Abstract
1. The excitatory effects of acetylcholine (ACh) on an identified group of Aplysia neurones have been studied under voltage clamp in an attempt to measure the average life time. tau, of the channels opened by ACh and the elementary current, iel, flowing through these channels. The value of tau was determined both from spectral noise analysis and from current relaxations after voltage steps. Both methods lead to similar values. iel was calculated from the ratio of the variance of the ACh induced noise to the mean ACh induced current. 2. tau is increased by hyperpolarization, or by lowering the temperature. At 12 degrees C, tau = 27 msec AT -80 MV, tau = 17 msec at mV. tau is about 5 times smaller at 21 degrees C than at 12 degrees C. 3. iel increases linearly with hyperpolarization. At -80 mV, in Tris-buffered sea water, the mean value of iel was 0.8 X 10)-12) A at 12 degrees C. At 21 degrees C, this value was multiplied by 1.8. 4. The estimate of the ACh reversal potential Erev obtained by extrapolation of the relation between iel and the membrane potential V was + 30 mV. The estimate obtained from the analysis of the instantaneous current changes produced by voltage steps was + 15 mV. The difference between the two values appears to be due to the development of a K curent activated by the entry of Ca into the cell during the ACh response. This current introduces an error in opposite directions into the two estimates of Erev, which can therefore be assumed to be intermediate between + 15 and + 30 mV. An assumed value of + 20 mV yields an elementary conductance of 8 X 10(-12) omega-1 at 12 degrees C in Tris-buffered sea water. 5. The total ACh induced current measured in steady-state conditions increases more with hyperpolarization than does iel. The difference can be entirely