Abstract
Each myosin molecule contains two heavy chains and a total of four low-molecular weight light chain subunits, two "essential" and two "regulatory" light chains (RLCs). Although the roles of myosin light chains in vertebrate striated muscle are poorly understood at present, recent studies on the RLC have suggested that it has a modulatory role with respect to Ca2+ sensitivity of tension and the rate of tension development, effects that may be mediated by Ca2+ binding to the RLC. To examine possible roles of the RLC Ca2+/Mg2+ binding site in tension development by skeletal muscle, we replaced endogenous RLC in rabbit skinned psoas fibers with an avian mutant RLC (D47A) having much reduced affinity for divalent cations. After replacement of up to 80% of the endogenous RLC with D47A RLC, maximum tension (at pCa 4.5) was significantly reduced compared with preexchange tension, and the amount of decrease was directly related to the extent of D47A exchange. Fiber stiffness changed in proportion to tension, indicating that the decrease in tension was due to a decrease in the number of tension-generating cross-bridges. Decreases in both tension and stiffness were substantially, although incompletely, reversed after reexchange of native RLC for D47A. RLC exchange was also performed using a wild-type RLC. Although a small decrease in tension was observed after wild-type RLC exchange, the decrease was not proportional to the extent of RLC exchange and was not reversed by reexchange of the native RLC. D47A exchange also decreased the Ca2+ sensitivity of tension and reduced the apparent cooperativity of tension development. The results suggest that divalent cation binding to myosin RLC plays an important role in tension generation in skeletal muscle fibers.
Full text
PDF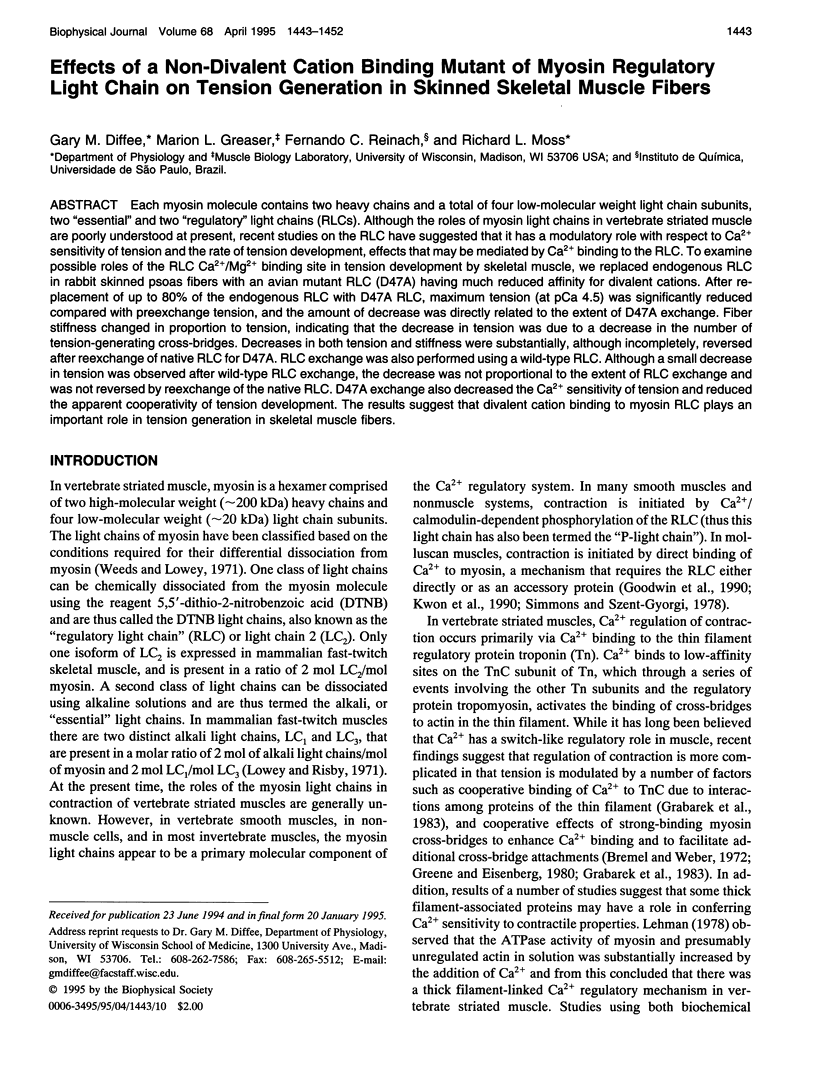
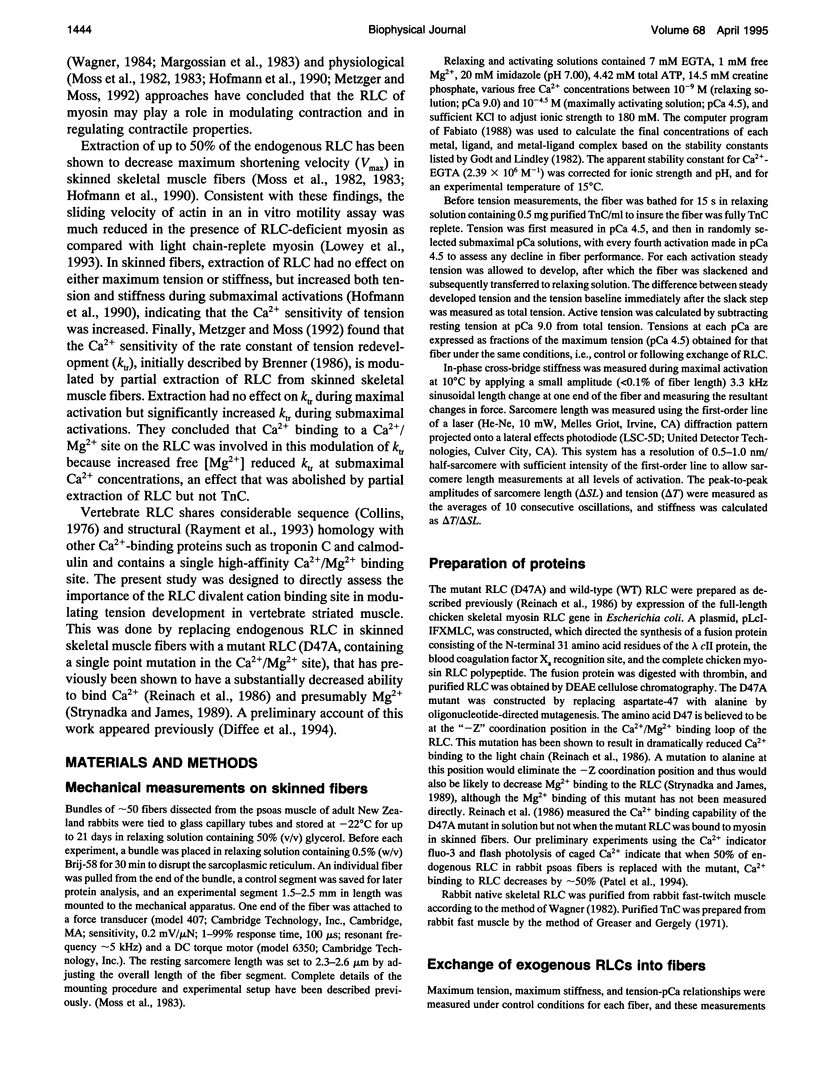
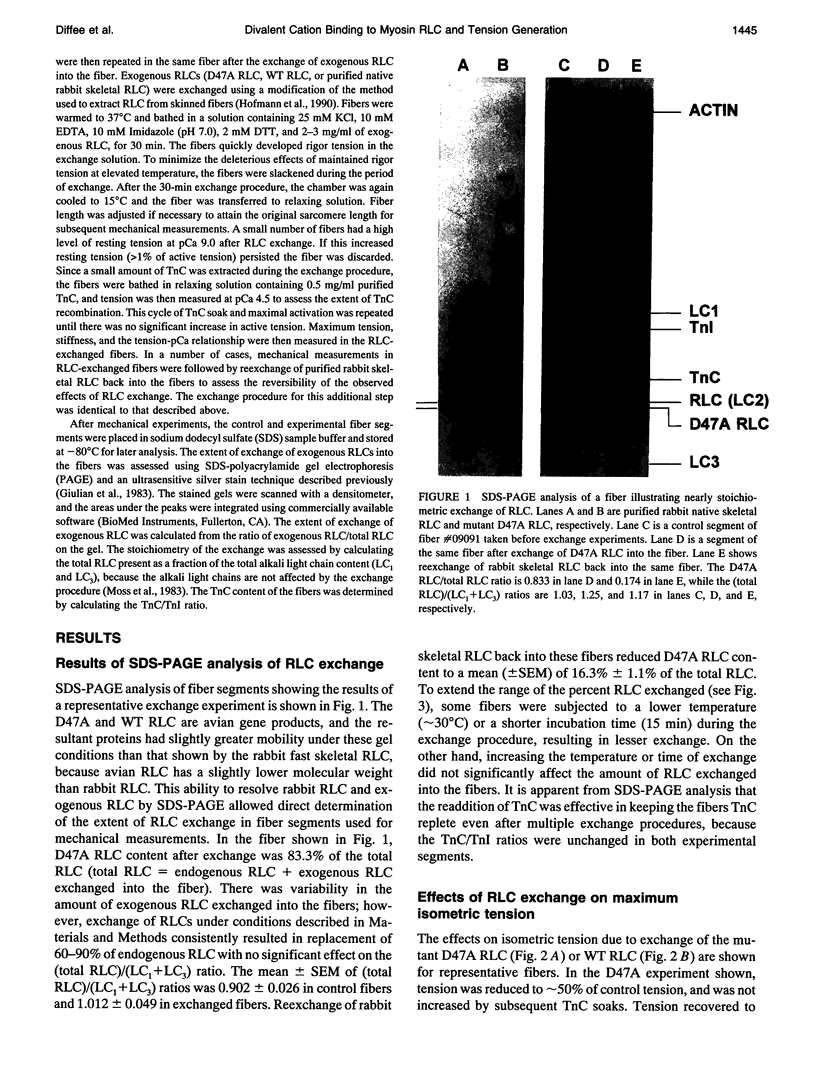
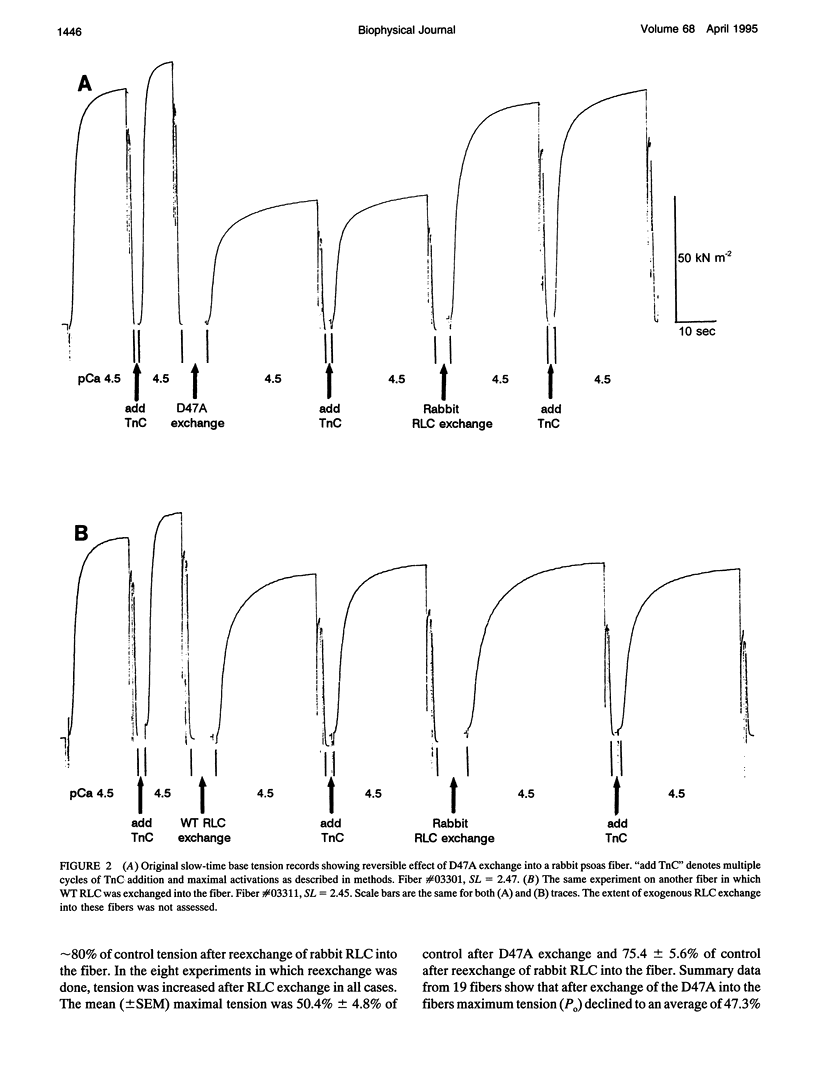
