Abstract
Total internal reflection (TIR) microfluorimetry was established as a method to measure continuously the volume of adherent cells and applied to measure membrane permeabilities in cells transfected with water channel homologs. Cytosol was labeled with the membrane-impermeant fluorophore calcein. Fluorescence was excited by the TIR evanescent field in a thin section of cytosol (approximately 150 nm) adjacent to the cell-substrate interface. Because cytosolic fluorophore number per cell remains constant, the TIR fluorescence signal should be inversely related to cell volume. For small volume changes in Sf-9 and LLC-PK1 cells, relative TIR fluorescence was nearly equal to inverse relative cell volume; deviations from the ideal were modeled theoretically. To measure plasma membrane osmotic water permeability, Pf, the time course of osmotically induced cell volume change was inferred from the TIR fluorescence signal. LLC-PK1 cells expressing the CHIP28 water channel had an HgCl2-sensitive, threefold increase in Pf compared to nontransfected cells (Pf = 0.0043 cm/s at 10 degrees C). Solute permeability was measured from the TIR fluorescence time course in response to solute gradients. Glycerol permeability in Sf-9 cells expressing the water channel homolog GLIP was (1.3 +/- 0.2) x 10(-5) cm/s (22 degrees C), greater than that of (0.36 +/- 0.04) x 10(-5) cm/s (n = 4, p < 0.05) for control cells, indicating