Abstract
1. Reversible depletion of synaptic vesicles from frog cutaneous pectoris neuromuscular junctions was studied by application of a Ringer solution containing 115 mM-K propionate.
2. During the release of transmitter, the synaptic vesicle membrane is added to the axolemmal membrane. Under the conditions of high K+-induced release, the synaptic vesicle membrane accumulates as folds formed in the region of the axolemmal membrane between the active zones. In depleted terminals, large vesicular structures appear and the evidence shows that some of them (possibly all) are formed as axolemmal infoldings. During formation of such infoldings the active zones remain fixed in position with respect to the post-junctional membrane.
3. During recovery in normal Ringer solution, which followed 30 min depolarization in high K+ Ringer solution, spontaneous m.e.p.p.s were detected as early as 9 min after the start of the recovery period and the average time for their reappearance was 17 min.
4. At the end of a 20 min recovery period which followed K+ depolarization, small accumulations of synaptic vesicles were again found within the terminal close to the active zones. At this time coated vesicles and coated pits were seen associated with the prejunctional axolemma and its infoldings. It appears that synaptic vesicles are re-formed directly from these coated vesicles.
5. After 60 min recovery from K+ depolarization, at which time stimulation of the motor nerve induced a muscle twitch, the structure of the terminals closely resembled that of control preparations.
6. The entire synaptic vesicle recycling process can take place in the absence of the neurone soma.
Full text
PDF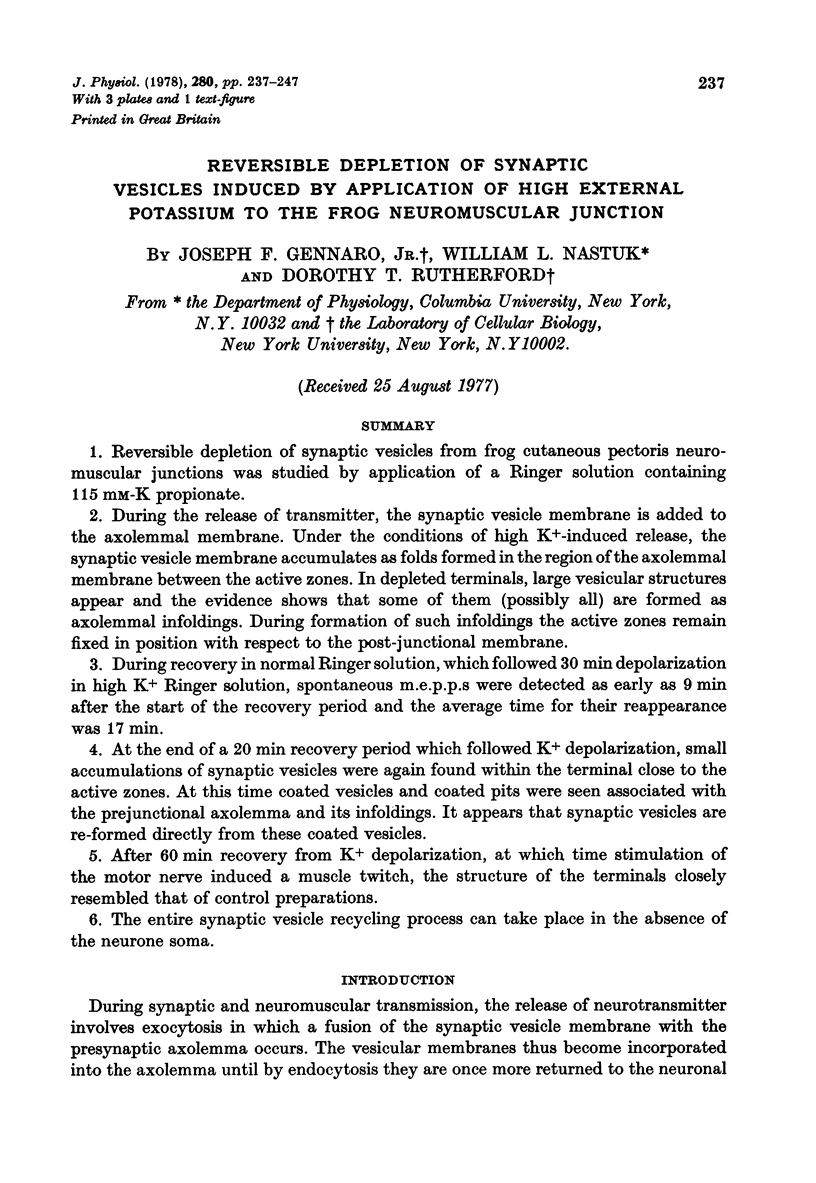