accounted for by the fact that hyperpolarization increases tau. 6. When carbachol or tetramethylammonium is applied instead of ACh, the value of iel is identical to that found with ACh, but tau is slightly shorter (about 75%). 7. Inward ACh induced currents can still be observed in solutions where all Na has been replaced by Cs, Mg, or Ca. 8. iel increases when Na is replaced by Cs; it decreases when Na is replaced by Mg or Ca. In all Na-free solutions, tau is larger than in Na sea water: the lengthening of tau is largest for Ca sea water, smallest for Cs sea water. An interpretation of these changes of gamma is proposed. This interpretation may also account for the voltage sensitivity of gamma in normal sea water. 9. Partial replacement of NaCl by TrisCl strikingly reduces the ACh induced current. gamma is not modified by Tris substitution, and the reduction of the total current is entirely accounted for by a steep decrease of iel. Tris does not seem to affect the pore opening and closing processes, but to block the ACh controlled channel.
Full text
PDF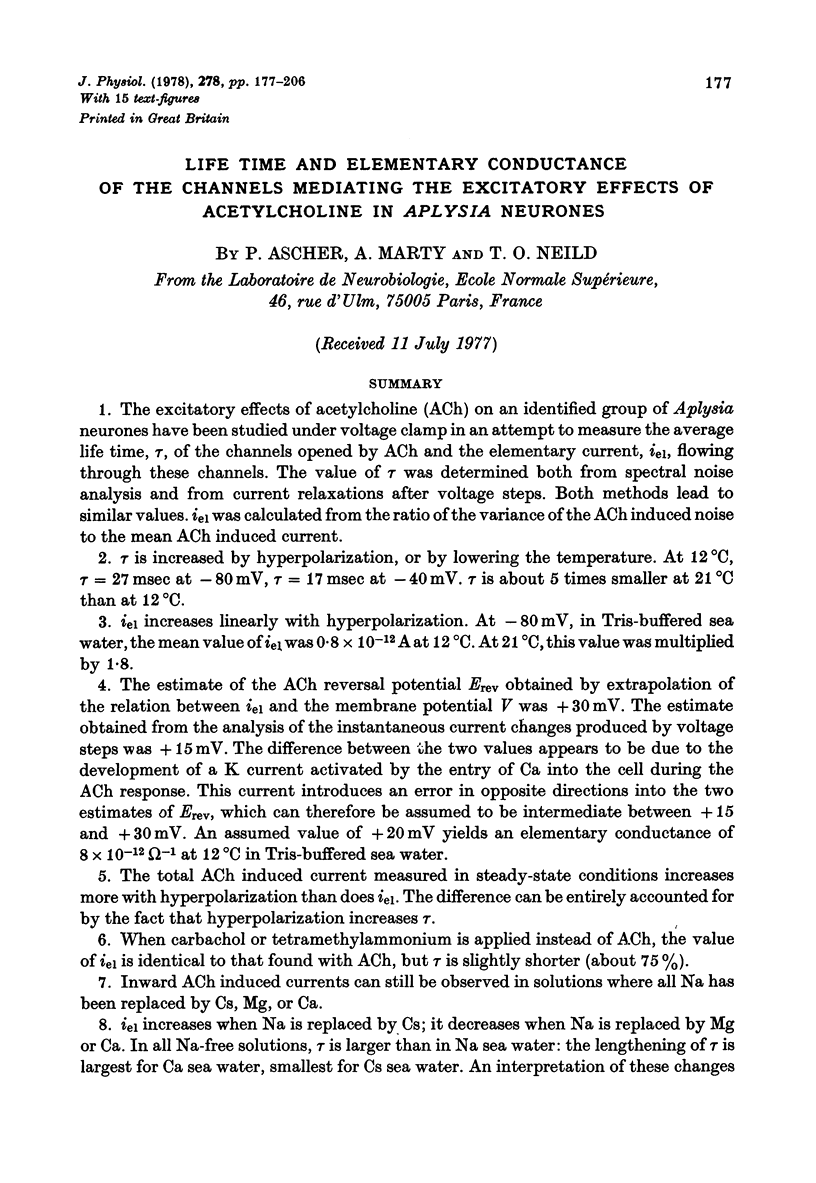
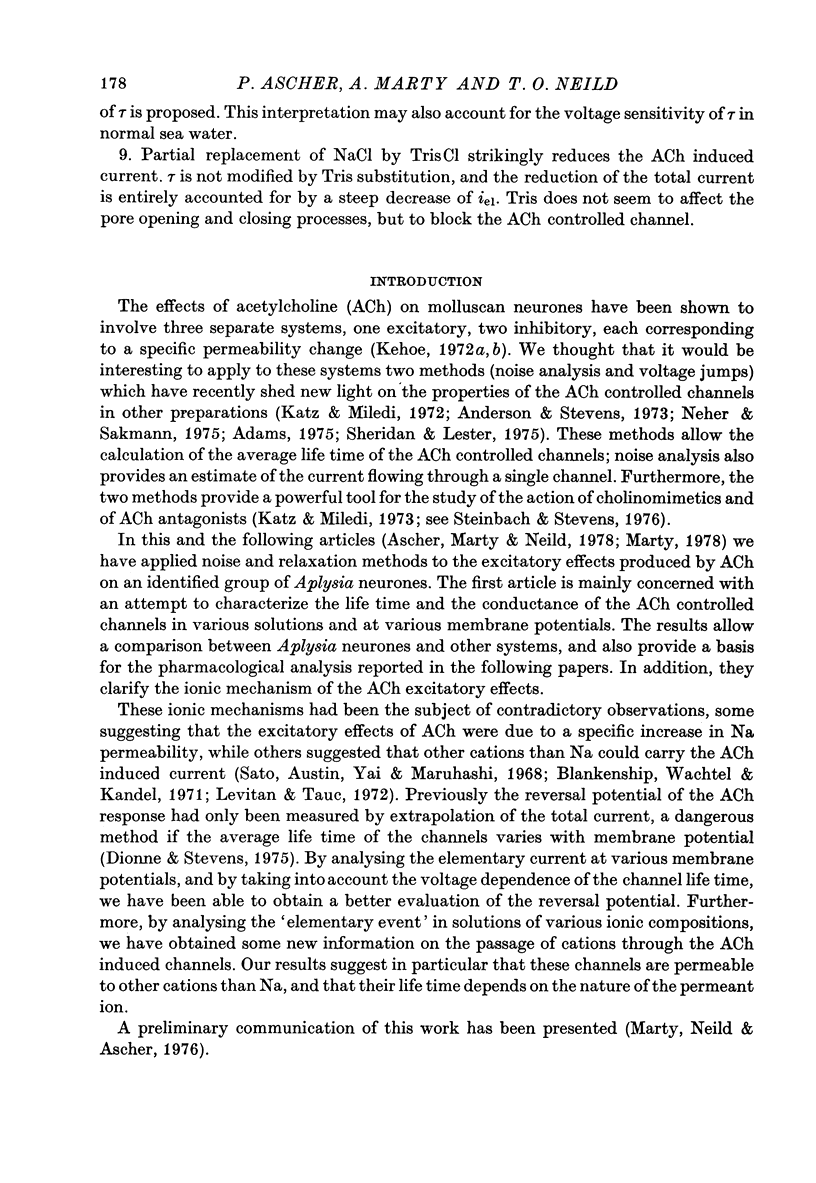
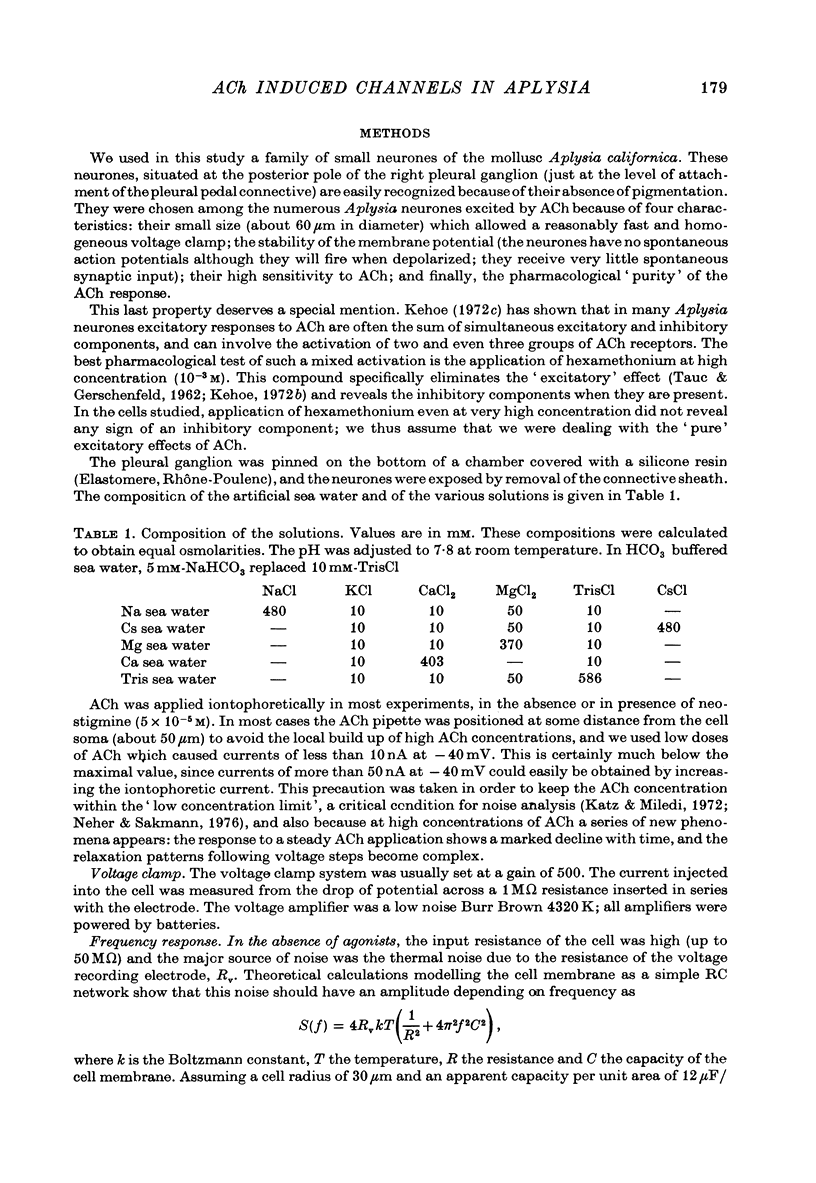
