Abstract
1. A three-electrode voltage clamp method was used to investigate the effects of Ba and Sr ions on the inwardly rectifying K conductance of resting frog sartorius muscle fibres. 2. When Ba2+ (0.01-5 mM) was added to the control (115 mM-K+) solution the inward currents recorded during hyperpolarizing voltage steps turned off exponentially with time as the blockade by Ba2+ developed. Outward currents showed no time-dependence. 3. Ba2+ ions reduced both the instantaneous and the steady-state values of currents recorded on hyperpolarization. The blockade was potential-dependent, steady-state currents being increasingly reduced with increasing hyperpolarization. 4. The concentration-effect relation for the blockade of instantaneous currents by Ba2+ could be fitted assuming 1:1 binding of Ba2+ to a receptor, with the block being proportional to the number of Ba2+-filled receptors. The apparent dissociation constant at the holding potential (-5 mV) was 0.65 mM. Concentration-effect relations were shifted along the concentration axis to lower concentrations by hyperpolarization. The apparent dissociation constant was reduced e-fold for a 16.8 mV change in potential. 5. Increasing the [Ba]o increased the rate of onset of the blockade at a given potential. 6. The rate of onset of the blockade had a high temperature dependence (Q10 = 3.15 +/- 0.08). 7. When [K]o was doubled to 230 mM, under conditions where [K]i was also doubled, [Ba]o had to be raised approximately fourfold to produce the same degree and rate of onset of blockade. Similarly, when [K]o was decreased, the degree and rate of onset of blockade were increased for a given [Ba]o. 8. The blockade could be readily removed by removal of Ba2+ from the bathing solution. In addition the blockade which develops on hyperpolarization is removed exponentially on return to the holding potential. 9. The blockade which exists at the holding potential may be removed by a depolarizing prepulse. 10. Sr causes a similar potential-dependent blockade to that by Ba2+, but is around 400 times less effective. 11. The results have been fitted with a model assuming that the permeability mechanism is an aqueous pore with a site which binds one Ba2+ ion or two K+ ions. The site must have affinity for Ba2+ and a low affinity for K+.
