Abstract
1. Intracellular recordings were made from inner hair cells in the first turn of the guinea-pig cochlea, the recording sites being confirmed by the injection of Procion yellow dye and subsequent histology. 2. The receptor potential, in response to a pure tone burst, consisted of an AC response which followed the wave form of the stimulus and was analogous to the extracellularly recorded cochlear microphonic and a depolarizating DC response which followed the envelope of the tone burst and was analogous to the extracellularly recorded summating potential. 3. The DC response was broadly tuned at high sound pressure having a maximal amplitude of 27 mV at a sound pressure level of ca. 100 db; however the bandwidth of the response was reduced at lower sound pressure level. Isoamplitude curves for the DC response were indistinguishable from the threshold curves for auditory nerve fibres. 4. The AC response was tuned in a similar fashion to the DC response except that it was attenuated at 6-9 db/octave with respect to the DC response. It is suggested that this difference was due to the effect of membrane capacitance and resistance on the AC response. In contrast the extracellularly recorded AC component was not subject to this attenuation. 5. The total resistance and capacitance in three cells were found to be 46-61 Momega and 7.8-15.8 muF respectively. 6. Intracellular resistance changes were measured during sound stimulation, the resistance change being proportional to the DC receptor potential, indicating constant current flow through the hair cell. The current varied between 0.37 and 0.81 nA between cells. The time constant for seven cells was found to lie between 0.31 and 0.76 msec. 7. A map of the basilar membrane showing position of hair cells against characteristic frequency corresponded to the cut-off frequencies of the basilar membrane