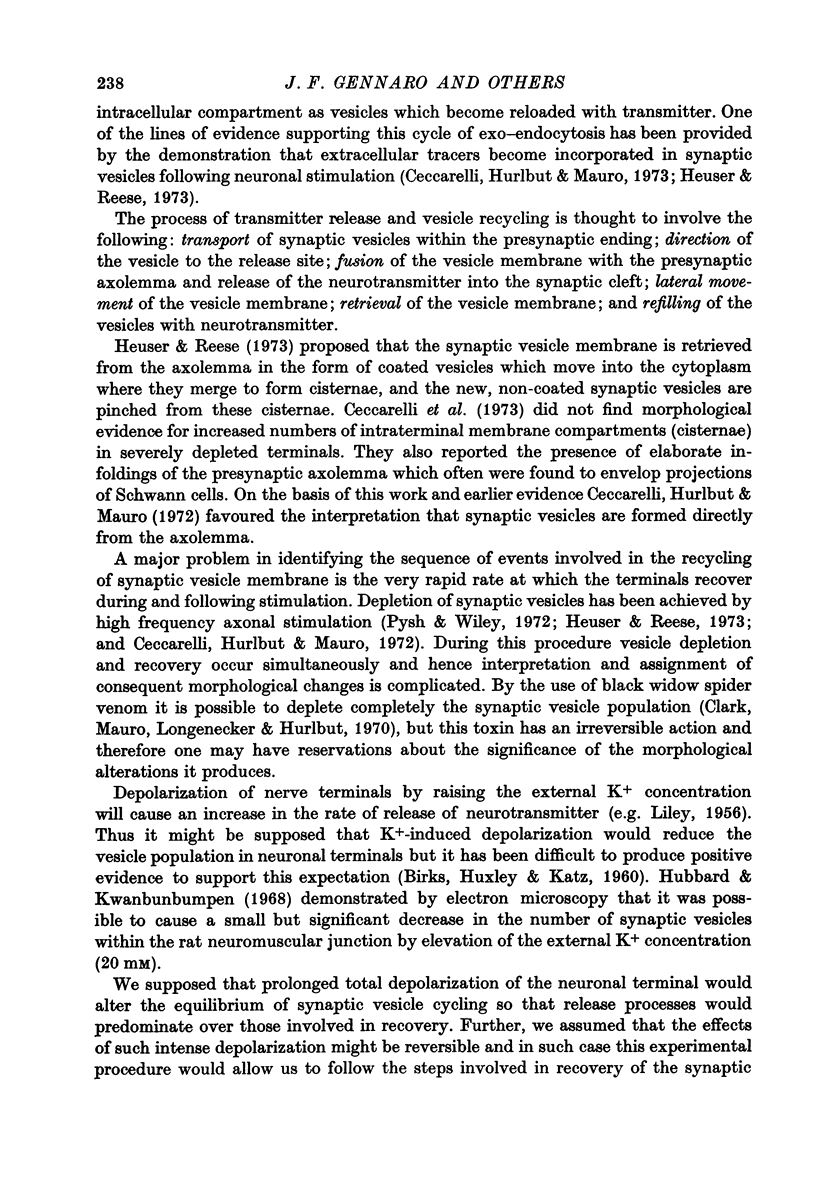
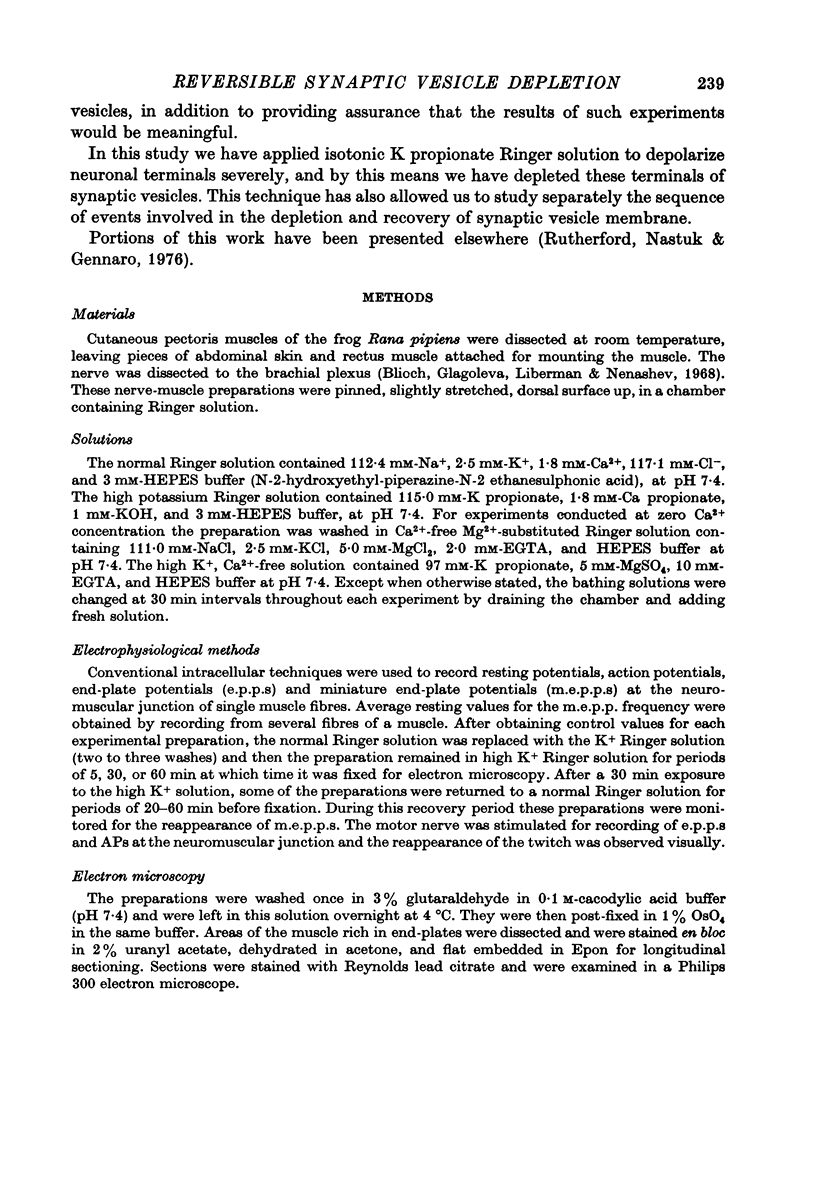
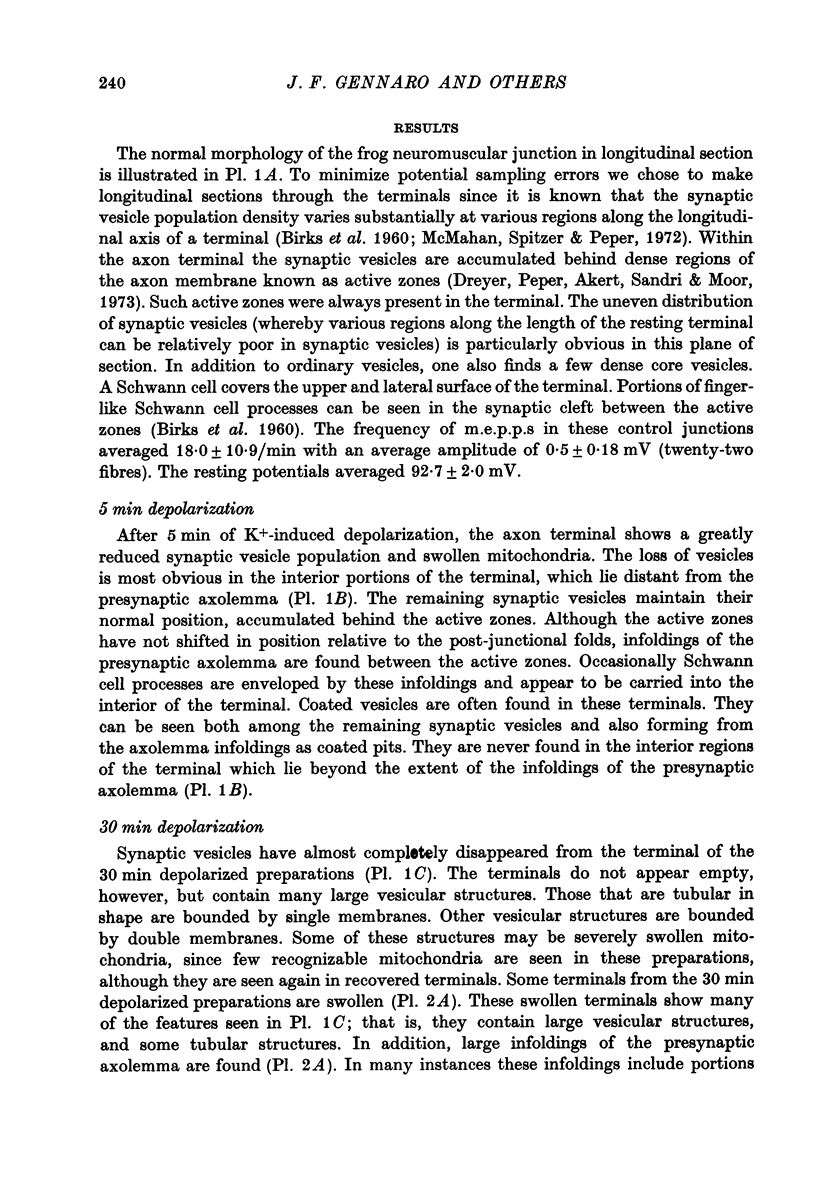
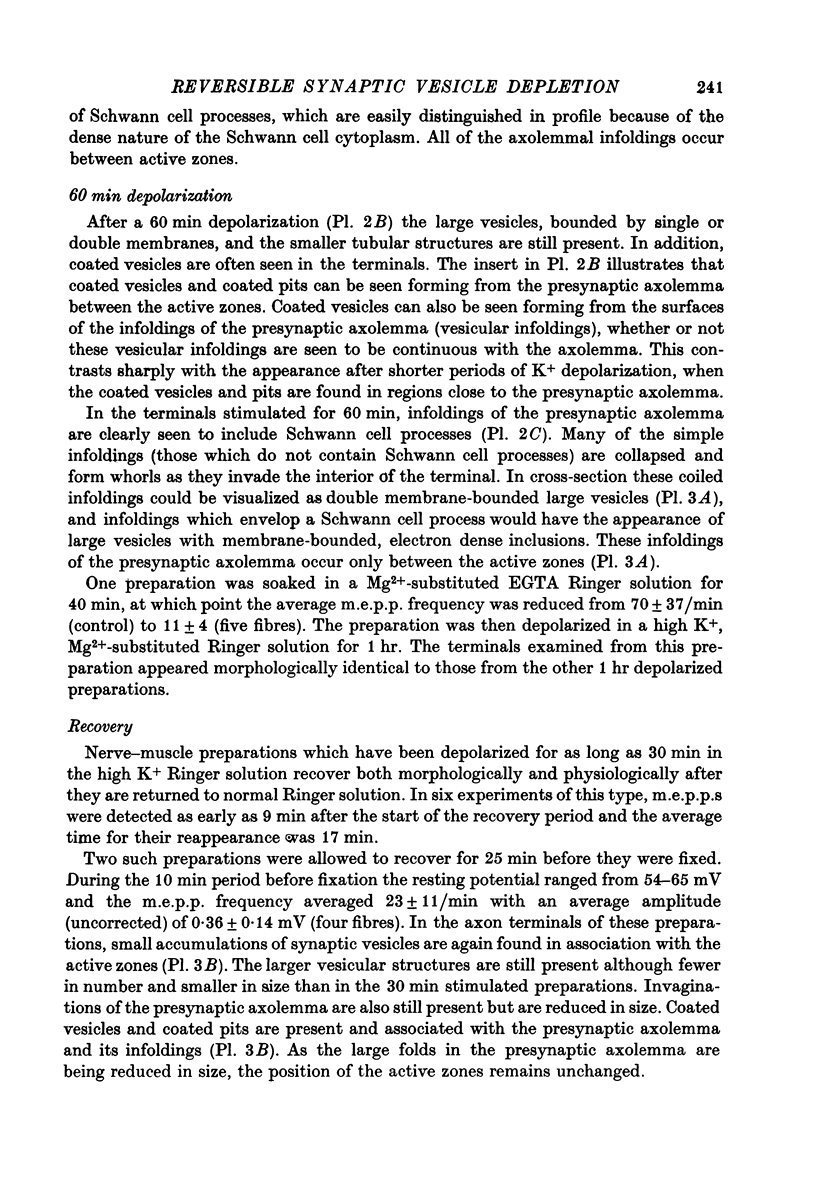
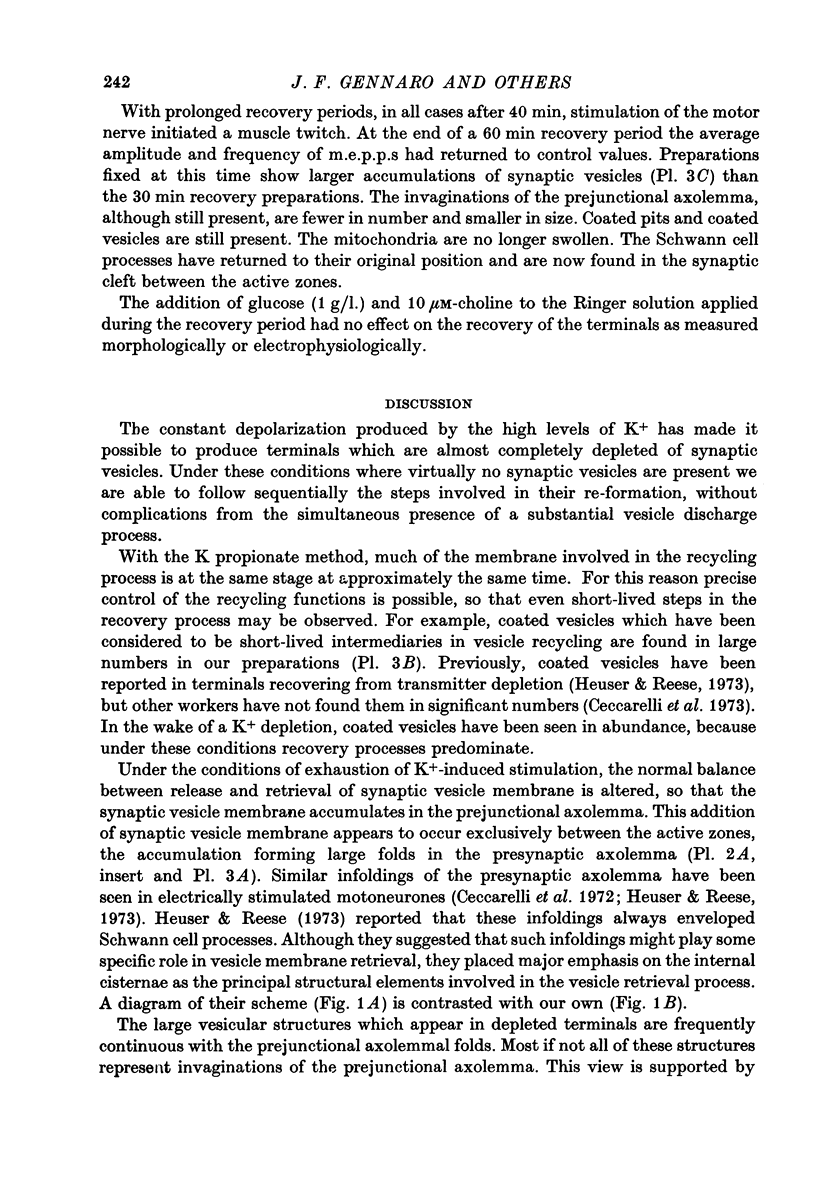
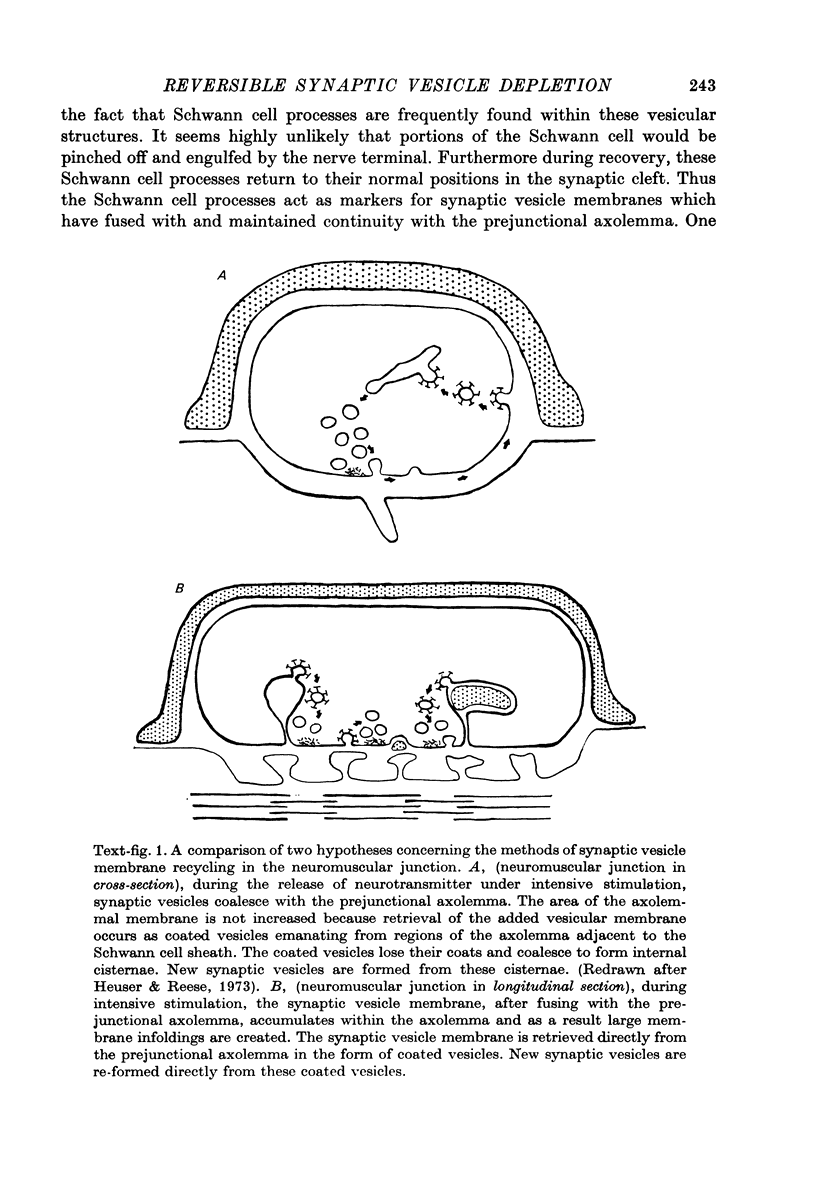
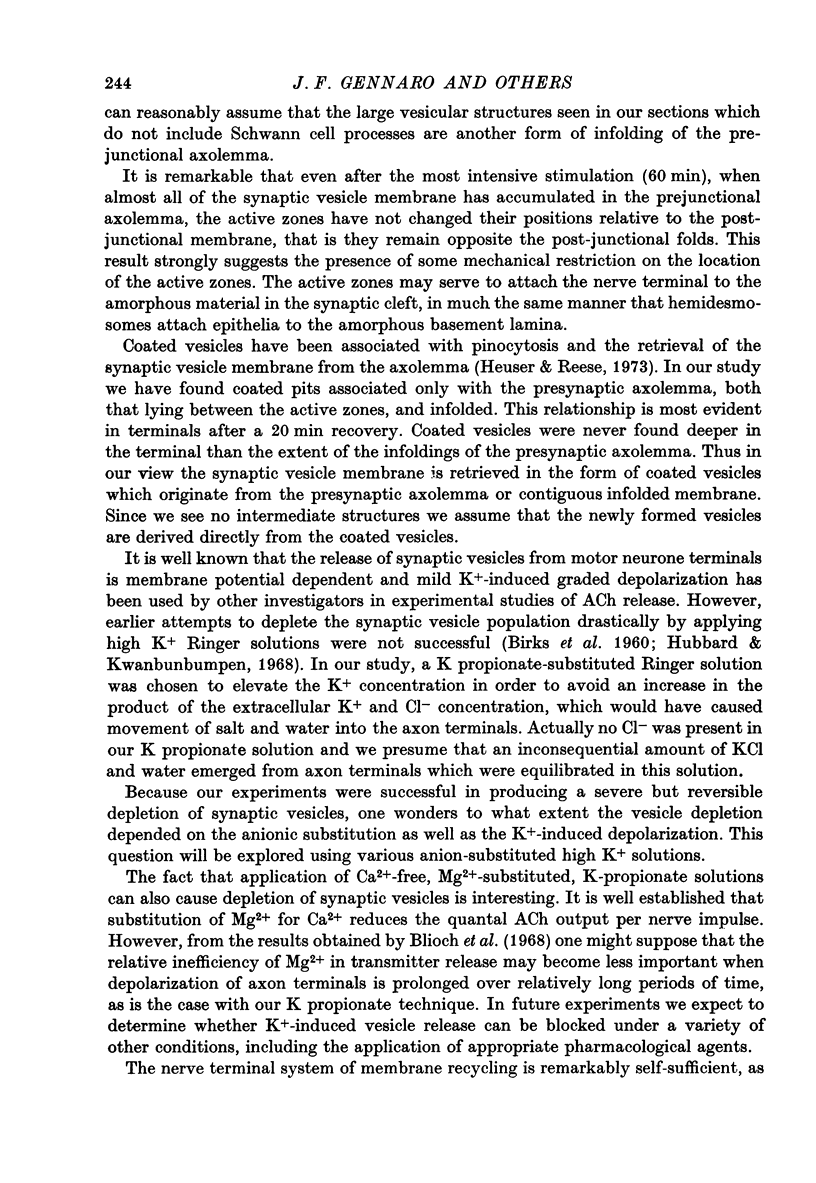
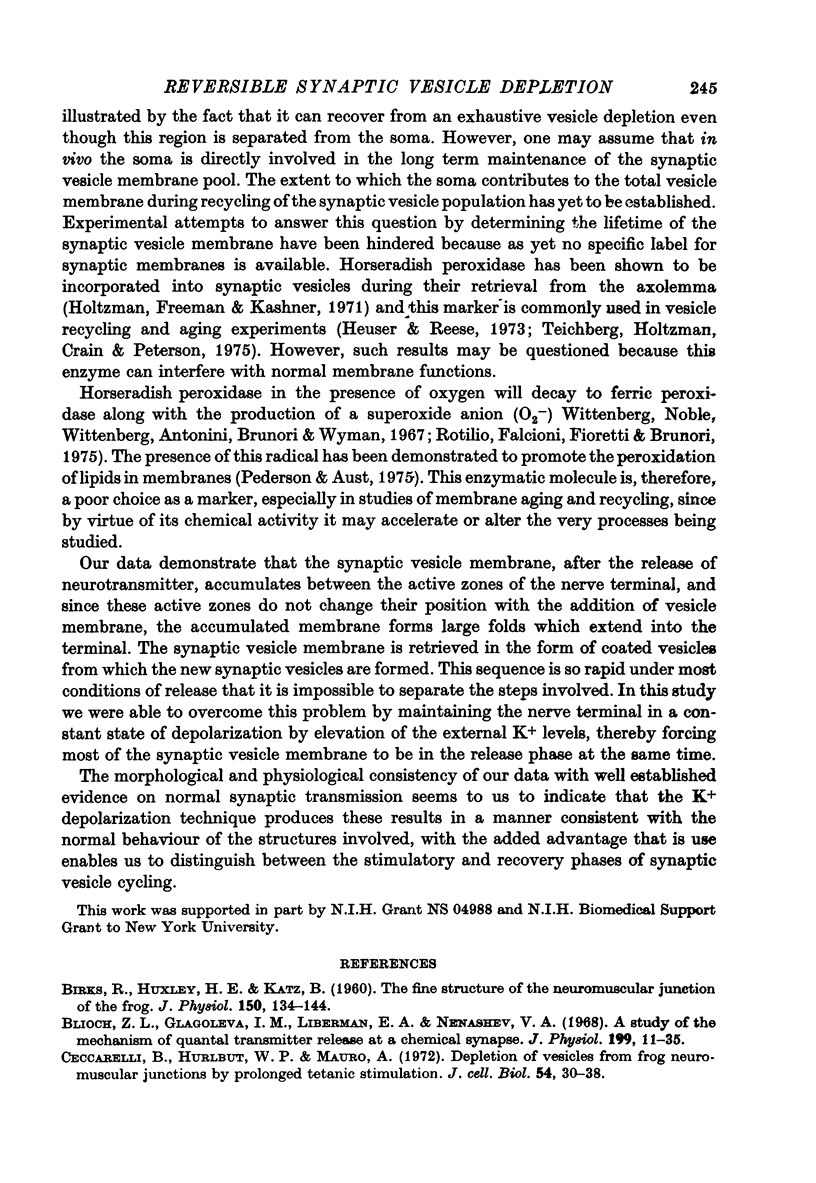