Full text
PDF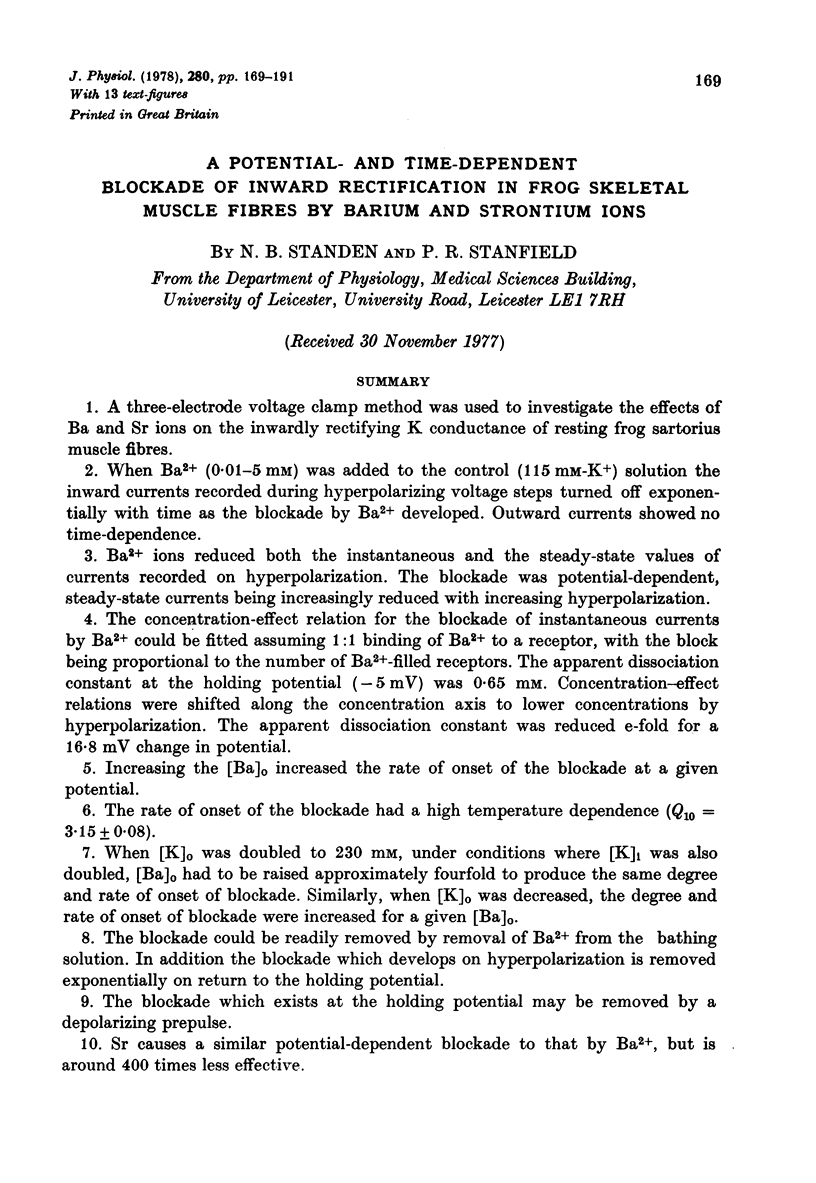
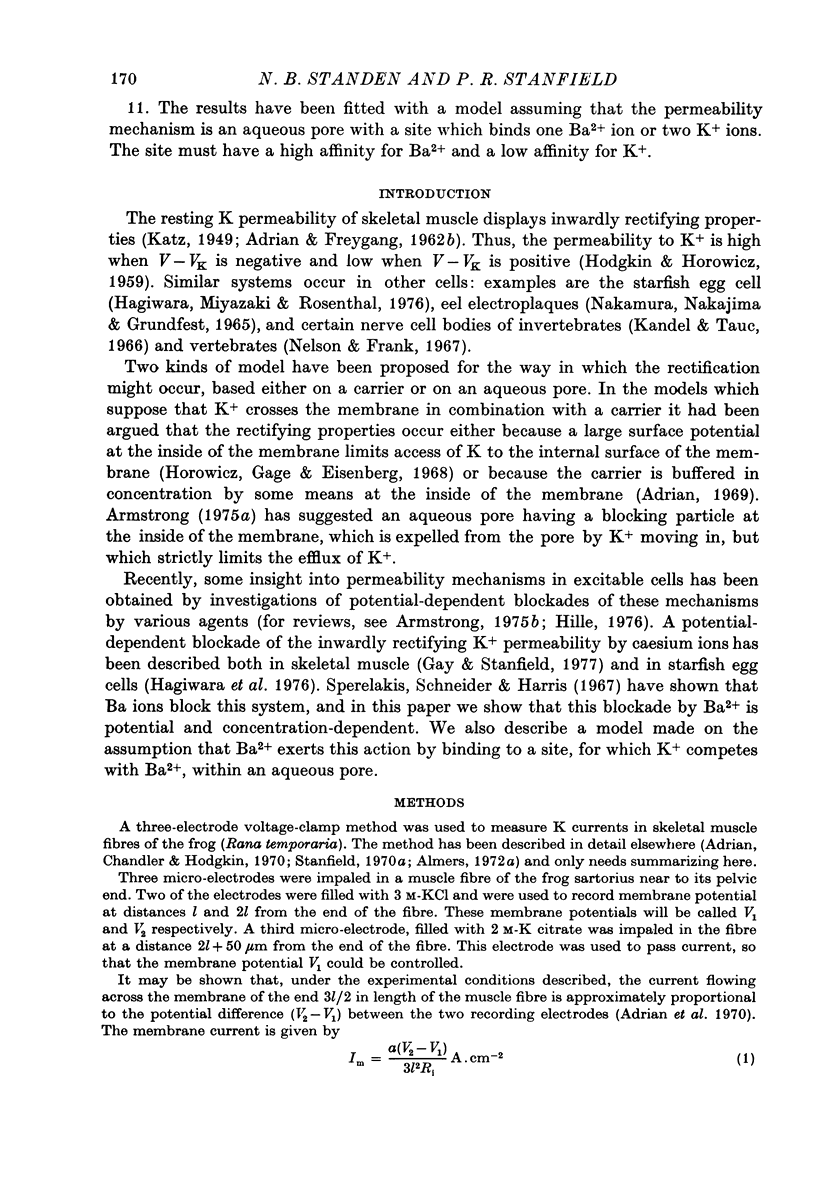
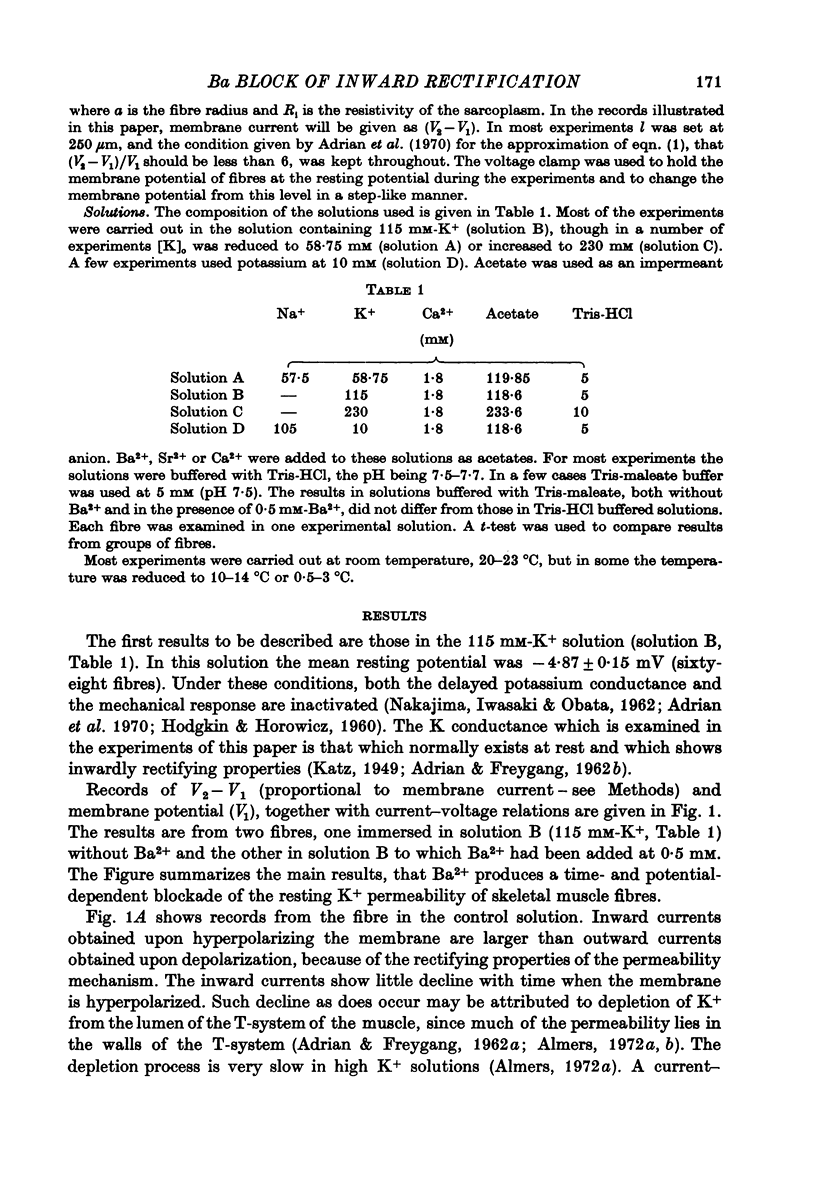
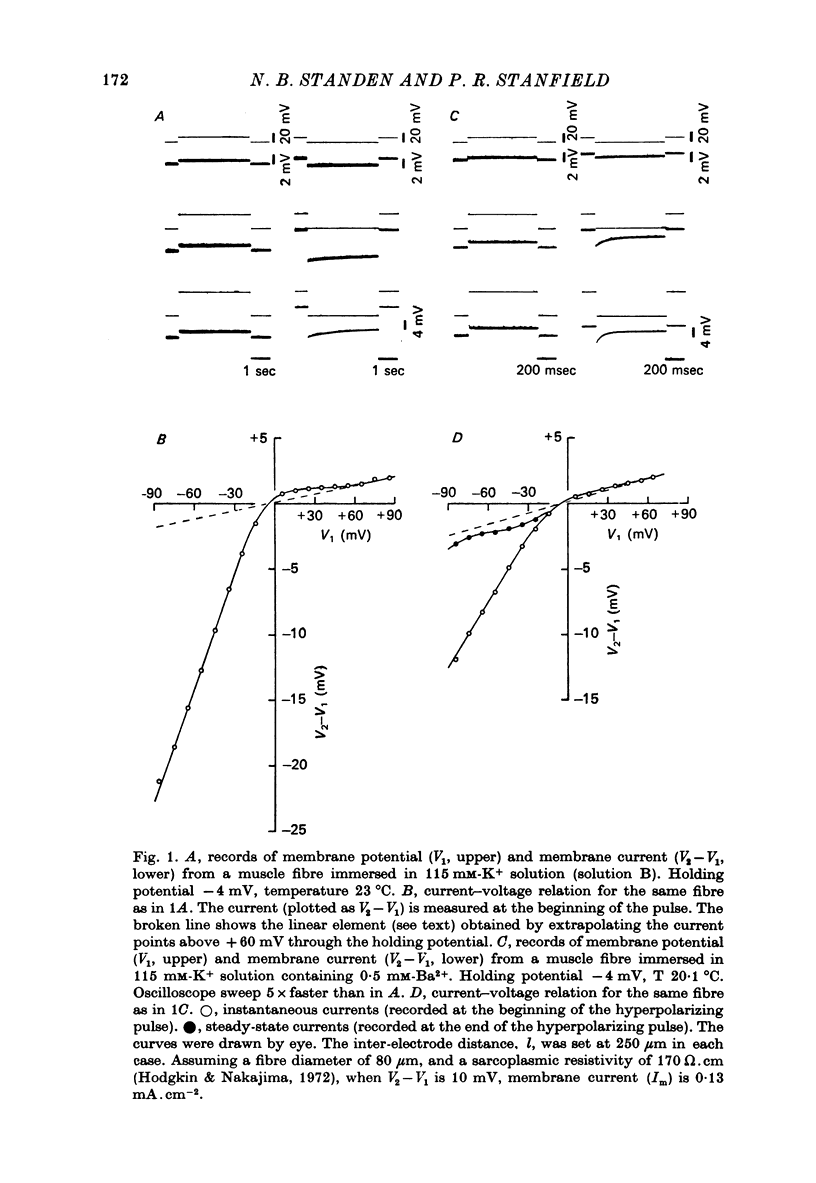
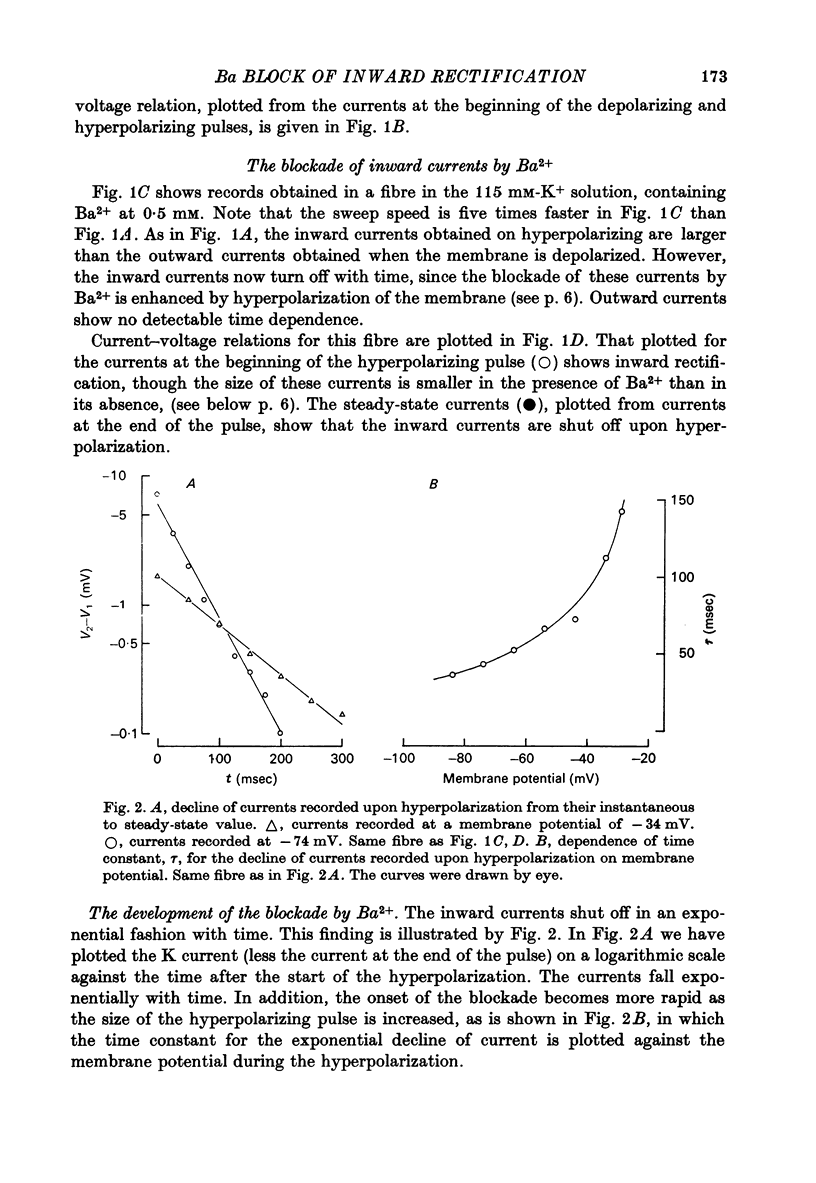
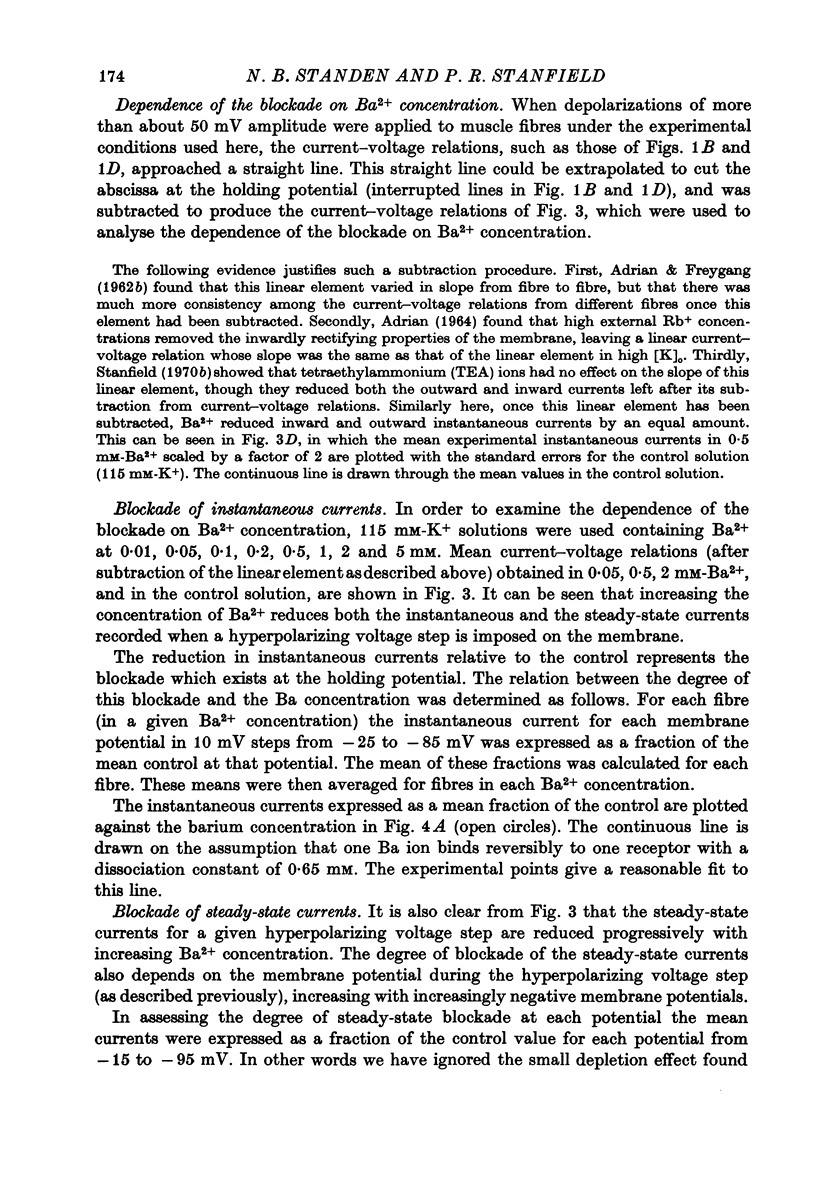
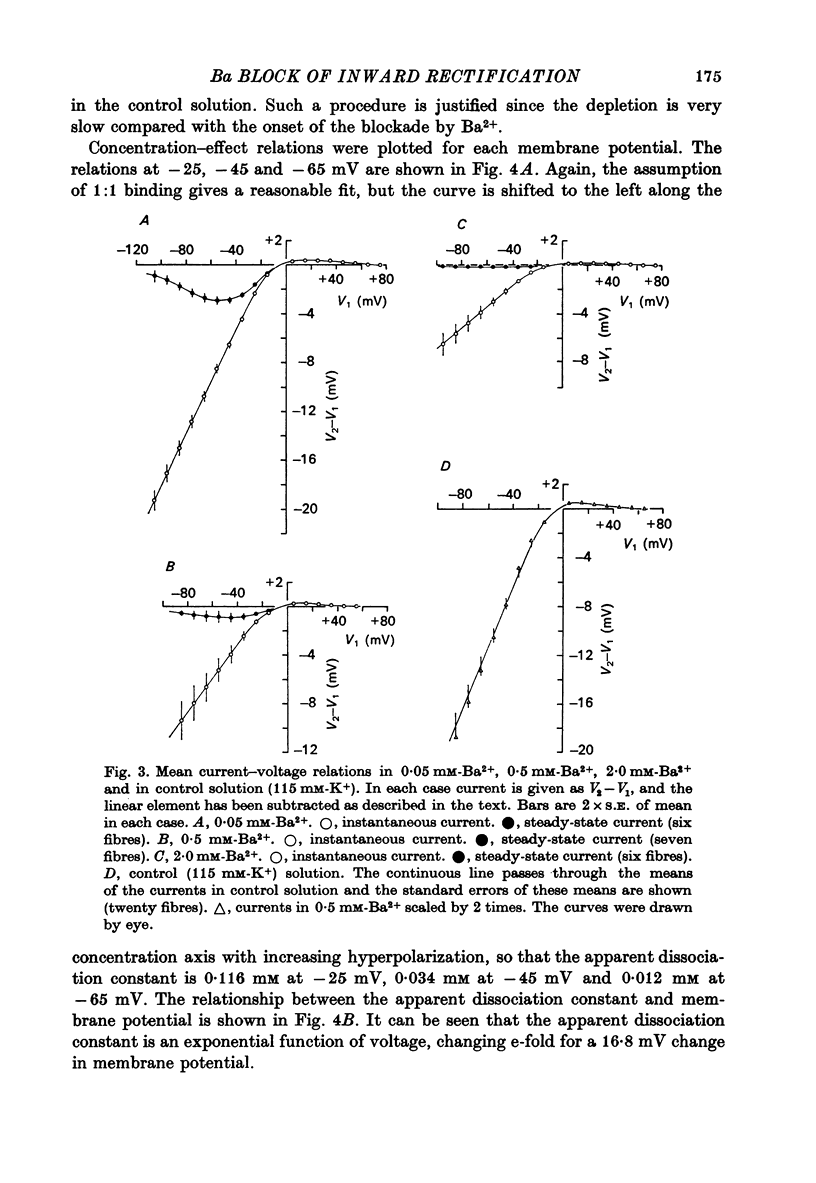
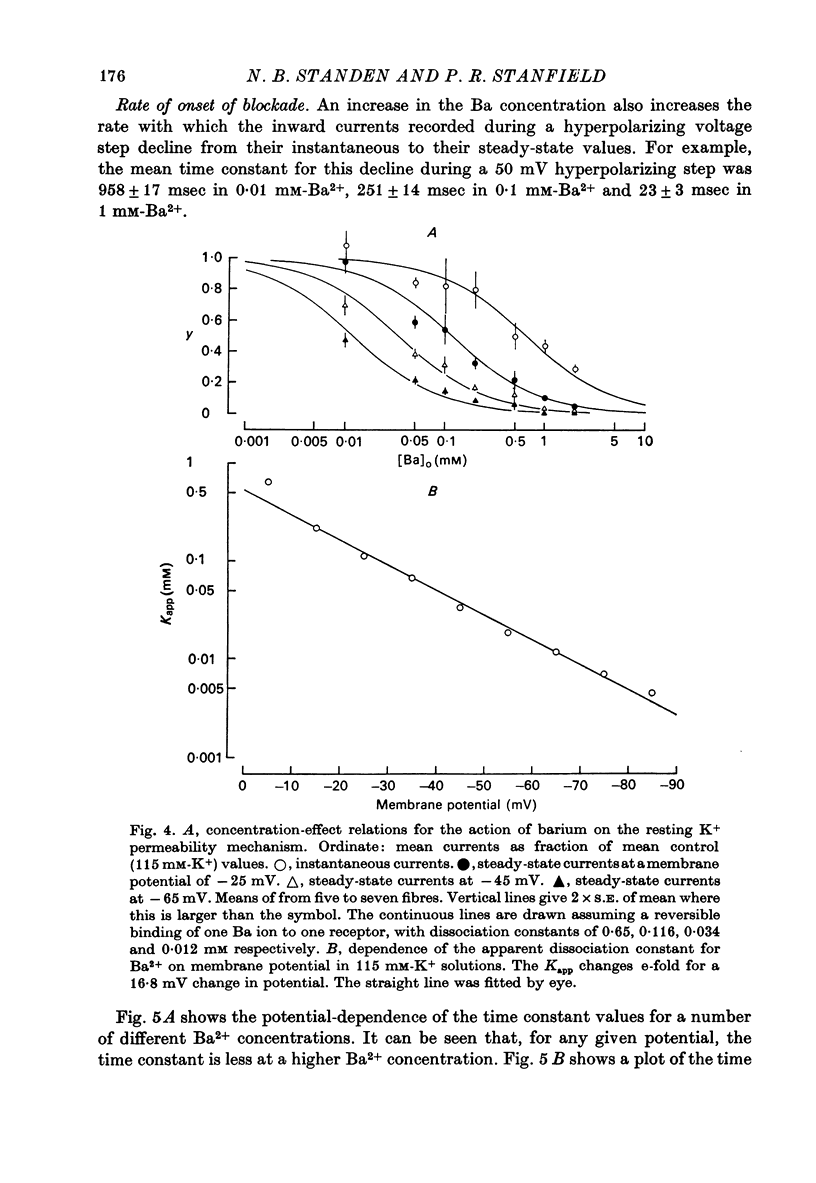
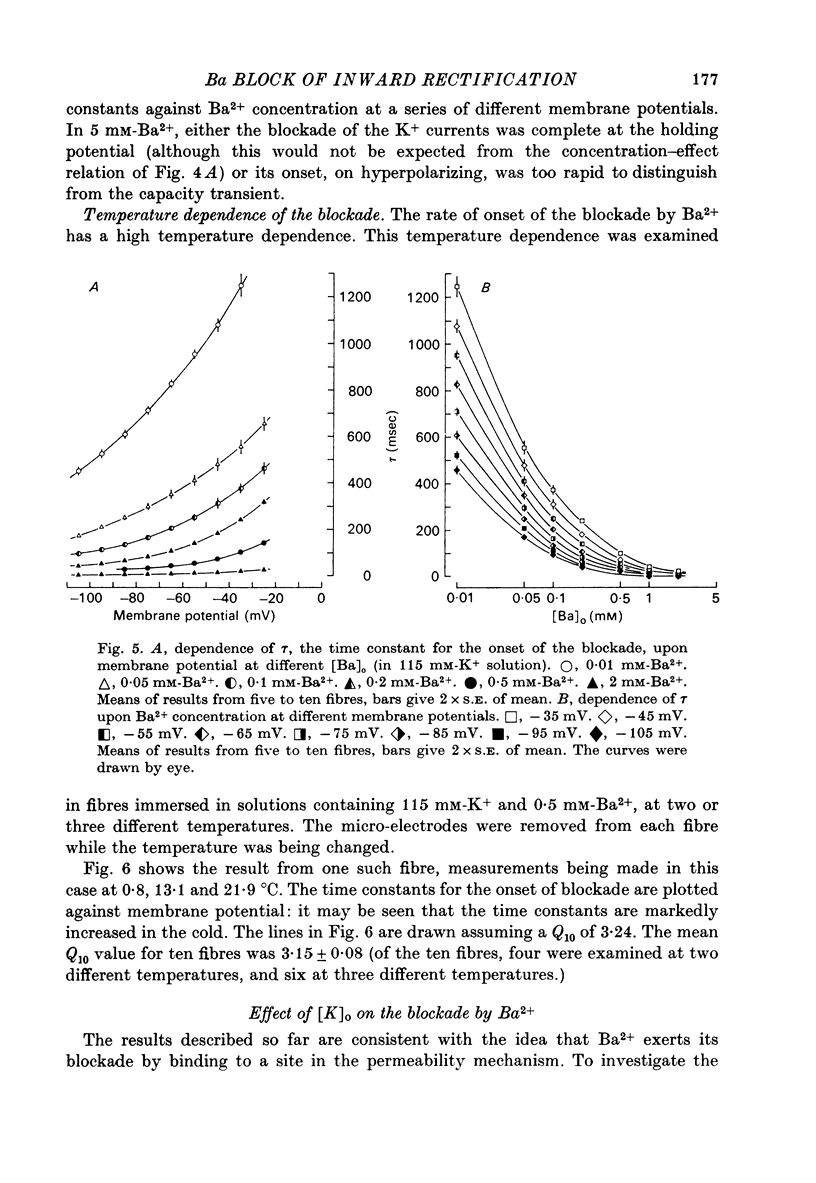
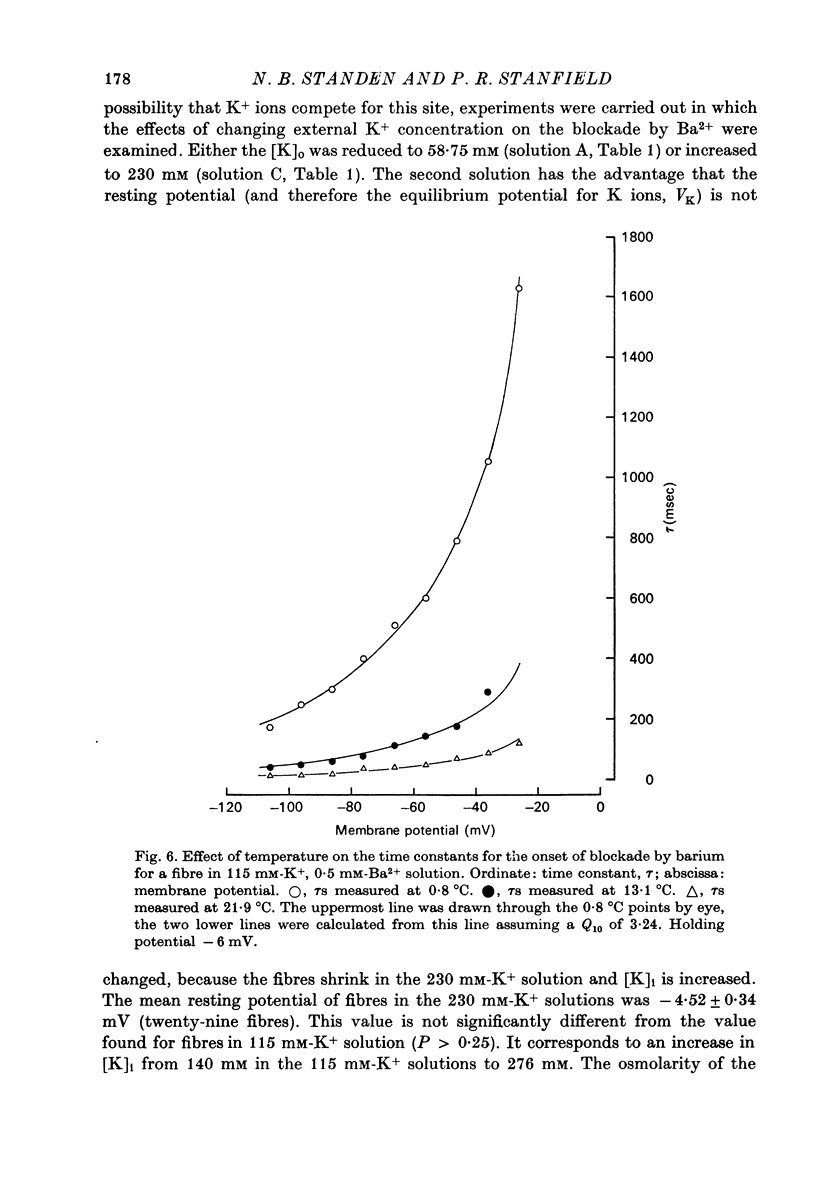
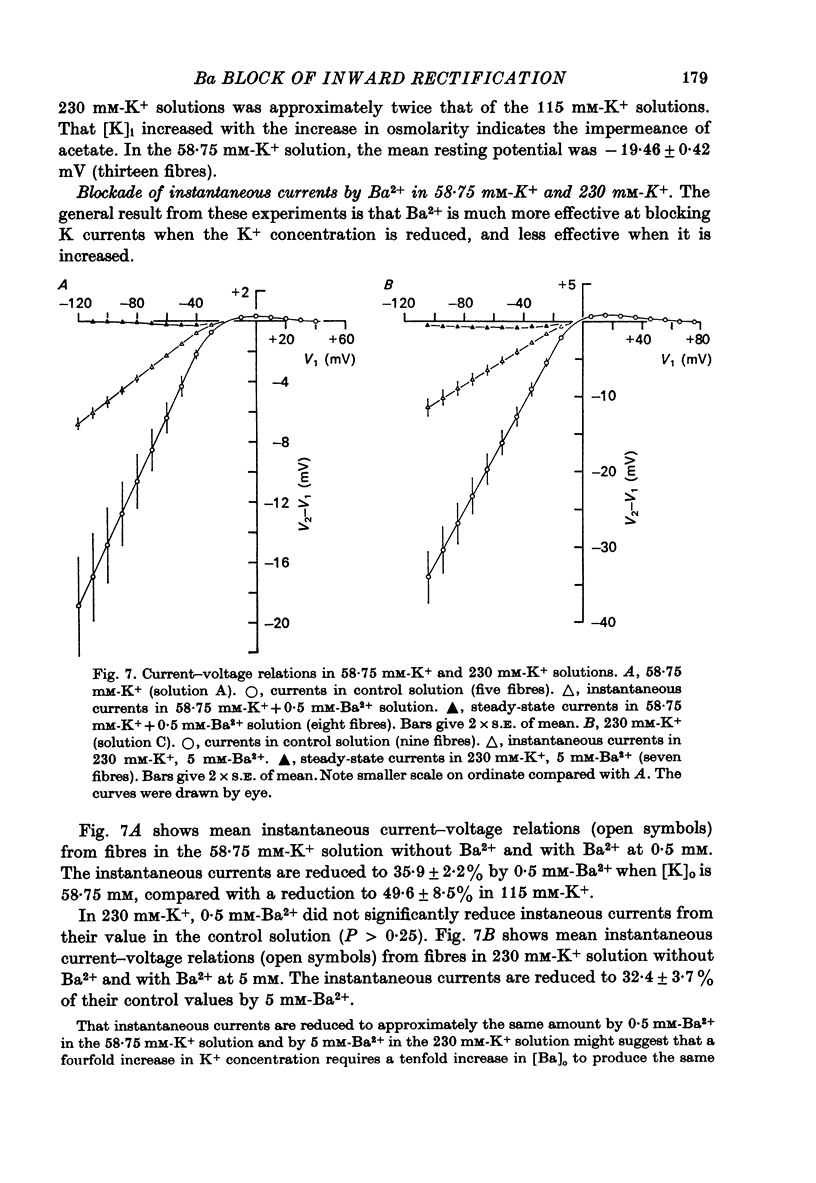
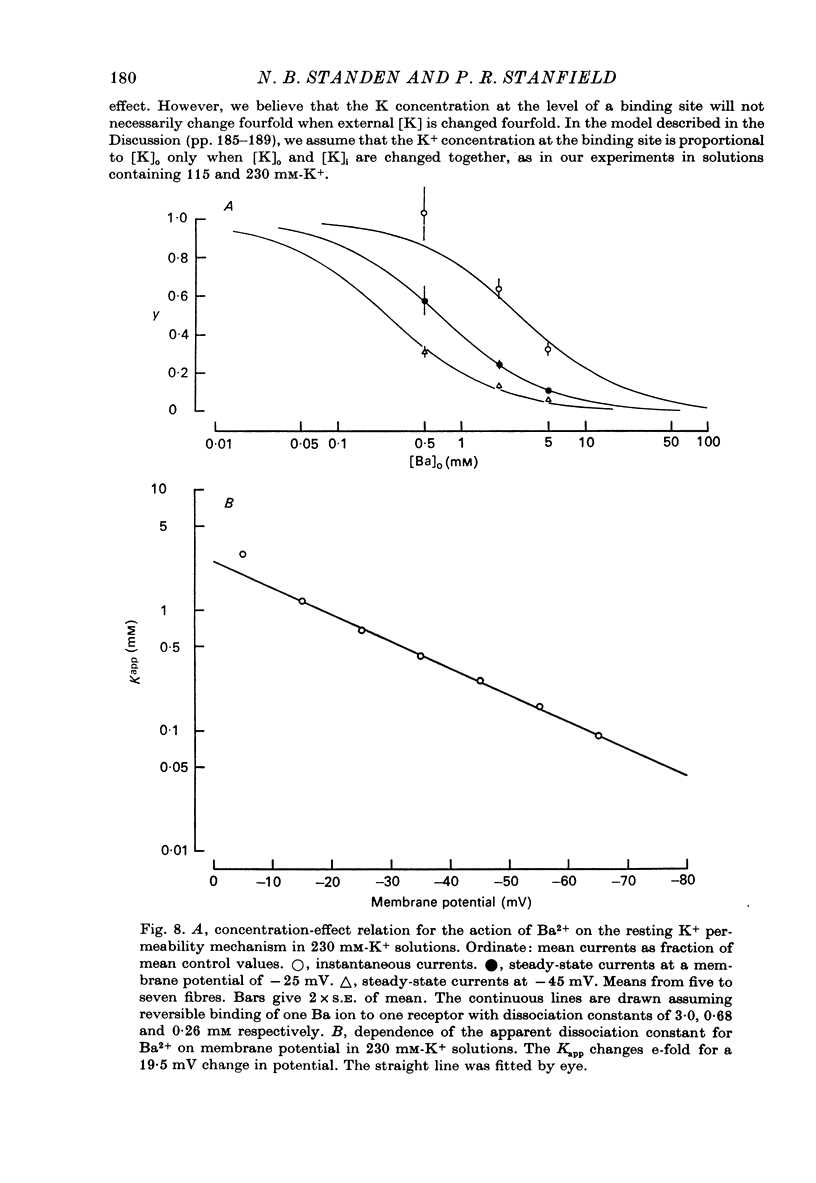
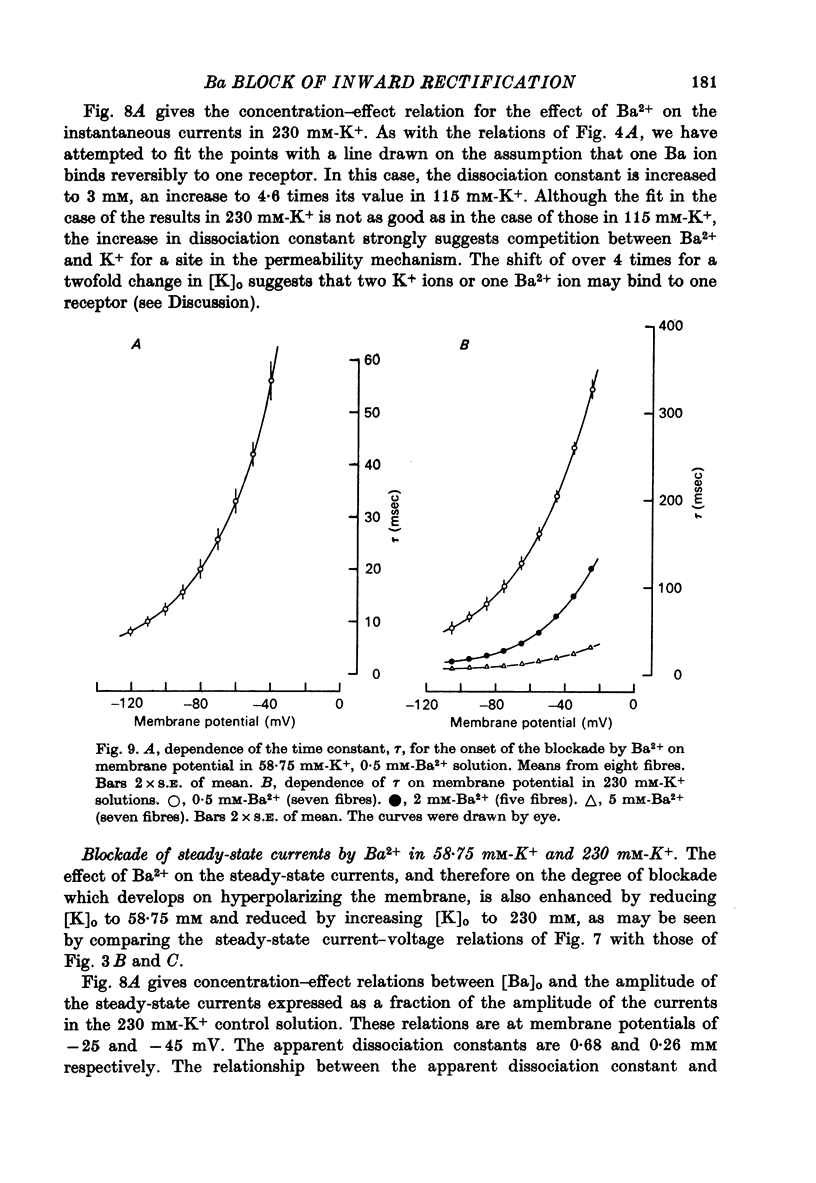
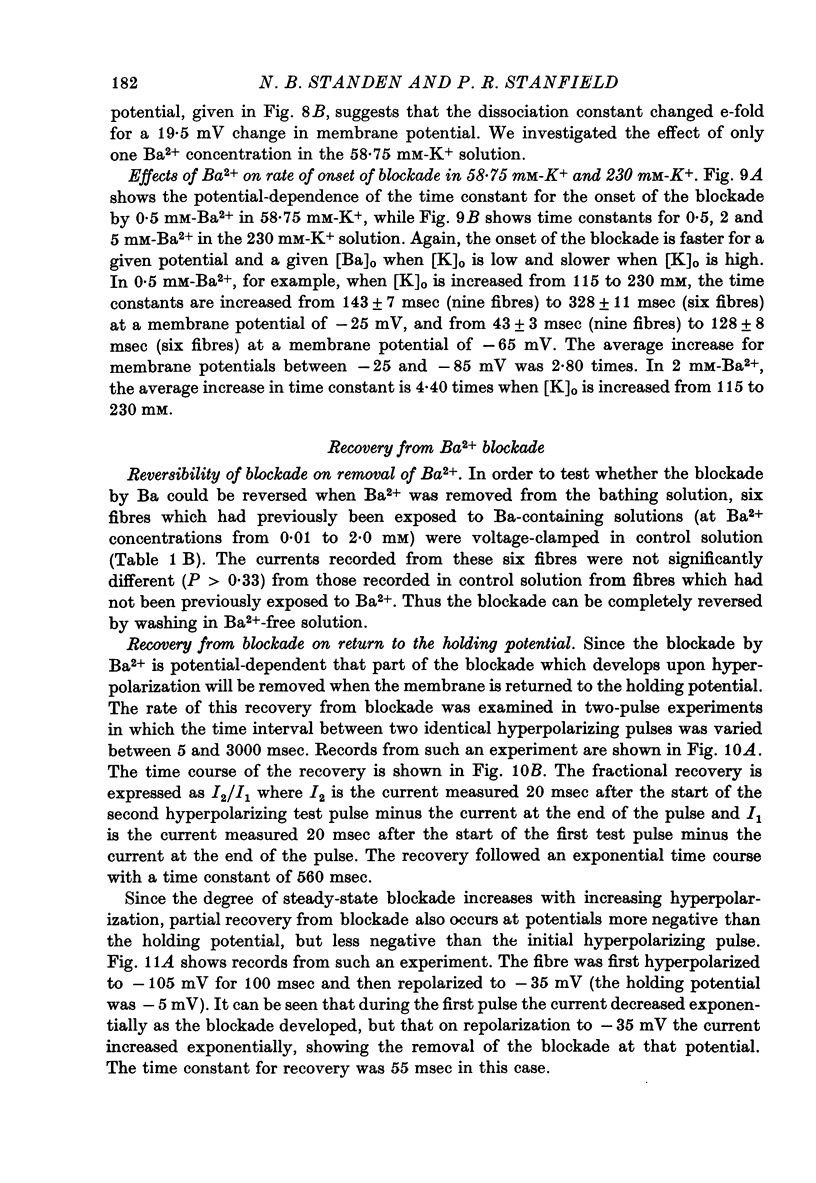
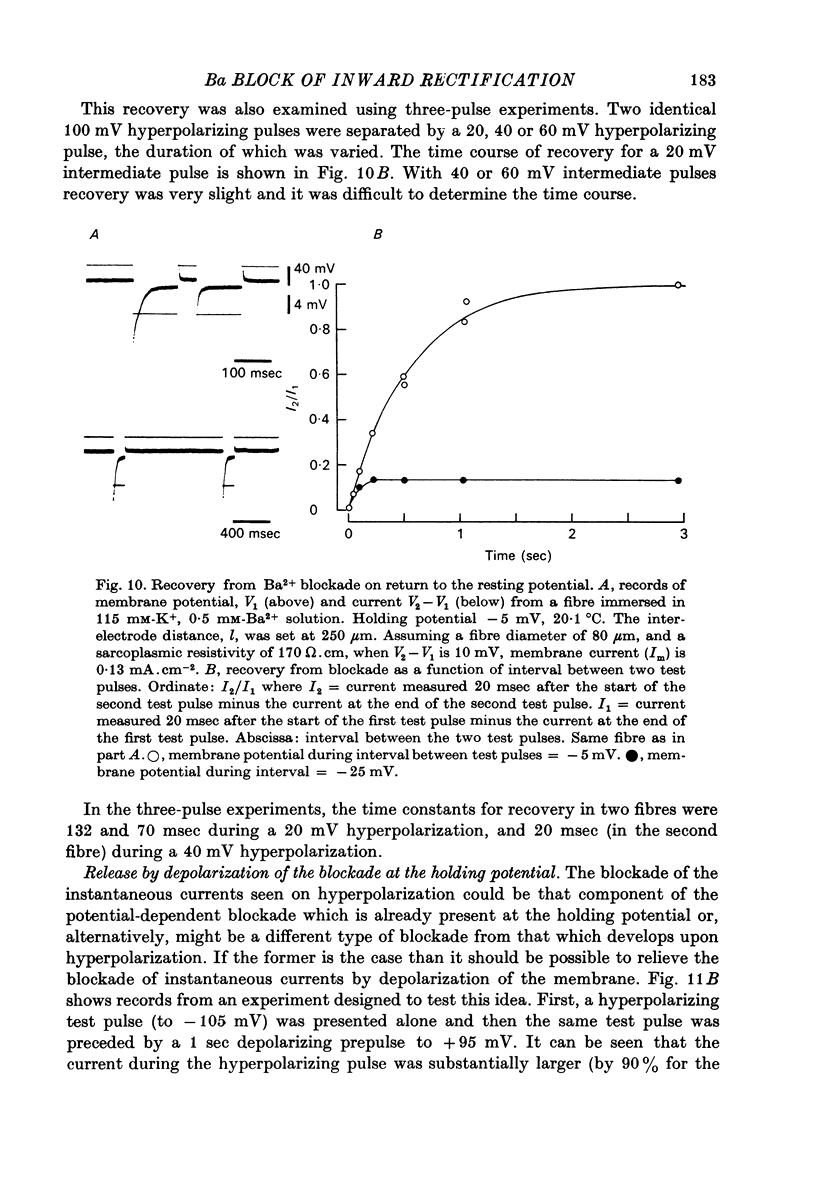
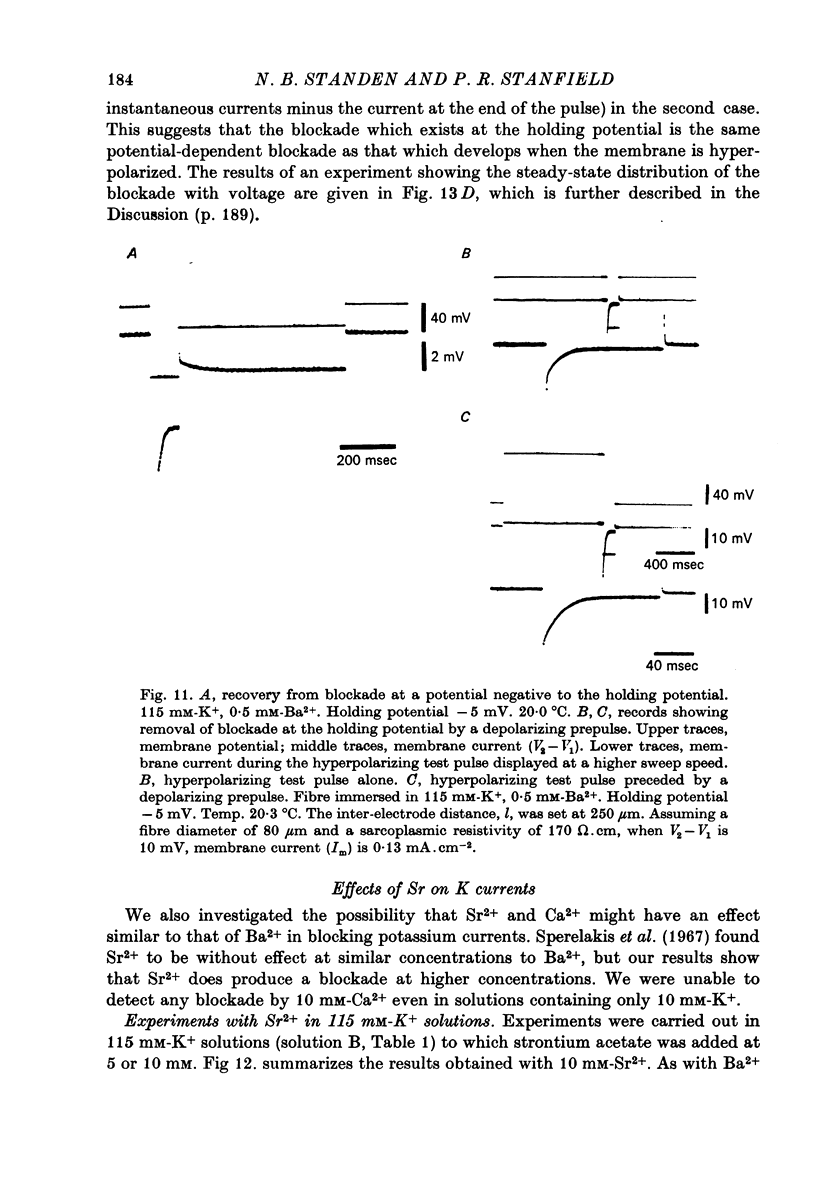
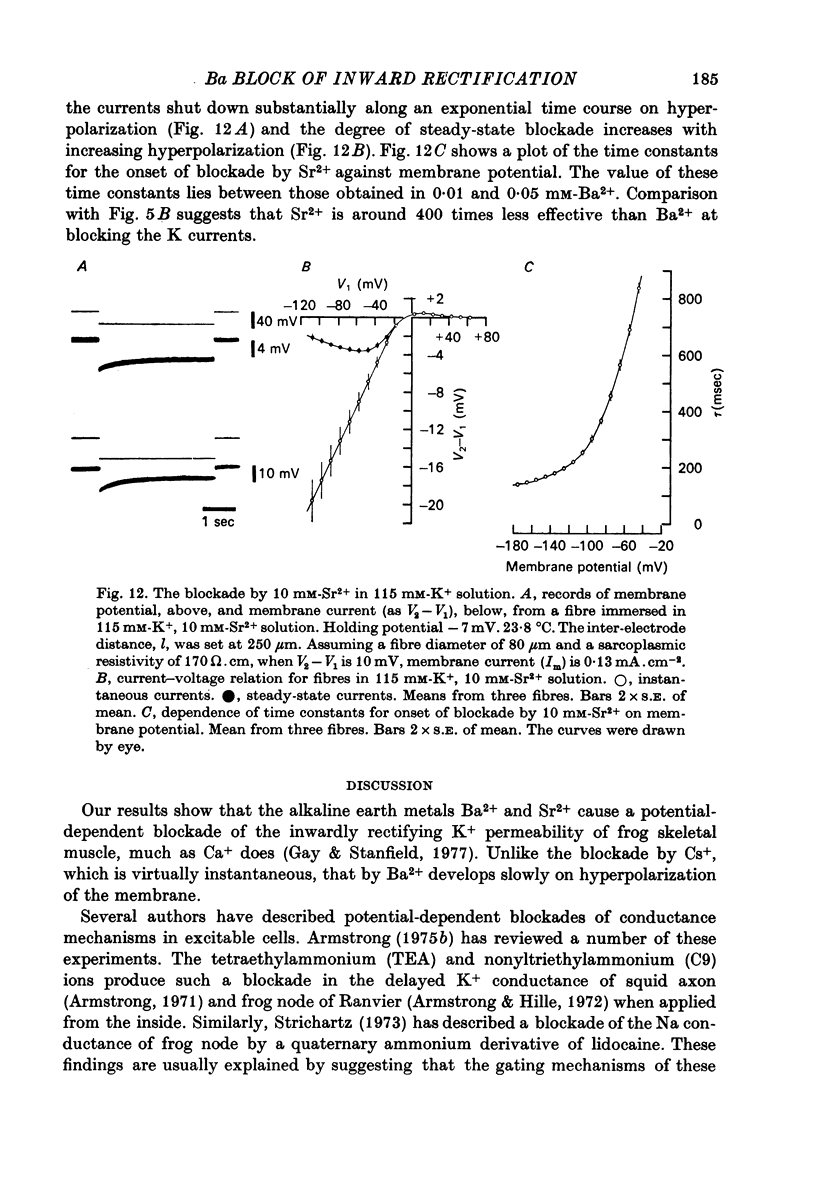
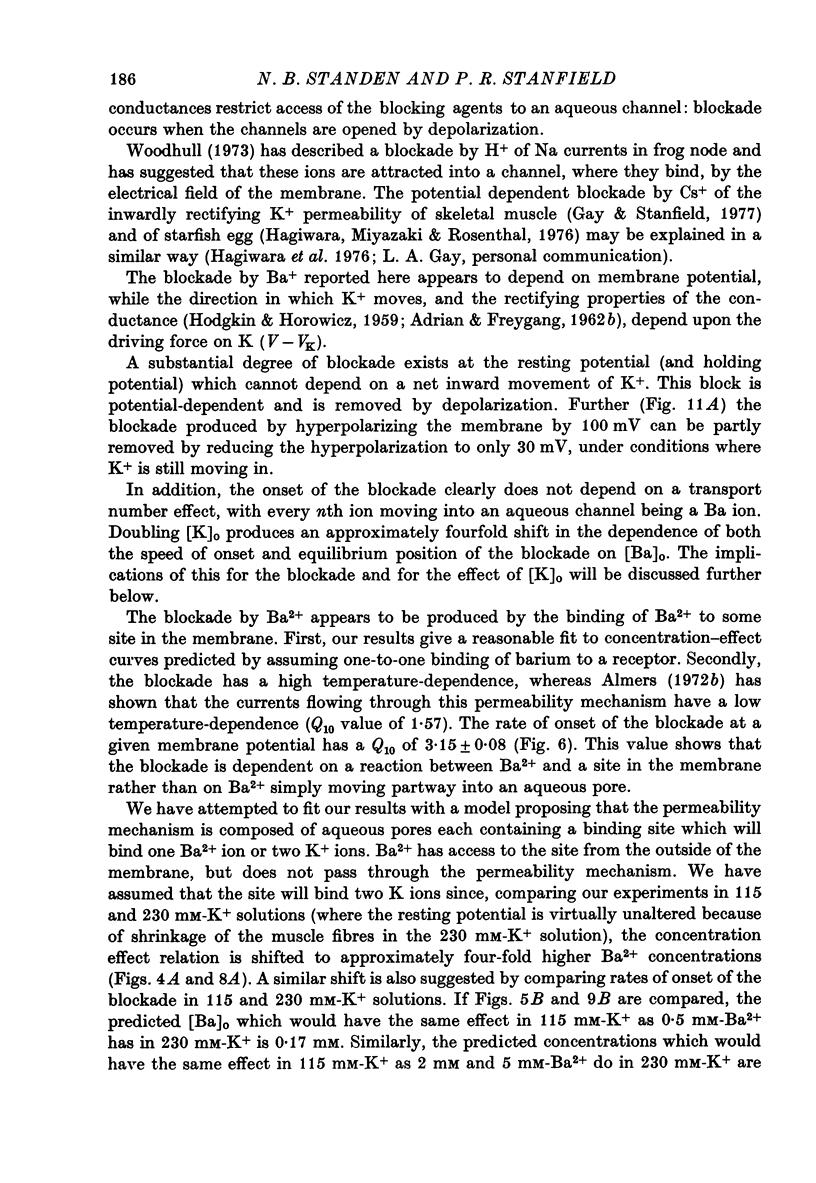
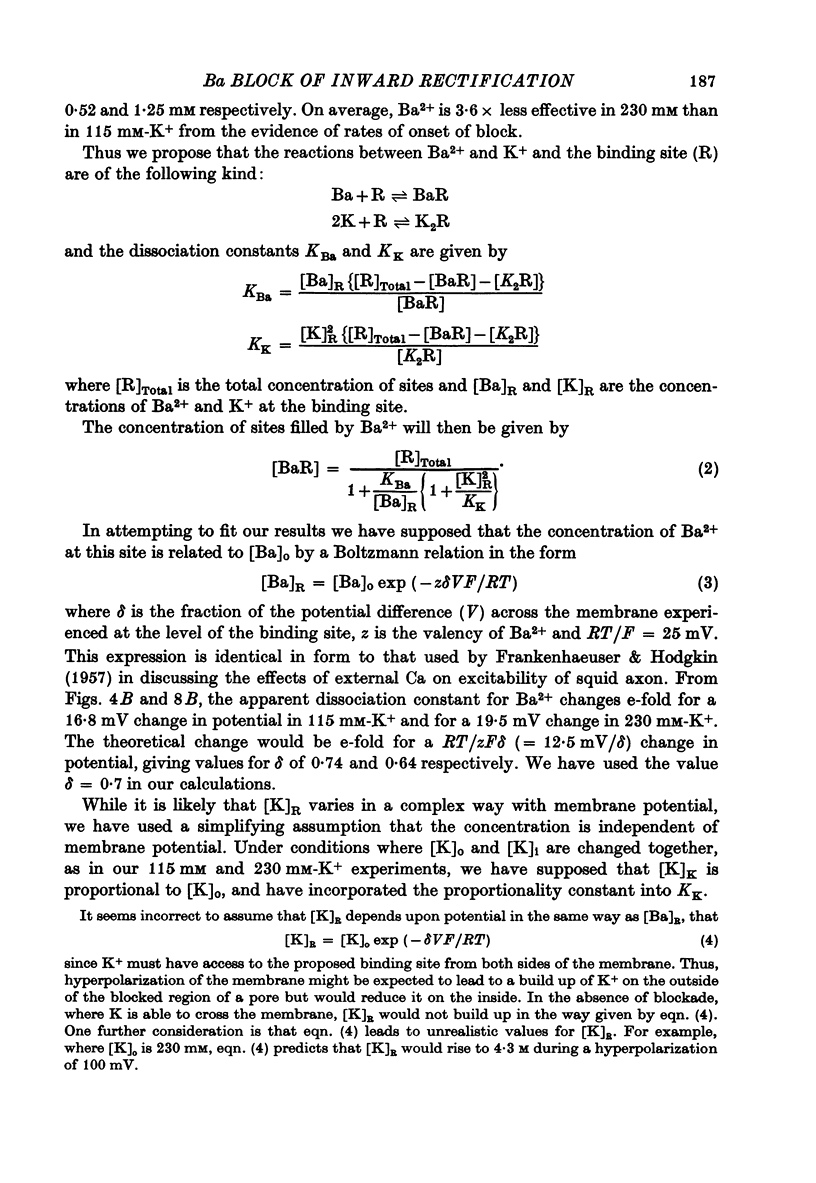
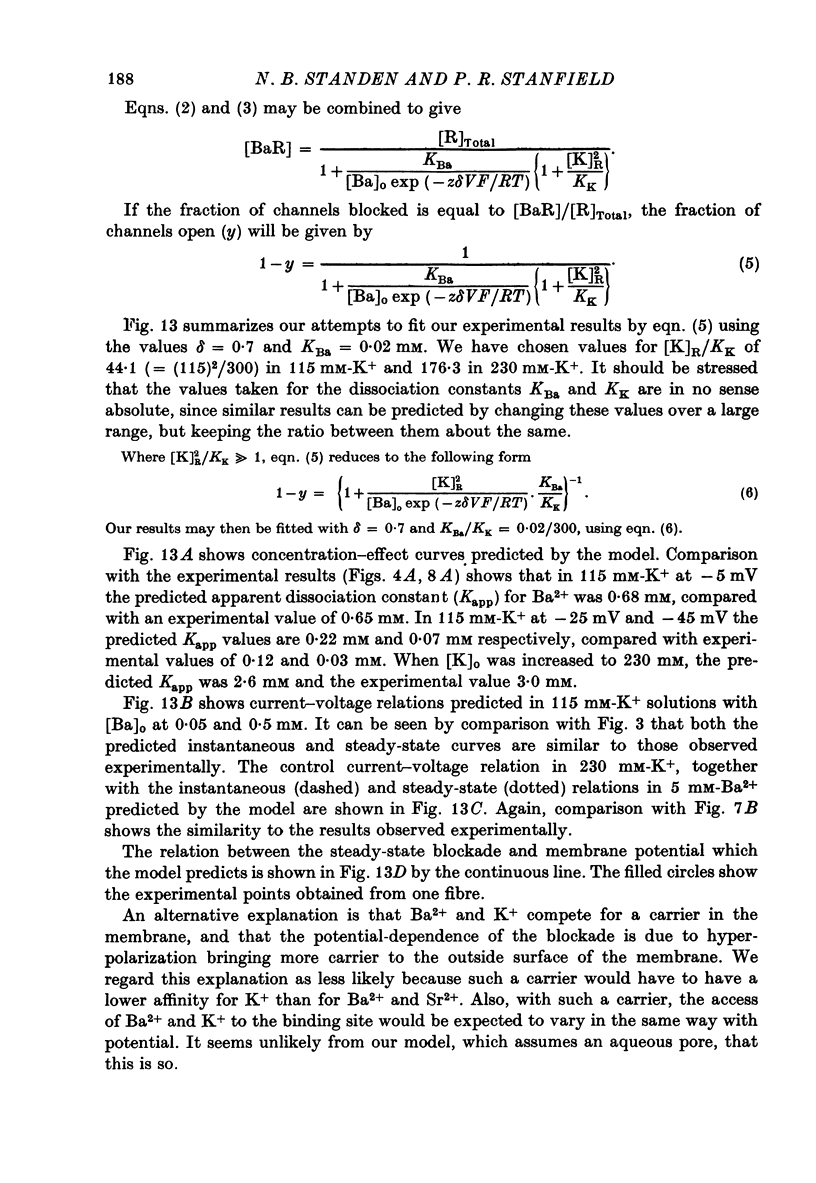
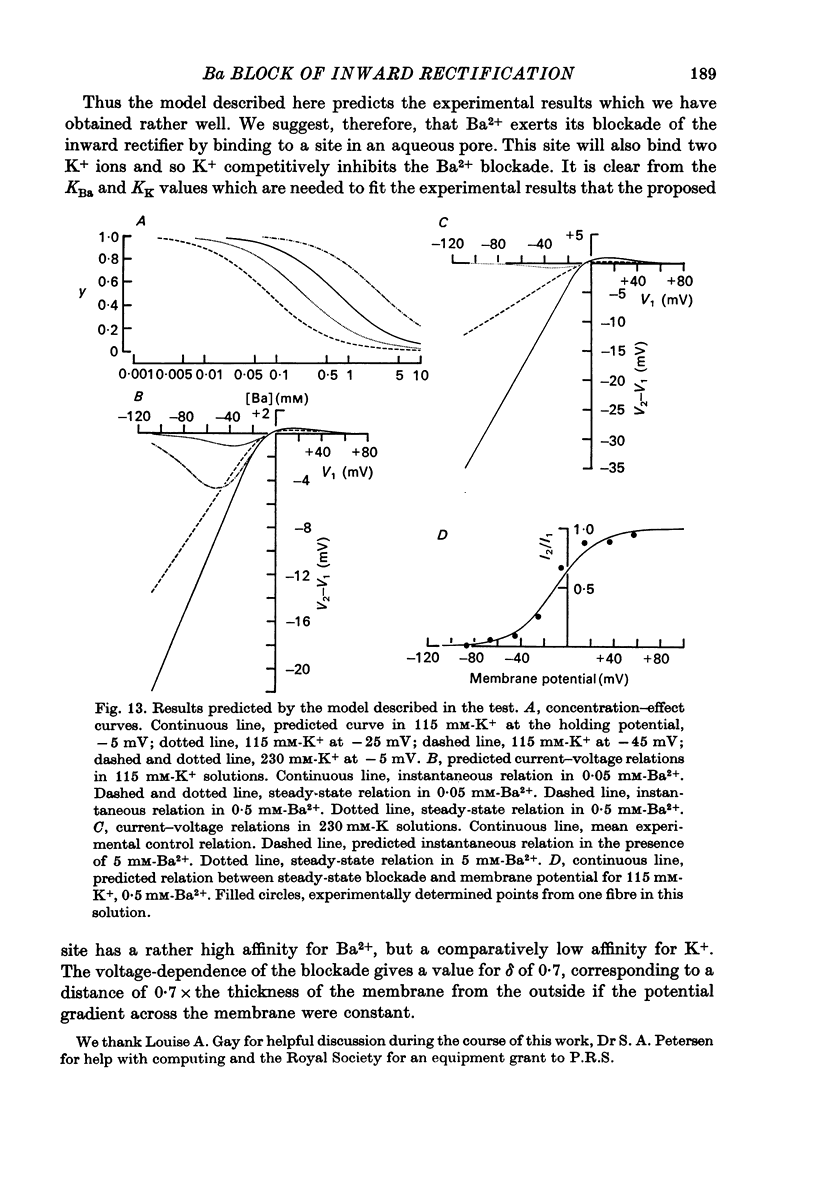
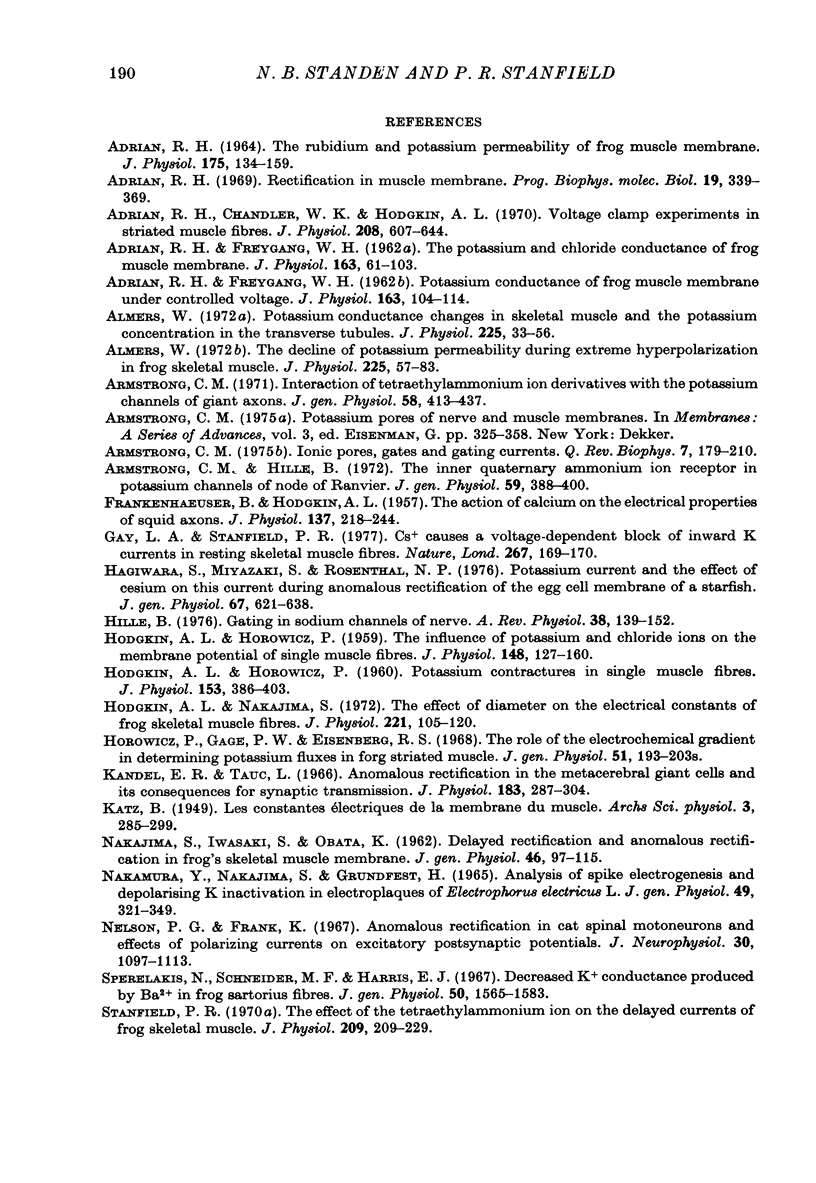
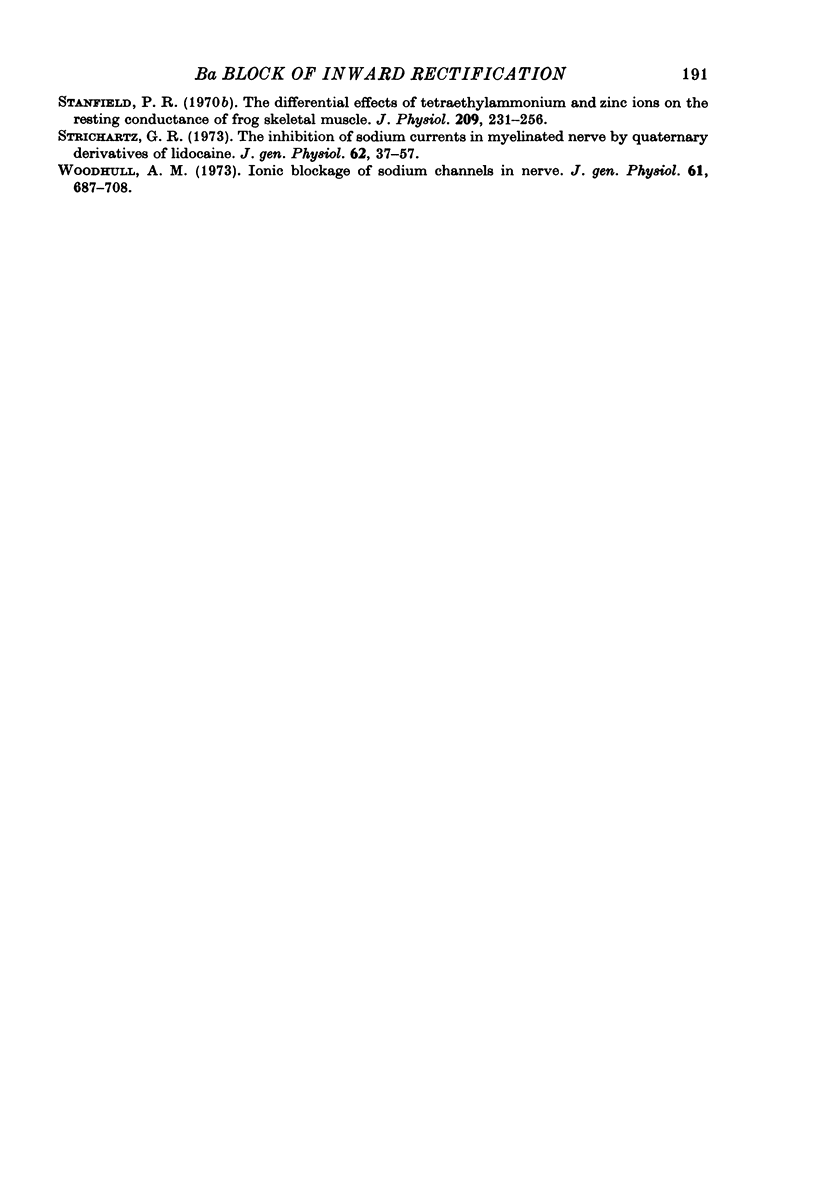
Selected References