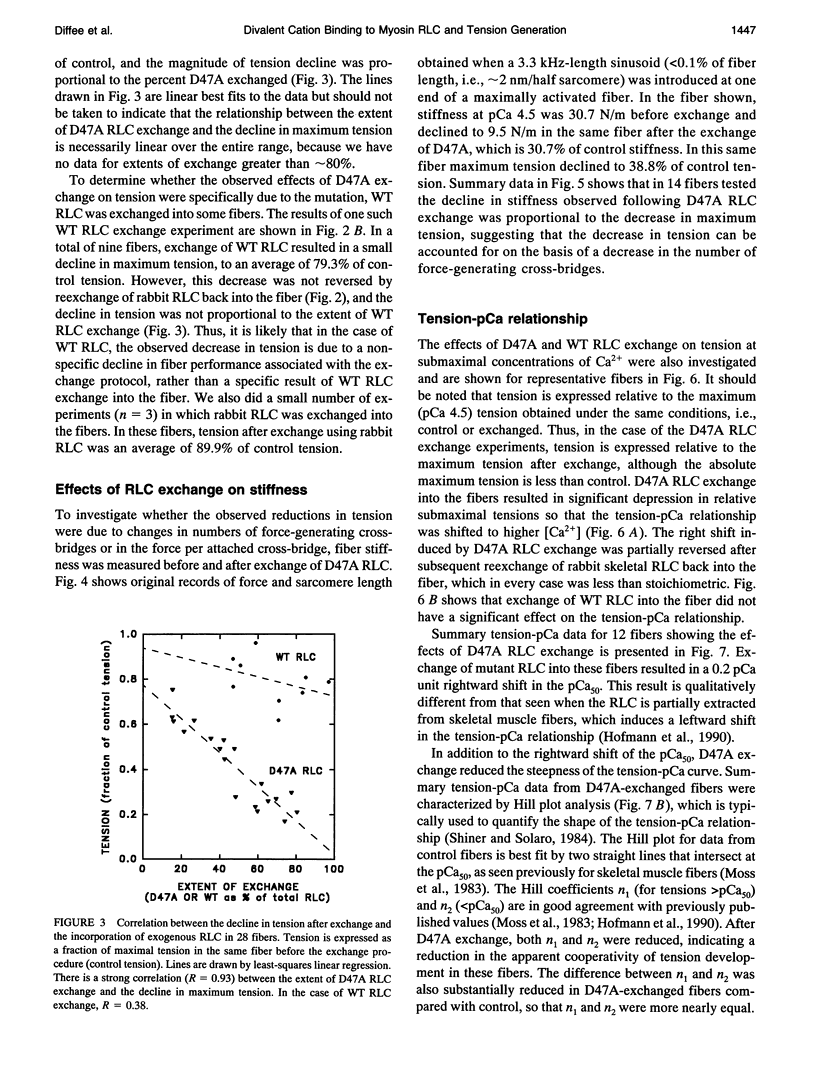
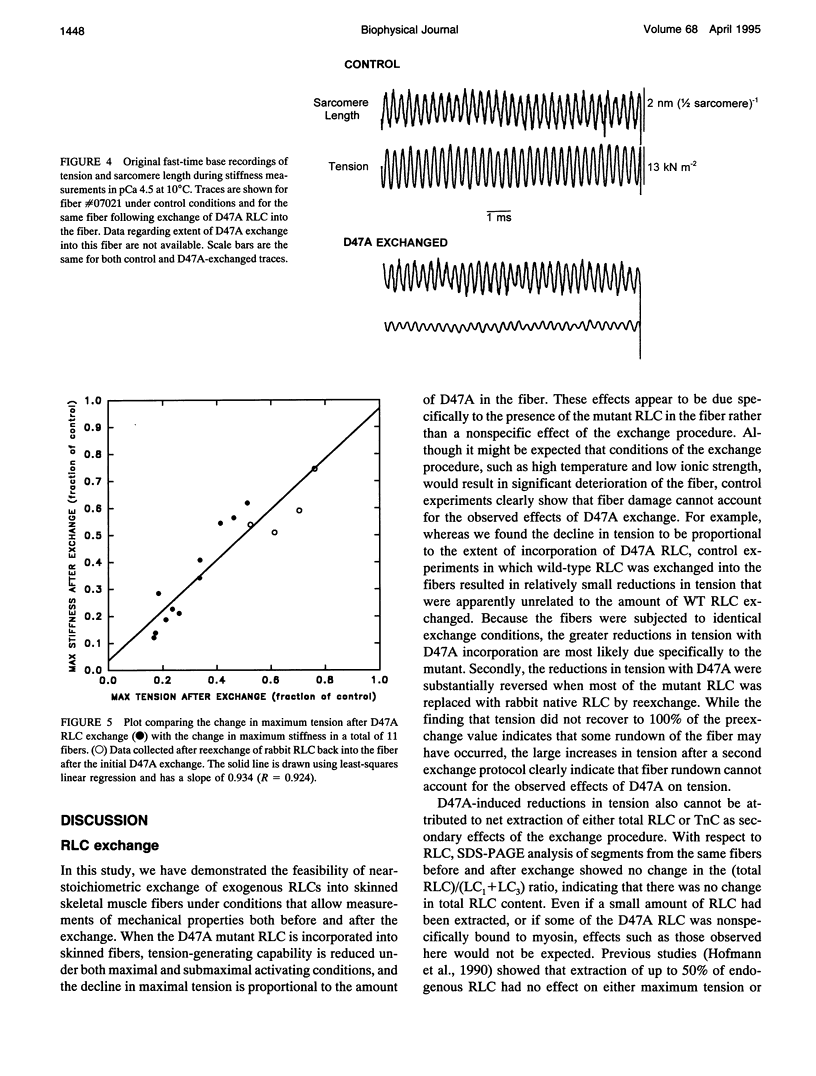
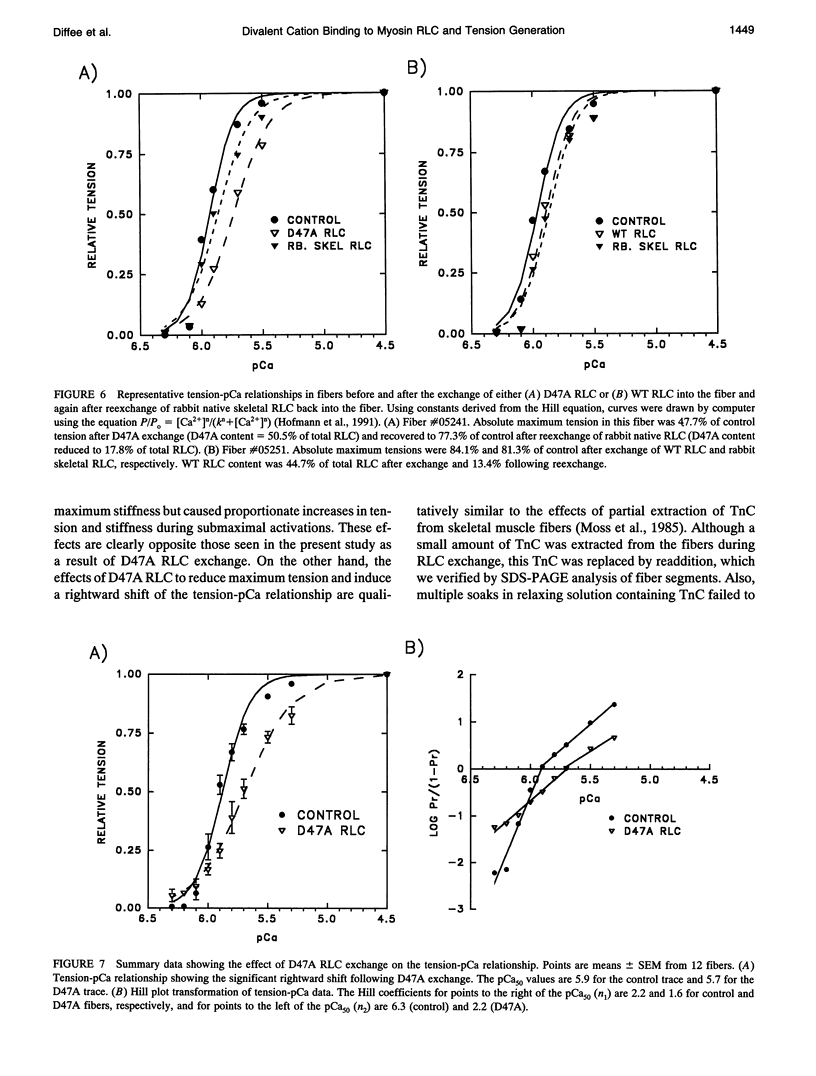
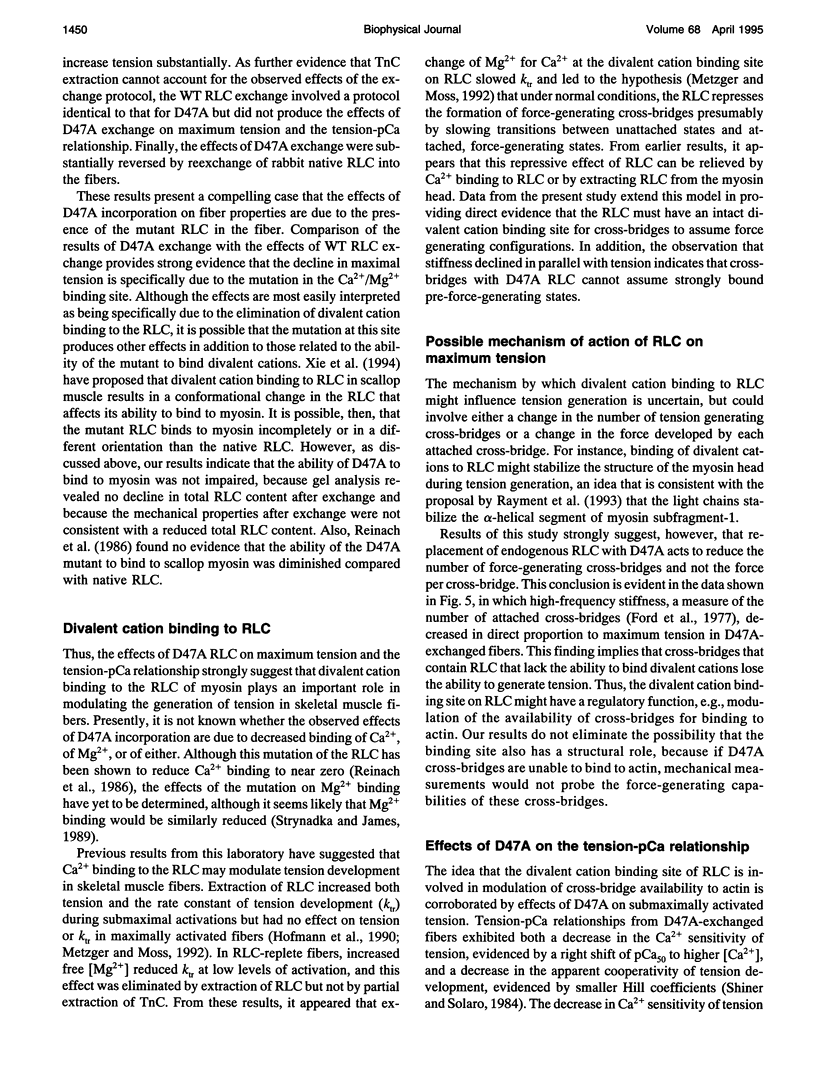
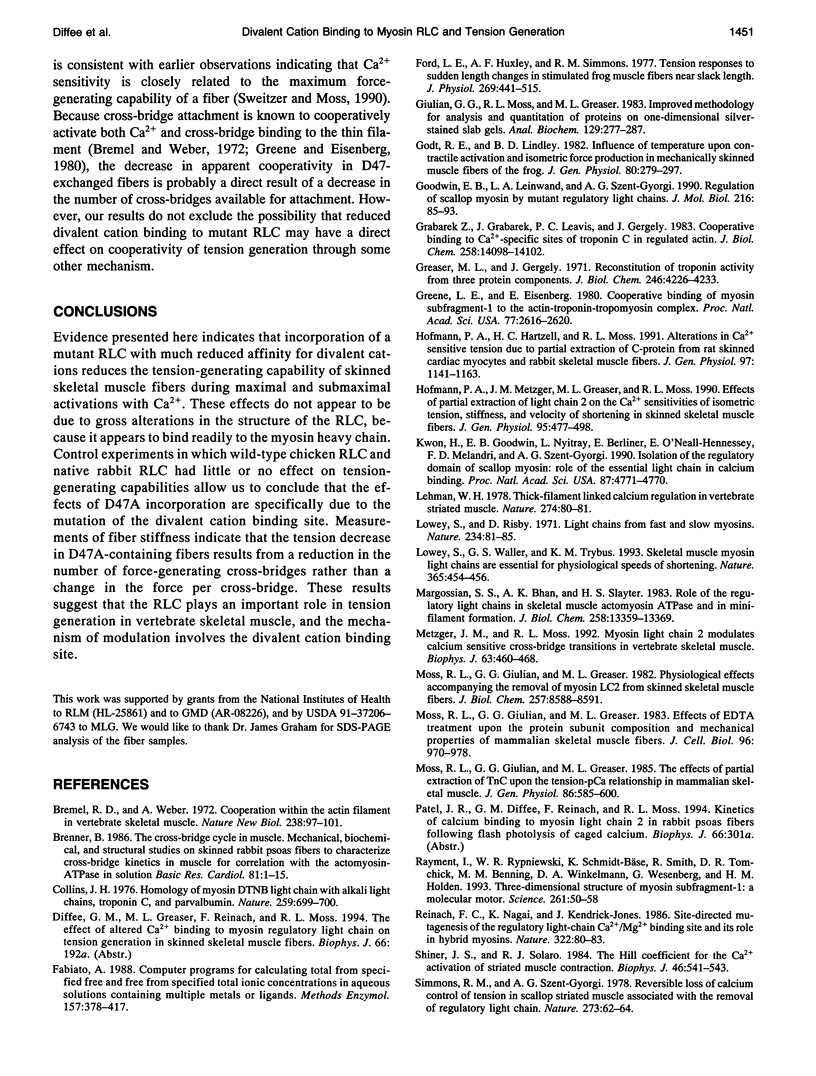
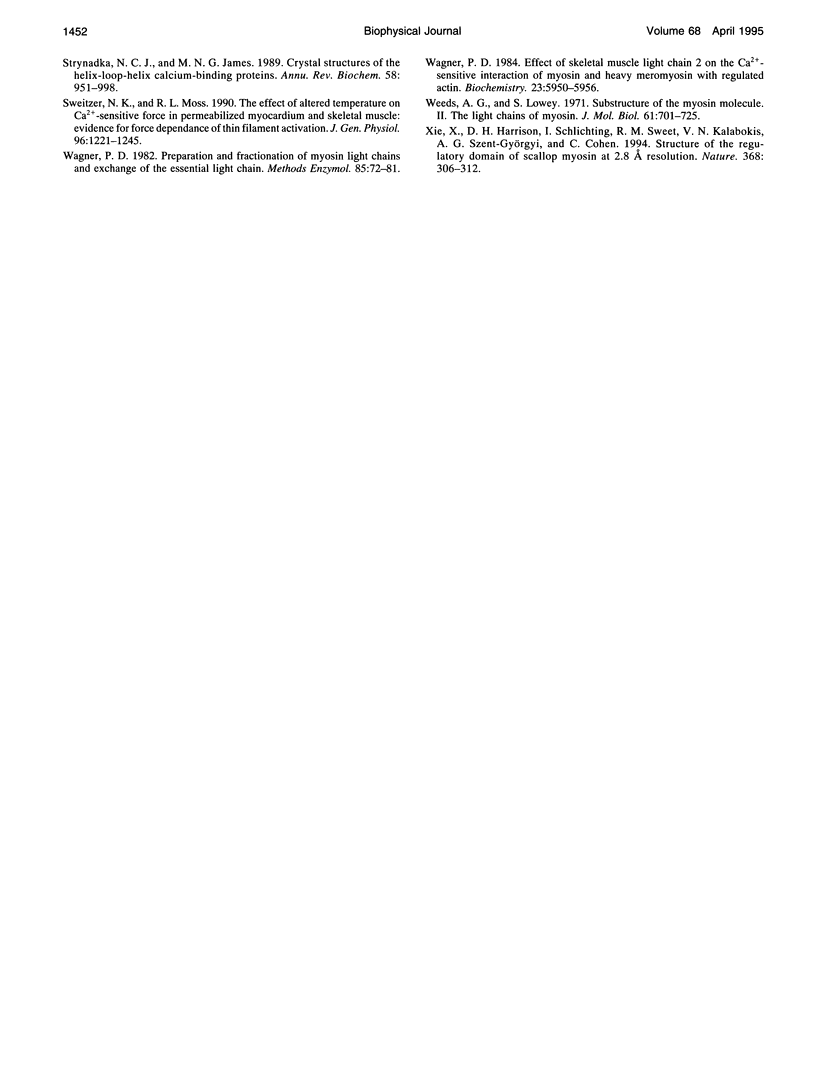
Images in this article
Selected References
These references are in PubMed. This may not be the complete list of references from this article.
