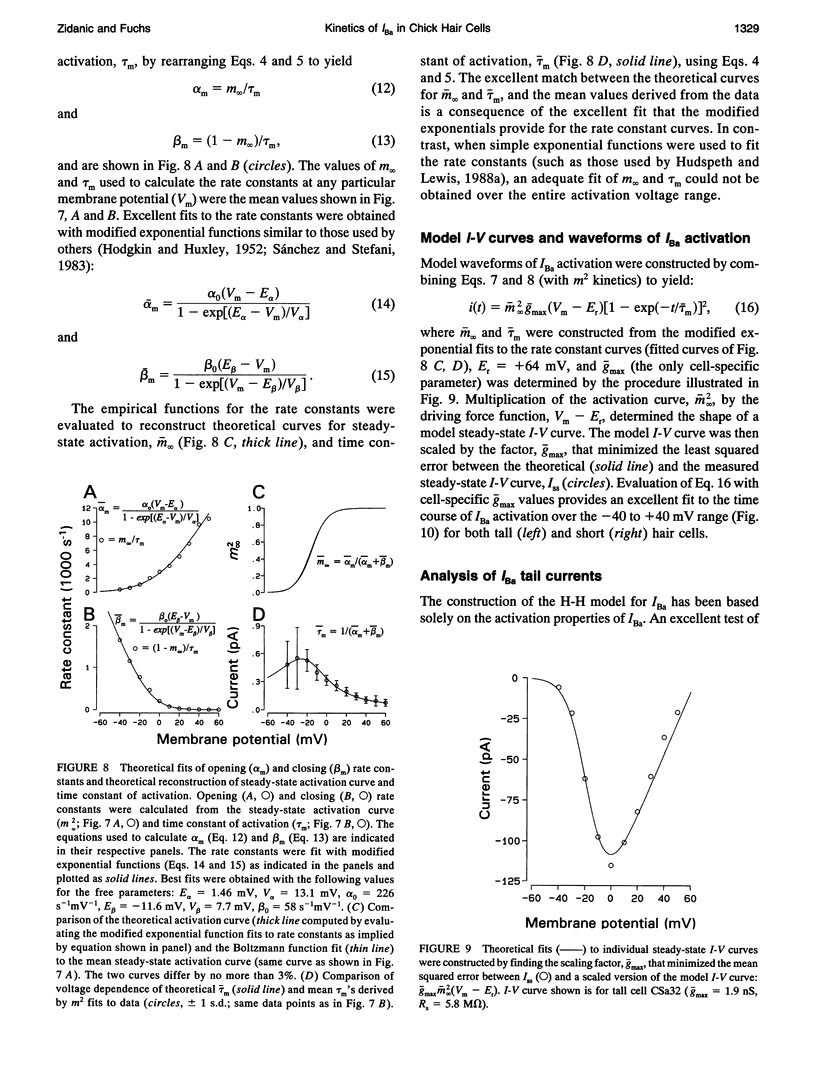
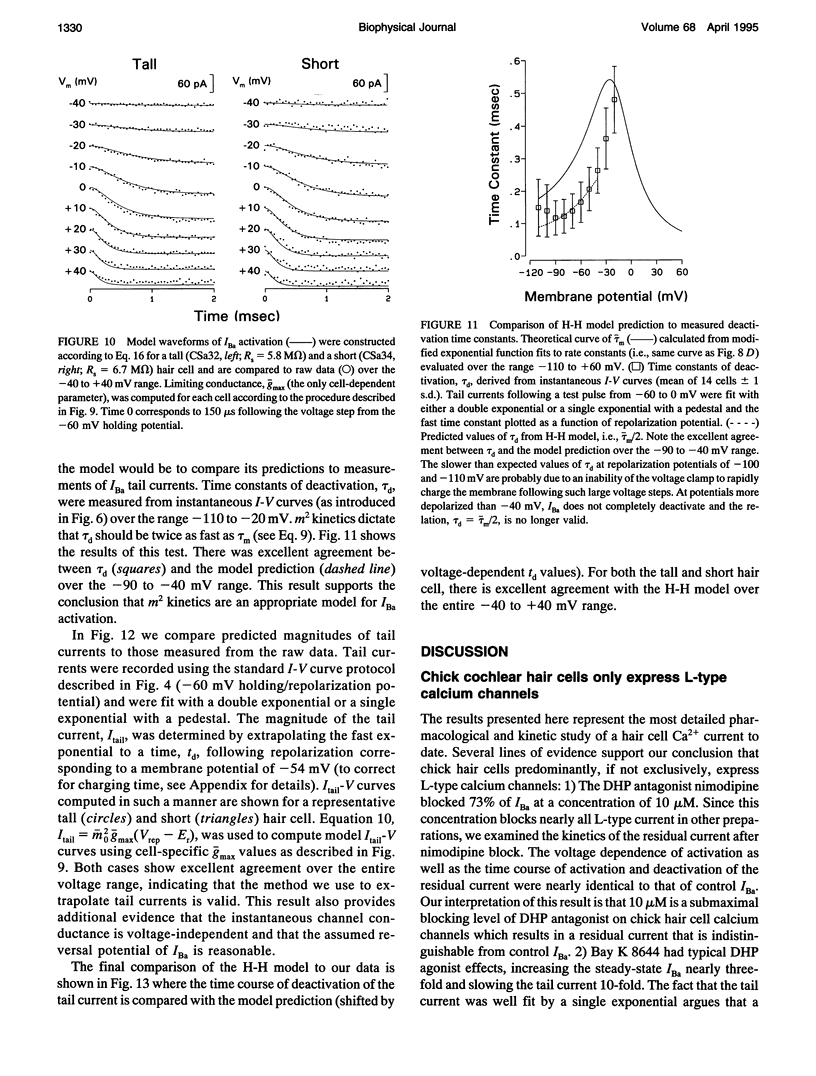
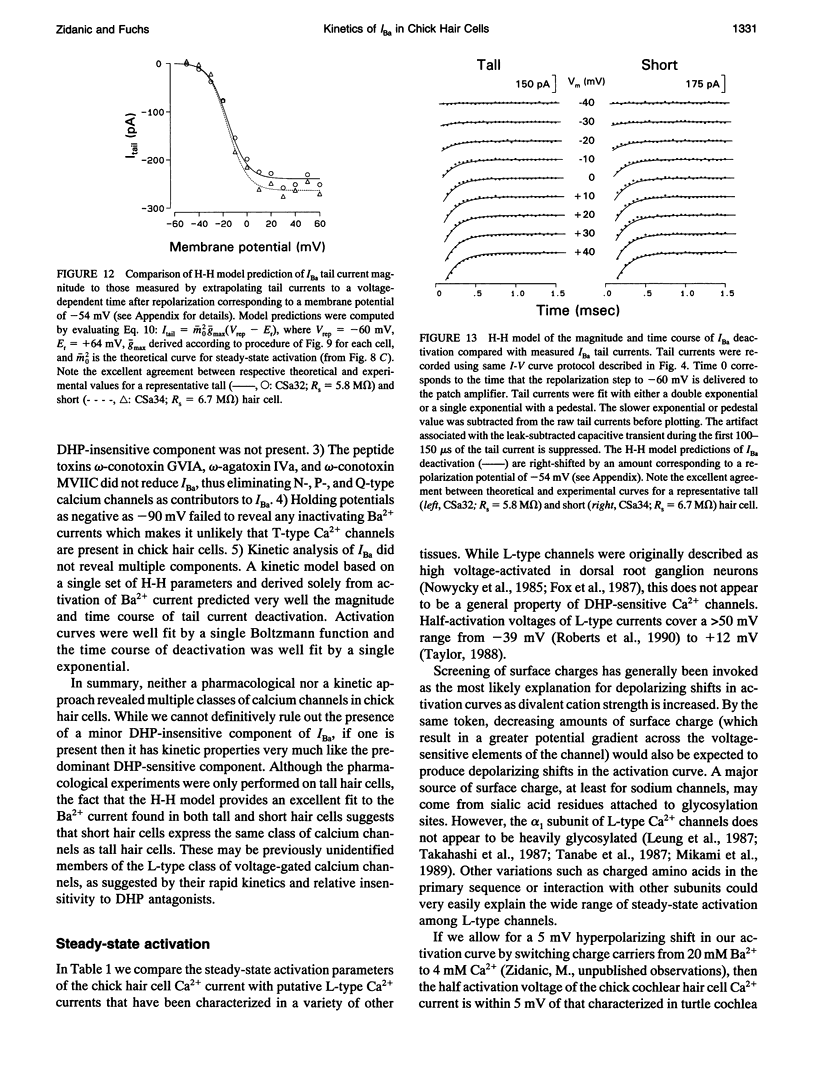
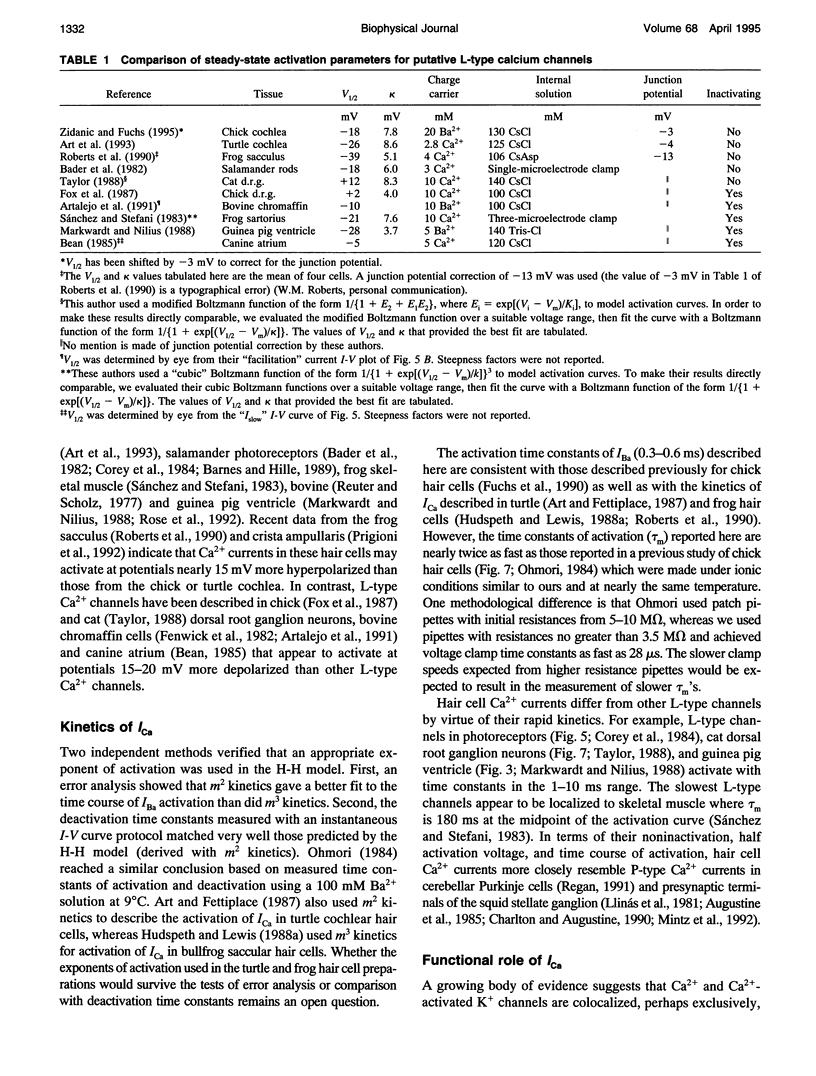
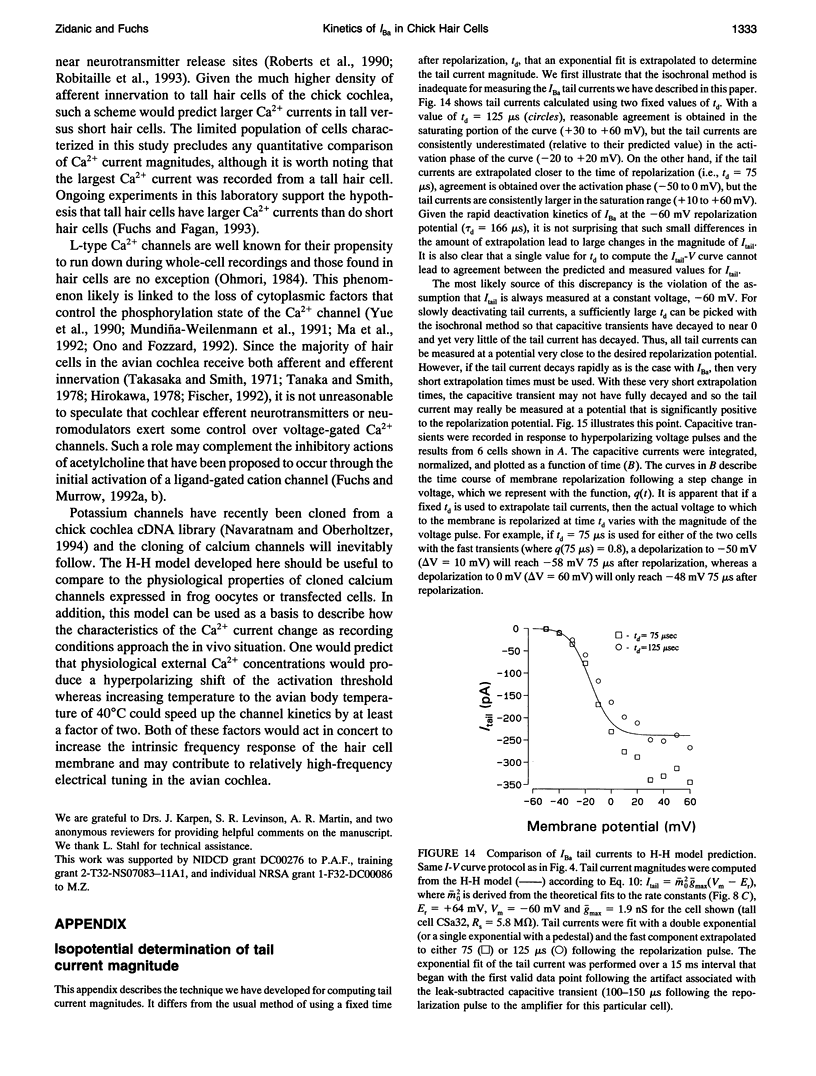
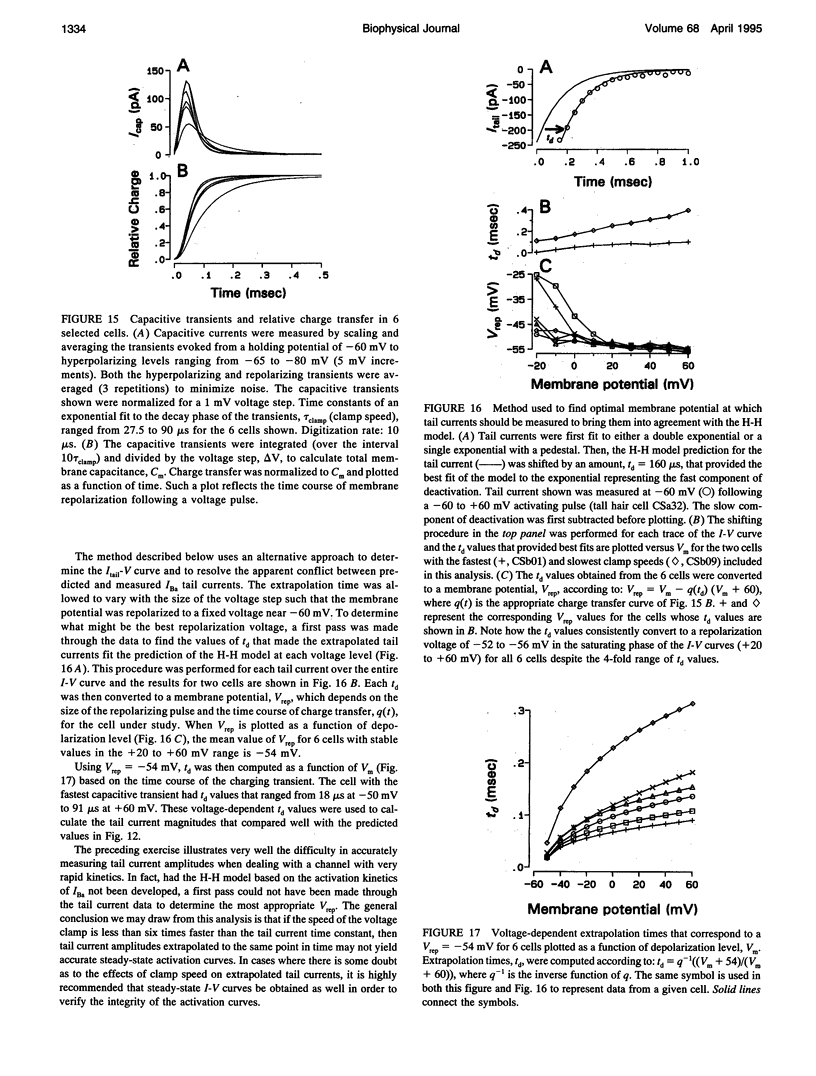
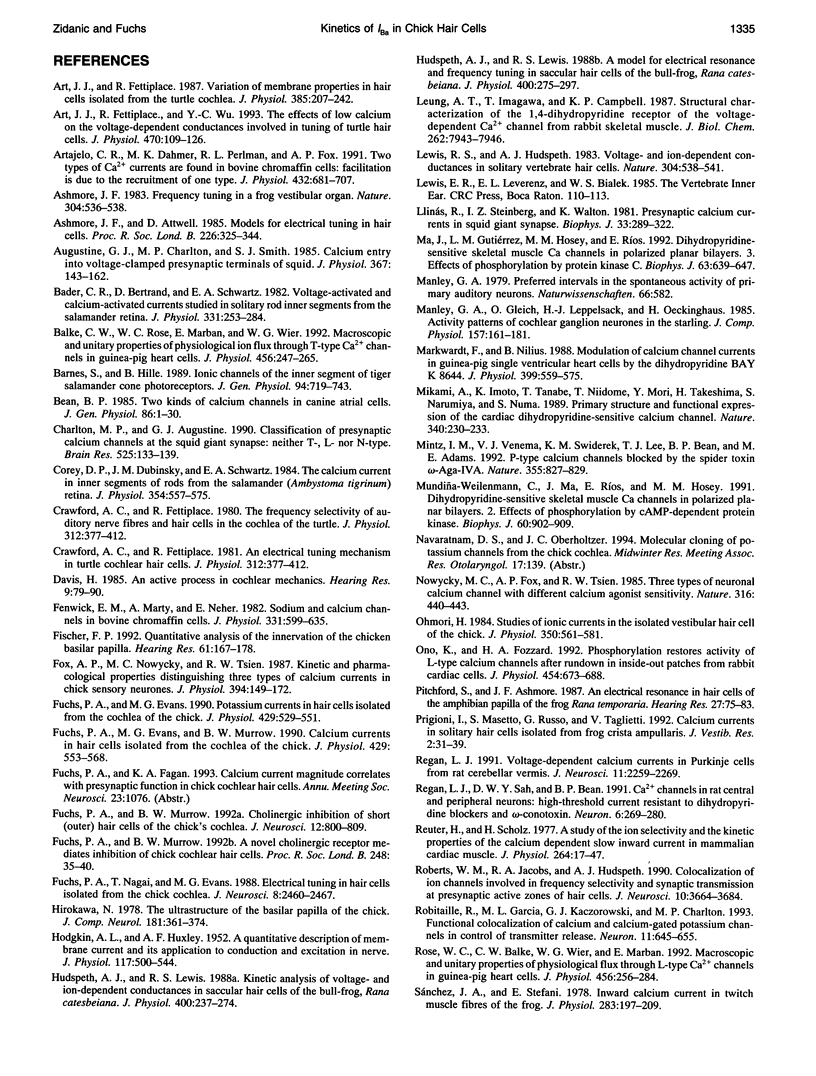
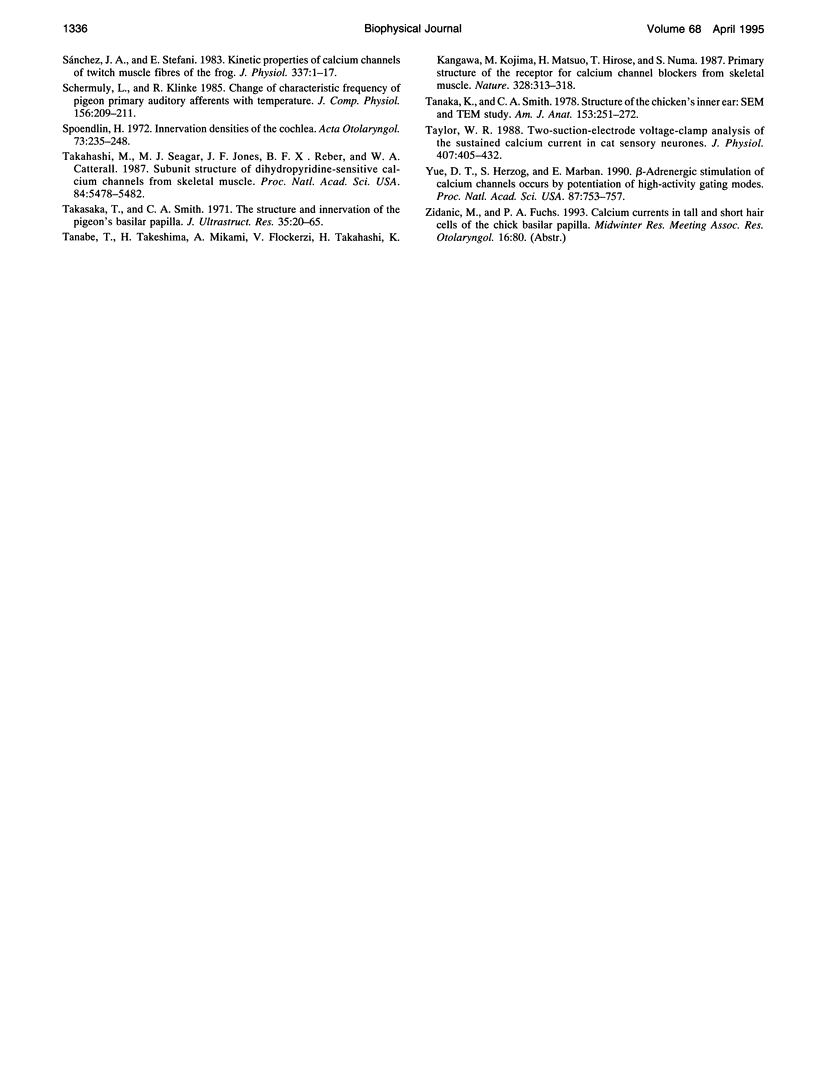
Selected References
These references are in PubMed. This may not be the complete list of references from this article.
- Art J. J., Fettiplace R. Variation of membrane properties in hair cells isolated from the turtle cochlea. J Physiol. 1987 Apr;385:207–242. doi: 10.1113/jphysiol.1987.sp016492. [DOI] [PMC free article] [PubMed] [Google Scholar]
- Art J. J., Fettiplace R., Wu Y. C. The effects of low calcium on the voltage-dependent conductances involved in tuning of turtle hair cells. J Physiol. 1993 Oct;470:109–126. doi: 10.1113/jphysiol.1993.sp019850. [DOI] [PMC free article] [PubMed] [Google Scholar]
- Artalejo C. R., Dahmer M. K., Perlman R. L., Fox A. P. Two types of Ca2+ currents are found in bovine chromaffin cells: facilitation is due to the recruitment of one type. J Physiol. 1991 Jan;432:681–707. doi: 10.1113/jphysiol.1991.sp018406. [DOI] [PMC free article] [PubMed] [Google Scholar]
- Ashmore J. F. Frequency tuning in a frog vestibular organ. Nature. 1983 Aug 11;304(5926):536–538. doi: 10.1038/304536a0. [DOI] [PubMed] [Google Scholar]
- Augustine G. J., Charlton M. P., Smith S. J. Calcium entry into voltage-clamped presynaptic terminals of squid. J Physiol. 1985 Oct;367:143–162. doi: 10.1113/jphysiol.1985.sp015818. [DOI] [PMC free article] [PubMed] [Google Scholar]
