Abstract
A mechanical test with micropipets is used to characterize cytoskeleton rearrangement and contraction induced by mechanical stresses in human neutrophils. The yield shear resultant of the cell cortex is on the order of 0.06 to 0.09 mN.m-1. The measured yield shear resultant suggests that the neutrophil cortex is a weakly cross-linked structure. When a tether is pulled out from the cell surface, a polymer structure starts to fill it and spreads out from the cell body. The rate of advancement of the polymerization front is almost constant and, therefore, is not diffusion limited. The measured rate is much smaller than the one of spontaneous actin polymerization, suggesting that the limiting process is either the dissociation of actin monomers from their dimers with the capping proteins or the rate of formation of new nucleation sites or both. Polymerization is also observed after applying sufficient mechanical stresses on a small portion of the cell surface. The polymerization is followed by mass transfer from the cell into the prestressed region and later on by contraction of the main cell body. The pressure generating the flow is located in the prestressed region and most probably is a result of its "swelling" and contraction. The contraction of the main cell body is very similar (in its time dependence and magnitude) to the contraction during phagocytosis. The measured maximum cortical tension is on the order of 0.5 mN.m-1, which for a 3.5-microns diameter pipet corresponds to a maximum contraction force of 11 nN.
Full text
PDF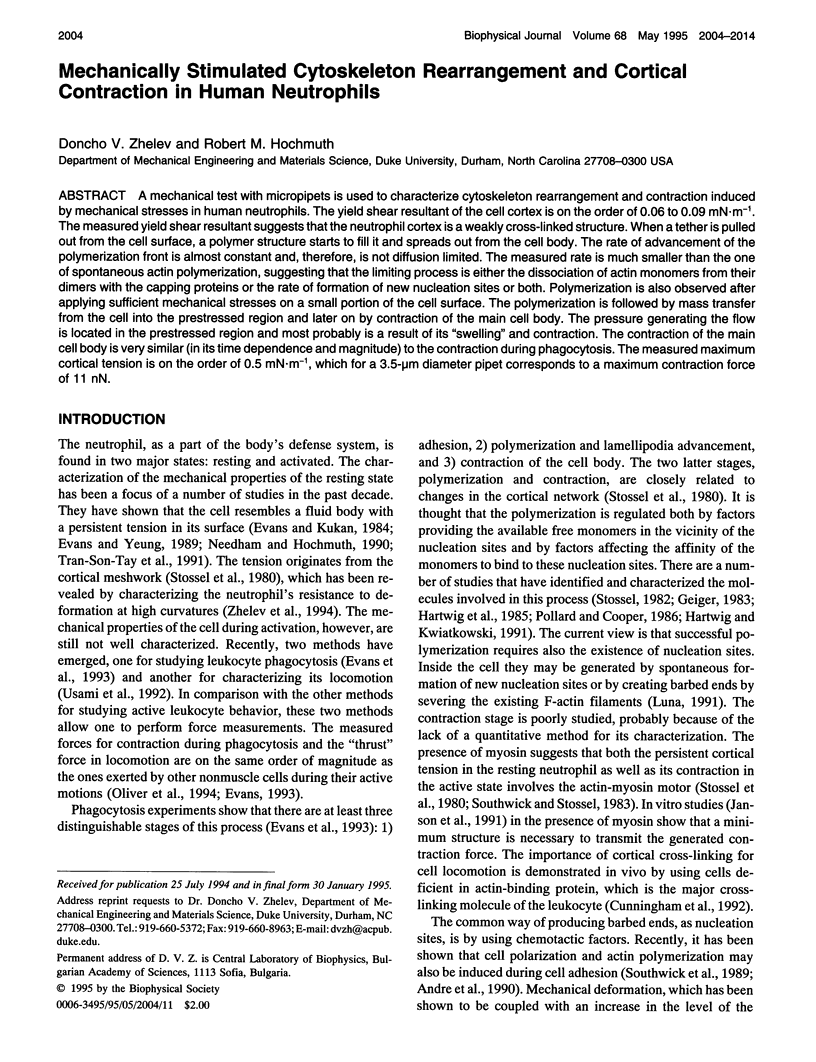
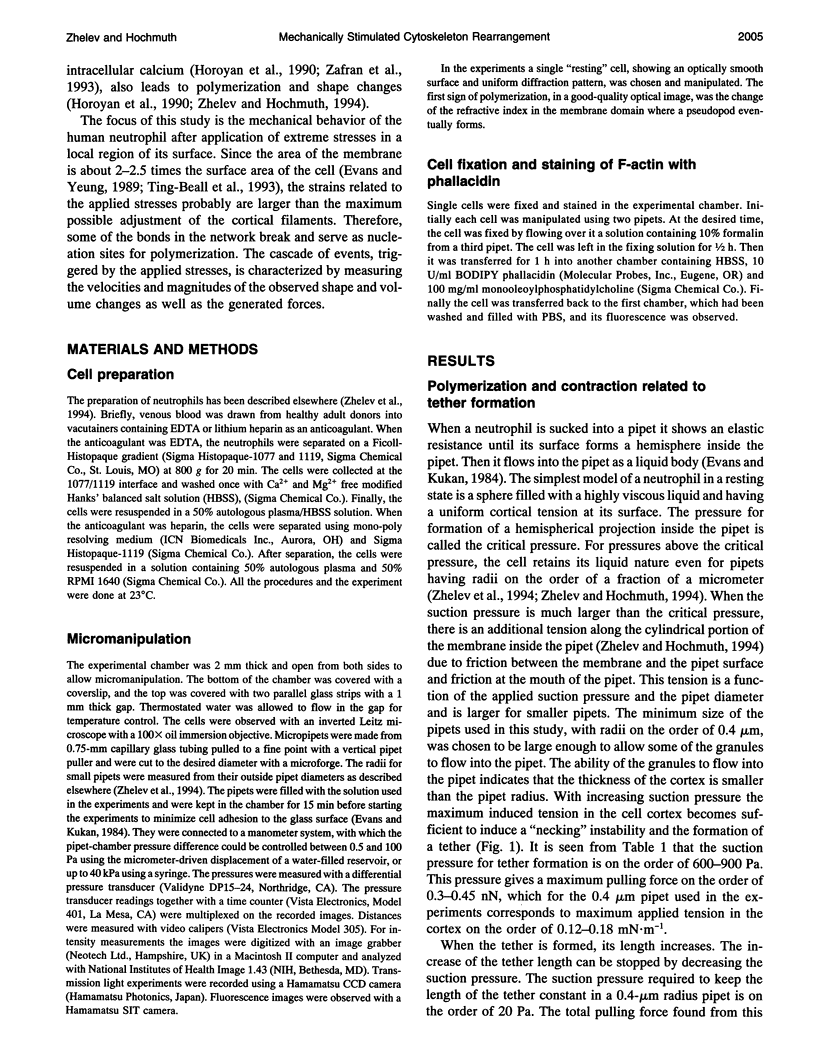
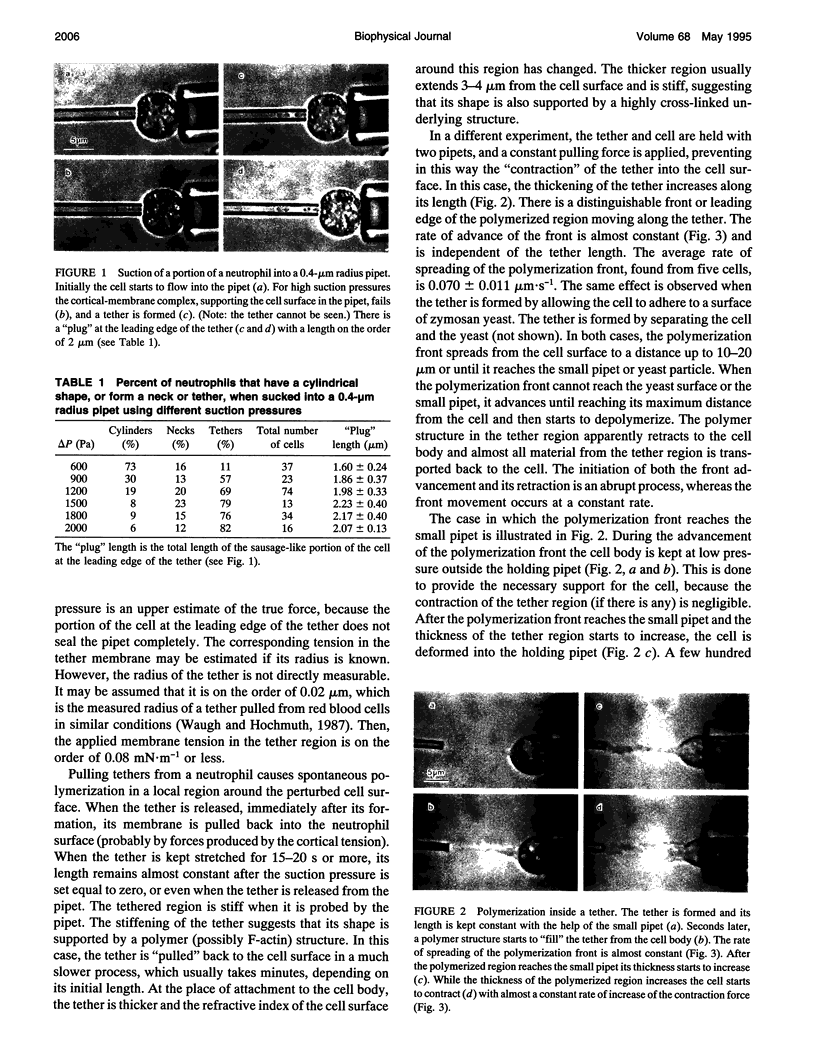
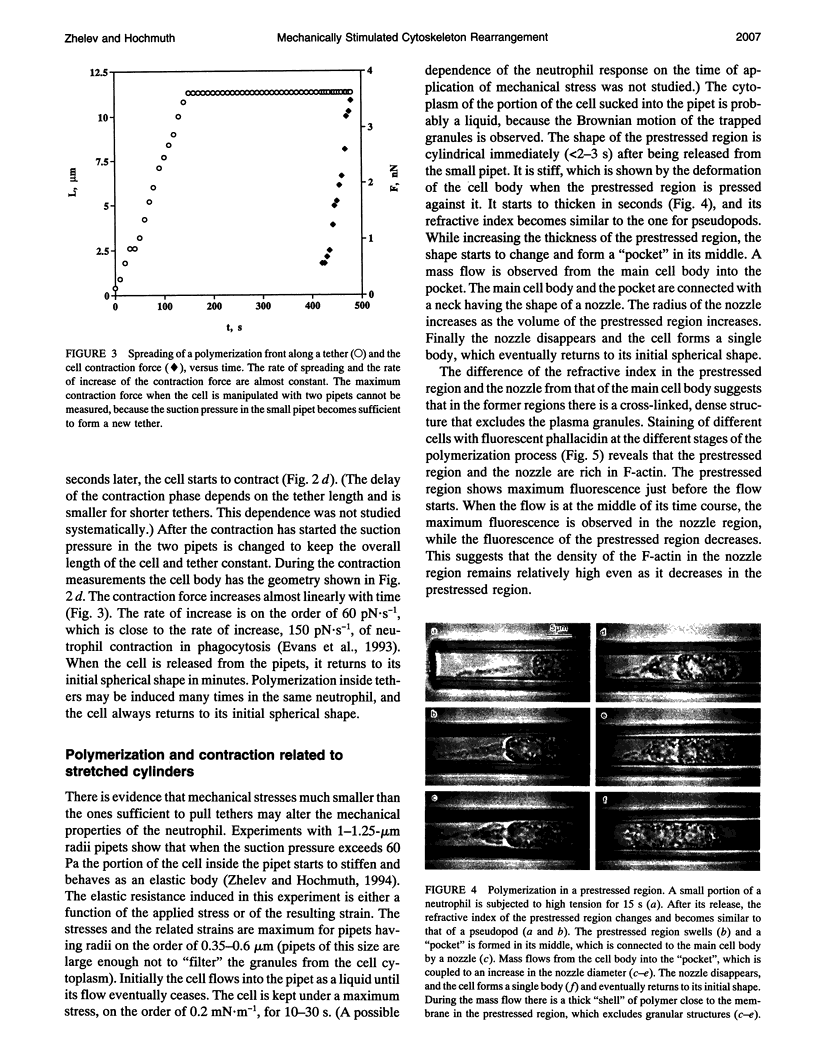
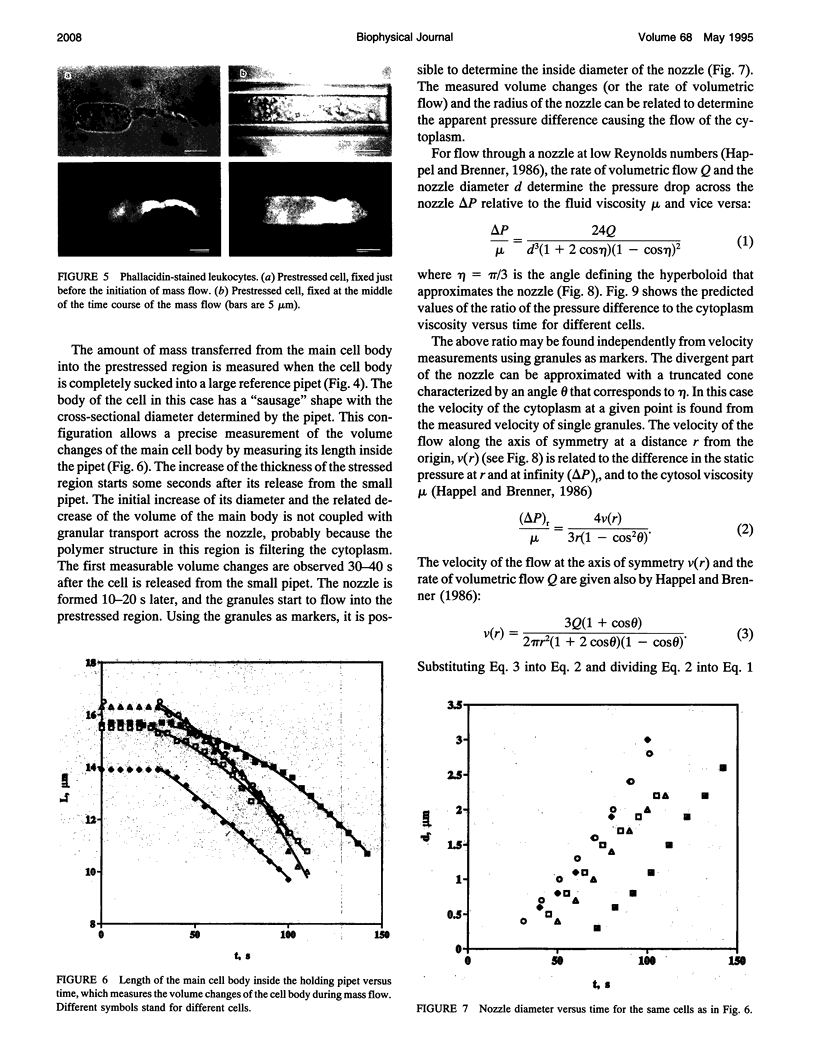
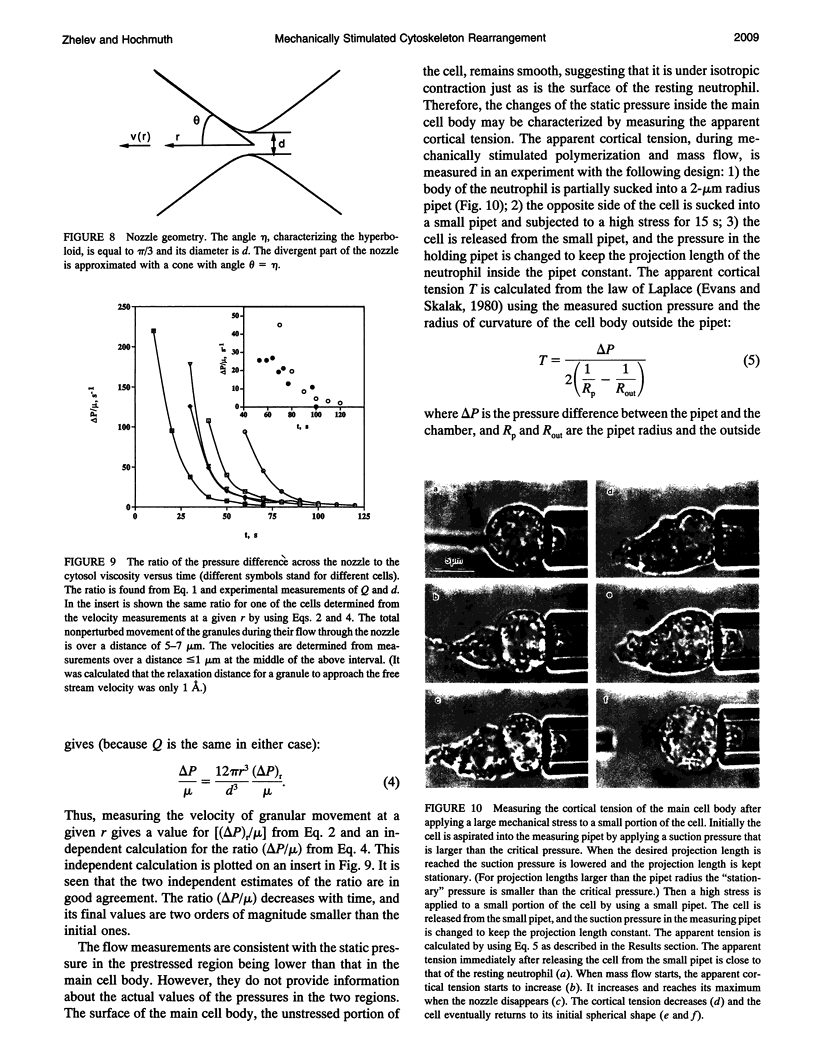