- Bremel R. D., Weber A. Cooperation within actin filament in vertebrate skeletal muscle. Nat New Biol. 1972 Jul 26;238(82):97–101. doi: 10.1038/newbio238097a0. [DOI] [PubMed] [Google Scholar]
- Brenner B. The cross-bridge cycle in muscle. Mechanical, biochemical, and structural studies on single skinned rabbit psoas fibers to characterize cross-bridge kinetics in muscle for correlation with the actomyosin-ATPase in solution. Basic Res Cardiol. 1986;81 (Suppl 1):1–15. doi: 10.1007/978-3-662-11374-5_1. [DOI] [PubMed] [Google Scholar]
- Collins J. H. Homology of myosin DTNB light chain with alkali light chains, troponin C and parvalbumin. Nature. 1976 Feb 26;259(5545):699–700. doi: 10.1038/259699a0. [DOI] [PubMed] [Google Scholar]
- Fabiato A. Computer programs for calculating total from specified free or free from specified total ionic concentrations in aqueous solutions containing multiple metals and ligands. Methods Enzymol. 1988;157:378–417. doi: 10.1016/0076-6879(88)57093-3. [DOI] [PubMed] [Google Scholar]
- Ford L. E., Huxley A. F., Simmons R. M. Tension responses to sudden length change in stimulated frog muscle fibres near slack length. J Physiol. 1977 Jul;269(2):441–515. doi: 10.1113/jphysiol.1977.sp011911. [DOI] [PMC free article] [PubMed] [Google Scholar]
- Giulian G. G., Moss R. L., Greaser M. Improved methodology for analysis and quantitation of proteins on one-dimensional silver-stained slab gels. Anal Biochem. 1983 Mar;129(2):277–287. doi: 10.1016/0003-2697(83)90551-1. [DOI] [PubMed] [Google Scholar]
- Godt R. E., Lindley B. D. Influence of temperature upon contractile activation and isometric force production in mechanically skinned muscle fibers of the frog. J Gen Physiol. 1982 Aug;80(2):279–297. doi: 10.1085/jgp.80.2.279. [DOI] [PMC free article] [PubMed] [Google Scholar]
- Goodwin E. B., Leinwand L. A., Szent-Györgyi A. G. Regulation of scallop myosin by mutant regulatory light chains. J Mol Biol. 1990 Nov 5;216(1):85–93. doi: 10.1016/S0022-2836(05)80062-2. [DOI] [PubMed] [Google Scholar]
- Grabarek Z., Grabarek J., Leavis P. C., Gergely J. Cooperative binding to the Ca2+-specific sites of troponin C in regulated actin and actomyosin. J Biol Chem. 1983 Dec 10;258(23):14098–14102. [PubMed] [Google Scholar]
- Greaser M. L., Gergely J. Reconstitution of troponin activity from three protein components. J Biol Chem. 1971 Jul 10;246(13):4226–4233. [PubMed] [Google Scholar]
- Greene L. E., Eisenberg E. Cooperative binding of myosin subfragment-1 to the actin-troponin-tropomyosin complex. Proc Natl Acad Sci U S A. 1980 May;77(5):2616–2620. doi: 10.1073/pnas.77.5.2616. [DOI] [PMC free article] [PubMed] [Google Scholar]
- Hofmann P. A., Hartzell H. C., Moss R. L. Alterations in Ca2+ sensitive tension due to partial extraction of C-protein from rat skinned cardiac myocytes and rabbit skeletal muscle fibers. J Gen Physiol. 1991 Jun;97(6):1141–1163. doi: 10.1085/jgp.97.6.1141. [DOI] [PMC free article] [PubMed] [Google Scholar]
- Hofmann P. A., Metzger J. M., Greaser M. L., Moss R. L. Effects of partial extraction of light chain 2 on the Ca2+ sensitivities of isometric tension, stiffness, and velocity of shortening in skinned skeletal muscle fibers. J Gen Physiol. 1990 Mar;95(3):477–498. doi: 10.1085/jgp.95.3.477. [DOI] [PMC free article] [PubMed] [Google Scholar]
- Kwon H., Goodwin E. B., Nyitray L., Berliner E., O'Neall-Hennessey E., Melandri F. D., Szent-Györgyi A. G. Isolation of the regulatory domain of scallop myosin: role of the essential light chain in calcium binding. Proc Natl Acad Sci U S A. 1990 Jun;87(12):4771–4775. doi: 10.1073/pnas.87.12.4771. [DOI] [PMC free article] [PubMed] [Google Scholar]
- Lehman W. Thick-filament-linked calcium regulation in vertebrate striated muscle. Nature. 1978 Jul 6;274(5666):80–81. doi: 10.1038/274080a0. [DOI] [PubMed] [Google Scholar]
- Lowey S., Risby D. Light chains from fast and slow muscle myosins. Nature. 1971 Nov 12;234(5324):81–85. doi: 10.1038/234081a0. [DOI] [PubMed] [Google Scholar]