functional expression of GLIP. Water and urea permeabilities were similar in GLIP-expressing and control cells. The TIR method should be applicable to the study of water and solute permeabilities and cell volume regulation in cells of arbitrary shape and size.
Full text
PDF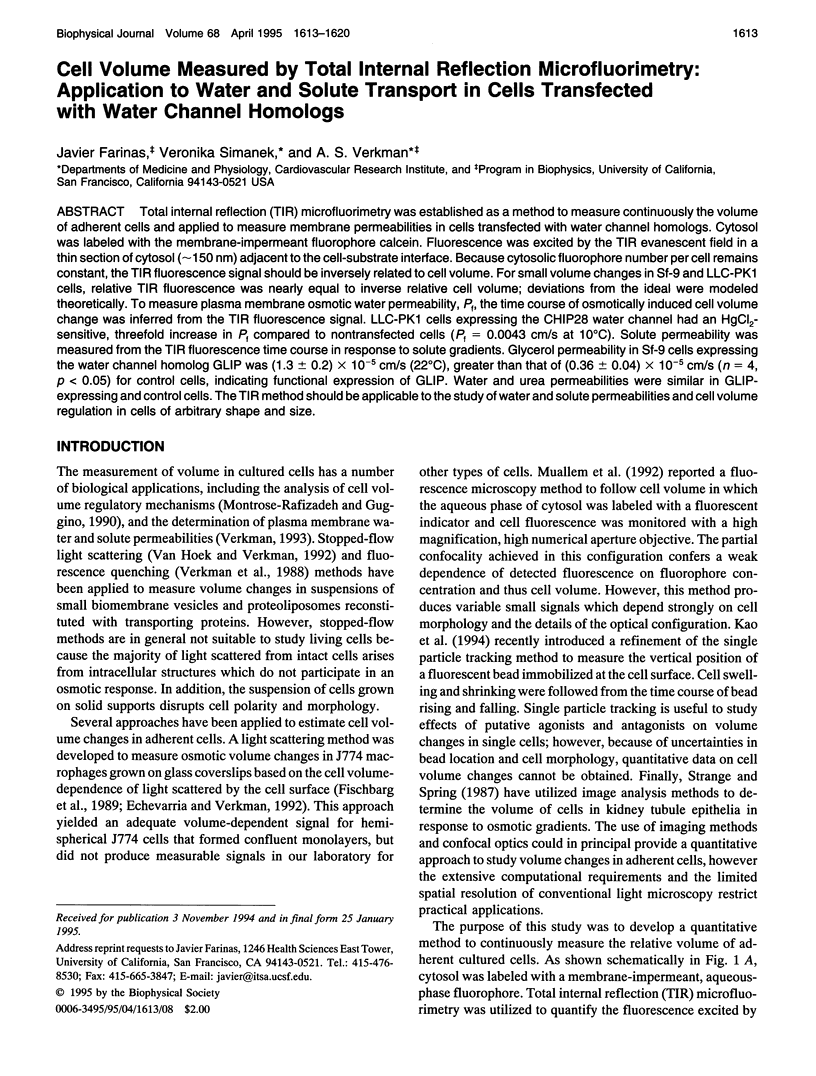
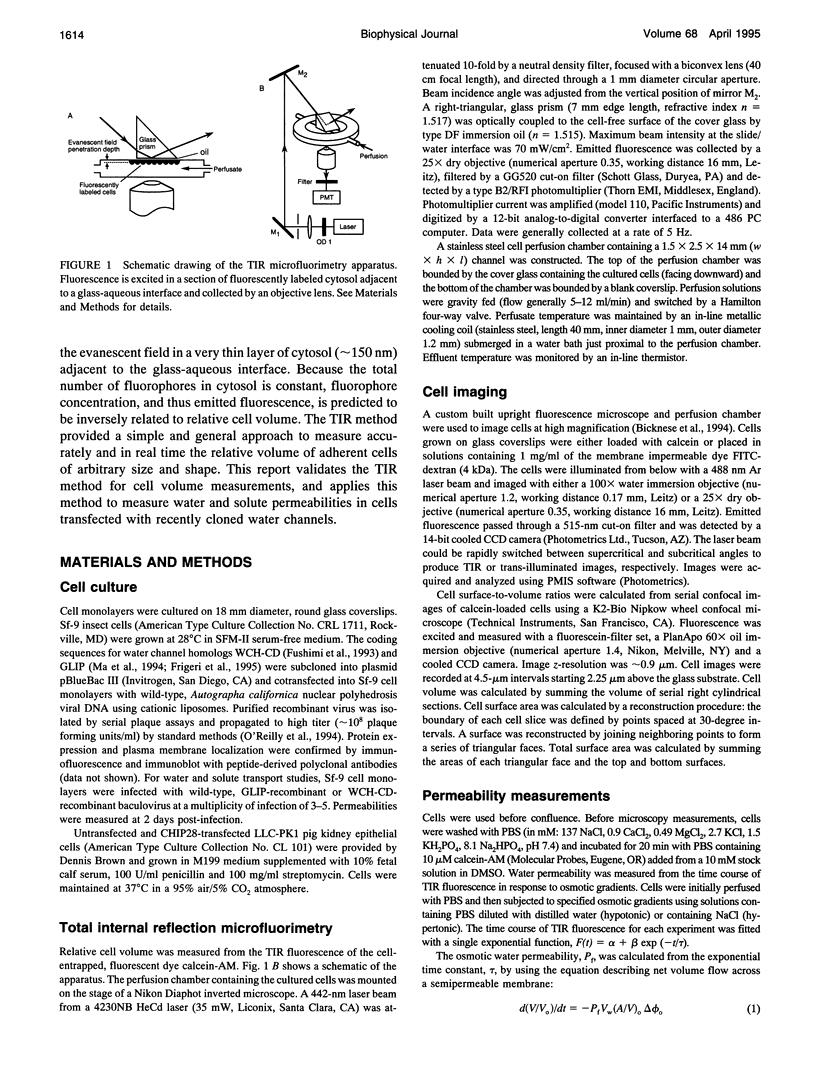