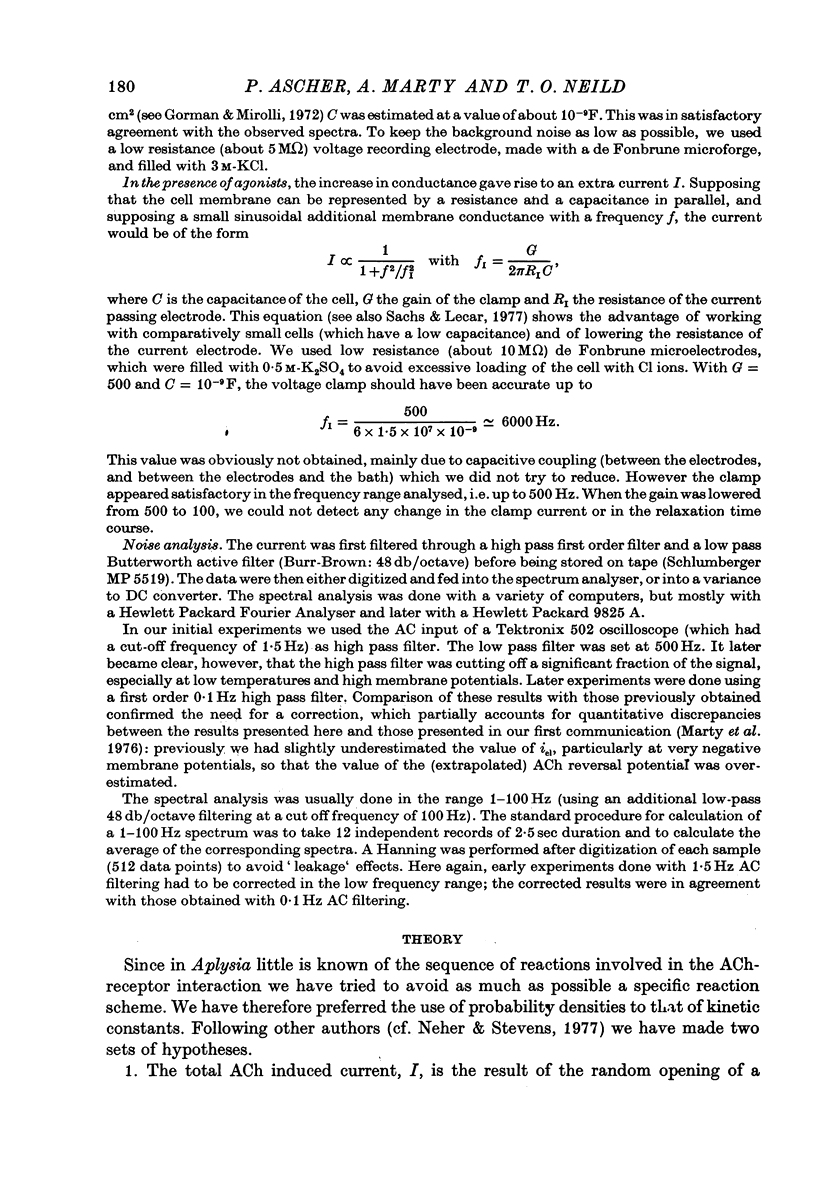
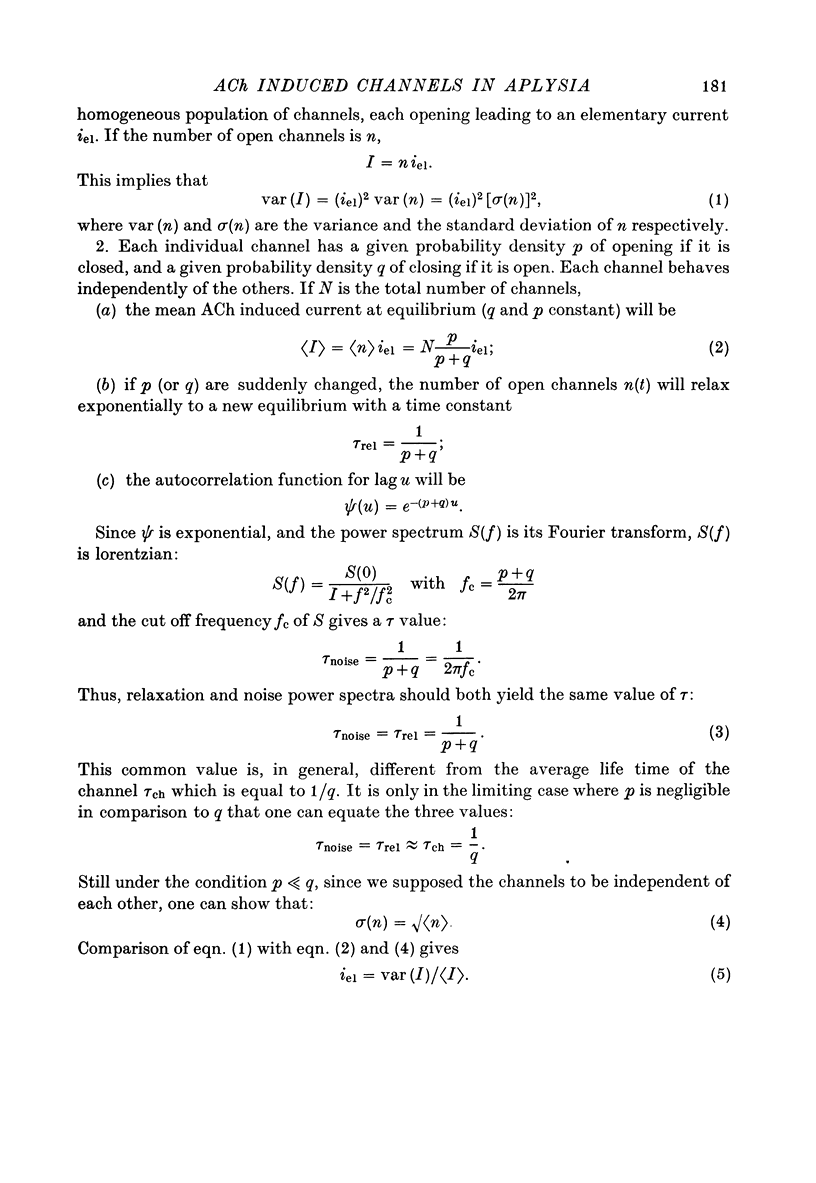
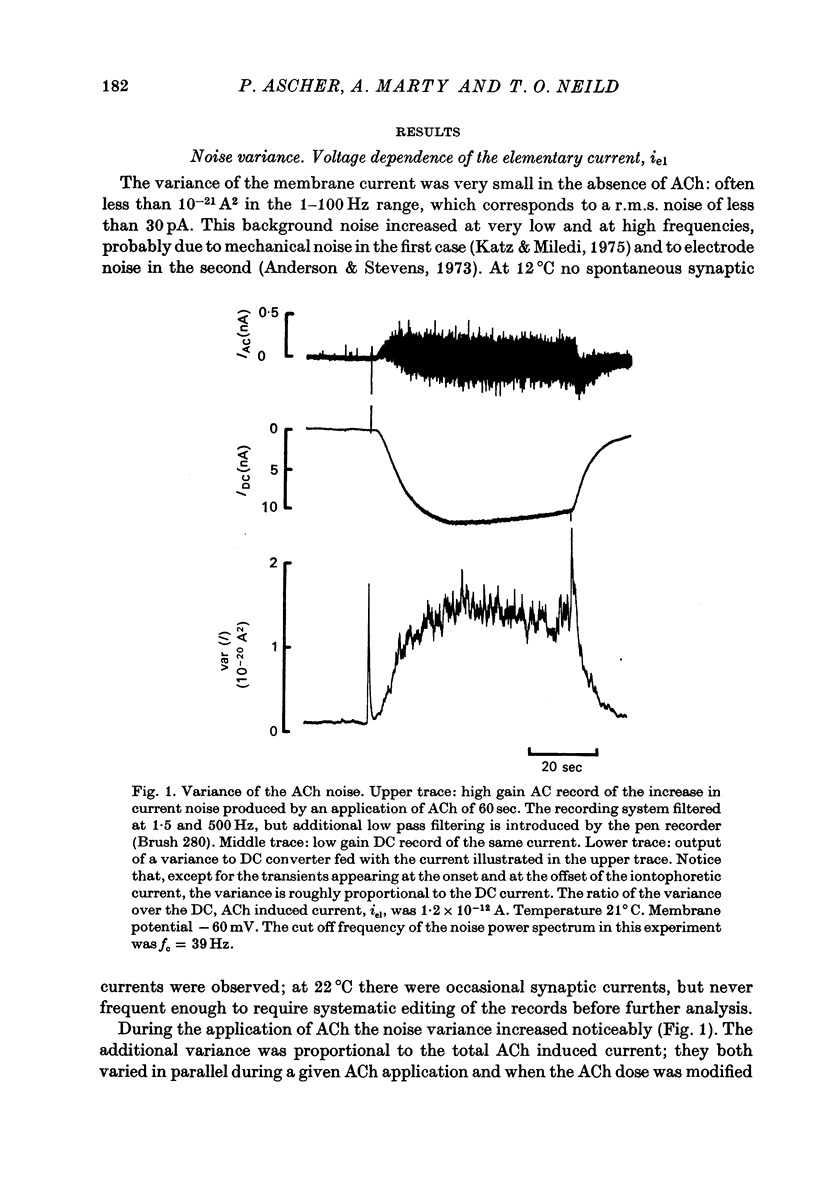
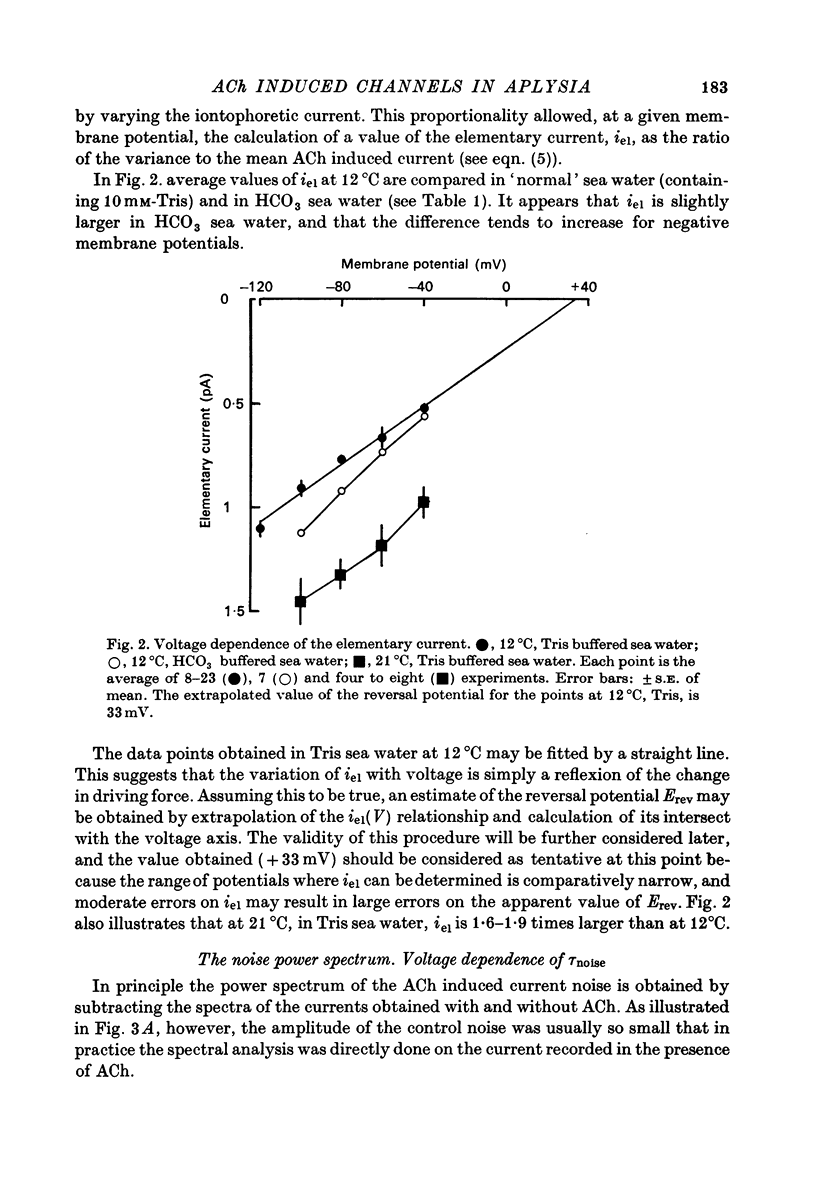
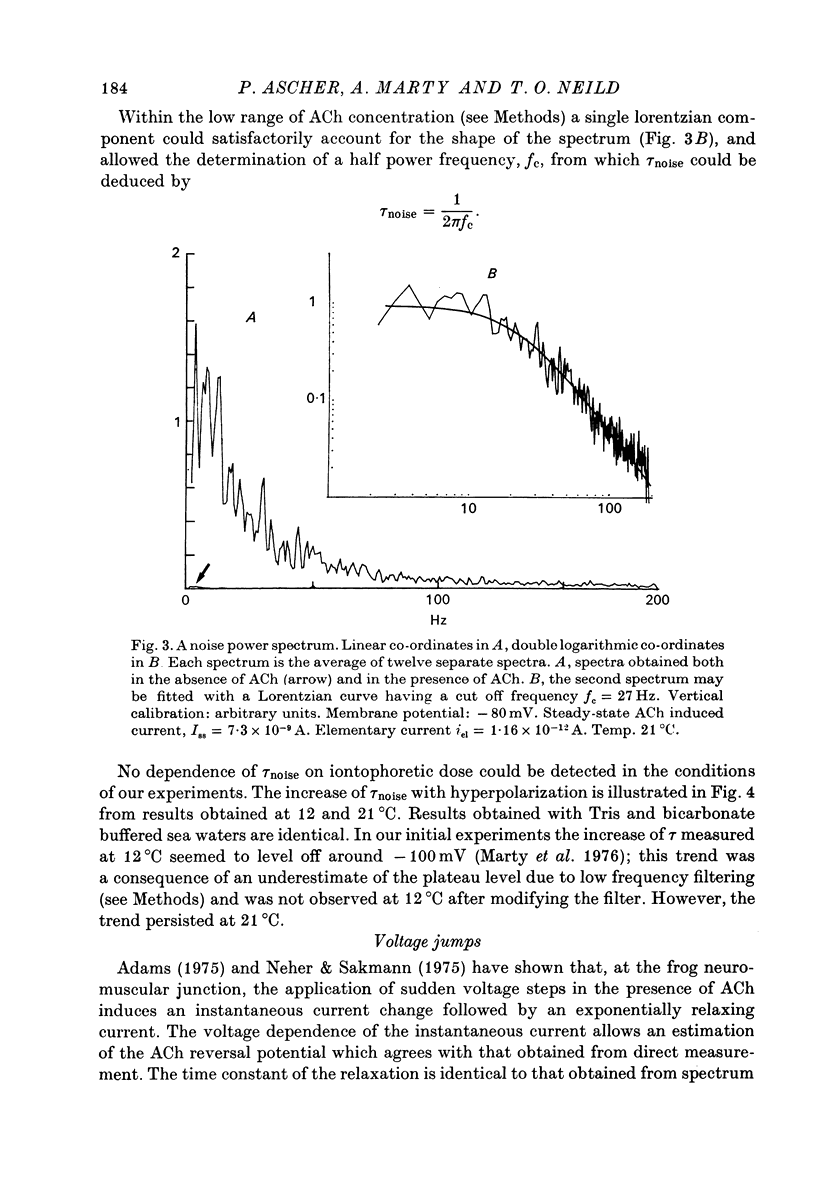
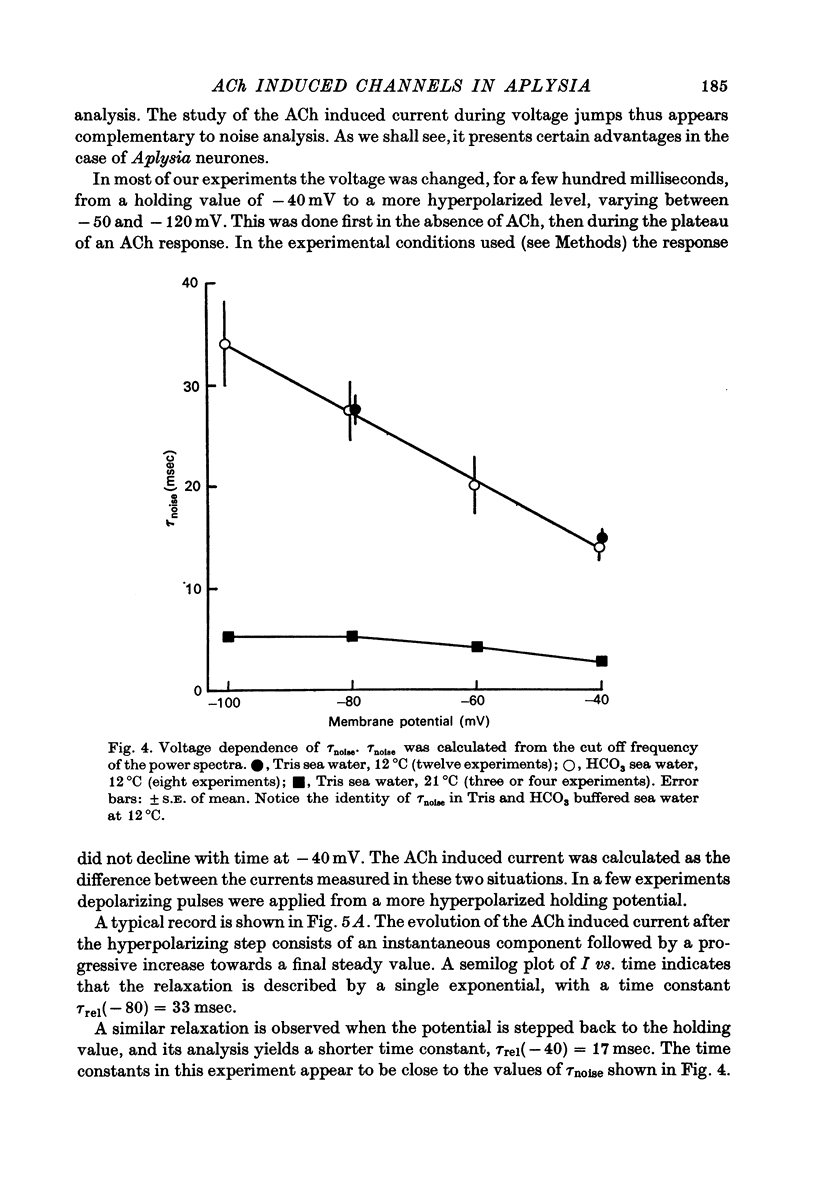
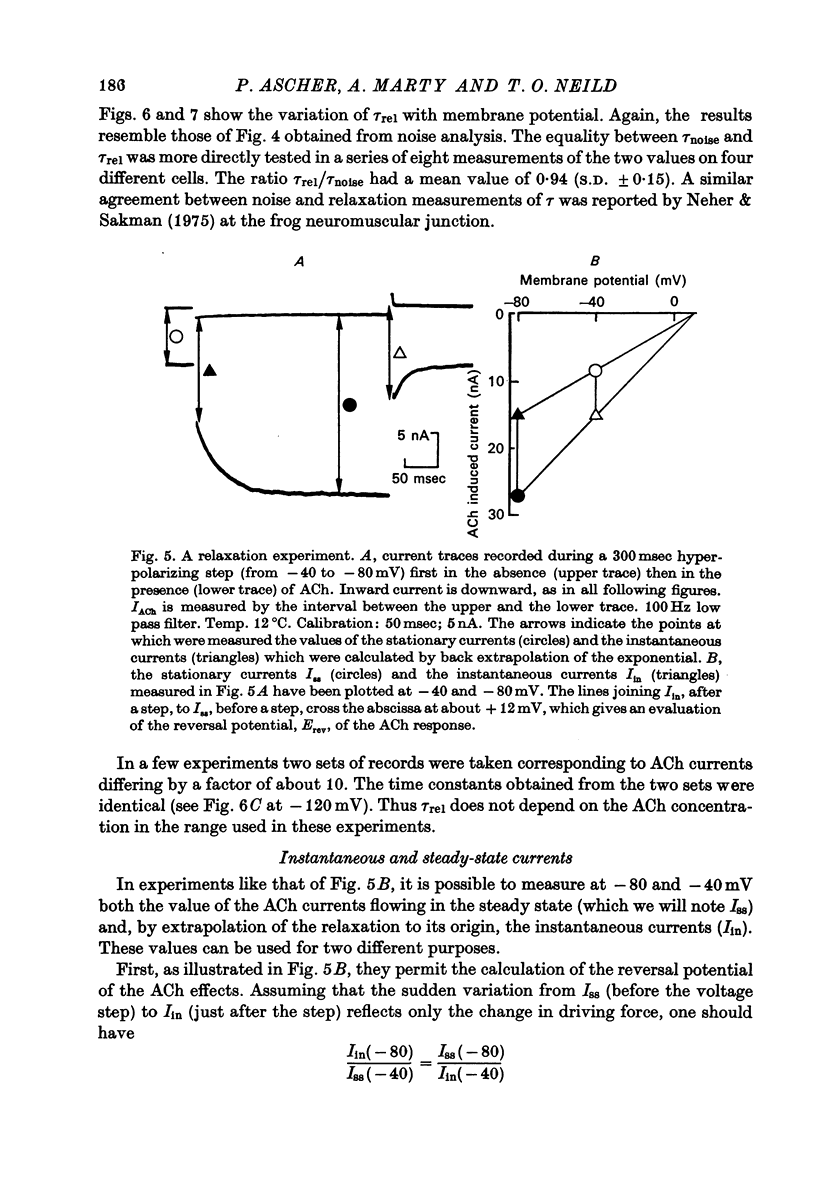
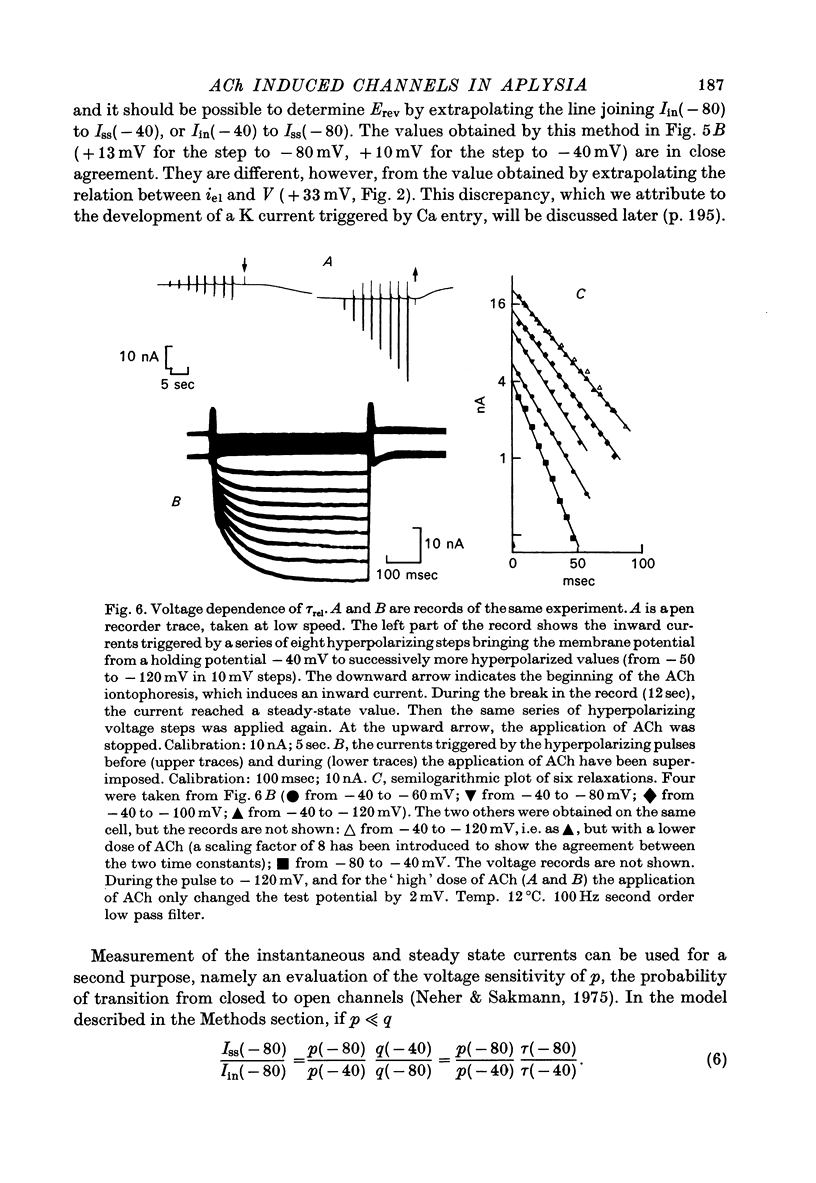
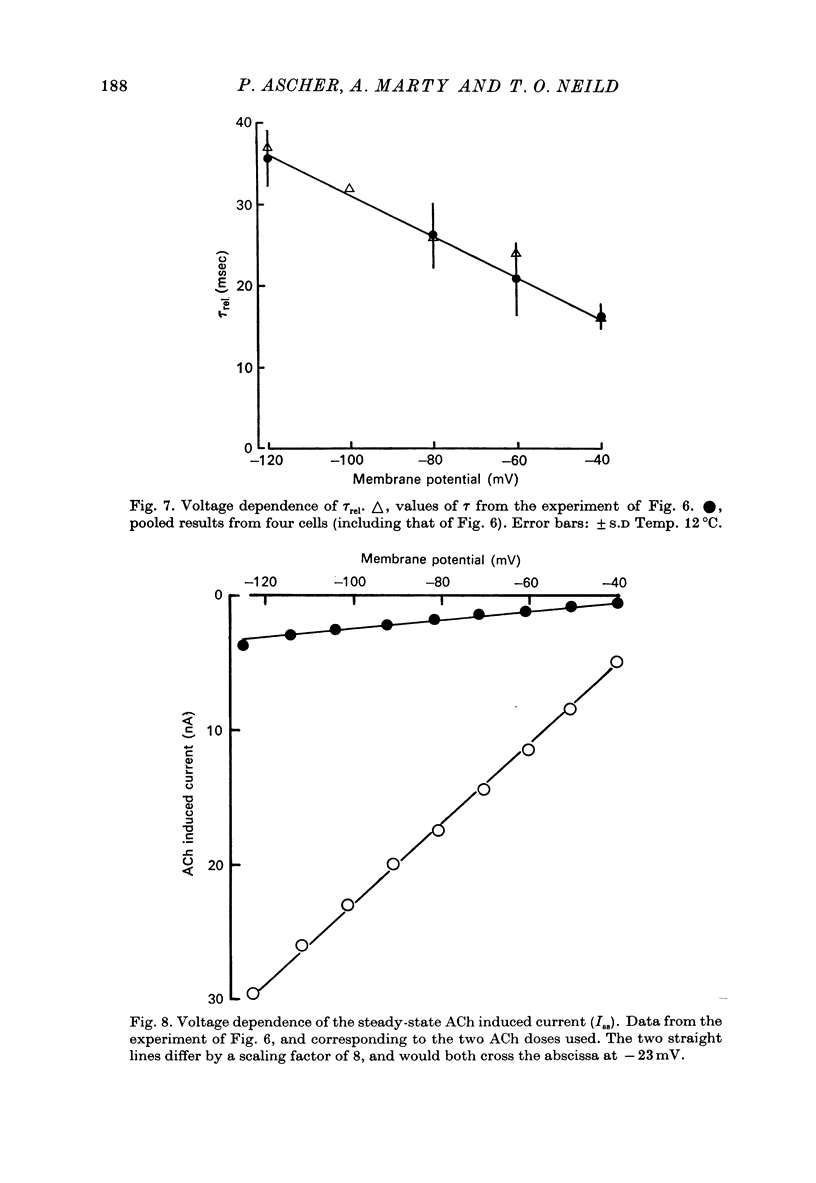
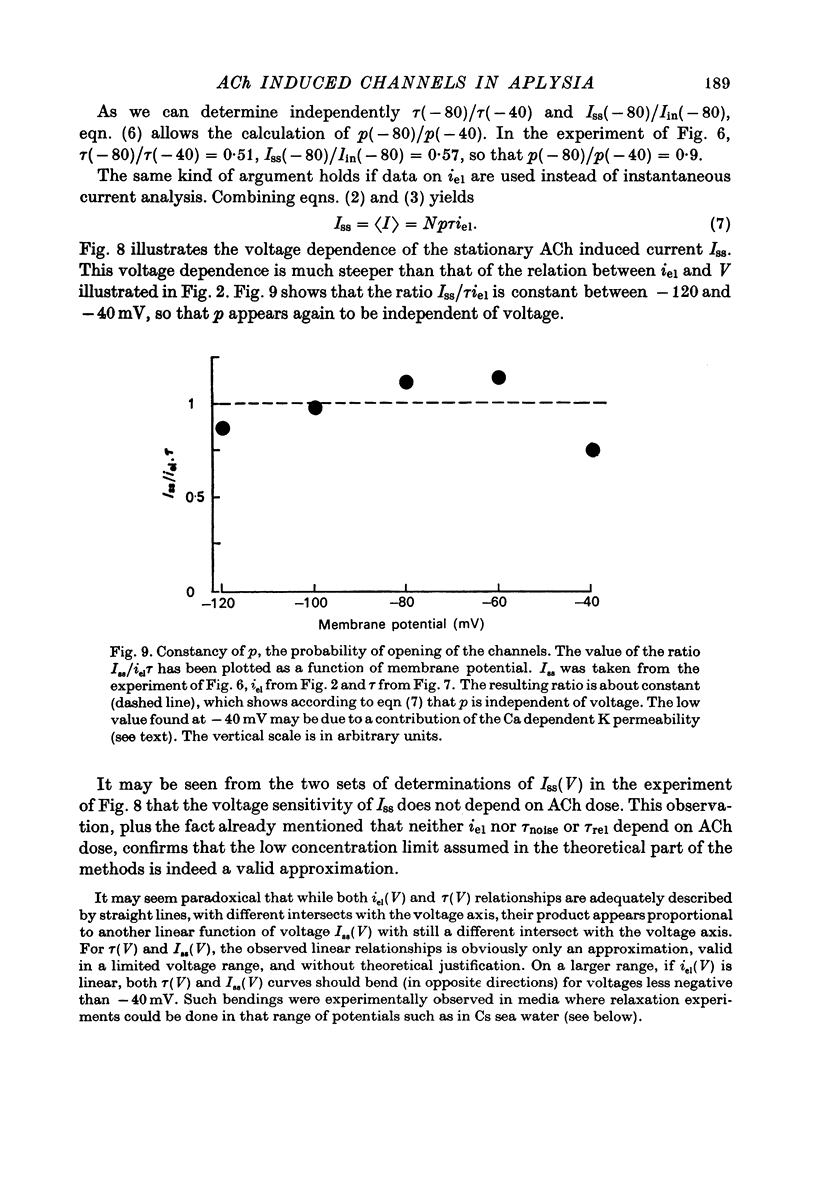
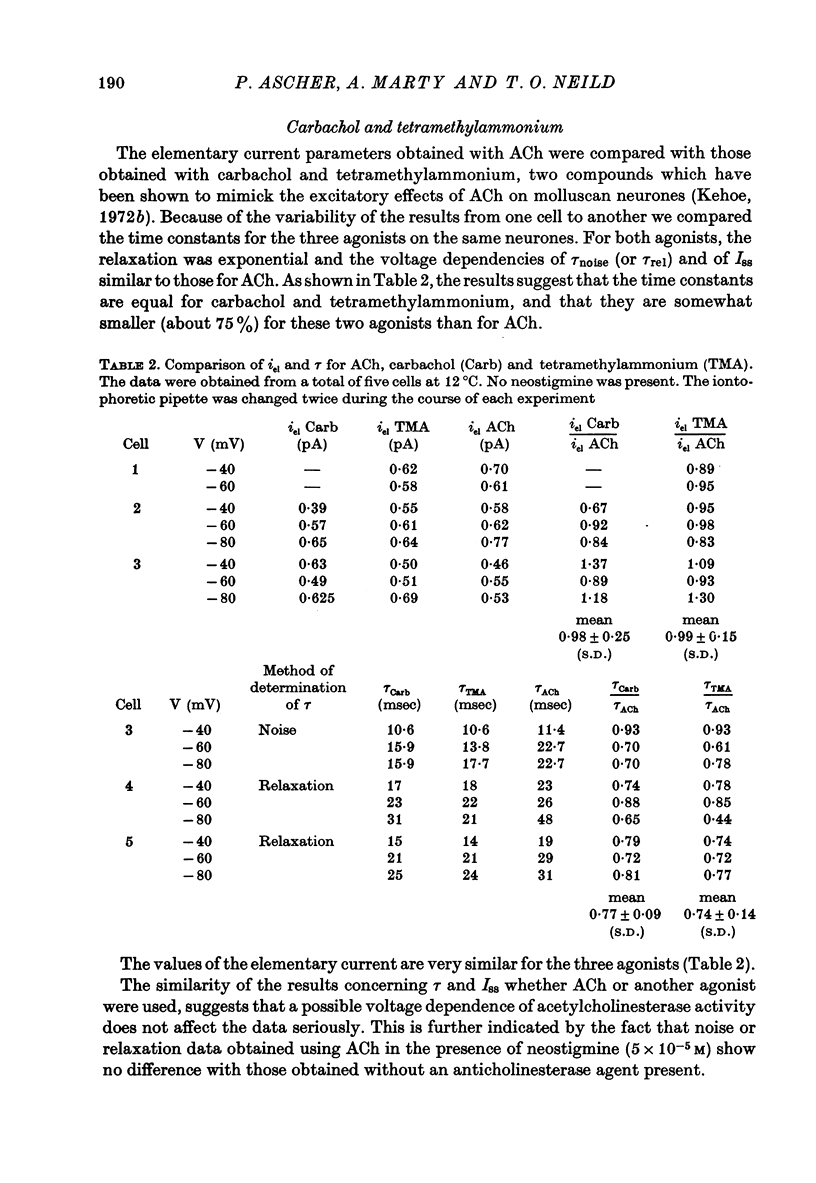
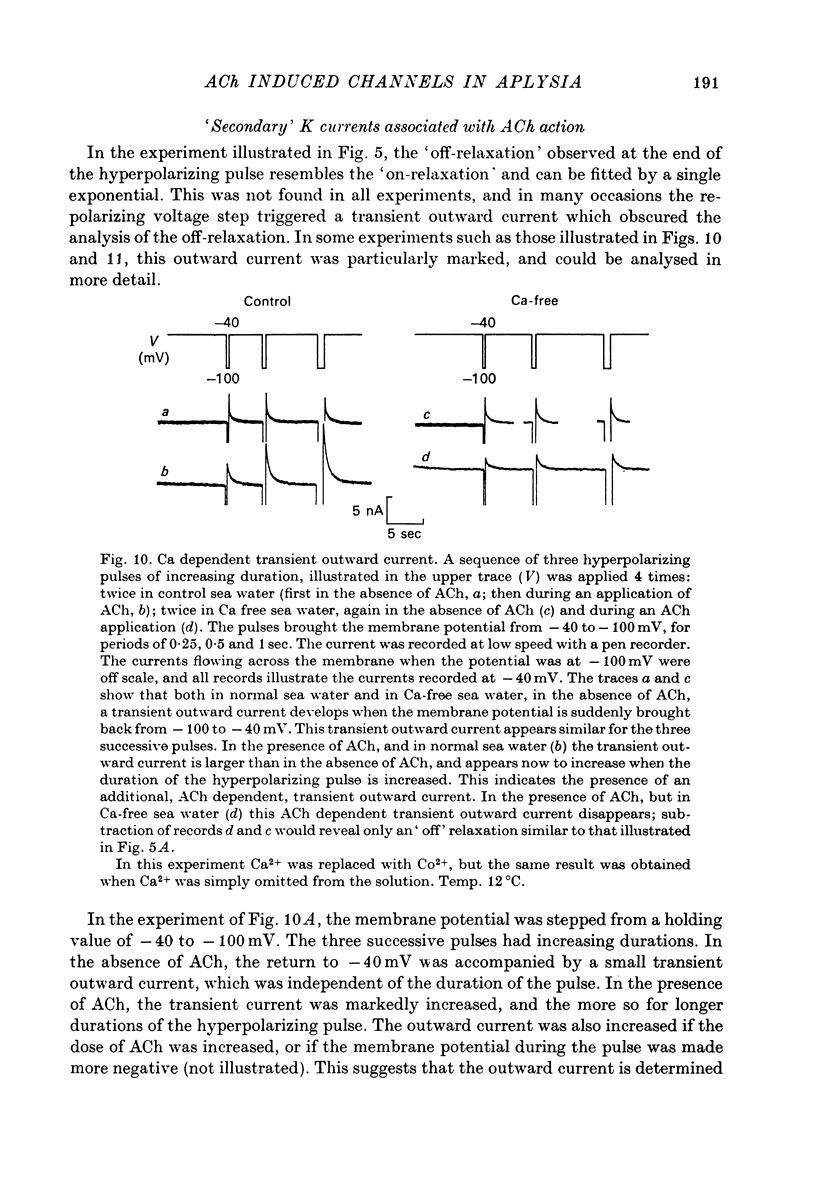
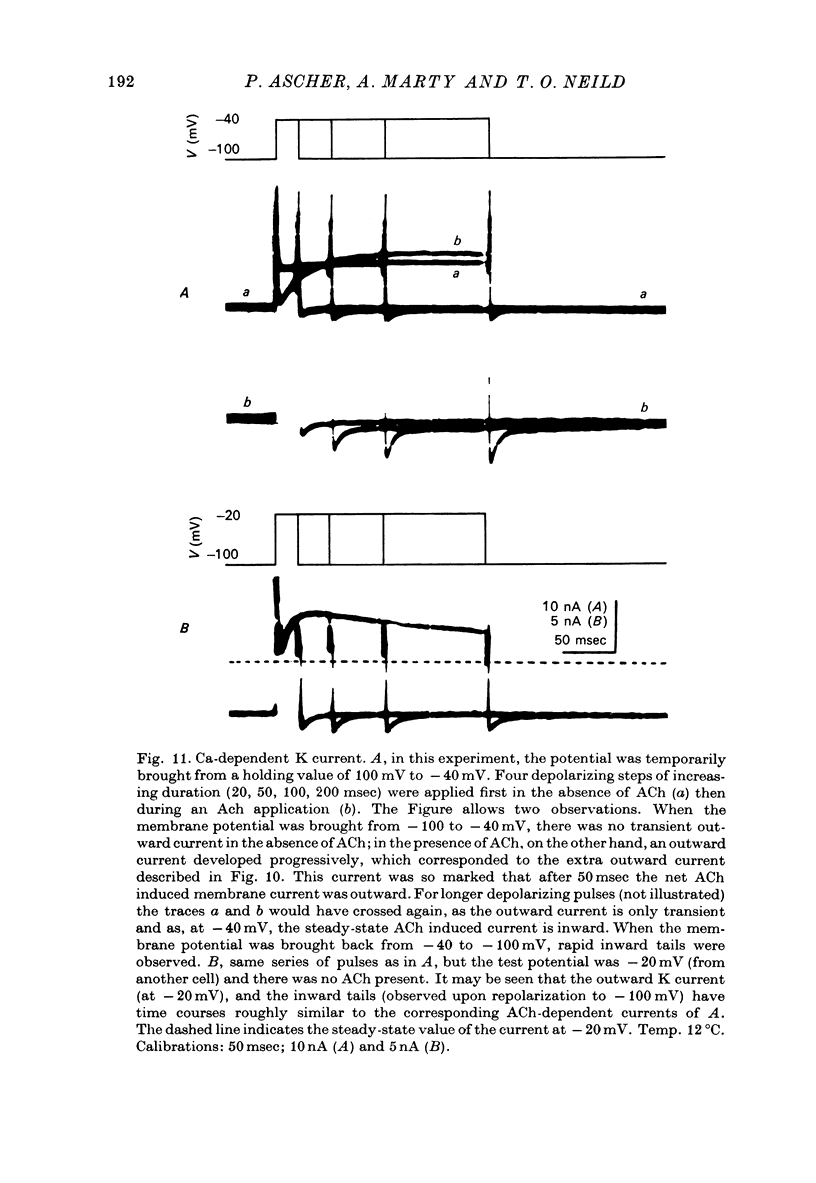
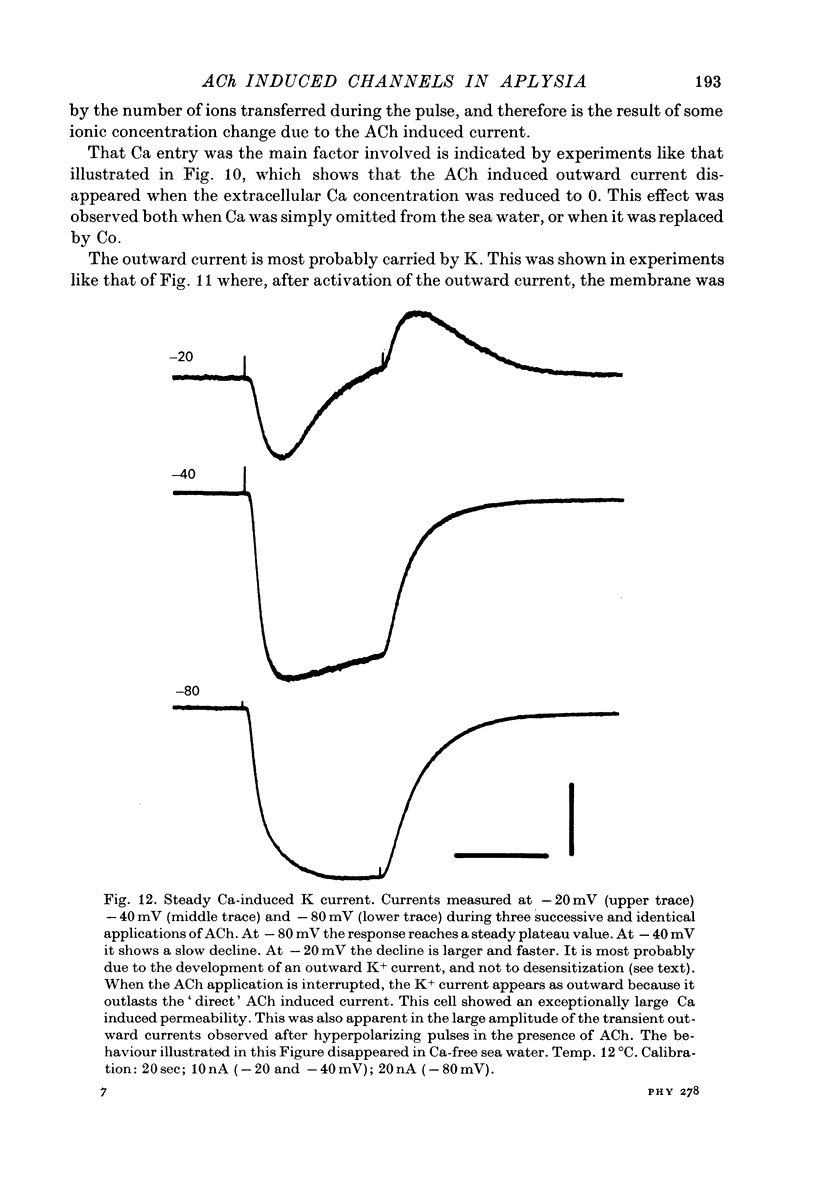
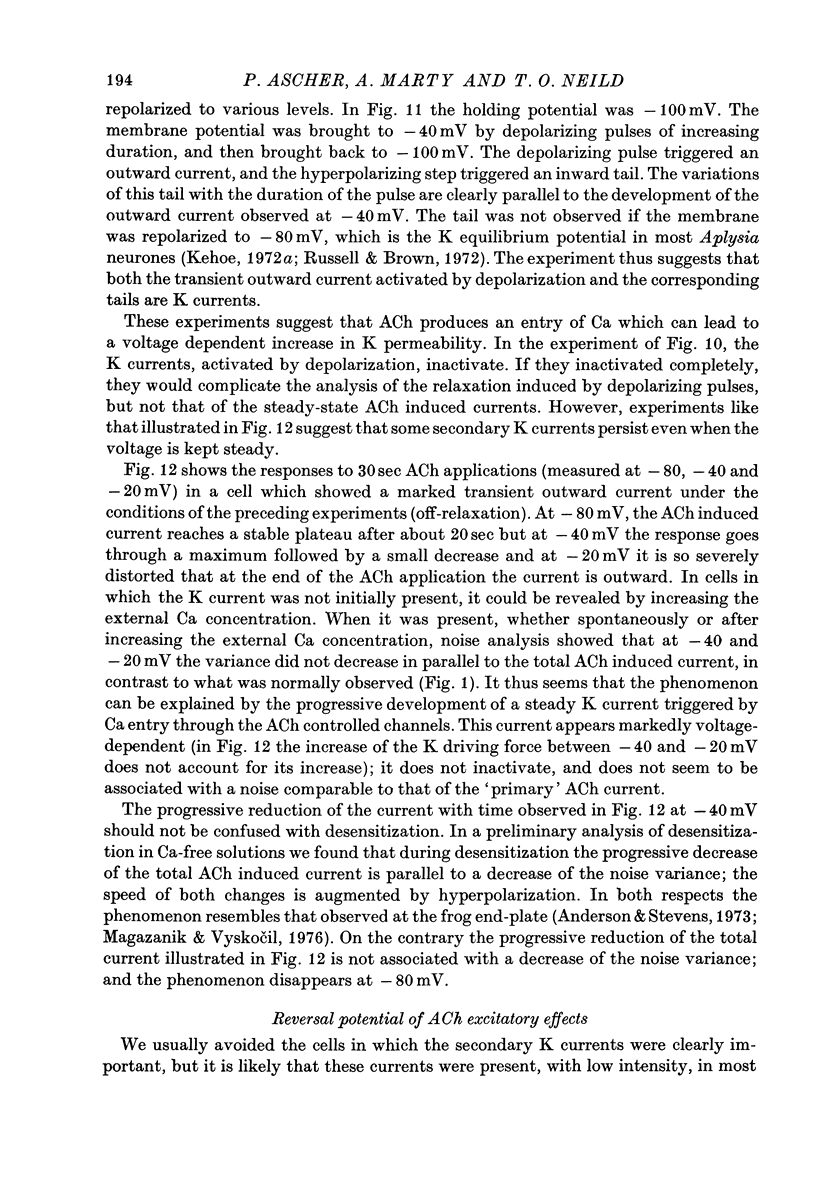