- Bader C. R., Bertrand D., Schwartz E. A. Voltage-activated and calcium-activated currents studied in solitary rod inner segments from the salamander retina. J Physiol. 1982 Oct;331:253–284. doi: 10.1113/jphysiol.1982.sp014372. [DOI] [PMC free article] [PubMed] [Google Scholar]
- Balke C. W., Rose W. C., Marban E., Wier W. G. Macroscopic and unitary properties of physiological ion flux through T-type Ca2+ channels in guinea-pig heart cells. J Physiol. 1992 Oct;456:247–265. doi: 10.1113/jphysiol.1992.sp019335. [DOI] [PMC free article] [PubMed] [Google Scholar]
- Barnes S., Hille B. Ionic channels of the inner segment of tiger salamander cone photoreceptors. J Gen Physiol. 1989 Oct;94(4):719–743. doi: 10.1085/jgp.94.4.719. [DOI] [PMC free article] [PubMed] [Google Scholar]
- Bean B. P. Two kinds of calcium channels in canine atrial cells. Differences in kinetics, selectivity, and pharmacology. J Gen Physiol. 1985 Jul;86(1):1–30. doi: 10.1085/jgp.86.1.1. [DOI] [PMC free article] [PubMed] [Google Scholar]
- Charlton M. P., Augustine G. J. Classification of presynaptic calcium channels at the squid giant synapse: neither T-, L- nor N-type. Brain Res. 1990 Aug 13;525(1):133–139. doi: 10.1016/0006-8993(90)91328-e. [DOI] [PubMed] [Google Scholar]
- Corey D. P., Dubinsky J. M., Schwartz E. A. The calcium current in inner segments of rods from the salamander (Ambystoma tigrinum) retina. J Physiol. 1984 Sep;354:557–575. doi: 10.1113/jphysiol.1984.sp015393. [DOI] [PMC free article] [PubMed] [Google Scholar]
- Crawford A. C., Fettiplace R. An electrical tuning mechanism in turtle cochlear hair cells. J Physiol. 1981 Mar;312:377–412. doi: 10.1113/jphysiol.1981.sp013634. [DOI] [PMC free article] [PubMed] [Google Scholar]
