Abstract
Electroporation involves the application of an electric field pulse that creates transient aqueous pathways in lipid bilayer membranes. Transport through these pathways can occur by different mechanisms during and after a pulse. To determine the time scale of transport and the mechanism(s) by which it occurs, efflux of a fluorescent molecule, calcein, across erythrocyte ghost membranes was measured with a fluorescence microscope photometer with millisecond time resolution during and after electroporation pulses several milliseconds in duration. One of four outcomes was typically observed. Ghosts were: (1) partially emptied of calcein, involving efflux primarily after the pulse; (2) completely emptied of calcein, involving efflux primarily after the pulse; (3) completely emptied of calcein, involving efflux both during and after the pulse; or (4) completely emptied of calcein, involving efflux primarily during the pulse. Partial emptying, involving significant efflux during the pulse, was generally not observed. We conclude that under some conditions transport caused by electroporation occurs predominantly by electrophoresis and/or electroosmosis during a pulse, although under other conditions transport occurs in part or almost completely by diffusion within milliseconds to seconds after a pulse.
Full text
PDF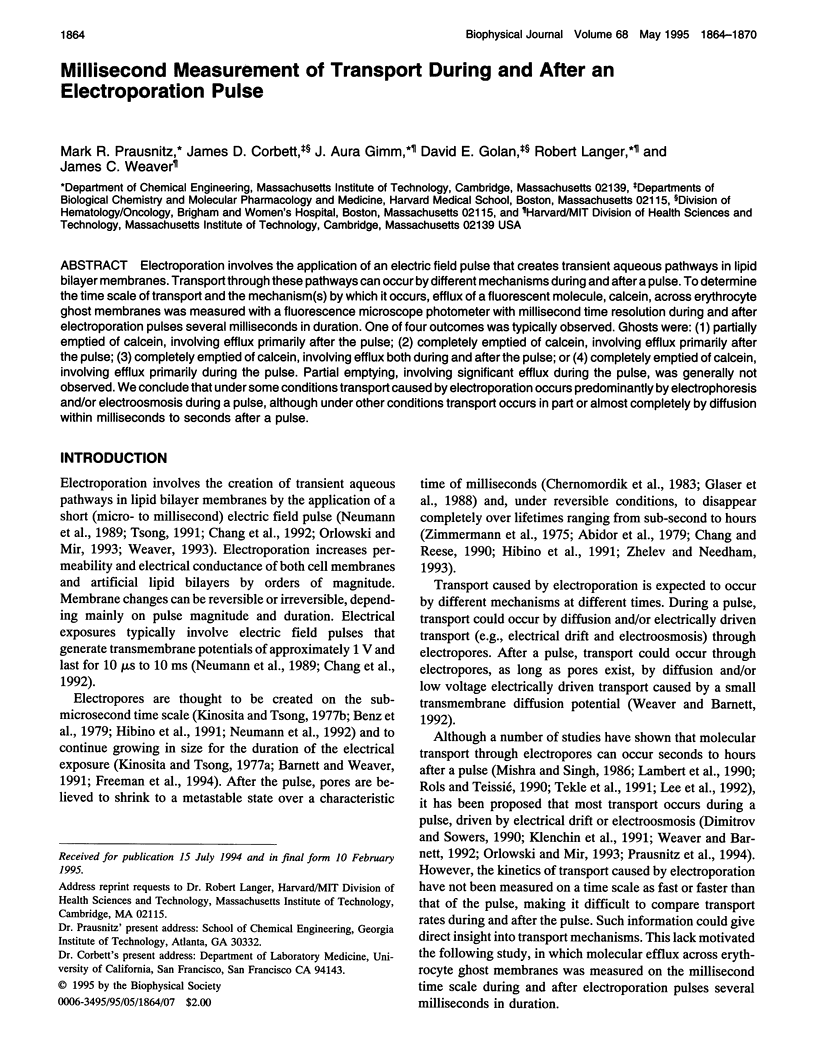