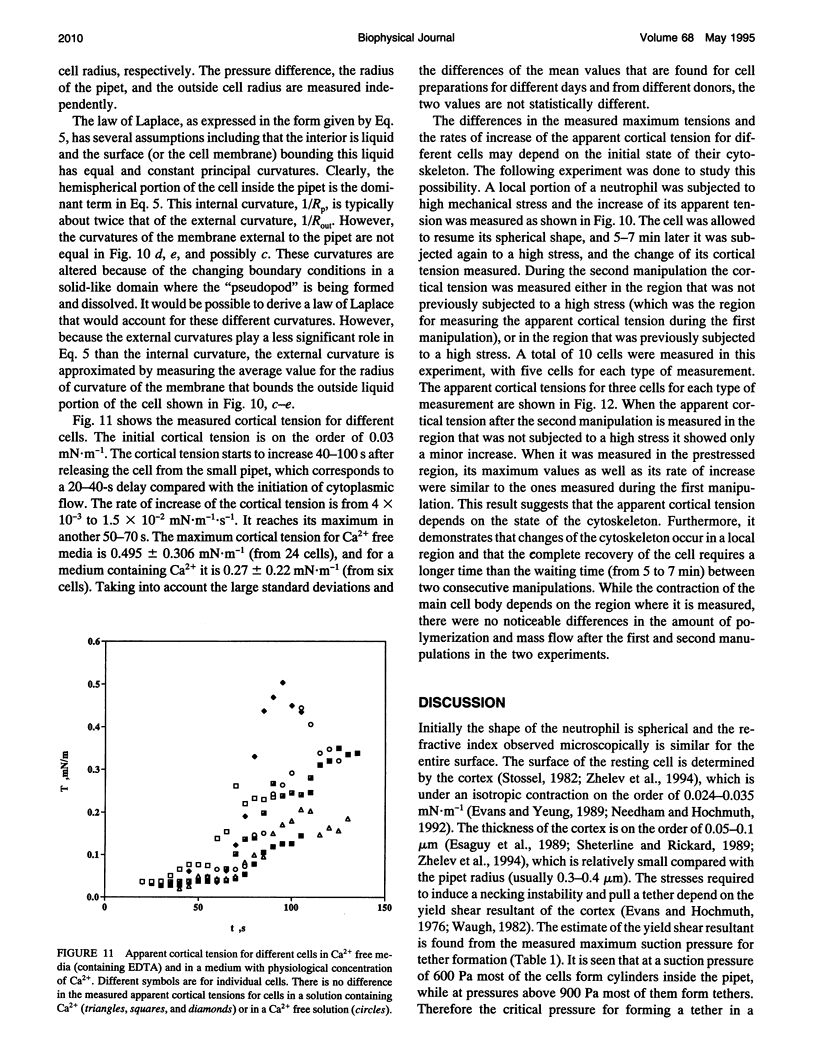
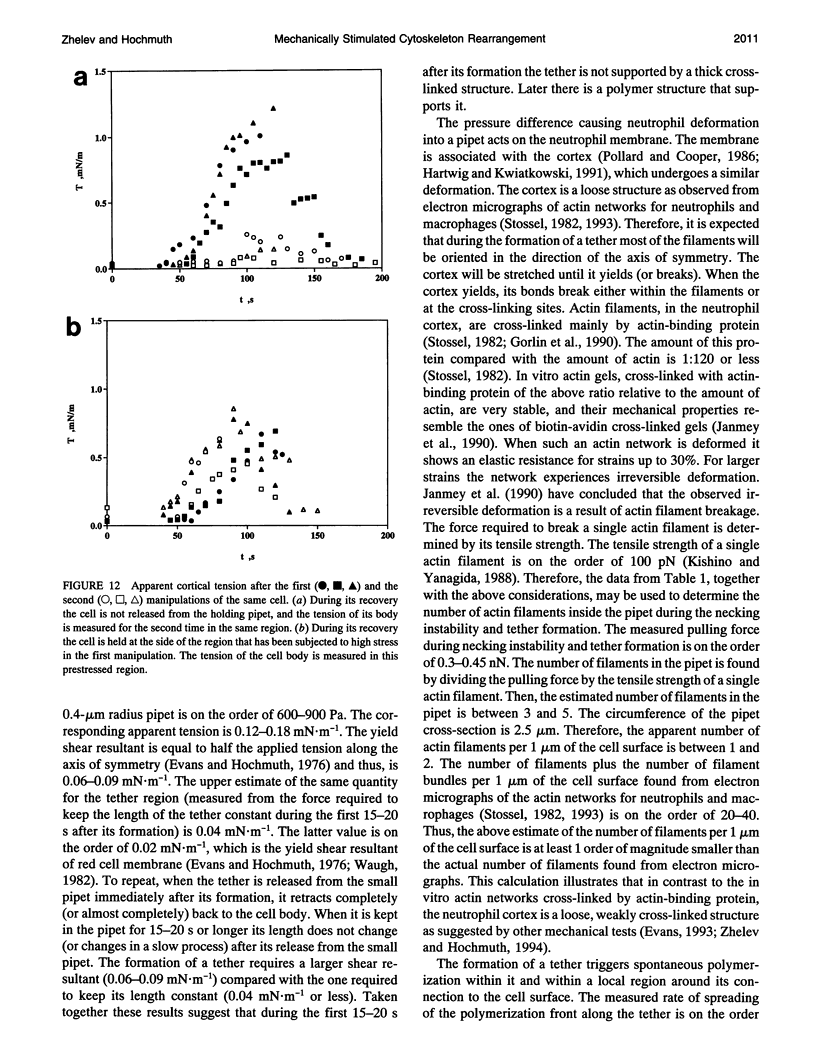
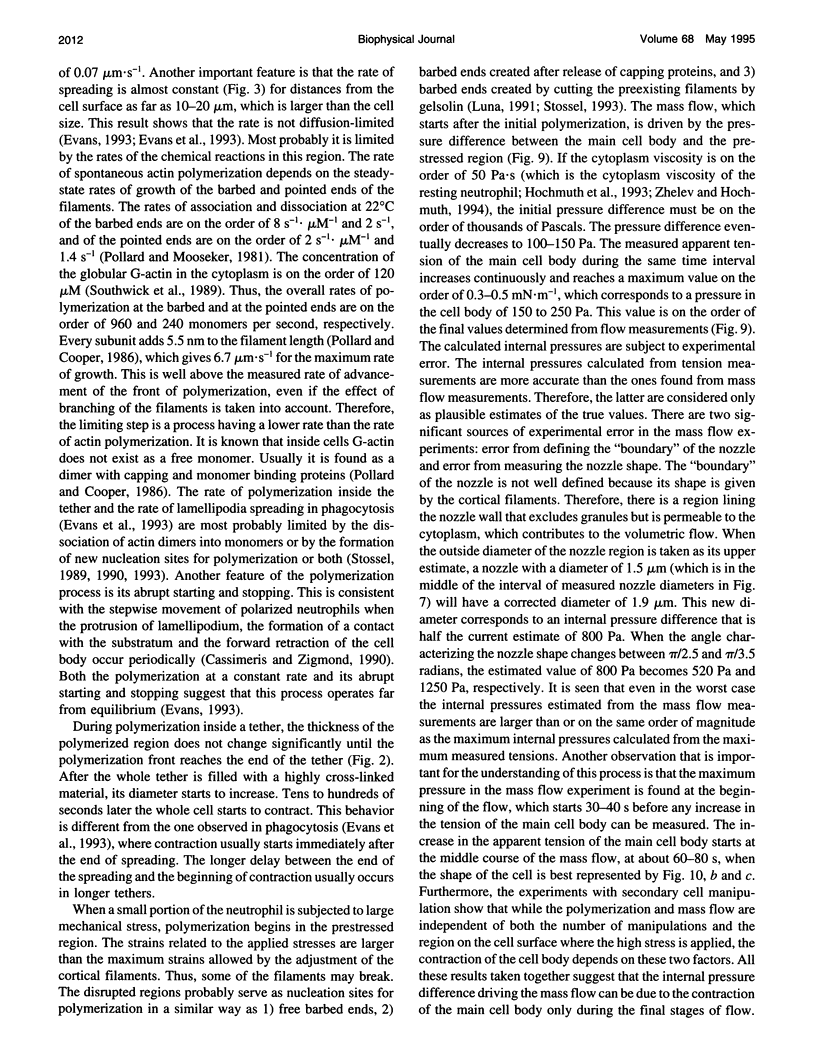
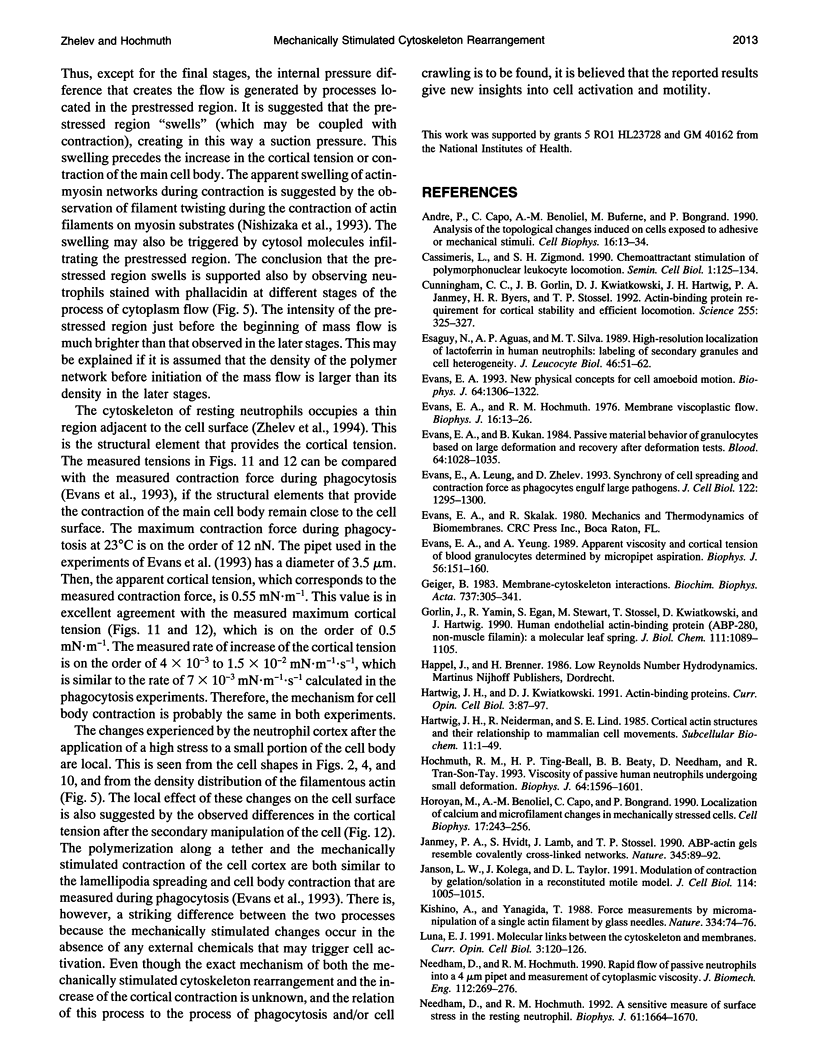
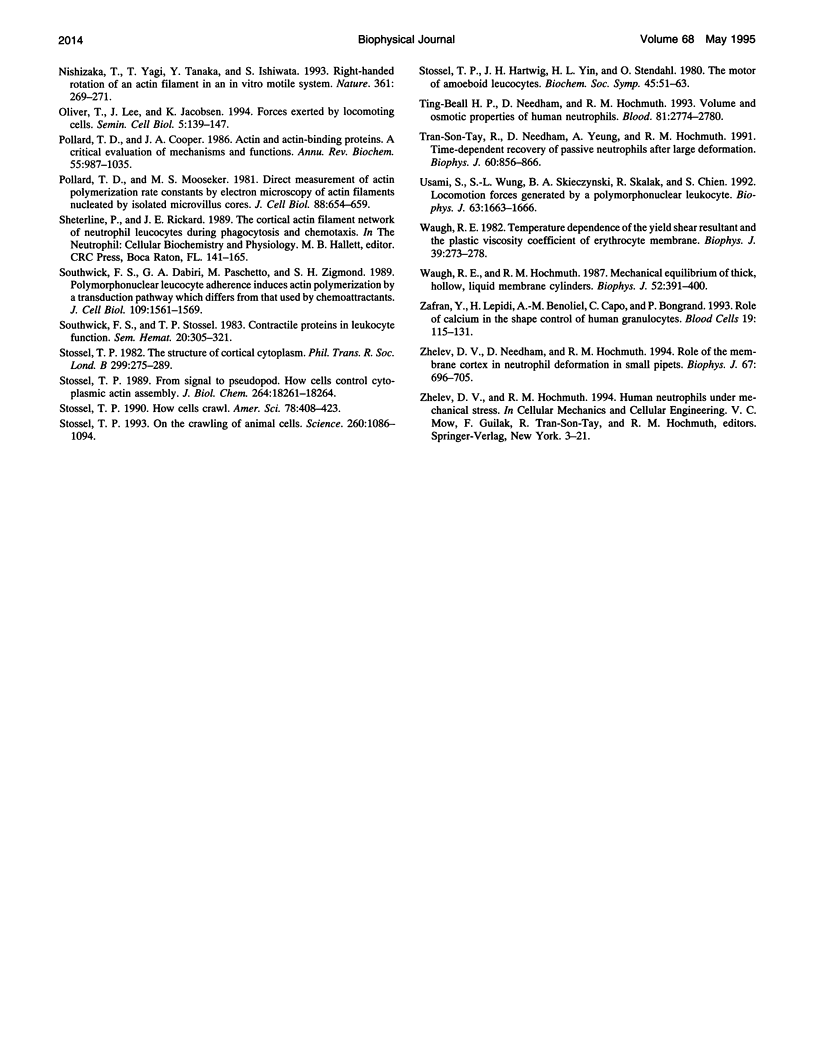
Images in this article
Selected References
These references are in PubMed. This may not be the complete list of references from this article.