- Crawford A. C., Fettiplace R. An electrical tuning mechanism in turtle cochlear hair cells. J Physiol. 1981 Mar;312:377–412. doi: 10.1113/jphysiol.1981.sp013634. [DOI] [PMC free article] [PubMed] [Google Scholar]
- Davis H. An active process in cochlear mechanics. Hear Res. 1983 Jan;9(1):79–90. doi: 10.1016/0378-5955(83)90136-3. [DOI] [PubMed] [Google Scholar]
- Fenwick E. M., Marty A., Neher E. Sodium and calcium channels in bovine chromaffin cells. J Physiol. 1982 Oct;331:599–635. doi: 10.1113/jphysiol.1982.sp014394. [DOI] [PMC free article] [PubMed] [Google Scholar]
- Fischer F. P. Quantitative analysis of the innervation of the chicken basilar papilla. Hear Res. 1992 Aug;61(1-2):167–178. doi: 10.1016/0378-5955(92)90048-r. [DOI] [PubMed] [Google Scholar]
- Fox A. P., Nowycky M. C., Tsien R. W. Kinetic and pharmacological properties distinguishing three types of calcium currents in chick sensory neurones. J Physiol. 1987 Dec;394:149–172. doi: 10.1113/jphysiol.1987.sp016864. [DOI] [PMC free article] [PubMed] [Google Scholar]
- Fuchs P. A., Evans M. G., Murrow B. W. Calcium currents in hair cells isolated from the cochlea of the chick. J Physiol. 1990 Oct;429:553–568. doi: 10.1113/jphysiol.1990.sp018272. [DOI] [PMC free article] [PubMed] [Google Scholar]
- Fuchs P. A., Evans M. G. Potassium currents in hair cells isolated from the cochlea of the chick. J Physiol. 1990 Oct;429:529–551. doi: 10.1113/jphysiol.1990.sp018271. [DOI] [PMC free article] [PubMed] [Google Scholar]
- Fuchs P. A., Murrow B. W. A novel cholinergic receptor mediates inhibition of chick cochlear hair cells. Proc Biol Sci. 1992 Apr 22;248(1321):35–40. doi: 10.1098/rspb.1992.0039. [DOI] [PubMed] [Google Scholar]
- Fuchs P. A., Murrow B. W. Cholinergic inhibition of short (outer) hair cells of the chick's cochlea. J Neurosci. 1992 Mar;12(3):800–809. doi: 10.1523/JNEUROSCI.12-03-00800.1992. [DOI] [PMC free article] [PubMed] [Google Scholar]