These references are in PubMed. This may not be the complete list of references from this article.
- ADRIAN R. H., FREYGANG W. H. Potassium conductance of frog muscle membrane under controlled voltage. J Physiol. 1962 Aug;163:104–114. doi: 10.1113/jphysiol.1962.sp006960. [DOI] [PMC free article] [PubMed] [Google Scholar]
- ADRIAN R. H. THE RUBIDIUM AND POTASSIUM PERMEABILITY OF FROG MUSCLE MEMBRANE. J Physiol. 1964 Dec;175:134–159. doi: 10.1113/jphysiol.1964.sp007508. [DOI] [PMC free article] [PubMed] [Google Scholar]
- Adrian R. H., Chandler W. K., Hodgkin A. L. Voltage clamp experiments in striated muscle fibres. J Physiol. 1970 Jul;208(3):607–644. doi: 10.1113/jphysiol.1970.sp009139. [DOI] [PMC free article] [PubMed] [Google Scholar]
- Adrian R. H., Freygang W. H. The potassium and chloride conductance of frog muscle membrane. J Physiol. 1962 Aug;163(1):61–103. doi: 10.1113/jphysiol.1962.sp006959. [DOI] [PMC free article] [PubMed] [Google Scholar]
- Adrian R. H. Rectification in muscle membrane. Prog Biophys Mol Biol. 1969;19(2):339–369. [PubMed] [Google Scholar]