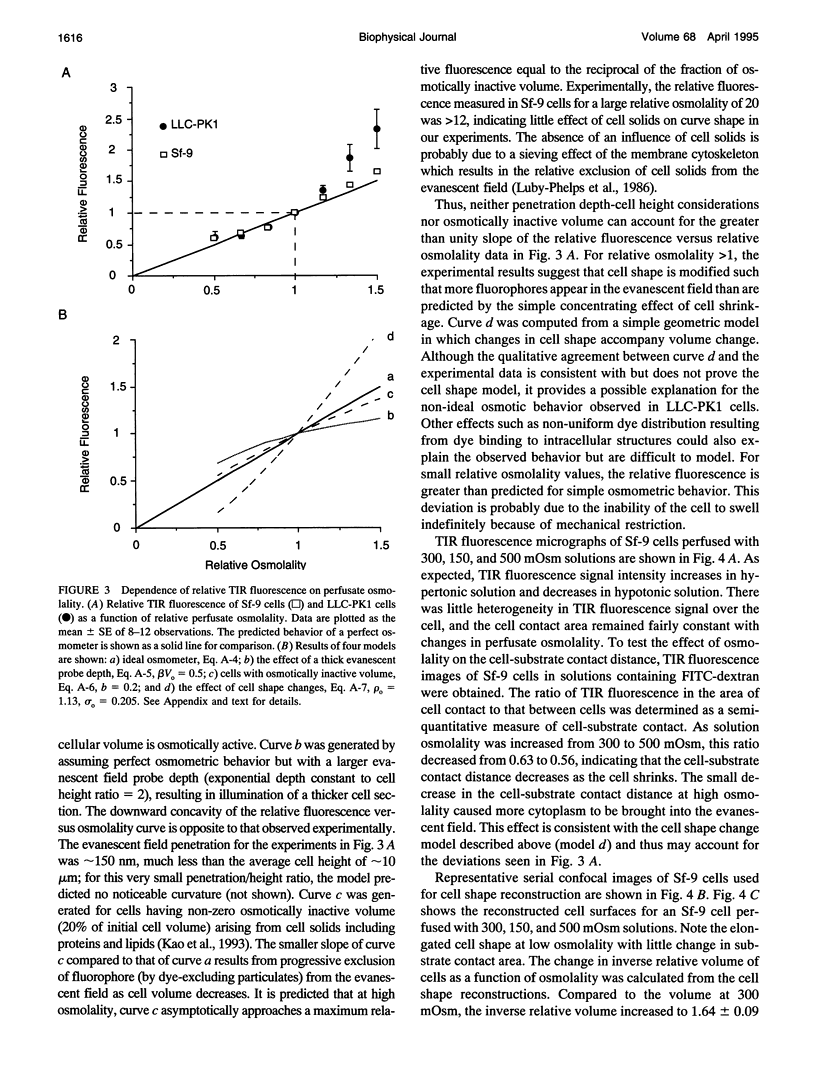
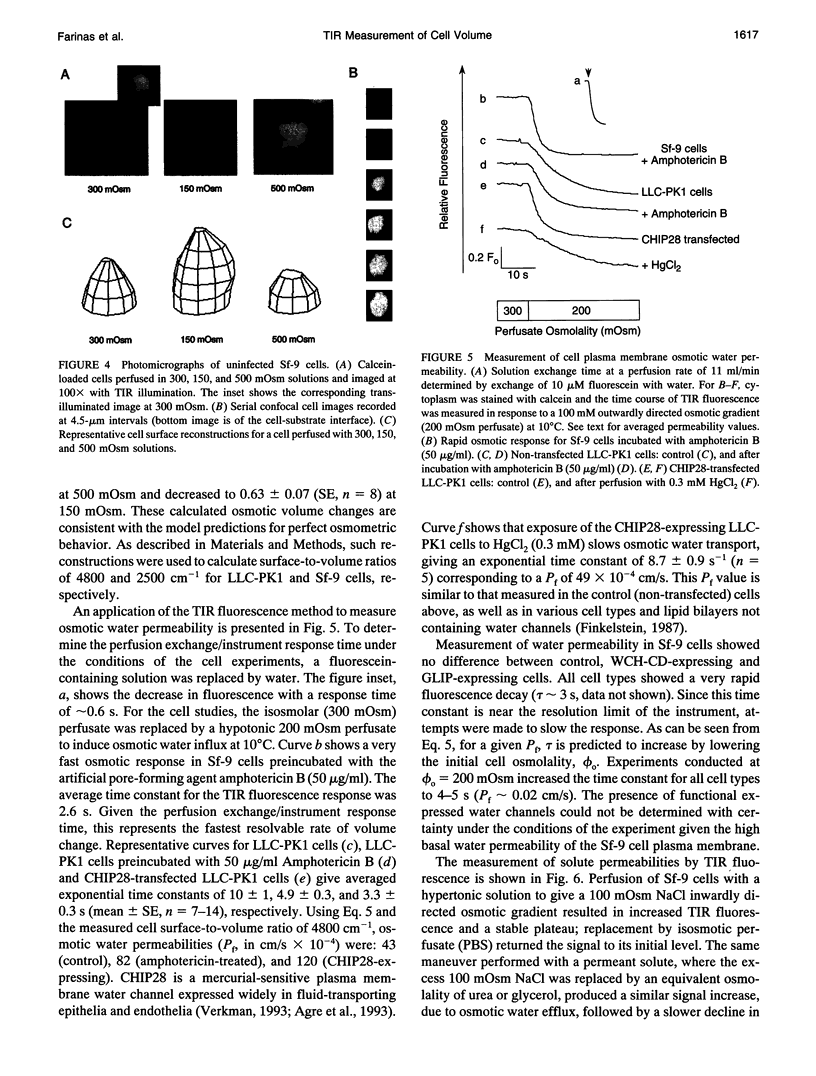
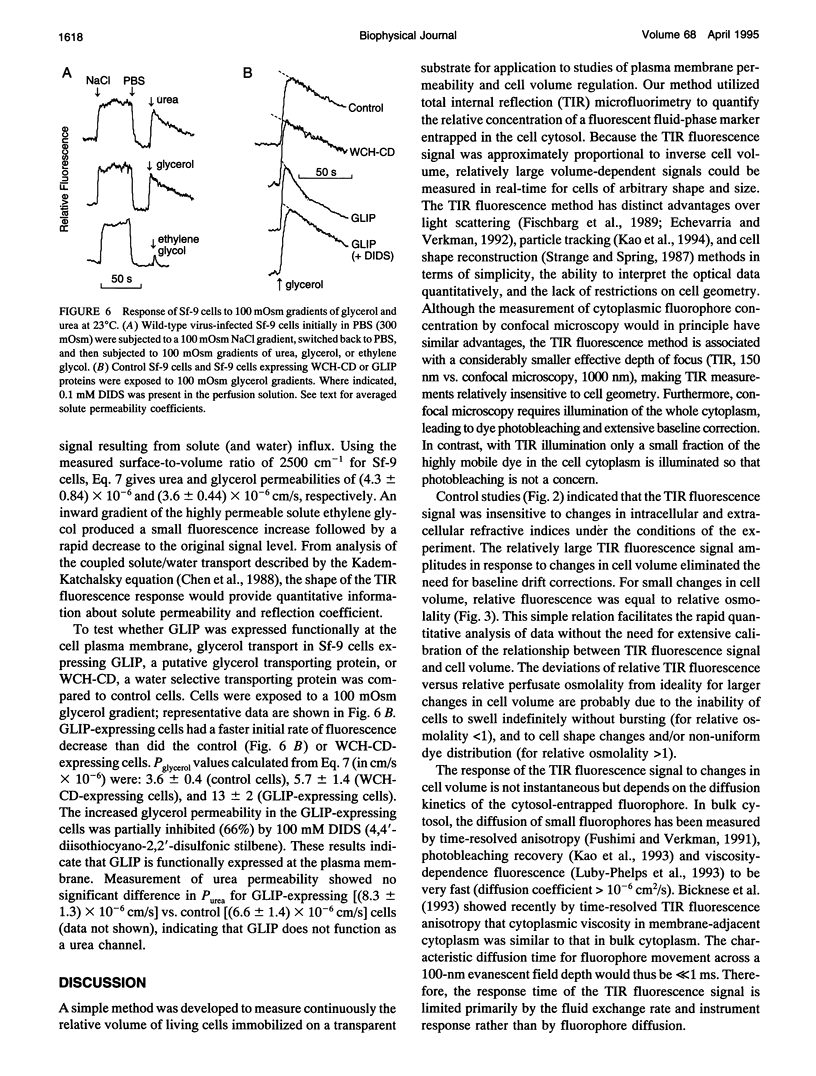
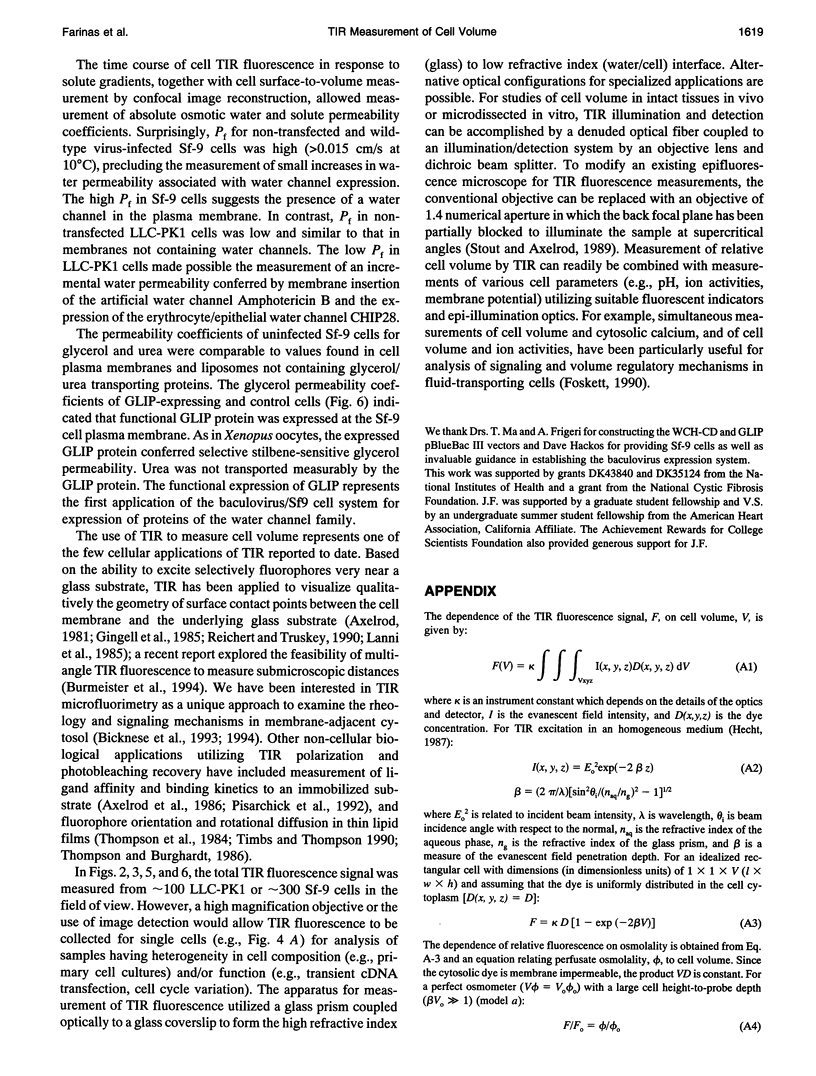
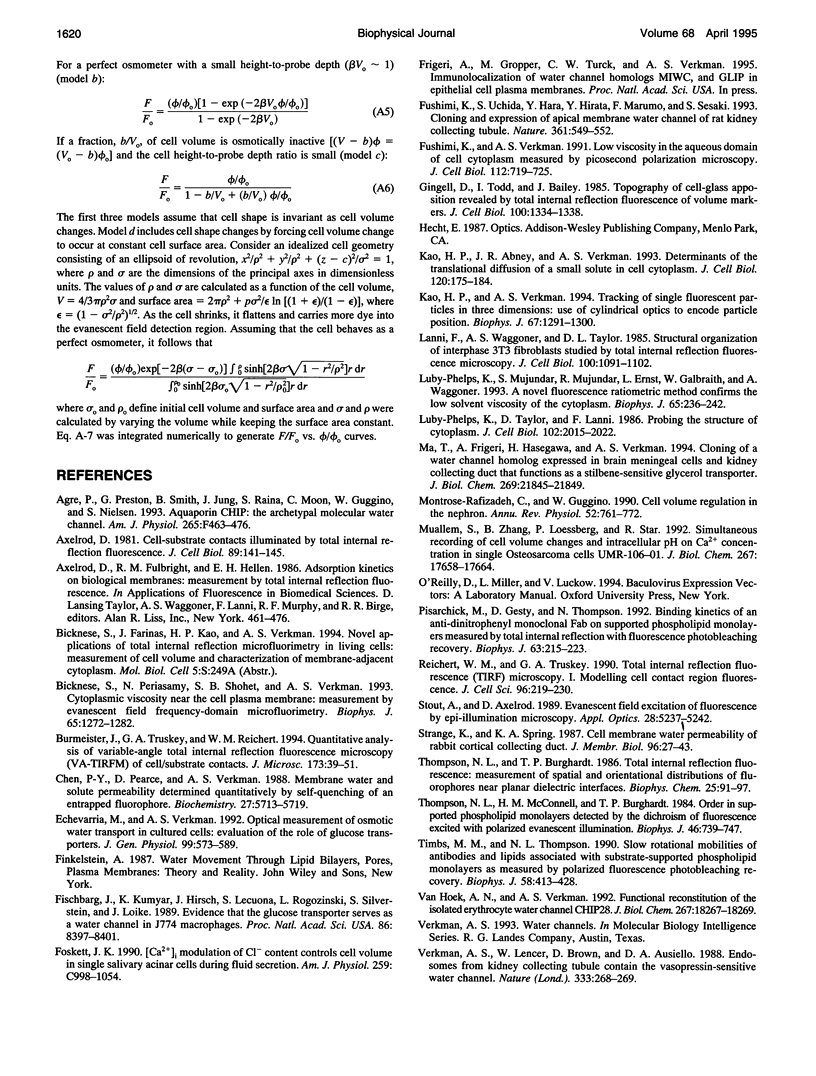
Images in this article
Selected References
These references are in PubMed. This may not be the complete list of references from this article.