- Fuchs P. A., Nagai T., Evans M. G. Electrical tuning in hair cells isolated from the chick cochlea. J Neurosci. 1988 Jul;8(7):2460–2467. doi: 10.1523/JNEUROSCI.08-07-02460.1988. [DOI] [PMC free article] [PubMed] [Google Scholar]
- HODGKIN A. L., HUXLEY A. F. A quantitative description of membrane current and its application to conduction and excitation in nerve. J Physiol. 1952 Aug;117(4):500–544. doi: 10.1113/jphysiol.1952.sp004764. [DOI] [PMC free article] [PubMed] [Google Scholar]
- Hirokawa N. The ultrastructure of the basilar papilla of the chick. J Comp Neurol. 1978 Sep 15;181(2):361–374. doi: 10.1002/cne.901810208. [DOI] [PubMed] [Google Scholar]
- Hudspeth A. J., Lewis R. S. A model for electrical resonance and frequency tuning in saccular hair cells of the bull-frog, Rana catesbeiana. J Physiol. 1988 Jun;400:275–297. doi: 10.1113/jphysiol.1988.sp017120. [DOI] [PMC free article] [PubMed] [Google Scholar]
- Hudspeth A. J., Lewis R. S. Kinetic analysis of voltage- and ion-dependent conductances in saccular hair cells of the bull-frog, Rana catesbeiana. J Physiol. 1988 Jun;400:237–274. doi: 10.1113/jphysiol.1988.sp017119. [DOI] [PMC free article] [PubMed] [Google Scholar]
- Leung A. T., Imagawa T., Campbell K. P. Structural characterization of the 1,4-dihydropyridine receptor of the voltage-dependent Ca2+ channel from rabbit skeletal muscle. Evidence for two distinct high molecular weight subunits. J Biol Chem. 1987 Jun 15;262(17):7943–7946. [PubMed] [Google Scholar]
- Lewis R. S., Hudspeth A. J. Voltage- and ion-dependent conductances in solitary vertebrate hair cells. Nature. 1983 Aug 11;304(5926):538–541. doi: 10.1038/304538a0. [DOI] [PubMed] [Google Scholar]