- Lowey S., Waller G. S., Trybus K. M. Skeletal muscle myosin light chains are essential for physiological speeds of shortening. Nature. 1993 Sep 30;365(6445):454–456. doi: 10.1038/365454a0. [DOI] [PubMed] [Google Scholar]
- Margossian S. S., Bhan A. K., Slayter H. S. Role of the regulatory light chains in skeletal muscle actomyosin ATPase and in minifilament formation. J Biol Chem. 1983 Nov 10;258(21):13359–13369. [PubMed] [Google Scholar]
- Metzger J. M., Moss R. L. Myosin light chain 2 modulates calcium-sensitive cross-bridge transitions in vertebrate skeletal muscle. Biophys J. 1992 Aug;63(2):460–468. doi: 10.1016/S0006-3495(92)81614-4. [DOI] [PMC free article] [PubMed] [Google Scholar]
- Moss R. L., Giulian G. G., Greaser M. L. Effects of EDTA treatment upon the protein subunit composition and mechanical properties of mammalian single skeletal muscle fibers. J Cell Biol. 1983 Apr;96(4):970–978. doi: 10.1083/jcb.96.4.970. [DOI] [PMC free article] [PubMed] [Google Scholar]
- Moss R. L., Giulian G. G., Greaser M. L. Physiological effects accompanying the removal of myosin LC2 from skinned skeletal muscle fibers. J Biol Chem. 1982 Aug 10;257(15):8588–8591. [PubMed] [Google Scholar]
- Moss R. L., Giulian G. G., Greaser M. L. The effects of partial extraction of TnC upon the tension-pCa relationship in rabbit skinned skeletal muscle fibers. J Gen Physiol. 1985 Oct;86(4):585–600. doi: 10.1085/jgp.86.4.585. [DOI] [PMC free article] [PubMed] [Google Scholar]
- Rayment I., Rypniewski W. R., Schmidt-Bäse K., Smith R., Tomchick D. R., Benning M. M., Winkelmann D. A., Wesenberg G., Holden H. M. Three-dimensional structure of myosin subfragment-1: a molecular motor. Science. 1993 Jul 2;261(5117):50–58. doi: 10.1126/science.8316857. [DOI] [PubMed] [Google Scholar]
- Reinach F. C., Nagai K., Kendrick-Jones J. Site-directed mutagenesis of the regulatory light-chain Ca2+/Mg2+ binding site and its role in hybrid myosins. Nature. 1986 Jul 3;322(6074):80–83. doi: 10.1038/322080a0. [DOI] [PubMed] [Google Scholar]
- Shiner J. S., Solaro R. J. The hill coefficient for the Ca2+-activation of striated muscle contraction. Biophys J. 1984 Oct;46(4):541–543. doi: 10.1016/S0006-3495(84)84051-5. [DOI] [PMC free article] [PubMed] [Google Scholar]
- Simmons R. M., Szent-Györgyi A. G. Reversible loss of calcium control of tension in scallop striated muscle associated with the removal of regulatory light chains. Nature. 1978 May 4;273(5657):62–64. doi: 10.1038/273062a0. [DOI] [PubMed] [Google Scholar]
- Strynadka N. C., James M. N. Crystal structures of the helix-loop-helix calcium-binding proteins. Annu Rev Biochem. 1989;58:951–998. doi: 10.1146/annurev.bi.58.070189.004511. [DOI] [PubMed] [Google Scholar]
- Sweitzer N. K., Moss R. L. The effect of altered temperature on Ca2(+)-sensitive force in permeabilized myocardium and skeletal muscle. Evidence for force dependence of thin filament activation. J Gen Physiol. 1990 Dec;96(6):1221–1245. doi: 10.1085/jgp.96.6.1221. [DOI] [PMC free article] [PubMed] [Google Scholar]
- Wagner P. D. Effect of skeletal muscle myosin light chain 2 on the Ca2+-sensitive interaction of myosin and heavy meromyosin with regulated actin. Biochemistry. 1984 Dec 4;23(25):5950–5956. doi: 10.1021/bi00320a010. [DOI] [PubMed] [Google Scholar]
- Wagner P. D. Preparation and fractionation of myosin light chains and exchange of the essential light chains. Methods Enzymol. 1982;85(Pt B):72–81. doi: 10.1016/0076-6879(82)85010-6. [DOI] [PubMed] [Google Scholar]
- Weeds A. G., Lowey S. Substructure of the myosin molecule. II. The light chains of myosin. J Mol Biol. 1971 Nov 14;61(3):701–725. doi: 10.1016/0022-2836(71)90074-x. [DOI] [PubMed] [Google Scholar]
- Xie X., Harrison D. H., Schlichting I., Sweet R. M., Kalabokis V. N., Szent-Györgyi A. G., Cohen C. Structure of the regulatory domain of scallop myosin at 2.8 A resolution. Nature. 1994 Mar 24;368(6469):306–312. doi: 10.1038/368306a0. [DOI] [PubMed] [Google Scholar]