- Agre P., Preston G. M., Smith B. L., Jung J. S., Raina S., Moon C., Guggino W. B., Nielsen S. Aquaporin CHIP: the archetypal molecular water channel. Am J Physiol. 1993 Oct;265(4 Pt 2):F463–F476. doi: 10.1152/ajprenal.1993.265.4.F463. [DOI] [PubMed] [Google Scholar]
- Axelrod D. Cell-substrate contacts illuminated by total internal reflection fluorescence. J Cell Biol. 1981 Apr;89(1):141–145. doi: 10.1083/jcb.89.1.141. [DOI] [PMC free article] [PubMed] [Google Scholar]
- Bicknese S., Periasamy N., Shohet S. B., Verkman A. S. Cytoplasmic viscosity near the cell plasma membrane: measurement by evanescent field frequency-domain microfluorimetry. Biophys J. 1993 Sep;65(3):1272–1282. doi: 10.1016/S0006-3495(93)81179-2. [DOI] [PMC free article] [PubMed] [Google Scholar]
- Burmeister J. S., Truskey G. A., Reichert W. M. Quantitative analysis of variable-angle total internal reflection fluorescence microscopy (VA-TIRFM) of cell/substrate contacts. J Microsc. 1994 Jan;173(Pt 1):39–51. doi: 10.1111/j.1365-2818.1994.tb03426.x. [DOI] [PubMed] [Google Scholar]
- Chen P. Y., Pearce D., Verkman A. S. Membrane water and solute permeability determined quantitatively by self-quenching of an entrapped fluorophore. Biochemistry. 1988 Jul 26;27(15):5713–5718. doi: 10.1021/bi00415a048. [DOI] [PubMed] [Google Scholar]
- Echevarria M., Verkman A. S. Optical measurement of osmotic water transport in cultured cells. Role of glucose transporters. J Gen Physiol. 1992 Apr;99(4):573–589. doi: 10.1085/jgp.99.4.573. [DOI] [PMC free article] [PubMed] [Google Scholar]
- Fischbarg J., Kuang K. Y., Hirsch J., Lecuona S., Rogozinski L., Silverstein S. C., Loike J. Evidence that the glucose transporter serves as a water channel in J774 macrophages. Proc Natl Acad Sci U S A. 1989 Nov;86(21):8397–8401. doi: 10.1073/pnas.86.21.8397. [DOI] [PMC free article] [PubMed] [Google Scholar]
- Foskett J. K. [Ca2+]i modulation of Cl- content controls cell volume in single salivary acinar cells during fluid secretion. Am J Physiol. 1990 Dec;259(6 Pt 1):C998–1004. doi: 10.1152/ajpcell.1990.259.6.C998. [DOI] [PubMed] [Google Scholar]
- Fushimi K., Uchida S., Hara Y., Hirata Y., Marumo F., Sasaki S. Cloning and expression of apical membrane water channel of rat kidney collecting tubule. Nature. 1993 Feb 11;361(6412):549–552. doi: 10.1038/361549a0. [DOI] [PubMed] [Google Scholar]
- Fushimi K., Verkman A. S. Low viscosity in the aqueous domain of cell cytoplasm measured by picosecond polarization microfluorimetry. J Cell Biol. 1991 Feb;112(4):719–725. doi: 10.1083/jcb.112.4.719. [DOI] [PMC free article] [PubMed] [Google Scholar]
- Gingell D., Todd I., Bailey J. Topography of cell-glass apposition revealed by total internal reflection fluorescence of volume markers. J Cell Biol. 1985 Apr;100(4):1334–1338. doi: 10.1083/jcb.100.4.1334. [DOI] [PMC free article] [PubMed] [Google Scholar]
- Kao H. P., Abney J. R., Verkman A. S. Determinants of the translational mobility of a small solute in cell cytoplasm. J Cell Biol. 1993 Jan;120(1):175–184. doi: 10.1083/jcb.120.1.175. [DOI] [PMC free article] [PubMed] [Google Scholar]
- Kao H. P., Verkman A. S. Tracking of single fluorescent particles in three dimensions: use of cylindrical optics to encode particle position. Biophys J. 1994 Sep;67(3):1291–1300. doi: 10.1016/S0006-3495(94)80601-0. [DOI] [PMC free article] [PubMed] [Google Scholar]