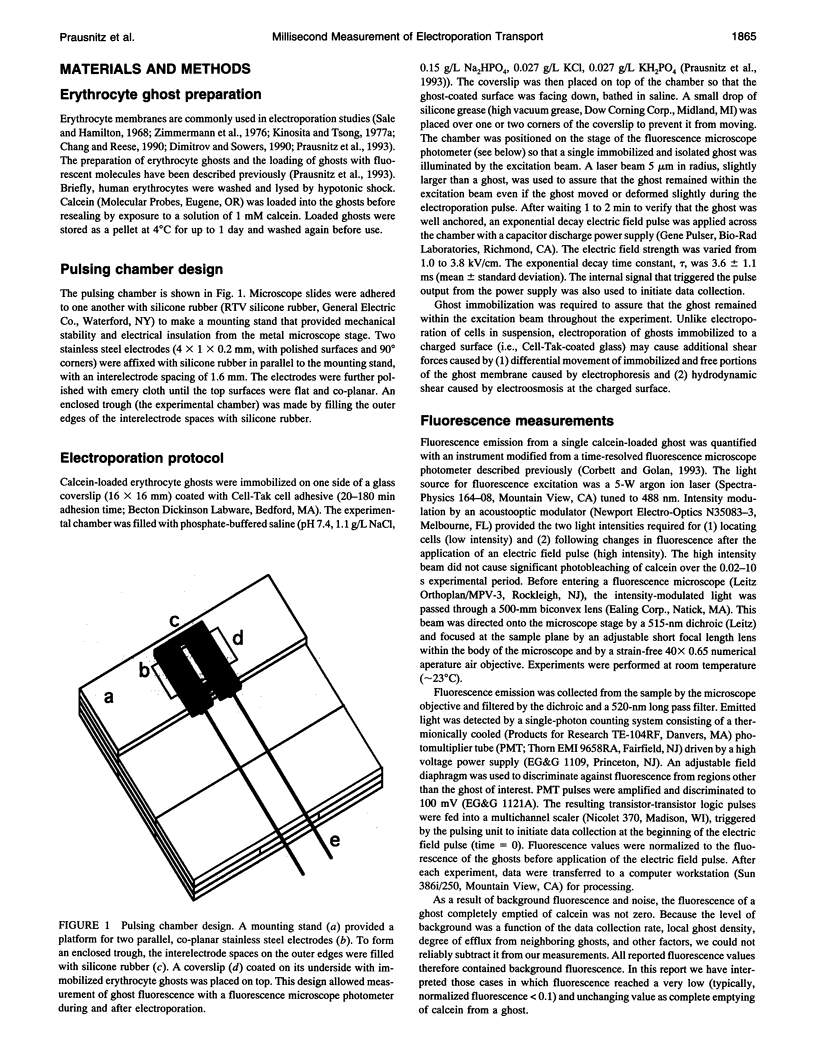
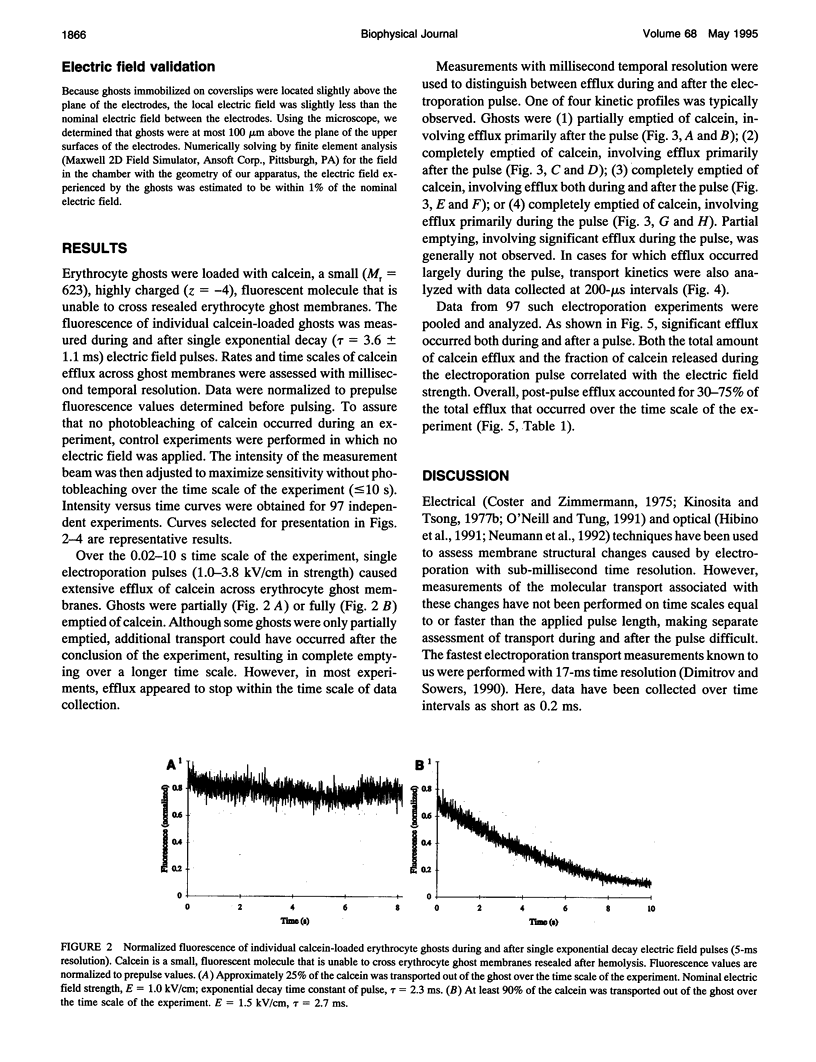
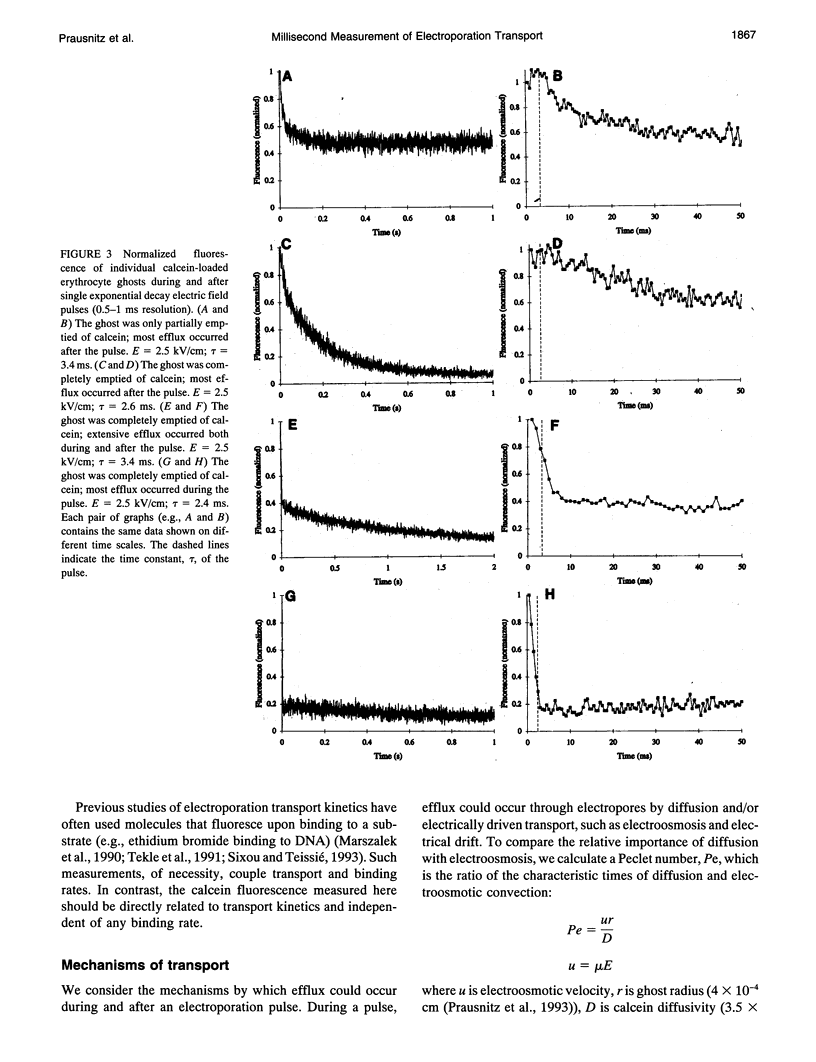
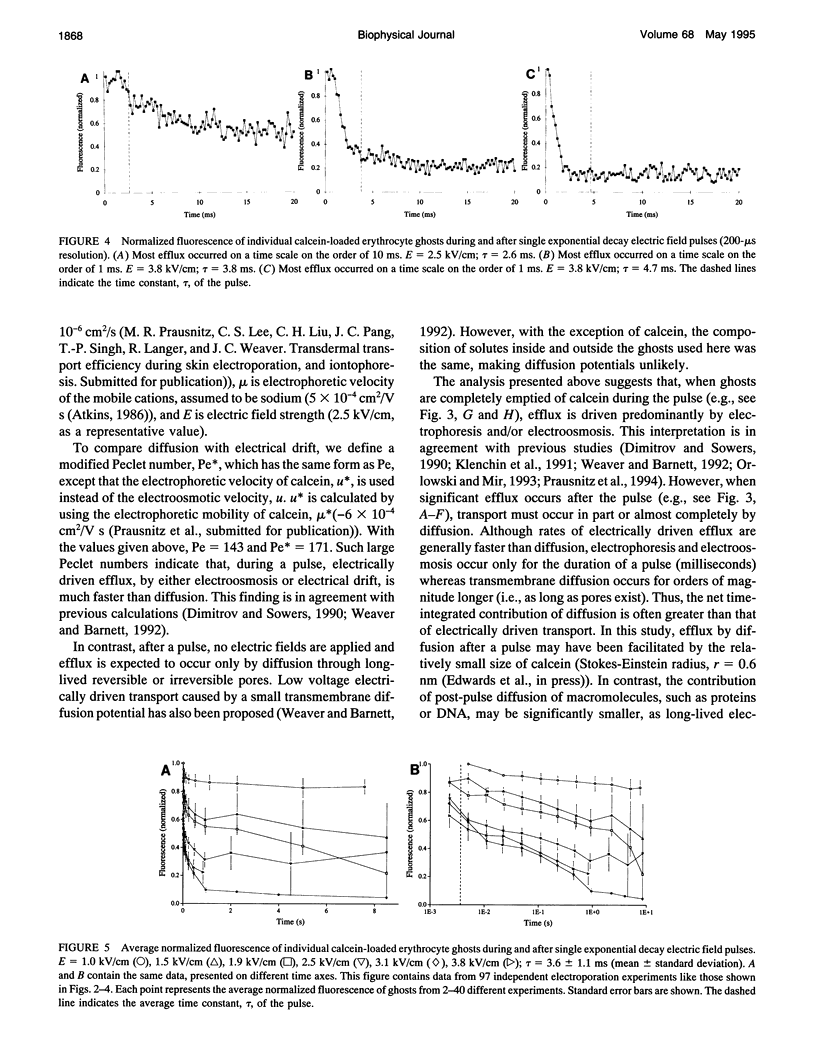
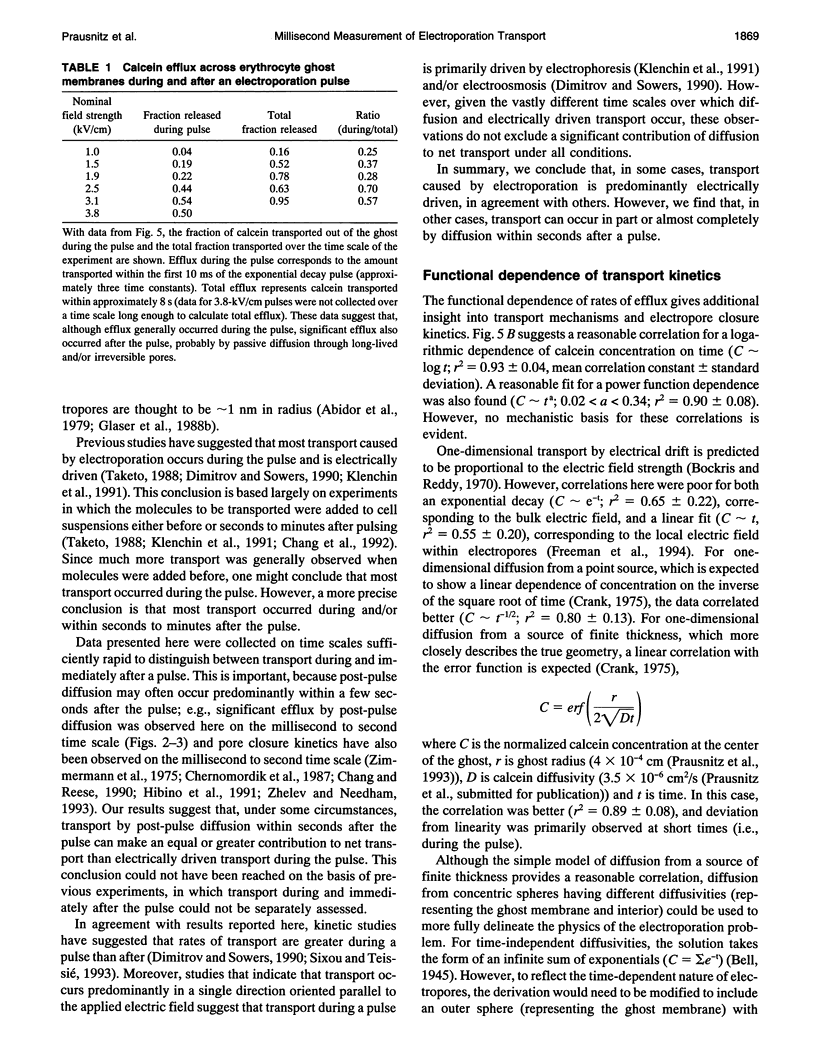
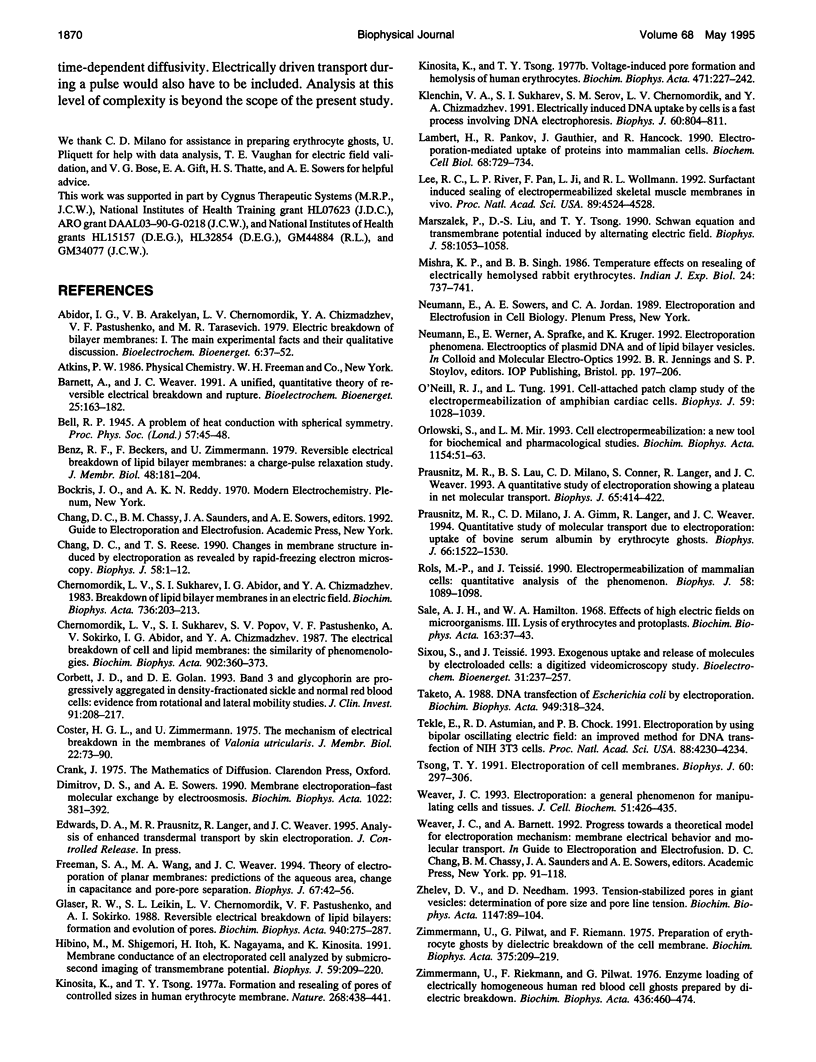
Selected References
These references are in PubMed. This may not be the complete list of references from this article.