- Llinás R., Steinberg I. Z., Walton K. Presynaptic calcium currents in squid giant synapse. Biophys J. 1981 Mar;33(3):289–321. doi: 10.1016/S0006-3495(81)84898-9. [DOI] [PMC free article] [PubMed] [Google Scholar]
- Ma J., Gutiérrez L. M., Hosey M. M., Ríos E. Dihydropyridine-sensitive skeletal muscle Ca channels in polarized planar bilayers. 3. Effects of phosphorylation by protein kinase C. Biophys J. 1992 Sep;63(3):639–647. doi: 10.1016/S0006-3495(92)81634-X. [DOI] [PMC free article] [PubMed] [Google Scholar]
- Manley G. A., Gleich O., Leppelsack H. J., Oeckinghaus H. Activity patterns of cochlear ganglion neurones in the starling. J Comp Physiol A. 1985 Sep;157(2):161–181. doi: 10.1007/BF01350025. [DOI] [PubMed] [Google Scholar]
- Manley G. A. Preferred intervals in the spontaneous activity of primary auditory neurons. Naturwissenschaften. 1979 Nov;66(11):582–584. doi: 10.1007/BF00368823. [DOI] [PubMed] [Google Scholar]
- Markwardt F., Nilius B. Modulation of calcium channel currents in guinea-pig single ventricular heart cells by the dihydropyridine Bay K 8644. J Physiol. 1988 May;399:559–575. doi: 10.1113/jphysiol.1988.sp017096. [DOI] [PMC free article] [PubMed] [Google Scholar]
- Mikami A., Imoto K., Tanabe T., Niidome T., Mori Y., Takeshima H., Narumiya S., Numa S. Primary structure and functional expression of the cardiac dihydropyridine-sensitive calcium channel. Nature. 1989 Jul 20;340(6230):230–233. doi: 10.1038/340230a0. [DOI] [PubMed] [Google Scholar]
- Mintz I. M., Venema V. J., Swiderek K. M., Lee T. D., Bean B. P., Adams M. E. P-type calcium channels blocked by the spider toxin omega-Aga-IVA. Nature. 1992 Feb 27;355(6363):827–829. doi: 10.1038/355827a0. [DOI] [PubMed] [Google Scholar]