- Lanni F., Waggoner A. S., Taylor D. L. Structural organization of interphase 3T3 fibroblasts studied by total internal reflection fluorescence microscopy. J Cell Biol. 1985 Apr;100(4):1091–1102. doi: 10.1083/jcb.100.4.1091. [DOI] [PMC free article] [PubMed] [Google Scholar]
- Luby-Phelps K., Mujumdar S., Mujumdar R. B., Ernst L. A., Galbraith W., Waggoner A. S. A novel fluorescence ratiometric method confirms the low solvent viscosity of the cytoplasm. Biophys J. 1993 Jul;65(1):236–242. doi: 10.1016/S0006-3495(93)81075-0. [DOI] [PMC free article] [PubMed] [Google Scholar]
- Luby-Phelps K., Taylor D. L., Lanni F. Probing the structure of cytoplasm. J Cell Biol. 1986 Jun;102(6):2015–2022. doi: 10.1083/jcb.102.6.2015. [DOI] [PMC free article] [PubMed] [Google Scholar]
- Ma T., Frigeri A., Hasegawa H., Verkman A. S. Cloning of a water channel homolog expressed in brain meningeal cells and kidney collecting duct that functions as a stilbene-sensitive glycerol transporter. J Biol Chem. 1994 Aug 26;269(34):21845–21849. [PubMed] [Google Scholar]
- Montrose-Rafizadeh C., Guggino W. B. Cell volume regulation in the nephron. Annu Rev Physiol. 1990;52:761–772. doi: 10.1146/annurev.ph.52.030190.003553. [DOI] [PubMed] [Google Scholar]
- Muallem S., Zhang B. X., Loessberg P. A., Star R. A. Simultaneous recording of cell volume changes and intracellular pH or Ca2+ concentration in single osteosarcoma cells UMR-106-01. J Biol Chem. 1992 Sep 5;267(25):17658–17664. [PubMed] [Google Scholar]
- Pisarchick M. L., Gesty D., Thompson N. L. Binding kinetics of an anti-dinitrophenyl monoclonal Fab on supported phospholipid monolayers measured by total internal reflection with fluorescence photobleaching recovery. Biophys J. 1992 Jul;63(1):215–223. doi: 10.1016/S0006-3495(92)81592-8. [DOI] [PMC free article] [PubMed] [Google Scholar]
- Reichert W. M., Truskey G. A. Total internal reflection fluorescence (TIRF) microscopy. I. Modelling cell contact region fluorescence. J Cell Sci. 1990 Jun;96(Pt 2):219–230. doi: 10.1242/jcs.96.2.219. [DOI] [PubMed] [Google Scholar]
- Strange K., Spring K. R. Cell membrane water permeability of rabbit cortical collecting duct. J Membr Biol. 1987;96(1):27–43. doi: 10.1007/BF01869332. [DOI] [PubMed] [Google Scholar]
- Thompson N. L., Burghardt T. P. Total internal reflection fluorescence. Measurement of spatial and orientational distributions of fluorophores near planar dielectric interfaces. Biophys Chem. 1986 Nov;25(1):91–97. doi: 10.1016/0301-4622(86)85069-4. [DOI] [PubMed] [Google Scholar]
- Thompson N. L., McConnell H. M., Burhardt T. P. Order in supported phospholipid monolayers detected by the dichroism of fluorescence excited with polarized evanescent illumination. Biophys J. 1984 Dec;46(6):739–747. doi: 10.1016/S0006-3495(84)84072-2. [DOI] [PMC free article] [PubMed] [Google Scholar]
- Timbs M. M., Thompson N. L. Slow rotational mobilities of antibodies and lipids associated with substrate-supported phospholipid monolayers as measured by polarized fluorescence photobleaching recovery. Biophys J. 1990 Aug;58(2):413–428. doi: 10.1016/S0006-3495(90)82387-0. [DOI] [PMC free article] [PubMed] [Google Scholar]
- Verkman A. S., Lencer W. I., Brown D., Ausiello D. A. Endosomes from kidney collecting tubule cells contain the vasopressin-sensitive water channel. Nature. 1988 May 19;333(6170):268–269. doi: 10.1038/333268a0. [DOI] [PubMed] [Google Scholar]
- van Hoek A. N., Verkman A. S. Functional reconstitution of the isolated erythrocyte water channel CHIP28. J Biol Chem. 1992 Sep 15;267(26):18267–18269. [PubMed] [Google Scholar]