- Benz R., Beckers F., Zimmermann U. Reversible electrical breakdown of lipid bilayer membranes: a charge-pulse relaxation study. J Membr Biol. 1979 Jul 16;48(2):181–204. doi: 10.1007/BF01872858. [DOI] [PubMed] [Google Scholar]
- Chang D. C., Reese T. S. Changes in membrane structure induced by electroporation as revealed by rapid-freezing electron microscopy. Biophys J. 1990 Jul;58(1):1–12. doi: 10.1016/S0006-3495(90)82348-1. [DOI] [PMC free article] [PubMed] [Google Scholar]
- Chernomordik L. V., Sukharev S. I., Popov S. V., Pastushenko V. F., Sokirko A. V., Abidor I. G., Chizmadzhev Y. A. The electrical breakdown of cell and lipid membranes: the similarity of phenomenologies. Biochim Biophys Acta. 1987 Sep 3;902(3):360–373. doi: 10.1016/0005-2736(87)90204-5. [DOI] [PubMed] [Google Scholar]
- Corbett J. D., Golan D. E. Band 3 and glycophorin are progressively aggregated in density-fractionated sickle and normal red blood cells. Evidence from rotational and lateral mobility studies. J Clin Invest. 1993 Jan;91(1):208–217. doi: 10.1172/JCI116172. [DOI] [PMC free article] [PubMed] [Google Scholar]
- Coster H. G., Simmermann U. The mechanism of electrical breakdown in the membranes of Valonai utricularis. J Membr Biol. 1975 Jun 3;22(1):73–90. doi: 10.1007/BF01868164. [DOI] [PubMed] [Google Scholar]
- Dimitrov D. S., Sowers A. E. Membrane electroporation--fast molecular exchange by electroosmosis. Biochim Biophys Acta. 1990 Mar;1022(3):381–392. doi: 10.1016/0005-2736(90)90289-z. [DOI] [PubMed] [Google Scholar]
- Freeman S. A., Wang M. A., Weaver J. C. Theory of electroporation of planar bilayer membranes: predictions of the aqueous area, change in capacitance, and pore-pore separation. Biophys J. 1994 Jul;67(1):42–56. doi: 10.1016/S0006-3495(94)80453-9. [DOI] [PMC free article] [PubMed] [Google Scholar]
- Glaser R. W., Leikin S. L., Chernomordik L. V., Pastushenko V. F., Sokirko A. I. Reversible electrical breakdown of lipid bilayers: formation and evolution of pores. Biochim Biophys Acta. 1988 May 24;940(2):275–287. doi: 10.1016/0005-2736(88)90202-7. [DOI] [PubMed] [Google Scholar]
- Hibino M., Shigemori M., Itoh H., Nagayama K., Kinosita K., Jr Membrane conductance of an electroporated cell analyzed by submicrosecond imaging of transmembrane potential. Biophys J. 1991 Jan;59(1):209–220. doi: 10.1016/S0006-3495(91)82212-3. [DOI] [PMC free article] [PubMed] [Google Scholar]
- Kinosita K., Jr, Tsong T. Y. Formation and resealing of pores of controlled sizes in human erythrocyte membrane. Nature. 1977 Aug 4;268(5619):438–441. doi: 10.1038/268438a0. [DOI] [PubMed] [Google Scholar]
- Kinosita K., Jr, Tsong T. Y. Voltage-induced pore formation and hemolysis of human erythrocytes. Biochim Biophys Acta. 1977 Dec 1;471(2):227–242. doi: 10.1016/0005-2736(77)90252-8. [DOI] [PubMed] [Google Scholar]
- Klenchin V. A., Sukharev S. I., Serov S. M., Chernomordik L. V., Chizmadzhev YuA Electrically induced DNA uptake by cells is a fast process involving DNA electrophoresis. Biophys J. 1991 Oct;60(4):804–811. doi: 10.1016/S0006-3495(91)82115-4. [DOI] [PMC free article] [PubMed] [Google Scholar]
- Lambert H., Pankov R., Gauthier J., Hancock R. Electroporation-mediated uptake of proteins into mammalian cells. Biochem Cell Biol. 1990 Apr;68(4):729–734. doi: 10.1139/o90-105. [DOI] [PubMed] [Google Scholar]
- Lee R. C., River L. P., Pan F. S., Ji L., Wollmann R. L. Surfactant-induced sealing of electropermeabilized skeletal muscle membranes in vivo. Proc Natl Acad Sci U S A. 1992 May 15;89(10):4524–4528. doi: 10.1073/pnas.89.10.4524. [DOI] [PMC free article] [PubMed] [Google Scholar]