- Almers W. Potassium conductance changes in skeletal muscle and the potassium concentration in the transverse tubules. J Physiol. 1972 Aug;225(1):33–56. doi: 10.1113/jphysiol.1972.sp009928. [DOI] [PMC free article] [PubMed] [Google Scholar]
- Armstrong C. M., Hille B. The inner quaternary ammonium ion receptor in potassium channels of the node of Ranvier. J Gen Physiol. 1972 Apr;59(4):388–400. doi: 10.1085/jgp.59.4.388. [DOI] [PMC free article] [PubMed] [Google Scholar]
- Armstrong C. M. Interaction of tetraethylammonium ion derivatives with the potassium channels of giant axons. J Gen Physiol. 1971 Oct;58(4):413–437. doi: 10.1085/jgp.58.4.413. [DOI] [PMC free article] [PubMed] [Google Scholar]
- Armstrong C. M. Ionic pores, gates, and gating currents. Q Rev Biophys. 1974 May;7(2):179–210. doi: 10.1017/s0033583500001402. [DOI] [PubMed] [Google Scholar]
- Armstrong C. M. Potassium pores of nerve and muscle membranes. Membranes. 1975;3:325–358. [PubMed] [Google Scholar]
- FRANKENHAEUSER B., HODGKIN A. L. The action of calcium on the electrical properties of squid axons. J Physiol. 1957 Jul 11;137(2):218–244. doi: 10.1113/jphysiol.1957.sp005808. [DOI] [PMC free article] [PubMed] [Google Scholar]