- Mundiña-Weilenmann C., Ma J., Ríos E., Hosey M. M. Dihydropyridine-sensitive skeletal muscle Ca channels in polarized planar bilayers. 2. Effects of phosphorylation by cAMP-dependent protein kinase. Biophys J. 1991 Oct;60(4):902–909. doi: 10.1016/S0006-3495(91)82124-5. [DOI] [PMC free article] [PubMed] [Google Scholar]
- Nowycky M. C., Fox A. P., Tsien R. W. Three types of neuronal calcium channel with different calcium agonist sensitivity. Nature. 1985 Aug 1;316(6027):440–443. doi: 10.1038/316440a0. [DOI] [PubMed] [Google Scholar]
- Ohmori H. Studies of ionic currents in the isolated vestibular hair cell of the chick. J Physiol. 1984 May;350:561–581. doi: 10.1113/jphysiol.1984.sp015218. [DOI] [PMC free article] [PubMed] [Google Scholar]
- Ono K., Fozzard H. A. Phosphorylation restores activity of L-type calcium channels after rundown in inside-out patches from rabbit cardiac cells. J Physiol. 1992 Aug;454:673–688. doi: 10.1113/jphysiol.1992.sp019286. [DOI] [PMC free article] [PubMed] [Google Scholar]
- Pitchford S., Ashmore J. F. An electrical resonance in hair cells of the amphibian papilla of the frog Rana temporaria. Hear Res. 1987;27(1):75–83. doi: 10.1016/0378-5955(87)90027-x. [DOI] [PubMed] [Google Scholar]
- Prigioni I., Masetto S., Russo G., Taglietti V. Calcium currents in solitary hair cells isolated from frog crista ampullaris. J Vestib Res. 1992;2(1):31–39. [PubMed] [Google Scholar]
- Regan L. J., Sah D. W., Bean B. P. Ca2+ channels in rat central and peripheral neurons: high-threshold current resistant to dihydropyridine blockers and omega-conotoxin. Neuron. 1991 Feb;6(2):269–280. doi: 10.1016/0896-6273(91)90362-4. [DOI] [PubMed] [Google Scholar]