- Andre P., Capo C., Benoliel A. M., Buferne M., Bongrand P. Analysis of the topological changes induced on cells exposed to adhesive or mechanical stimuli. Cell Biophys. 1990 Jan-Apr;16(1-2):13–34. doi: 10.1007/BF02989690. [DOI] [PubMed] [Google Scholar]
- Cassimeris L., Zigmond S. H. Chemoattractant stimulation of polymorphonuclear leucocyte locomotion. Semin Cell Biol. 1990 Apr;1(2):125–134. [PubMed] [Google Scholar]
- Cunningham C. C., Gorlin J. B., Kwiatkowski D. J., Hartwig J. H., Janmey P. A., Byers H. R., Stossel T. P. Actin-binding protein requirement for cortical stability and efficient locomotion. Science. 1992 Jan 17;255(5042):325–327. doi: 10.1126/science.1549777. [DOI] [PubMed] [Google Scholar]
- Esaguy N., Aguas A. P., Silva M. T. High-resolution localization of lactoferrin in human neutrophils: labeling of secondary granules and cell heterogeneity. J Leukoc Biol. 1989 Jul;46(1):51–62. doi: 10.1002/jlb.46.1.51. [DOI] [PubMed] [Google Scholar]
- Evans E. A., Hochmuth R. M. Membrane viscoplastic flow. Biophys J. 1976 Jan;16(1):13–26. doi: 10.1016/S0006-3495(76)85659-7. [DOI] [PMC free article] [PubMed] [Google Scholar]
- Evans E., Kukan B. Passive material behavior of granulocytes based on large deformation and recovery after deformation tests. Blood. 1984 Nov;64(5):1028–1035. [PubMed] [Google Scholar]
- Evans E., Leung A., Zhelev D. Synchrony of cell spreading and contraction force as phagocytes engulf large pathogens. J Cell Biol. 1993 Sep;122(6):1295–1300. doi: 10.1083/jcb.122.6.1295. [DOI] [PMC free article] [PubMed] [Google Scholar]
- Evans E. New physical concepts for cell amoeboid motion. Biophys J. 1993 Apr;64(4):1306–1322. doi: 10.1016/S0006-3495(93)81497-8. [DOI] [PMC free article] [PubMed] [Google Scholar]
- Evans E., Yeung A. Apparent viscosity and cortical tension of blood granulocytes determined by micropipet aspiration. Biophys J. 1989 Jul;56(1):151–160. doi: 10.1016/S0006-3495(89)82660-8. [DOI] [PMC free article] [PubMed] [Google Scholar]
- Geiger B. Membrane-cytoskeleton interaction. Biochim Biophys Acta. 1983 Aug 11;737(3-4):305–341. doi: 10.1016/0304-4157(83)90005-9. [DOI] [PubMed] [Google Scholar]
- Gorlin J. B., Yamin R., Egan S., Stewart M., Stossel T. P., Kwiatkowski D. J., Hartwig J. H. Human endothelial actin-binding protein (ABP-280, nonmuscle filamin): a molecular leaf spring. J Cell Biol. 1990 Sep;111(3):1089–1105. doi: 10.1083/jcb.111.3.1089. [DOI] [PMC free article] [PubMed] [Google Scholar]
- Hartwig J. H., Kwiatkowski D. J. Actin-binding proteins. Curr Opin Cell Biol. 1991 Feb;3(1):87–97. doi: 10.1016/0955-0674(91)90170-4. [DOI] [PubMed] [Google Scholar]
- Hartwig J. H., Niederman R., Lind S. E. Cortical actin structures and their relationship to mammalian cell movements. Subcell Biochem. 1985;11:1–49. doi: 10.1007/978-1-4899-1698-3_1. [DOI] [PubMed] [Google Scholar]
- Hochmuth R. M., Ting-Beall H. P., Beaty B. B., Needham D., Tran-Son-Tay R. Viscosity of passive human neutrophils undergoing small deformations. Biophys J. 1993 May;64(5):1596–1601. doi: 10.1016/S0006-3495(93)81530-3. [DOI] [PMC free article] [PubMed] [Google Scholar]
- Horoyan M., Benoliel A. M., Capo C., Bongrand P. Localization of calcium and microfilament changes in mechanically stressed cells. Cell Biophys. 1990 Dec;17(3):243–256. doi: 10.1007/BF02990720. [DOI] [PMC free article] [PubMed] [Google Scholar]
- Janmey P. A., Hvidt S., Lamb J., Stossel T. P. Resemblance of actin-binding protein/actin gels to covalently crosslinked networks. Nature. 1990 May 3;345(6270):89–92. doi: 10.1038/345089a0. [DOI] [PubMed] [Google Scholar]
- Janson L. W., Kolega J., Taylor D. L. Modulation of contraction by gelation/solation in a reconstituted motile model. J Cell Biol. 1991 Sep;114(5):1005–1015. doi: 10.1083/jcb.114.5.1005. [DOI] [PMC free article] [PubMed] [Google Scholar]
- Kishino A., Yanagida T. Force measurements by micromanipulation of a single actin filament by glass needles. Nature. 1988 Jul 7;334(6177):74–76. doi: 10.1038/334074a0. [DOI] [PubMed] [Google Scholar]
- Luna E. J. Molecular links between the cytoskeleton and membranes. Curr Opin Cell Biol. 1991 Feb;3(1):120–126. doi: 10.1016/0955-0674(91)90174-w. [DOI] [PubMed] [Google Scholar]