- Gay L. A., Stanfield P. R. Cs(+) causes a voltage-dependent block of inward K currents in resting skeletal muscle fibres. Nature. 1977 May 12;267(5607):169–170. doi: 10.1038/267169a0. [DOI] [PubMed] [Google Scholar]
- HODGKIN A. L., HOROWICZ P. Potassium contractures in single muscle fibres. J Physiol. 1960 Sep;153:386–403. doi: 10.1113/jphysiol.1960.sp006541. [DOI] [PMC free article] [PubMed] [Google Scholar]
- HODGKIN A. L., HOROWICZ P. The influence of potassium and chloride ions on the membrane potential of single muscle fibres. J Physiol. 1959 Oct;148:127–160. doi: 10.1113/jphysiol.1959.sp006278. [DOI] [PMC free article] [PubMed] [Google Scholar]
- Hagiwara S., Miyazaki S., Rosenthal N. P. Potassium current and the effect of cesium on this current during anomalous rectification of the egg cell membrane of a starfish. J Gen Physiol. 1976 Jun;67(6):621–638. doi: 10.1085/jgp.67.6.621. [DOI] [PMC free article] [PubMed] [Google Scholar]
- Hodgkin A. L., Nakajima S. The effect of diameter on the electrical constants of frog skeletal muscle fibres. J Physiol. 1972 Feb;221(1):105–120. doi: 10.1113/jphysiol.1972.sp009742. [DOI] [PMC free article] [PubMed] [Google Scholar]