- Regan L. J. Voltage-dependent calcium currents in Purkinje cells from rat cerebellar vermis. J Neurosci. 1991 Jul;11(7):2259–2269. doi: 10.1523/JNEUROSCI.11-07-02259.1991. [DOI] [PMC free article] [PubMed] [Google Scholar]
- Reuter H., Scholz H. A study of the ion selectivity and the kinetic properties of the calcium dependent slow inward current in mammalian cardiac muscle. J Physiol. 1977 Jan;264(1):17–47. doi: 10.1113/jphysiol.1977.sp011656. [DOI] [PMC free article] [PubMed] [Google Scholar]
- Roberts W. M., Jacobs R. A., Hudspeth A. J. Colocalization of ion channels involved in frequency selectivity and synaptic transmission at presynaptic active zones of hair cells. J Neurosci. 1990 Nov;10(11):3664–3684. doi: 10.1523/JNEUROSCI.10-11-03664.1990. [DOI] [PMC free article] [PubMed] [Google Scholar]
- Robitaille R., Garcia M. L., Kaczorowski G. J., Charlton M. P. Functional colocalization of calcium and calcium-gated potassium channels in control of transmitter release. Neuron. 1993 Oct;11(4):645–655. doi: 10.1016/0896-6273(93)90076-4. [DOI] [PubMed] [Google Scholar]
- Rose W. C., Balke C. W., Wier W. G., Marban E. Macroscopic and unitary properties of physiological ion flux through L-type Ca2+ channels in guinea-pig heart cells. J Physiol. 1992 Oct;456:267–284. doi: 10.1113/jphysiol.1992.sp019336. [DOI] [PMC free article] [PubMed] [Google Scholar]
- Sanchez J. A., Stefani E. Inward calcium current in twitch muscle fibres of the frog. J Physiol. 1978 Oct;283:197–209. doi: 10.1113/jphysiol.1978.sp012496. [DOI] [PMC free article] [PubMed] [Google Scholar]
- Spoendlin H. Innervation densities of the cochlea. Acta Otolaryngol. 1972 Feb-Mar;73(2):235–248. doi: 10.3109/00016487209138937. [DOI] [PubMed] [Google Scholar]