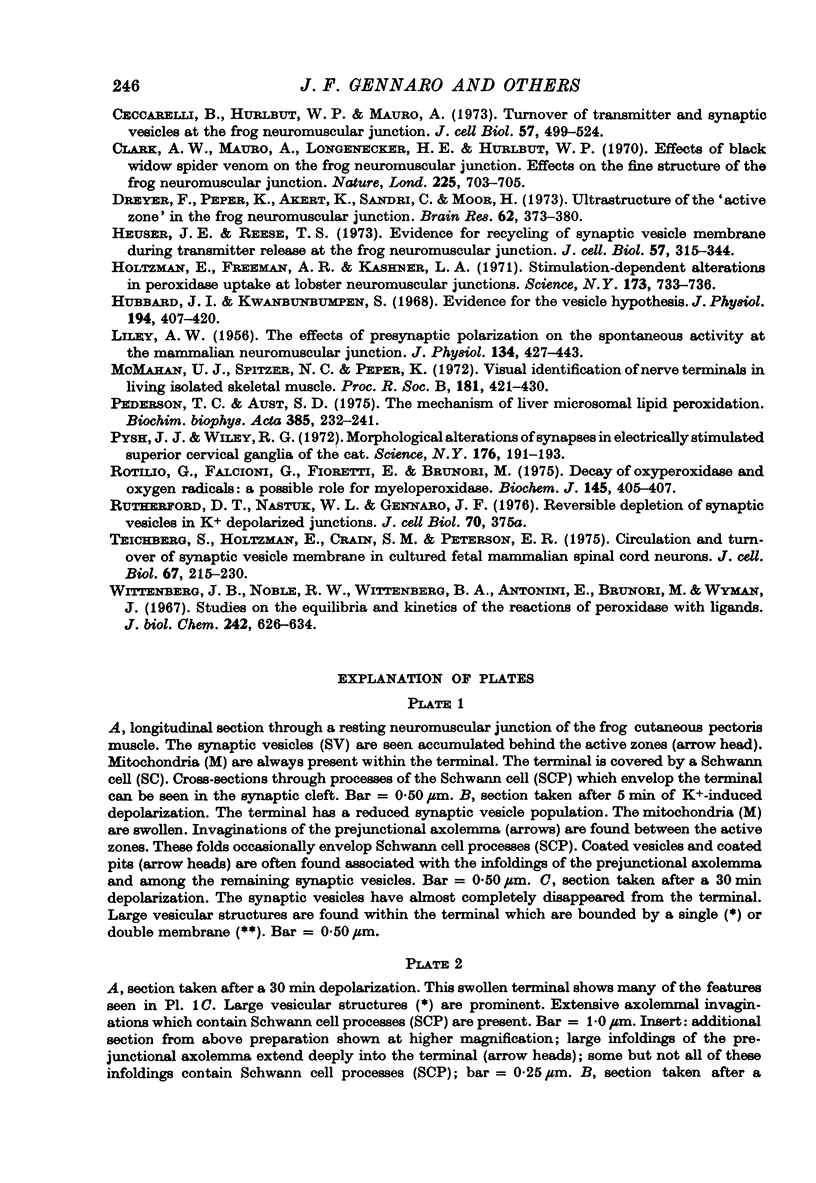
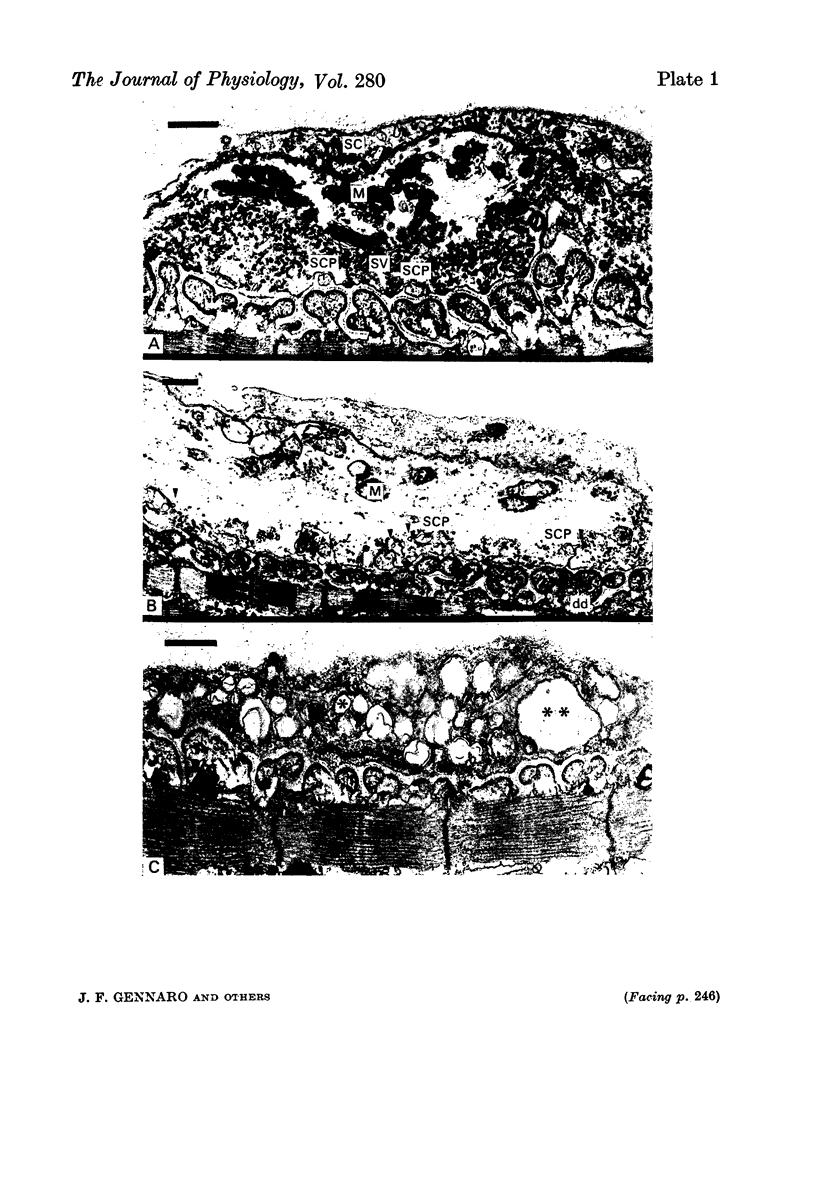
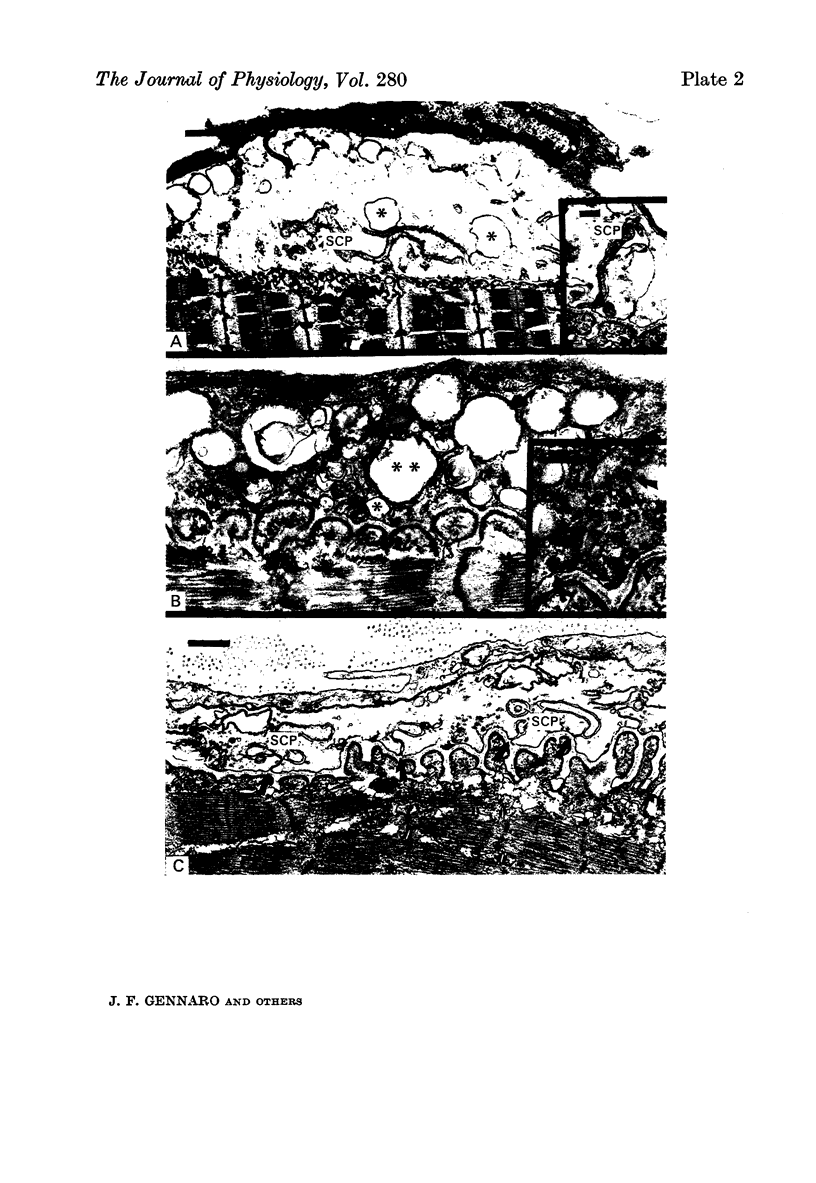
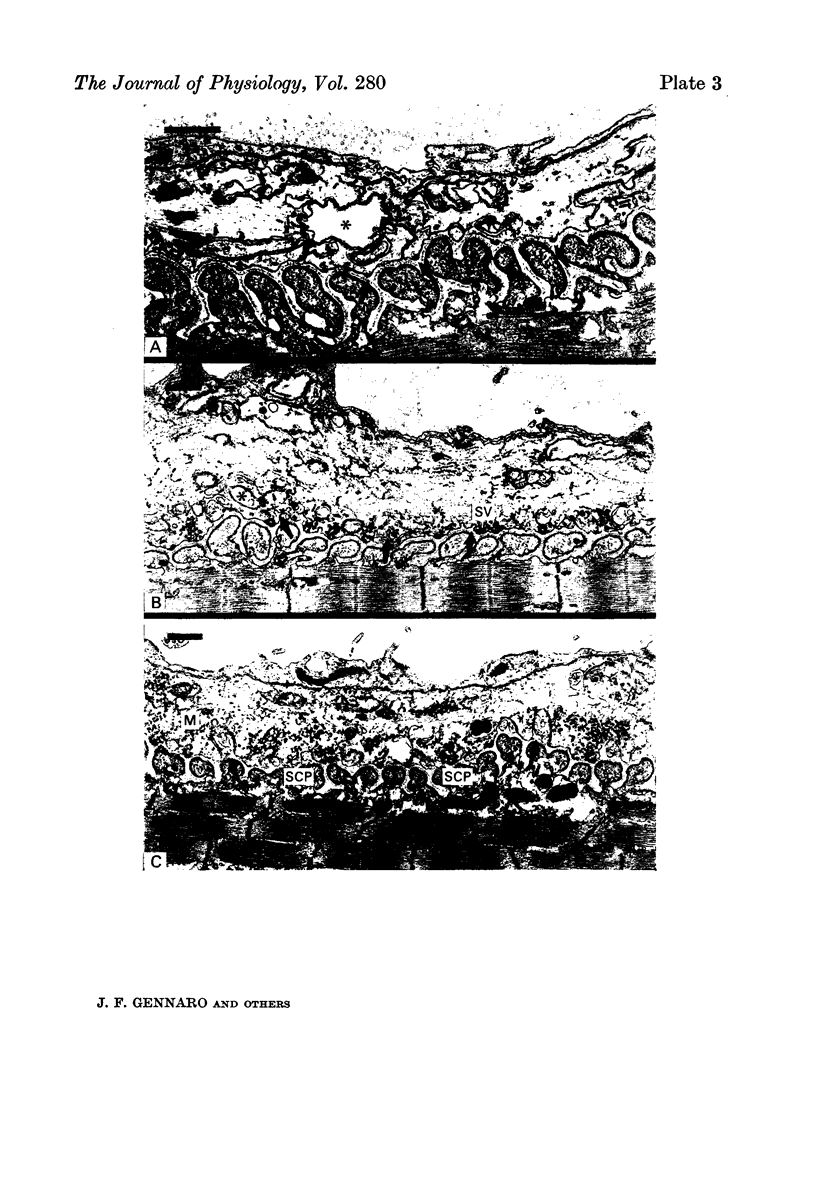
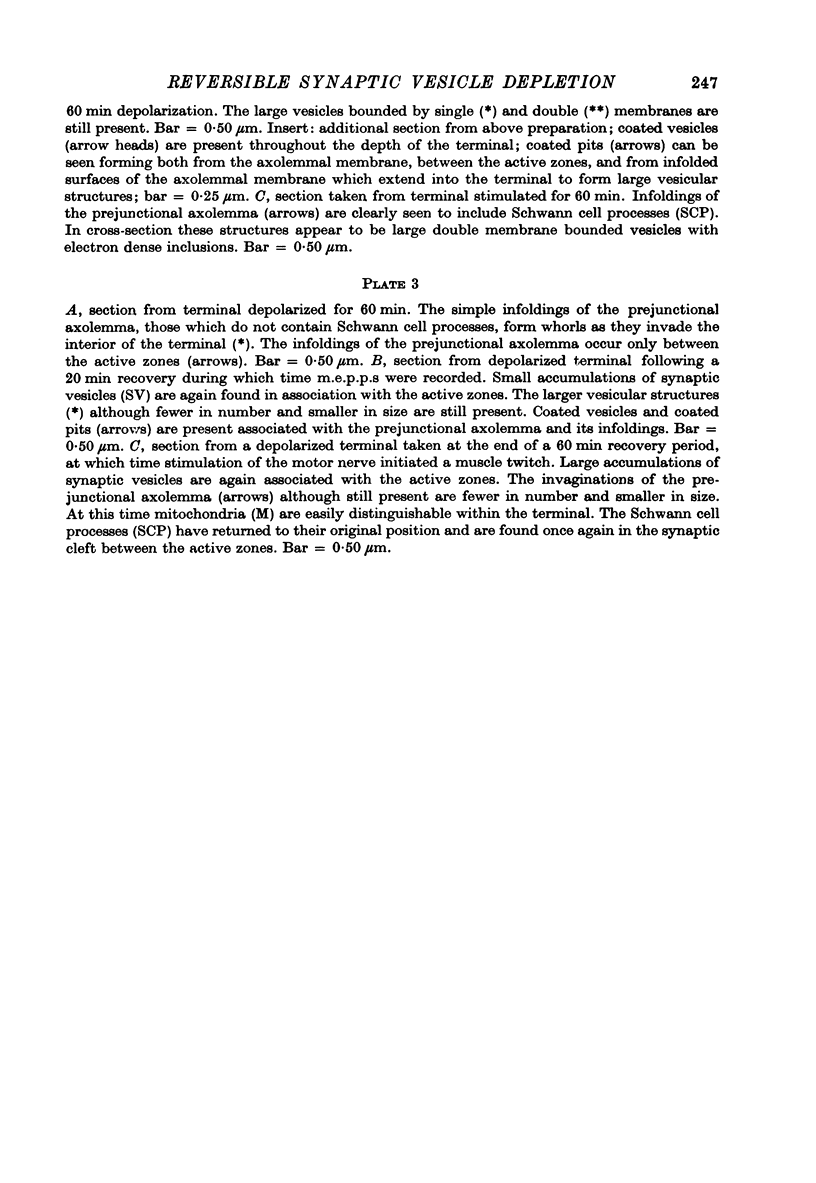
Images in this article
Selected References
These references are in PubMed. This may not be the complete list of references from this article.