- Marszalek P., Liu D. S., Tsong T. Y. Schwan equation and transmembrane potential induced by alternating electric field. Biophys J. 1990 Oct;58(4):1053–1058. doi: 10.1016/S0006-3495(90)82447-4. [DOI] [PMC free article] [PubMed] [Google Scholar]
- Mishra K. P., Singh B. B. Temperature effects on resealing of electrically hemolysed rabbit erythrocytes. Indian J Exp Biol. 1986 Dec;24(12):737–741. [PubMed] [Google Scholar]
- O'Neill R. J., Tung L. Cell-attached patch clamp study of the electropermeabilization of amphibian cardiac cells. Biophys J. 1991 May;59(5):1028–1039. doi: 10.1016/S0006-3495(91)82318-9. [DOI] [PMC free article] [PubMed] [Google Scholar]
- Orlowski S., Mir L. M. Cell electropermeabilization: a new tool for biochemical and pharmacological studies. Biochim Biophys Acta. 1993 Jun 8;1154(1):51–63. doi: 10.1016/0304-4157(93)90016-h. [DOI] [PubMed] [Google Scholar]
- Prausnitz M. R., Lau B. S., Milano C. D., Conner S., Langer R., Weaver J. C. A quantitative study of electroporation showing a plateau in net molecular transport. Biophys J. 1993 Jul;65(1):414–422. doi: 10.1016/S0006-3495(93)81081-6. [DOI] [PMC free article] [PubMed] [Google Scholar]
- Prausnitz M. R., Milano C. D., Gimm J. A., Langer R., Weaver J. C. Quantitative study of molecular transport due to electroporation: uptake of bovine serum albumin by erythrocyte ghosts. Biophys J. 1994 May;66(5):1522–1530. doi: 10.1016/S0006-3495(94)80943-9. [DOI] [PMC free article] [PubMed] [Google Scholar]
- Rols M. P., Teissié J. Electropermeabilization of mammalian cells. Quantitative analysis of the phenomenon. Biophys J. 1990 Nov;58(5):1089–1098. doi: 10.1016/S0006-3495(90)82451-6. [DOI] [PMC free article] [PubMed] [Google Scholar]
- Sale A. J., Hamilton W. A. Effects of high electric fields on micro-organisms. 3. Lysis of erythrocytes and protoplasts. Biochim Biophys Acta. 1968 Aug;163(1):37–43. doi: 10.1016/0005-2736(68)90030-8. [DOI] [PubMed] [Google Scholar]
- Taketo A. DNA transfection of Escherichia coli by electroporation. Biochim Biophys Acta. 1988 Mar 31;949(3):318–324. doi: 10.1016/0167-4781(88)90158-3. [DOI] [PubMed] [Google Scholar]
- Tekle E., Astumian R. D., Chock P. B. Electroporation by using bipolar oscillating electric field: an improved method for DNA transfection of NIH 3T3 cells. Proc Natl Acad Sci U S A. 1991 May 15;88(10):4230–4234. doi: 10.1073/pnas.88.10.4230. [DOI] [PMC free article] [PubMed] [Google Scholar]
- Tsong T. Y. Electroporation of cell membranes. Biophys J. 1991 Aug;60(2):297–306. doi: 10.1016/S0006-3495(91)82054-9. [DOI] [PMC free article] [PubMed] [Google Scholar]
- Weaver J. C. Electroporation: a general phenomenon for manipulating cells and tissues. J Cell Biochem. 1993 Apr;51(4):426–435. doi: 10.1002/jcb.2400510407. [DOI] [PubMed] [Google Scholar]
- Zhelev D. V., Needham D. Tension-stabilized pores in giant vesicles: determination of pore size and pore line tension. Biochim Biophys Acta. 1993 Apr 8;1147(1):89–104. doi: 10.1016/0005-2736(93)90319-u. [DOI] [PubMed] [Google Scholar]
- Zimmermann U., Pilwat G., Riemann F. Preparation of erythrocyte ghosts by dielectric breakdown of the cell membrane. Biochim Biophys Acta. 1975 Jan 28;375(2):209–219. doi: 10.1016/0005-2736(75)90189-3. [DOI] [PubMed] [Google Scholar]
- Zimmermann U., Riemann F., Pilwat G. Enzyme loading of electrically homogeneous human red blood cell ghosts prepared by dielelctric breakdown. Biochim Biophys Acta. 1976 Jun 17;436(2):460–474. doi: 10.1016/0005-2736(76)90208-x. [DOI] [PubMed] [Google Scholar]