- Sánchez J. A., Stefani E. Kinetic properties of calcium channels of twitch muscle fibres of the frog. J Physiol. 1983 Apr;337:1–17. doi: 10.1113/jphysiol.1983.sp014607. [DOI] [PMC free article] [PubMed] [Google Scholar]
- Takahashi M., Seagar M. J., Jones J. F., Reber B. F., Catterall W. A. Subunit structure of dihydropyridine-sensitive calcium channels from skeletal muscle. Proc Natl Acad Sci U S A. 1987 Aug;84(15):5478–5482. doi: 10.1073/pnas.84.15.5478. [DOI] [PMC free article] [PubMed] [Google Scholar]
- Takasaka T., Smith C. A. The structure and innervation of the pigeon's basilar papilla. J Ultrastruct Res. 1971 Apr;35(1):20–65. doi: 10.1016/s0022-5320(71)80141-7. [DOI] [PubMed] [Google Scholar]
- Tanabe T., Takeshima H., Mikami A., Flockerzi V., Takahashi H., Kangawa K., Kojima M., Matsuo H., Hirose T., Numa S. Primary structure of the receptor for calcium channel blockers from skeletal muscle. Nature. 1987 Jul 23;328(6128):313–318. doi: 10.1038/328313a0. [DOI] [PubMed] [Google Scholar]
- Tanaka K., Smith C. A. Structure of the chicken's inner ear: SEM and TEM study. Am J Anat. 1978 Oct;153(2):251–271. doi: 10.1002/aja.1001530206. [DOI] [PubMed] [Google Scholar]
- Taylor W. R. Two-suction-electrode voltage-clamp analysis of the sustained calcium current in cat sensory neurones. J Physiol. 1988 Dec;407:405–432. doi: 10.1113/jphysiol.1988.sp017423. [DOI] [PMC free article] [PubMed] [Google Scholar]
- Yue D. T., Herzig S., Marban E. Beta-adrenergic stimulation of calcium channels occurs by potentiation of high-activity gating modes. Proc Natl Acad Sci U S A. 1990 Jan;87(2):753–757. doi: 10.1073/pnas.87.2.753. [DOI] [PMC free article] [PubMed] [Google Scholar]