- BIRKS R., HUXLEY H. E., KATZ B. The fine structure of the neuromuscular junction of the frog. J Physiol. 1960 Jan;150:134–144. doi: 10.1113/jphysiol.1960.sp006378. [DOI] [PMC free article] [PubMed] [Google Scholar]
- Blioch Z. L., Glagoleva I. M., Liberman E. A., Nenashev V. A. A study of the mechanism of quantal transmitter release at a chemical synapse. J Physiol. 1968 Nov;199(1):11–35. doi: 10.1113/jphysiol.1968.sp008637. [DOI] [PMC free article] [PubMed] [Google Scholar]
- Ceccarelli B., Hurlbut W. P., Mauro A. Depletion of vesicles from frog neuromuscular junctions by prolonged tetanic stimulation. J Cell Biol. 1972 Jul;54(1):30–38. doi: 10.1083/jcb.54.1.30. [DOI] [PMC free article] [PubMed] [Google Scholar]
- Ceccarelli B., Hurlbut W. P., Mauro A. Turnover of transmitter and synaptic vesicles at the frog neuromuscular junction. J Cell Biol. 1973 May;57(2):499–524. doi: 10.1083/jcb.57.2.499. [DOI] [PMC free article] [PubMed] [Google Scholar]
- Clark A. W., Mauro A., Longenecker H. E., Jr, Hurlbut W. P. Effects of black widow spider venom on the frog neuromuscular junction. Effects on the fine structure of the frog neuromuscular junction. Nature. 1970 Feb 21;225(5234):703–705. doi: 10.1038/225703a0. [DOI] [PubMed] [Google Scholar]
- Dreyer F., Peper K., Akert K., Sandri C., Moor H. Ultrastructure of the "active zone" in the frog neuromuscular junction. Brain Res. 1973 Nov 23;62(2):373–380. doi: 10.1016/0006-8993(73)90699-9. [DOI] [PubMed] [Google Scholar]
- Heuser J. E., Reese T. S. Evidence for recycling of synaptic vesicle membrane during transmitter release at the frog neuromuscular junction. J Cell Biol. 1973 May;57(2):315–344. doi: 10.1083/jcb.57.2.315. [DOI] [PMC free article] [PubMed] [Google Scholar]
- Holtzman E., Freeman A. R., Kashner L. A. Stimulation-dependent alterations in peroxidase uptake at lobster neuromuscular junctions. Science. 1971 Aug 20;173(3998):733–736. doi: 10.1126/science.173.3998.733. [DOI] [PubMed] [Google Scholar]
- Hubbard J. I., Kwanbunbumpen S. Evidence for the vesicle hypothesis. J Physiol. 1968 Feb;194(2):407–420. doi: 10.1113/jphysiol.1968.sp008415. [DOI] [PMC free article] [PubMed] [Google Scholar]
- LILEY A. W. The effects of presynaptic polarization on the spontaneous activity at the mammalian neuromuscular junction. J Physiol. 1956 Nov 28;134(2):427–443. doi: 10.1113/jphysiol.1956.sp005655. [DOI] [PMC free article] [PubMed] [Google Scholar]
- Pederson T. C., Aust S. D. The mechanism of liver microsomal lipid peroxidation. Biochim Biophys Acta. 1975 Apr 7;385(2):232–241. doi: 10.1016/0304-4165(75)90351-7. [DOI] [PubMed] [Google Scholar]
- Pysh J. J., Wiley R. G. Morphologic alterations of synapses in electrically stimulated superior cervical ganglia of the cat. Science. 1972 Apr 14;176(4031):191–193. doi: 10.1126/science.176.4031.191. [DOI] [PubMed] [Google Scholar]
- Rotilio G., Falcioni G., Fioretti E., Brunoir M. Decay of oxyperoxidase and oxygen radicals; a possible role for myeloperoxidase. Biochem J. 1975 Feb;145(2):405–407. doi: 10.1042/bj1450405. [DOI] [PMC free article] [PubMed] [Google Scholar]
- Teichberg S., Holtzman E., Crain S. M., Peterson E. R. Circulation and turnover of synaptic vesicle membrane in cultured fetal mammalian spinal cord neurons. J Cell Biol. 1975 Oct;67(1):215–230. doi: 10.1083/jcb.67.1.215. [DOI] [PMC free article] [PubMed] [Google Scholar]
- Wittenberg J. B., Noble R. W., Wittenberg B. A., Antonini E., Brunori M., Wyman J. Studies on the equilibria and kinetics of the reactions of peroxidase with ligands. II. The reaction of ferroperoxidase with oxygen. J Biol Chem. 1967 Feb 25;242(4):626–634. [PubMed] [Google Scholar]