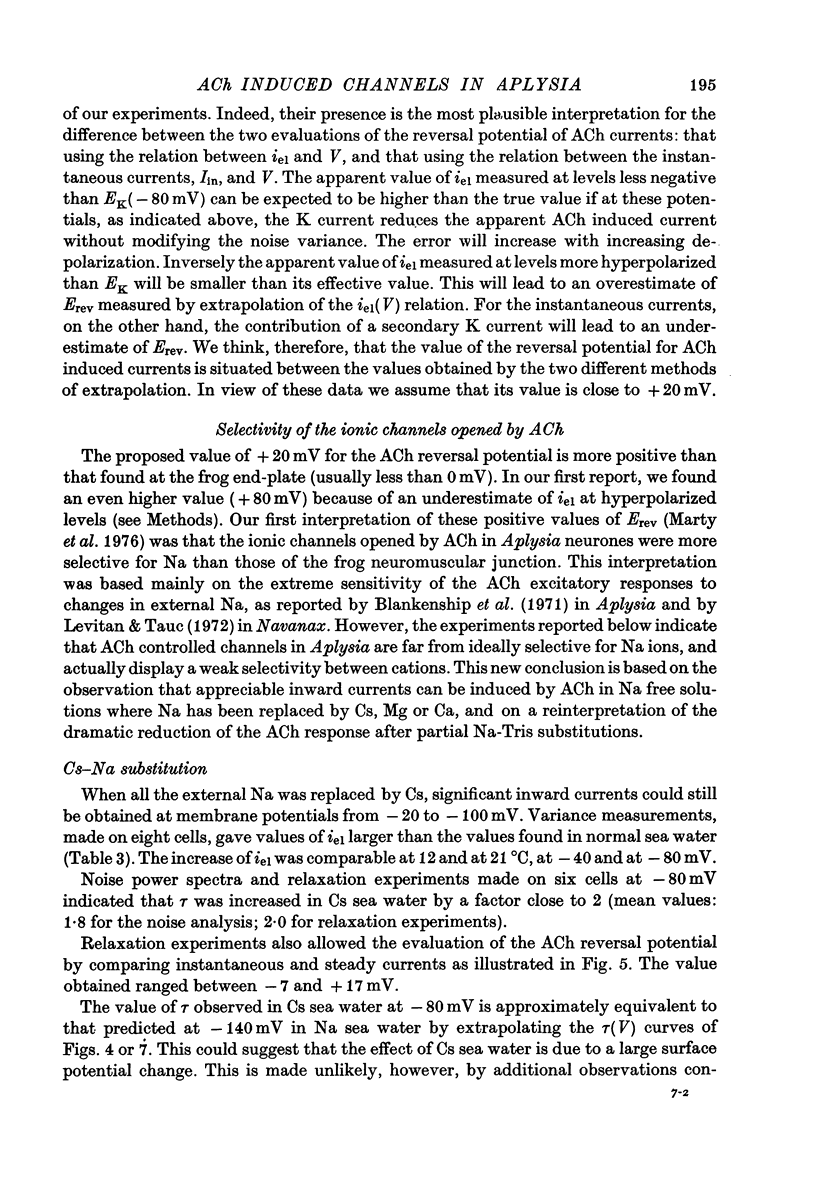
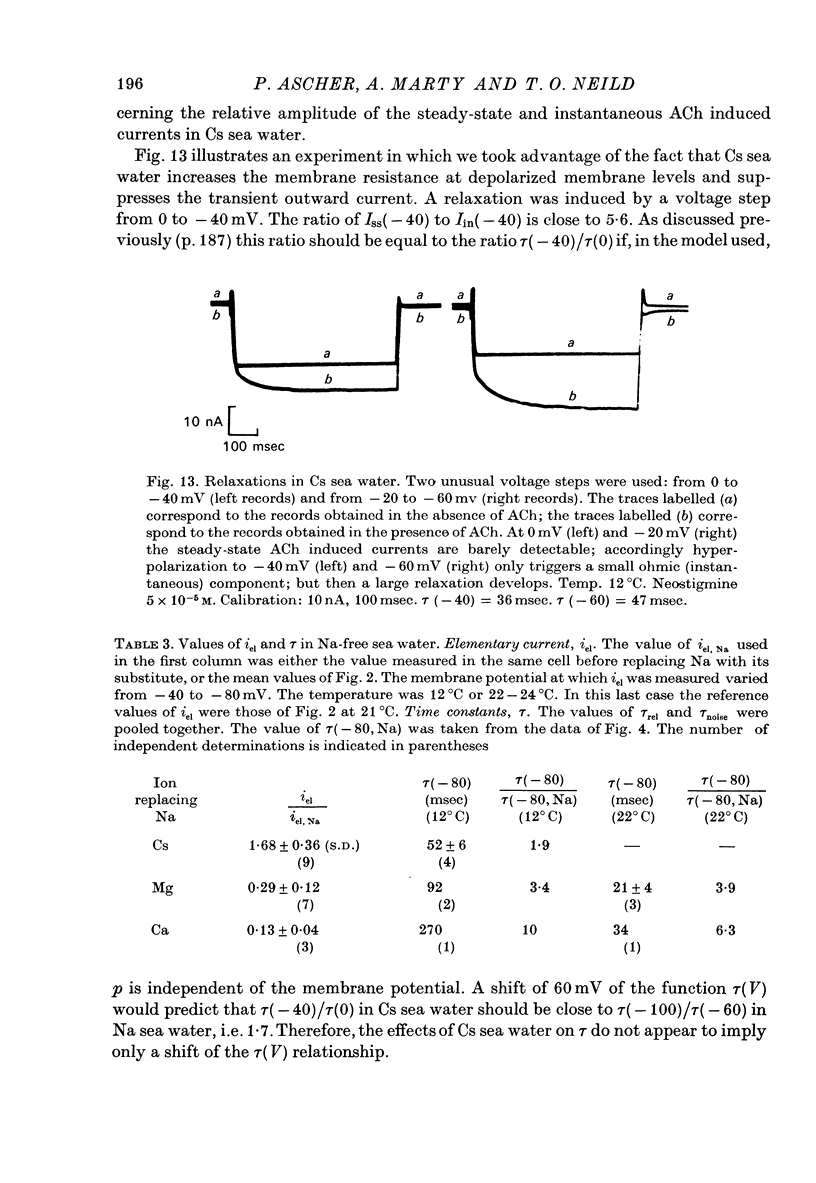
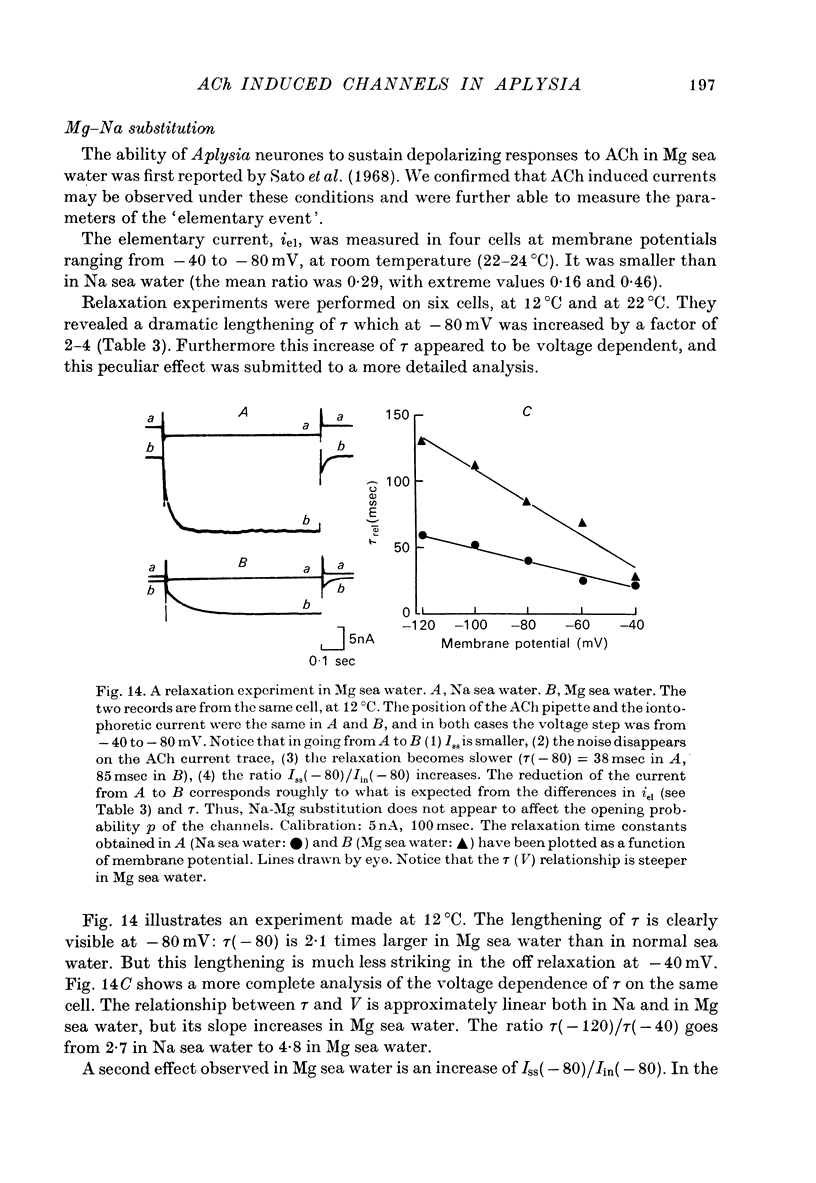
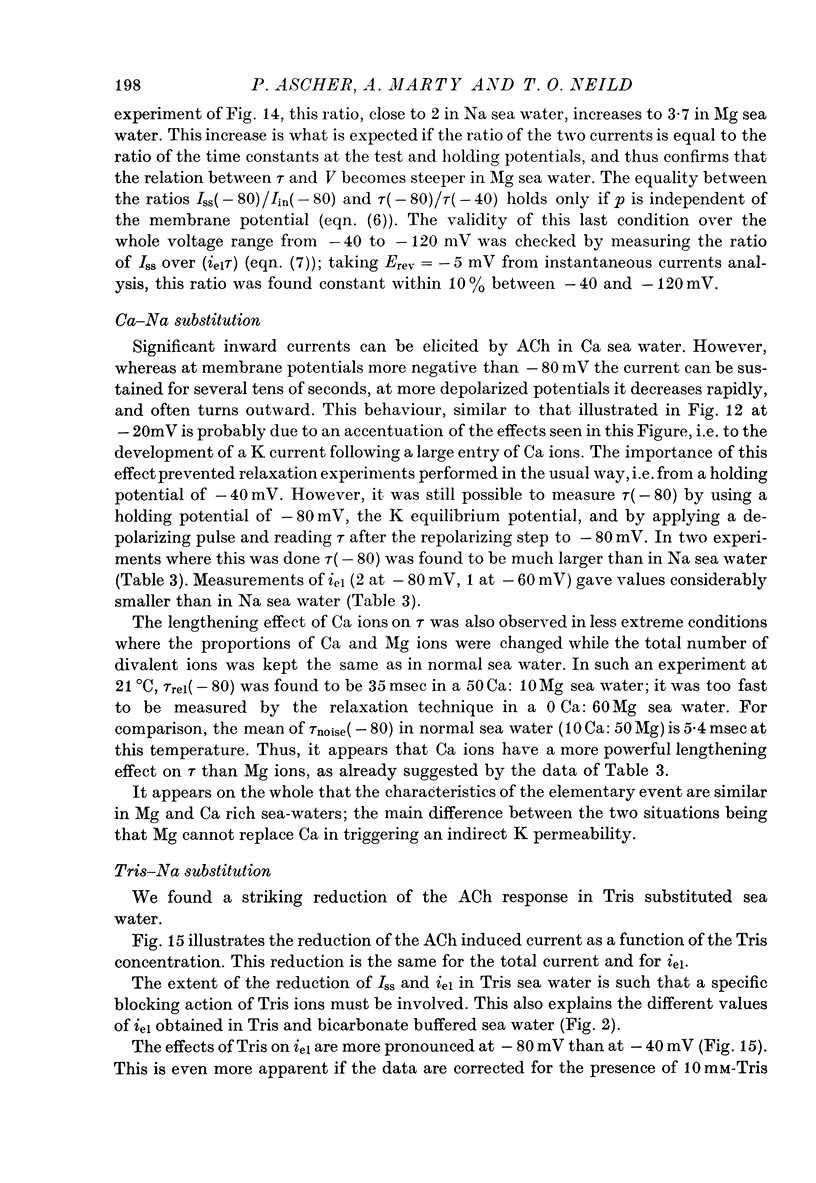
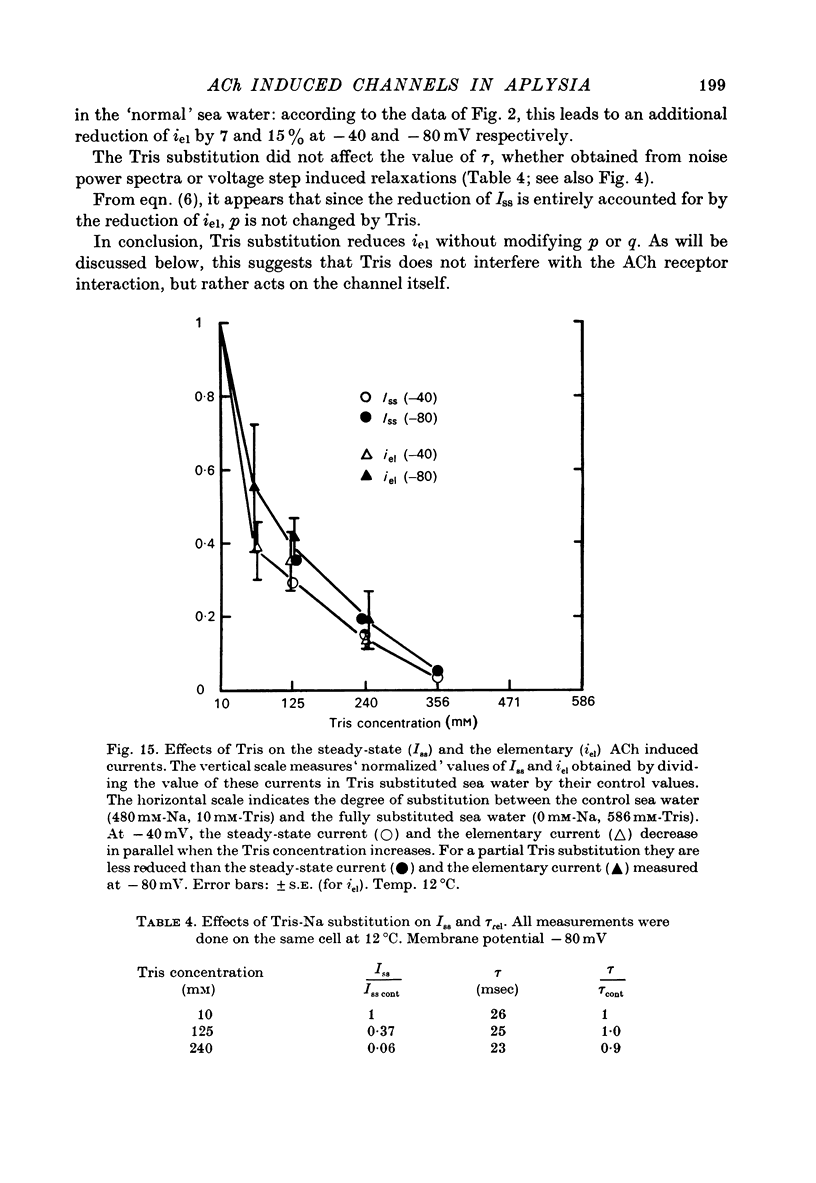
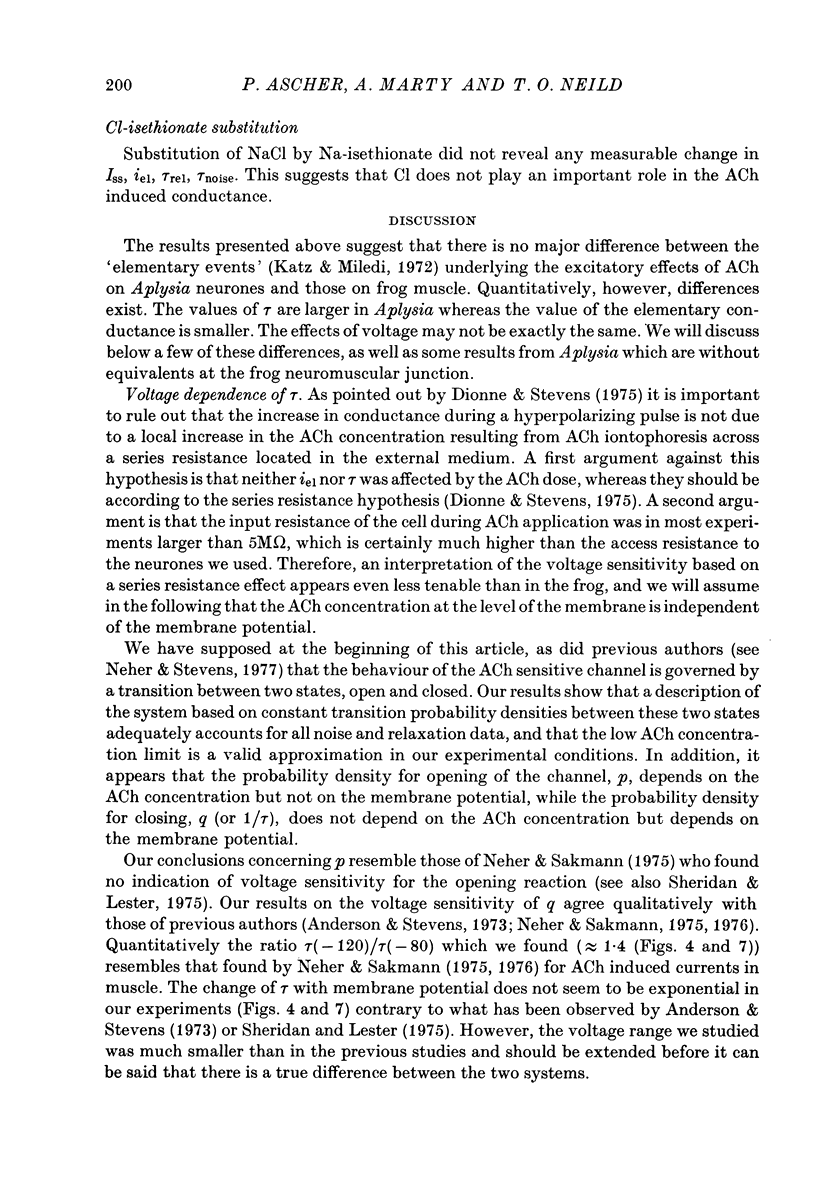
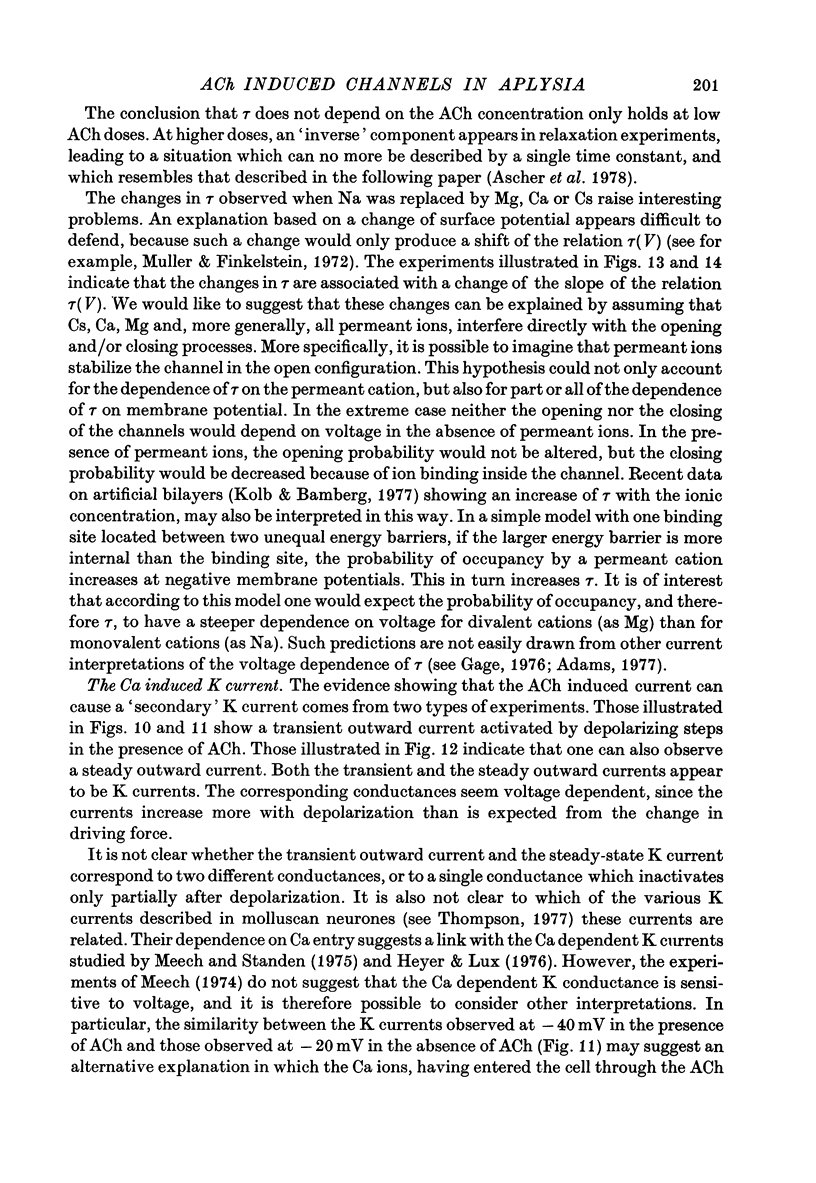
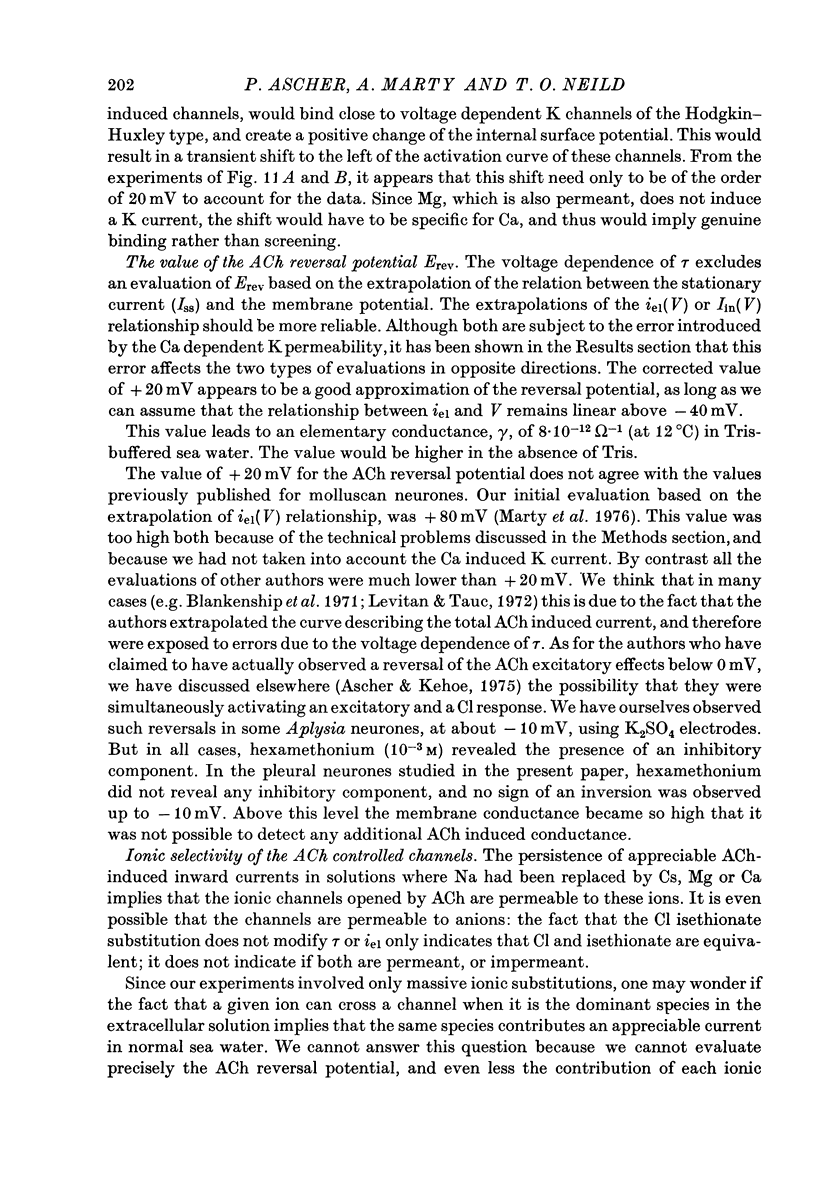
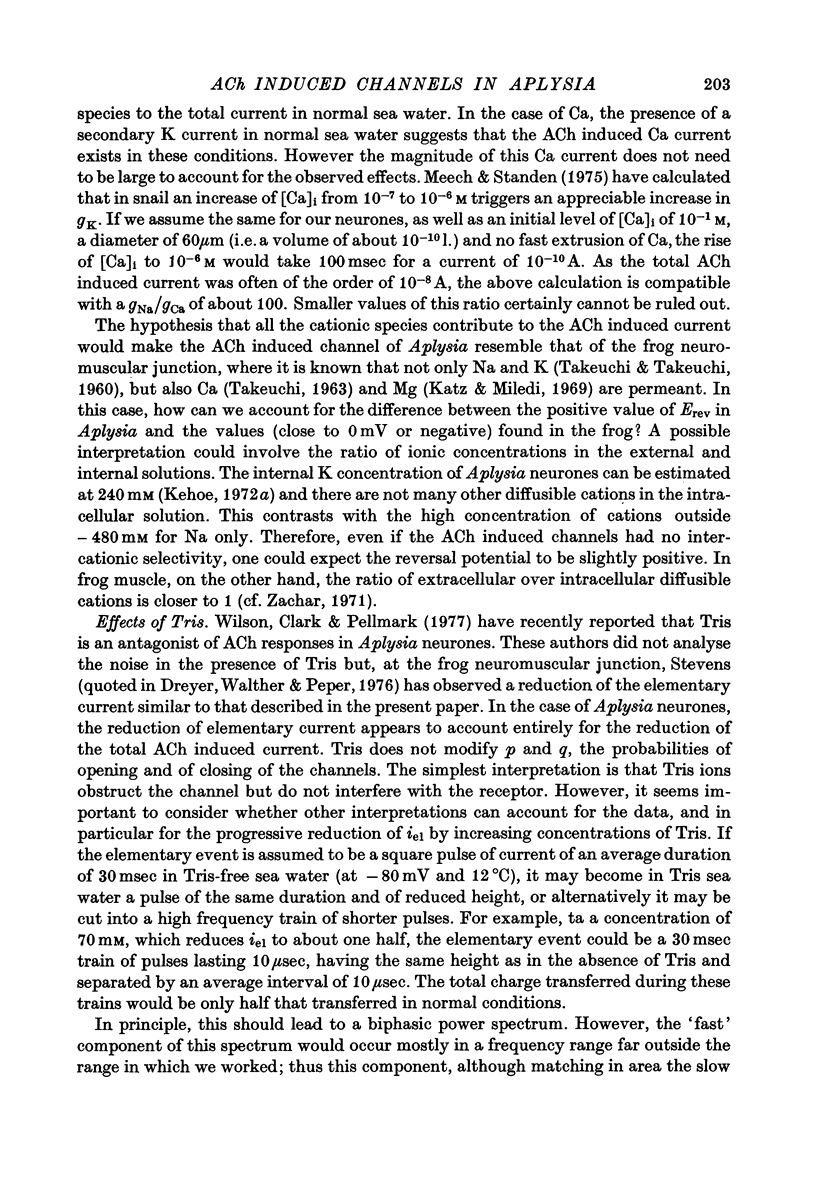
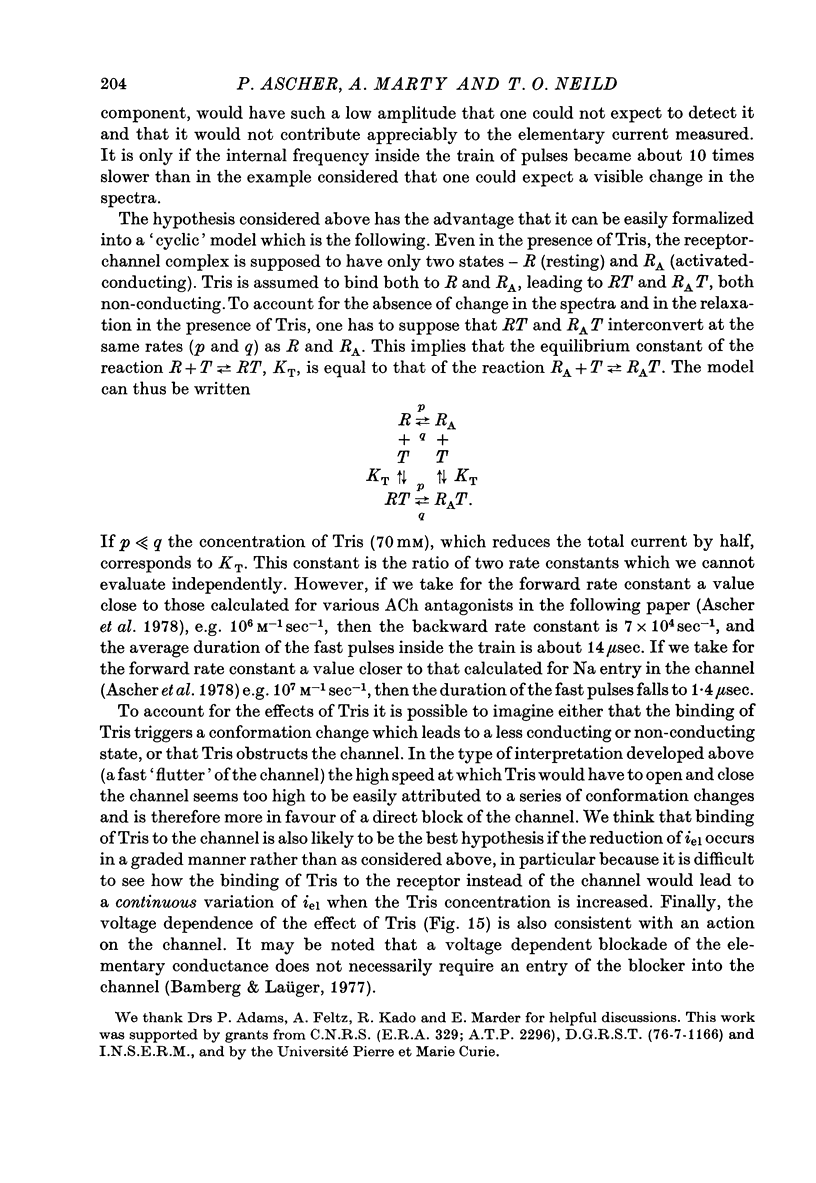
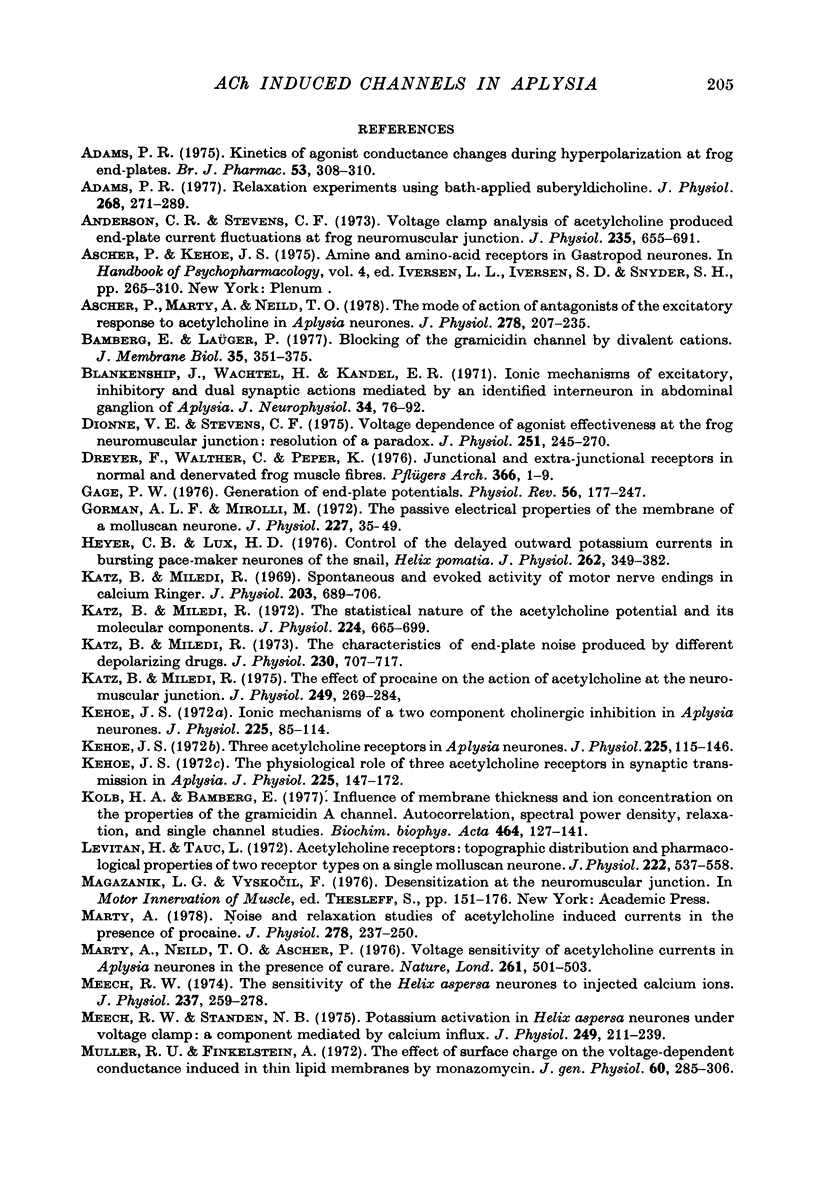
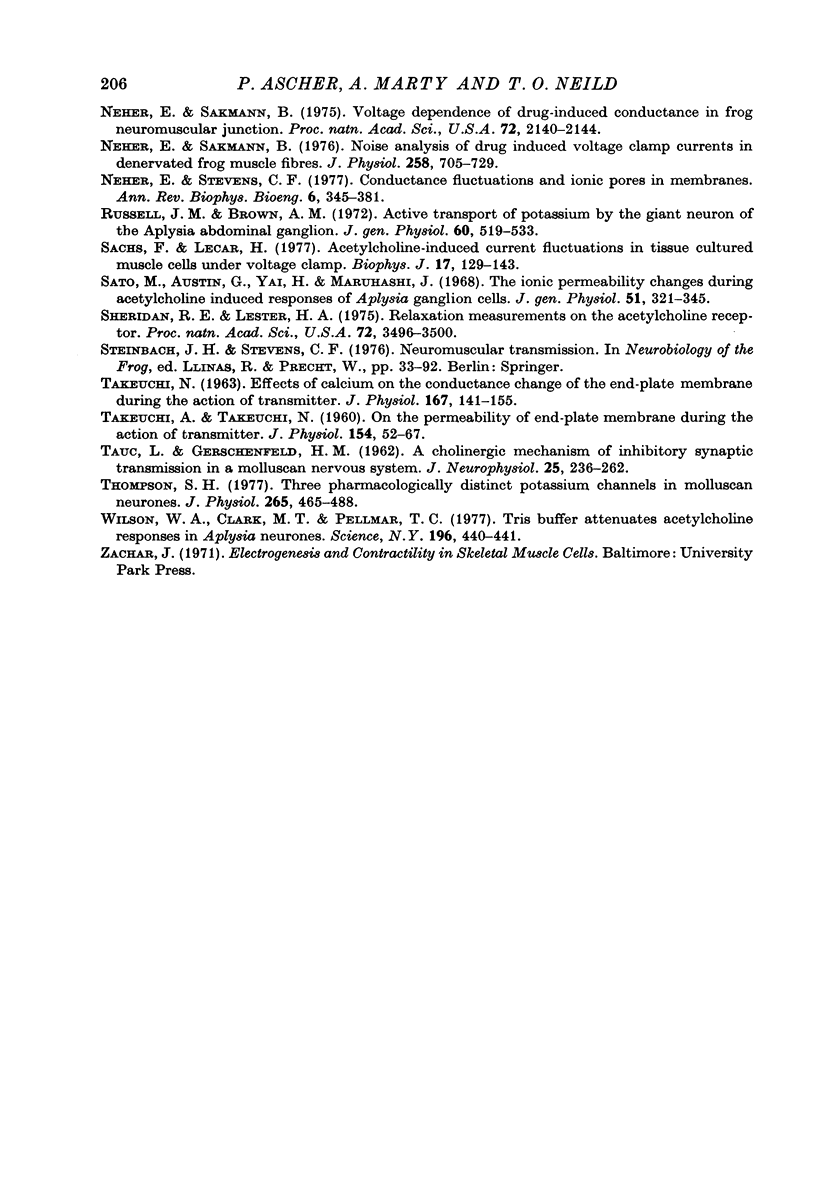
Selected References
These references are in PubMed. This may not be the complete list of references from this article.