- Needham D., Hochmuth R. M. A sensitive measure of surface stress in the resting neutrophil. Biophys J. 1992 Jun;61(6):1664–1670. doi: 10.1016/S0006-3495(92)81970-7. [DOI] [PMC free article] [PubMed] [Google Scholar]
- Needham D., Hochmuth R. M. Rapid flow of passive neutrophils into a 4 microns pipet and measurement of cytoplasmic viscosity. J Biomech Eng. 1990 Aug;112(3):269–276. doi: 10.1115/1.2891184. [DOI] [PubMed] [Google Scholar]
- Nishizaka T., Yagi T., Tanaka Y., Ishiwata S. Right-handed rotation of an actin filament in an in vitro motile system. Nature. 1993 Jan 21;361(6409):269–271. doi: 10.1038/361269a0. [DOI] [PubMed] [Google Scholar]
- Oliver T., Lee J., Jacobson K. Forces exerted by locomoting cells. Semin Cell Biol. 1994 Jun;5(3):139–147. doi: 10.1006/scel.1994.1018. [DOI] [PubMed] [Google Scholar]
- Pollard T. D., Cooper J. A. Actin and actin-binding proteins. A critical evaluation of mechanisms and functions. Annu Rev Biochem. 1986;55:987–1035. doi: 10.1146/annurev.bi.55.070186.005011. [DOI] [PubMed] [Google Scholar]
- Pollard T. D., Mooseker M. S. Direct measurement of actin polymerization rate constants by electron microscopy of actin filaments nucleated by isolated microvillus cores. J Cell Biol. 1981 Mar;88(3):654–659. doi: 10.1083/jcb.88.3.654. [DOI] [PMC free article] [PubMed] [Google Scholar]
- Southwick F. S., Dabiri G. A., Paschetto M., Zigmond S. H. Polymorphonuclear leukocyte adherence induces actin polymerization by a transduction pathway which differs from that used by chemoattractants. J Cell Biol. 1989 Oct;109(4 Pt 1):1561–1569. doi: 10.1083/jcb.109.4.1561. [DOI] [PMC free article] [PubMed] [Google Scholar]
- Southwick F. S., Stossel T. P. Contractile proteins in leukocyte function. Semin Hematol. 1983 Oct;20(4):305–321. [PubMed] [Google Scholar]
- Stossel T. P. From signal to pseudopod. How cells control cytoplasmic actin assembly. J Biol Chem. 1989 Nov 5;264(31):18261–18264. [PubMed] [Google Scholar]
- Stossel T. P., Hartwig J. H., Yin H. L., Stendahl O. The motor of amoeboid leucocytes. Biochem Soc Symp. 1980;45:51–63. [PubMed] [Google Scholar]
- Stossel T. P. On the crawling of animal cells. Science. 1993 May 21;260(5111):1086–1094. doi: 10.1126/science.8493552. [DOI] [PubMed] [Google Scholar]
- Stossel T. P. The structure of cortical cytoplasm. Philos Trans R Soc Lond B Biol Sci. 1982 Nov 4;299(1095):275–289. doi: 10.1098/rstb.1982.0132. [DOI] [PubMed] [Google Scholar]
- Ting-Beall H. P., Needham D., Hochmuth R. M. Volume and osmotic properties of human neutrophils. Blood. 1993 May 15;81(10):2774–2780. [PubMed] [Google Scholar]
- Tran-Son-Tay R., Needham D., Yeung A., Hochmuth R. M. Time-dependent recovery of passive neutrophils after large deformation. Biophys J. 1991 Oct;60(4):856–866. doi: 10.1016/S0006-3495(91)82119-1. [DOI] [PMC free article] [PubMed] [Google Scholar]
- Usami S., Wung S. L., Skierczynski B. A., Skalak R., Chien S. Locomotion forces generated by a polymorphonuclear leukocyte. Biophys J. 1992 Dec;63(6):1663–1666. doi: 10.1016/S0006-3495(92)81745-9. [DOI] [PMC free article] [PubMed] [Google Scholar]
- Waugh R. E., Hochmuth R. M. Mechanical equilibrium of thick, hollow, liquid membrane cylinders. Biophys J. 1987 Sep;52(3):391–400. doi: 10.1016/S0006-3495(87)83227-7. [DOI] [PMC free article] [PubMed] [Google Scholar]
- Waugh R. E. Temperature dependence of the yield shear resultant and the plastic viscosity coefficient of erythrocyte membrane. Implications about molecular events during membrane failure. Biophys J. 1982 Sep;39(3):273–278. doi: 10.1016/S0006-3495(82)84517-7. [DOI] [PMC free article] [PubMed] [Google Scholar]
- Zaffran Y., Lepidi H., Benoliel A. M., Capo C., Bongrand P. Role of calcium in the shape control of human granulocytes. Blood Cells. 1993;19(1):115–131. [PubMed] [Google Scholar]
- Zhelev D. V., Needham D., Hochmuth R. M. Role of the membrane cortex in neutrophil deformation in small pipets. Biophys J. 1994 Aug;67(2):696–705. doi: 10.1016/S0006-3495(94)80529-6. [DOI] [PMC free article] [PubMed] [Google Scholar]