- Horowicz P., Gage P. W., Eisenberg R. S. The role of the electrochemical gradient in determining potassium fluxes in frog striated muscle. J Gen Physiol. 1968 May 1;51(5):193–203. [PMC free article] [PubMed] [Google Scholar]
- Kandel E. R., Tauc L. Anomalous rectification in the metacerebral giant cells and its consequences for synaptic transmission. J Physiol. 1966 Mar;183(2):287–304. doi: 10.1113/jphysiol.1966.sp007867. [DOI] [PMC free article] [PubMed] [Google Scholar]
- NAKAJIMA S., IWASAKI S., OBATA K. Delayed rectification and anomalous rectification in frog's skeletal muscle membrane. J Gen Physiol. 1962 Sep;46:97–115. doi: 10.1085/jgp.46.1.97. [DOI] [PMC free article] [PubMed] [Google Scholar]
- Nakamura Y., Nakajima S., Grundfest H. Analysis of Spike Electrogenesis and Depolarizing K Inactivation in Electroplaques of Electrophorus electricus, L. J Gen Physiol. 1965 Nov 1;49(2):321–349. doi: 10.1085/jgp.49.2.321. [DOI] [PMC free article] [PubMed] [Google Scholar]
- Nelson P. G., Frank K. Anomalous rectification in cat spinal motoneurons and effect of polarizing currents on excitatory postsynaptic potential. J Neurophysiol. 1967 Sep;30(5):1097–1113. doi: 10.1152/jn.1967.30.5.1097. [DOI] [PubMed] [Google Scholar]
- Sperelakis N., Schneider M. F., Harris E. J. Decreased K+ conductance produced by Ba++ in frog sartorius fibers. J Gen Physiol. 1967 Jul;50(6):1565–1583. doi: 10.1085/jgp.50.6.1565. [DOI] [PMC free article] [PubMed] [Google Scholar]
- Stanfield P. R. The effect of the tetraethylammonium ion on the delayed currents of frog skeletal muscle. J Physiol. 1970 Jul;209(1):209–229. doi: 10.1113/jphysiol.1970.sp009163. [DOI] [PMC free article] [PubMed] [Google Scholar]
- Strichartz G. R. The inhibition of sodium currents in myelinated nerve by quaternary derivatives of lidocaine. J Gen Physiol. 1973 Jul;62(1):37–57. doi: 10.1085/jgp.62.1.37. [DOI] [PMC free article] [PubMed] [Google Scholar]
- Woodhull A. M. Ionic blockage of sodium channels in nerve. J Gen Physiol. 1973 Jun;61(6):687–708. doi: 10.1085/jgp.61.6.687. [DOI] [PMC free article] [PubMed] [Google Scholar]