- Adams P. R. Kinetics of agonist conductance changes during hyperolarization at frog endplates. Br J Pharmacol. 1975 Feb;53(2):308–310. doi: 10.1111/j.1476-5381.1975.tb07364.x. [DOI] [PMC free article] [PubMed] [Google Scholar]
- Adams P. R. Relaxation experiments using bath-applied suberyldicholine. J Physiol. 1977 Jun;268(2):271–289. doi: 10.1113/jphysiol.1977.sp011857. [DOI] [PMC free article] [PubMed] [Google Scholar]
- Anderson C. R., Stevens C. F. Voltage clamp analysis of acetylcholine produced end-plate current fluctuations at frog neuromuscular junction. J Physiol. 1973 Dec;235(3):655–691. doi: 10.1113/jphysiol.1973.sp010410. [DOI] [PMC free article] [PubMed] [Google Scholar]
- Ascher P., Marty A., Neild T. O. The mode of action of antagonists of the excitatory response to acetylcholine in Aplysia neurones. J Physiol. 1978 May;278:207–235. doi: 10.1113/jphysiol.1978.sp012300. [DOI] [PMC free article] [PubMed] [Google Scholar]
- Blankenship J. E., Wachtel H., Kandel E. R. Ionic mechanisms of excitatory, inhibitory, and dual synaptic actions mediated by an identified interneuron in abdominal ganglion of Aplysia. J Neurophysiol. 1971 Jan;34(1):76–92. doi: 10.1152/jn.1971.34.1.76. [DOI] [PubMed] [Google Scholar]
- Dionne V. E., Stevens C. F. Voltage dependence of agonist effectiveness at the frog neuromuscular junction: resolution of a paradox. J Physiol. 1975 Oct;251(2):245–270. doi: 10.1113/jphysiol.1975.sp011090. [DOI] [PMC free article] [PubMed] [Google Scholar]
- Dreyer F., Walther C., Peper K. Junctional and extrajunctional acetylcholine receptors in normal and denervated frog muscle fibres. Noise analysis experiments with different agonists. Pflugers Arch. 1976 Oct 15;366(1):1–9. doi: 10.1007/BF02486555. [DOI] [PubMed] [Google Scholar]
- Gage P. W. Generation of end-plate potentials. Physiol Rev. 1976 Jan;56(1):177–247. doi: 10.1152/physrev.1976.56.1.177. [DOI] [PubMed] [Google Scholar]
- Gorman A. L., Mirolli M. The passive electrical properties of the membrane of a molluscan neurone. J Physiol. 1972 Dec;227(1):35–49. doi: 10.1113/jphysiol.1972.sp010018. [DOI] [PMC free article] [PubMed] [Google Scholar]
- Heyer C. B., Lux H. D. Control of the delayed outward potassium currents in bursting pace-maker neurones of the snail, Helix pomatia. J Physiol. 1976 Nov;262(2):349–382. doi: 10.1113/jphysiol.1976.sp011599. [DOI] [PMC free article] [PubMed] [Google Scholar]
- Katz B., Miledi R. Spontaneous and evoked activity of motor nerve endings in calcium Ringer. J Physiol. 1969 Aug;203(3):689–706. doi: 10.1113/jphysiol.1969.sp008887. [DOI] [PMC free article] [PubMed] [Google Scholar]
- Katz B., Miledi R. The characteristics of 'end-plate noise' produced by different depolarizing drugs. J Physiol. 1973 May;230(3):707–717. doi: 10.1113/jphysiol.1973.sp010213. [DOI] [PMC free article] [PubMed] [Google Scholar]
- Katz B., Miledi R. The effect of procaine on the action of acetylcholine at the neuromuscular junction. J Physiol. 1975 Jul;249(2):269–284. doi: 10.1113/jphysiol.1975.sp011015. [DOI] [PMC free article] [PubMed] [Google Scholar]
- Katz B., Miledi R. The statistical nature of the acetycholine potential and its molecular components. J Physiol. 1972 Aug;224(3):665–699. doi: 10.1113/jphysiol.1972.sp009918. [DOI] [PMC free article] [PubMed] [Google Scholar]
- Kehoe J. Ionic mechanisms of a two-component cholinergic inhibition in Aplysia neurones. J Physiol. 1972 Aug;225(1):85–114. doi: 10.1113/jphysiol.1972.sp009930. [DOI] [PMC free article] [PubMed] [Google Scholar]
- Kehoe J. The physiological role of three acetylcholine receptors in synaptic transmission in Aplysia. J Physiol. 1972 Aug;225(1):147–172. doi: 10.1113/jphysiol.1972.sp009932. [DOI] [PMC free article] [PubMed] [Google Scholar]
- Kehoe J. Three acetylcholine receptors in Aplysia neurones. J Physiol. 1972 Aug;225(1):115–146. doi: 10.1113/jphysiol.1972.sp009931. [DOI] [PMC free article] [PubMed] [Google Scholar]
- Kolb H. A., Bamberg E. Influence of membrane thickness and ion concentration on the properties of the gramicidin a channel. Autocorrelation, spectral power density, relaxation and single-channel studies. Biochim Biophys Acta. 1977 Jan 4;464(1):127–141. doi: 10.1016/0005-2736(77)90376-5. [DOI] [PubMed] [Google Scholar]
- Levitan H., Tauc L. Acetylcholine receptors: topographic distribution and pharmacological properties of two receptor types on a single molluscan neurone. J Physiol. 1972 May;222(3):537–558. doi: 10.1113/jphysiol.1972.sp009813. [DOI] [PMC free article] [PubMed] [Google Scholar]
- Marty A., Neild T., Ascher P. Voltage sensitivity of acetylcholine currents in Aplysia neurones in the presence of curare. Nature. 1976 Jun 10;261(5560):501–503. doi: 10.1038/261501a0. [DOI] [PubMed] [Google Scholar]
- Marty A. Noise and relaxation studies of acetylcholine induced currents in the presence of procaine. J Physiol. 1978 May;278:237–250. doi: 10.1113/jphysiol.1978.sp012301. [DOI] [PMC free article] [PubMed] [Google Scholar]
- Meech R. W., Standen N. B. Potassium activation in Helix aspersa neurones under voltage clamp: a component mediated by calcium influx. J Physiol. 1975 Jul;249(2):211–239. doi: 10.1113/jphysiol.1975.sp011012. [DOI] [PMC free article] [PubMed] [Google Scholar]
- Meech R. W. The sensitivity of Helix aspersa neurones to injected calcium ions. J Physiol. 1974 Mar;237(2):259–277. doi: 10.1113/jphysiol.1974.sp010481. [DOI] [PMC free article] [PubMed] [Google Scholar]
- Muller R. U., Finkelstein A. The effect of surface charge on the voltage-dependent conductance induced in thin lipid membranes by monazomycin. J Gen Physiol. 1972 Sep;60(3):285–306. doi: 10.1085/jgp.60.3.285. [DOI] [PMC free article] [PubMed] [Google Scholar]
- Neher E., Sakmann B. Noise analysis of drug induced voltage clamp currents in denervated frog muscle fibres. J Physiol. 1976 Jul;258(3):705–729. doi: 10.1113/jphysiol.1976.sp011442. [DOI] [PMC free article] [PubMed] [Google Scholar]
- Neher E., Sakmann B. Voltage-dependence of drug-induced conductance in frog neuromuscular junction. Proc Natl Acad Sci U S A. 1975 Jun;72(6):2140–2144. doi: 10.1073/pnas.72.6.2140. [DOI] [PMC free article] [PubMed] [Google Scholar]
- Neher E., Stevens C. F. Conductance fluctuations and ionic pores in membranes. Annu Rev Biophys Bioeng. 1977;6:345–381. doi: 10.1146/annurev.bb.06.060177.002021. [DOI] [PubMed] [Google Scholar]
- Russell J. M., Brown A. M. Active transport of potassium by the giant neuron of the aplysia abdominal ganglion. J Gen Physiol. 1972 Nov;60(5):519–533. doi: 10.1085/jgp.60.5.519. [DOI] [PMC free article] [PubMed] [Google Scholar]
- Sachs F., Lecar H. Acetylcholine-induced current fluctuations in tissue-cultured muscle cells under voltage clamp. Biophys J. 1977 Feb;17(2):129–143. doi: 10.1016/S0006-3495(77)85631-2. [DOI] [PMC free article] [PubMed] [Google Scholar]
- Sato M., Austin G., Yai H., Maruhashi J. The ionic permeability changes during acetylcholine-induced responses of Aplysia ganglion cells. J Gen Physiol. 1968 Mar;51(3):321–345. doi: 10.1085/jgp.51.3.321. [DOI] [PMC free article] [PubMed] [Google Scholar]
- Sheridan R. E., Lester H. A. Relaxation measurements on the acetylcholine receptor. Proc Natl Acad Sci U S A. 1975 Sep;72(9):3496–3500. doi: 10.1073/pnas.72.9.3496. [DOI] [PMC free article] [PubMed] [Google Scholar]
- TAKEUCHI A., TAKEUCHI N. On the permeability of end-plate membrane during the action of transmitter. J Physiol. 1960 Nov;154:52–67. doi: 10.1113/jphysiol.1960.sp006564. [DOI] [PMC free article] [PubMed] [Google Scholar]
- TAUC L., GERSCHENFELD H. M. A cholinergic mechanism of inhibitory synaptic transmission in a molluscan nervous system. J Neurophysiol. 1962 Mar;25:236–262. doi: 10.1152/jn.1962.25.2.236. [DOI] [PubMed] [Google Scholar]
- Thompson S. H. Three pharmacologically distinct potassium channels in molluscan neurones. J Physiol. 1977 Feb;265(2):465–488. doi: 10.1113/jphysiol.1977.sp011725. [DOI] [PMC free article] [PubMed] [Google Scholar]
- Wilson W. A., Clark M. T., Pellmar T. C. Tris buffer attenuates acetylcholine responses in Aplysia neurons. Science. 1977 Apr 22;196(4288):440–441. doi: 10.1126/science.15317. [DOI] [PubMed] [Google Scholar]