mechanical measurements and the innervation sites of spiral ganglion cells.
Full text
PDF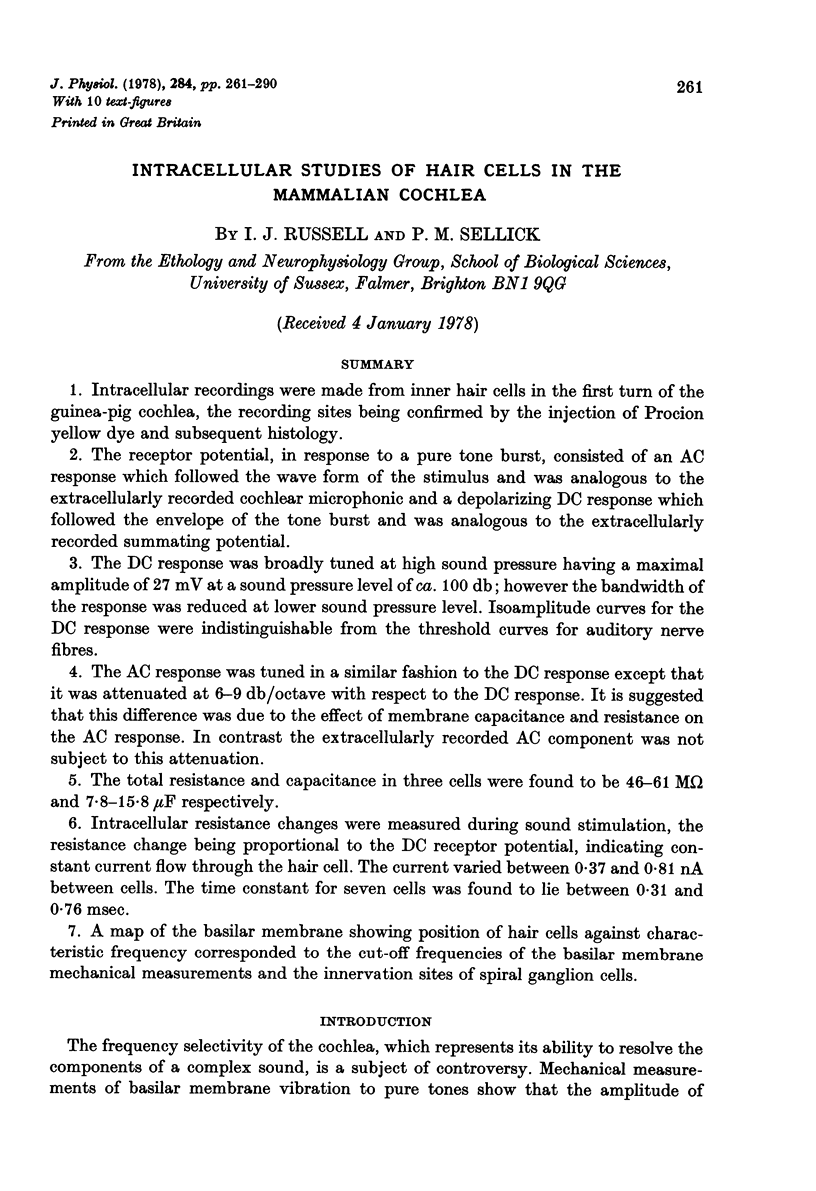
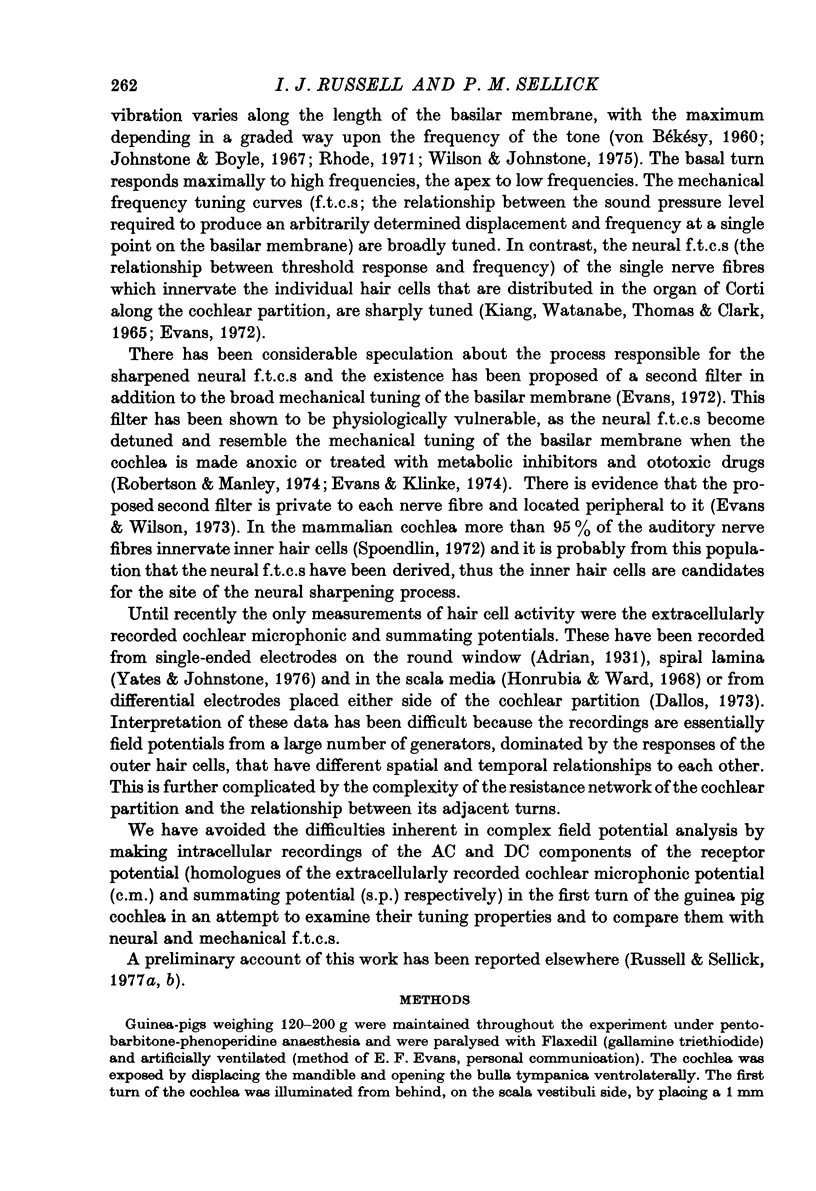
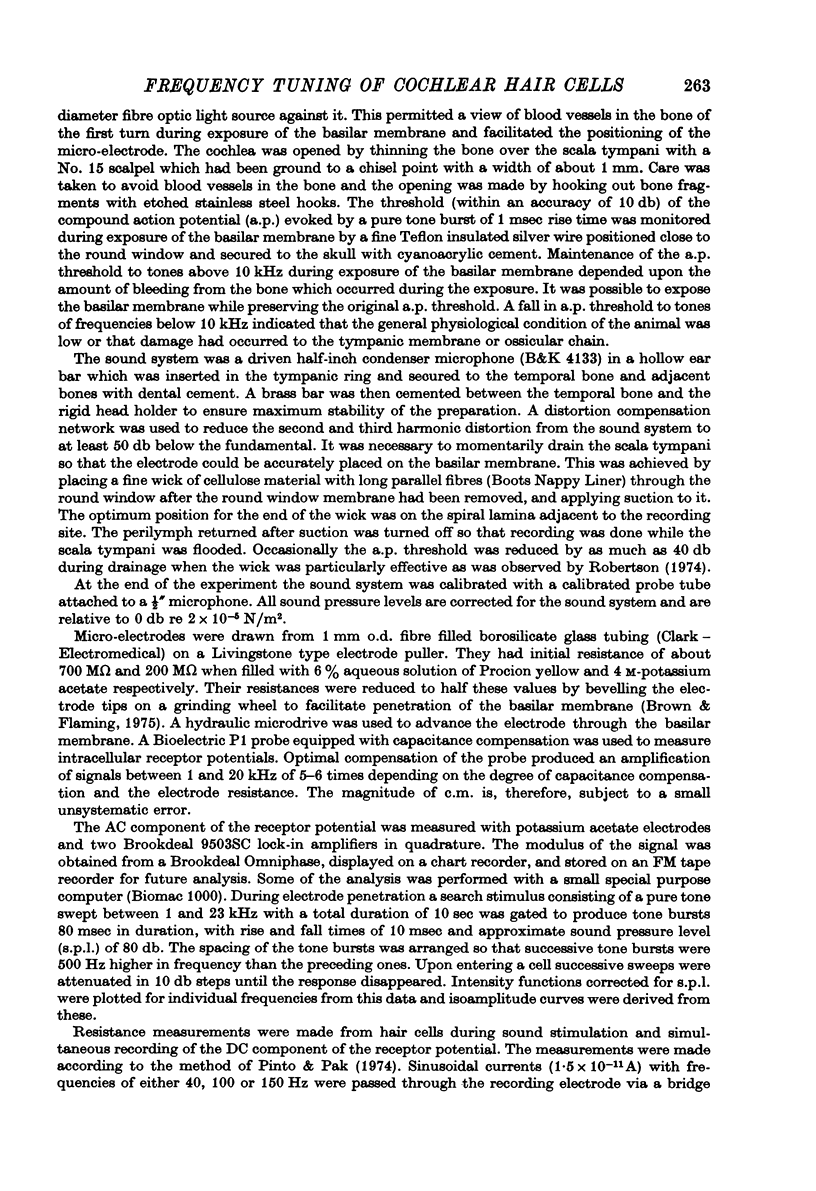
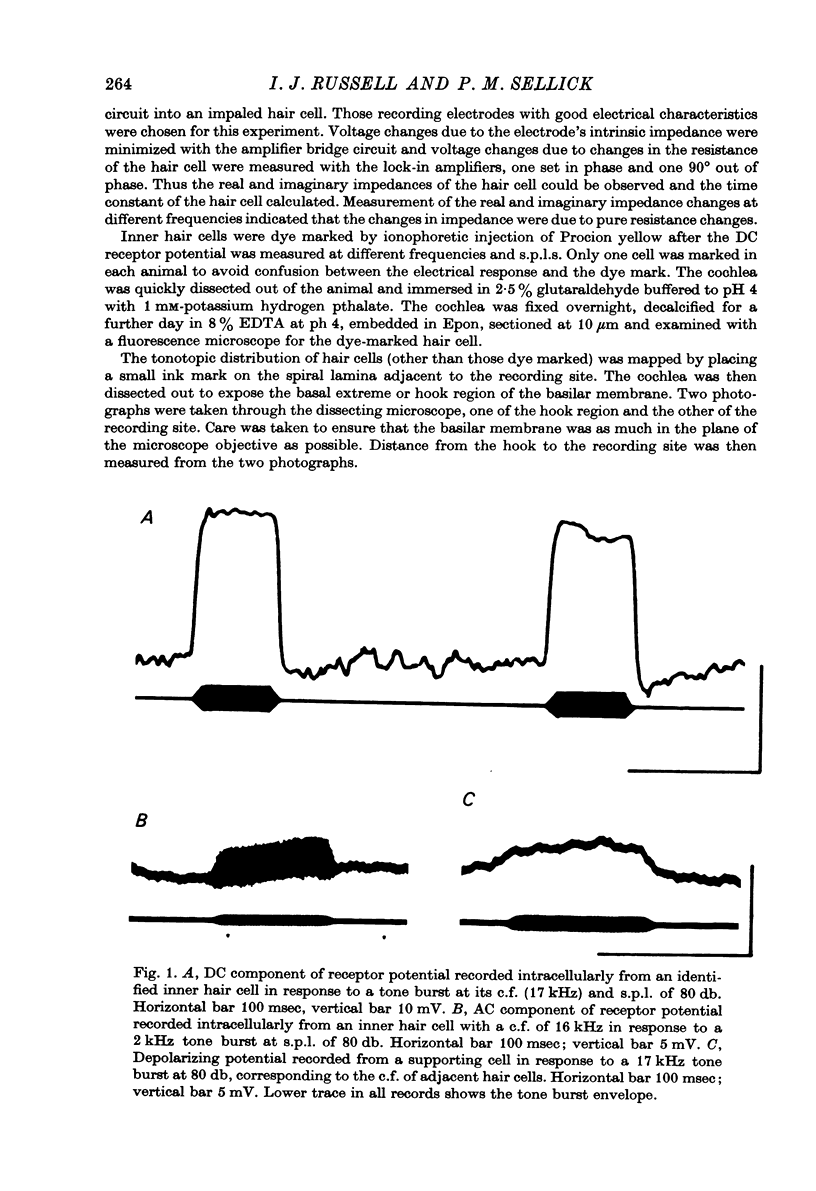

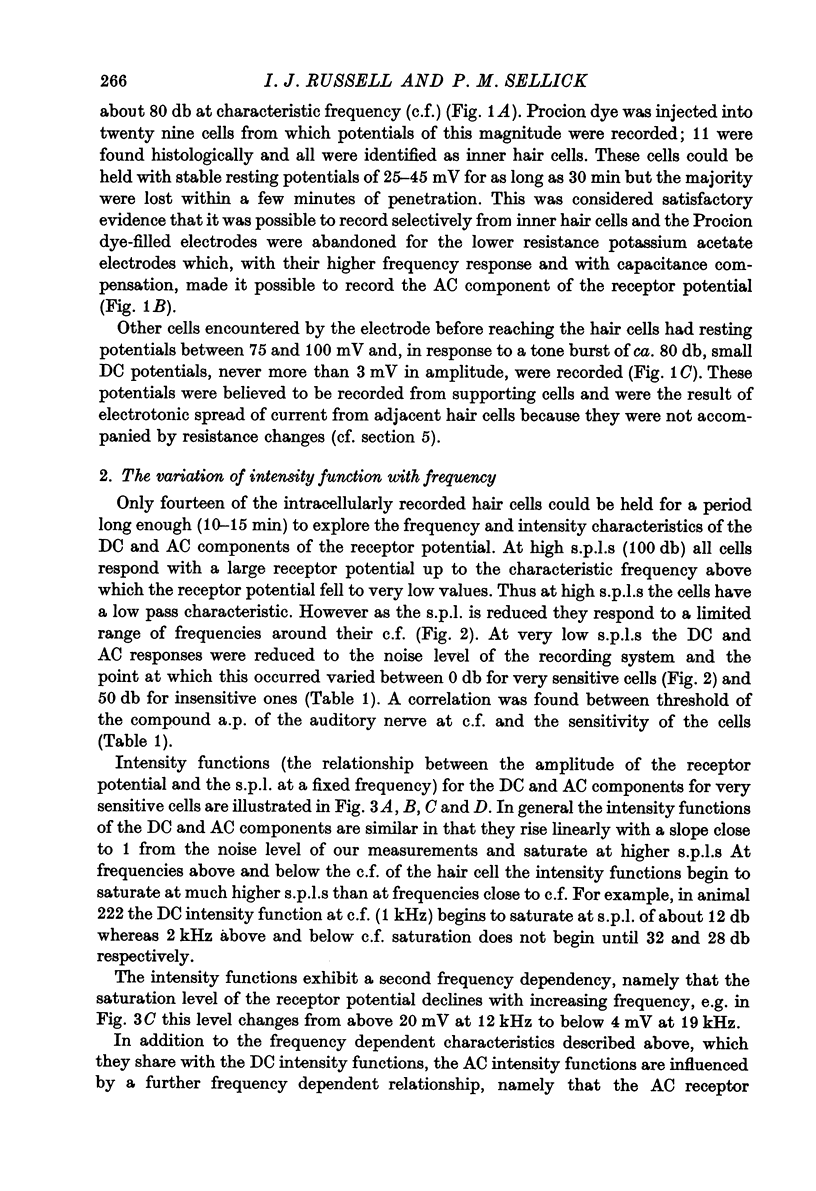
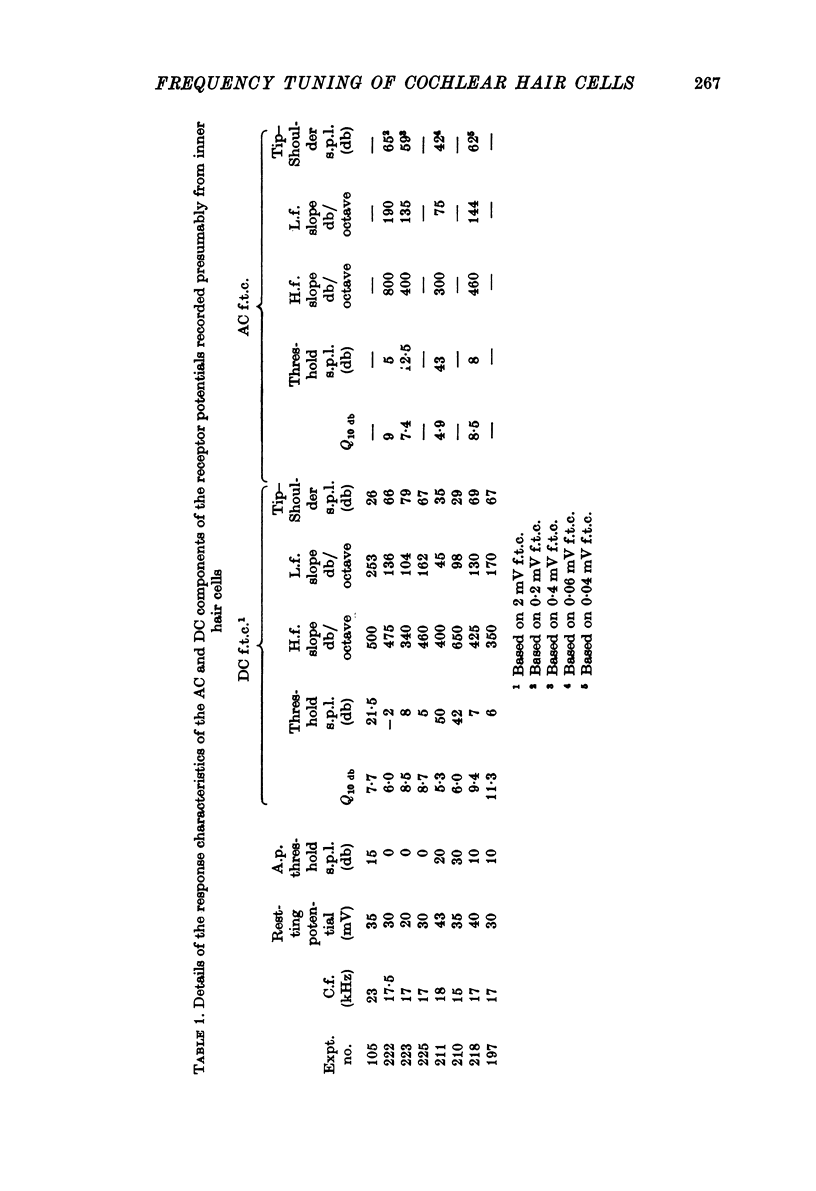
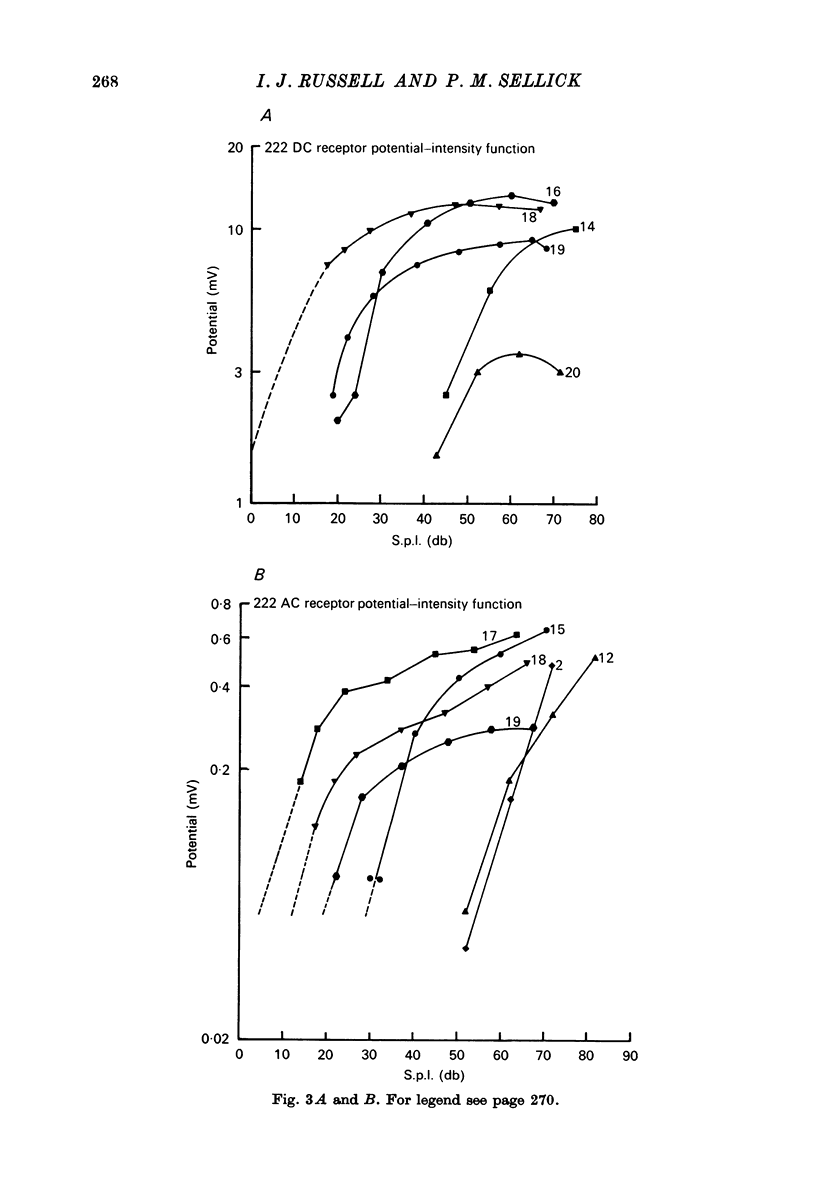
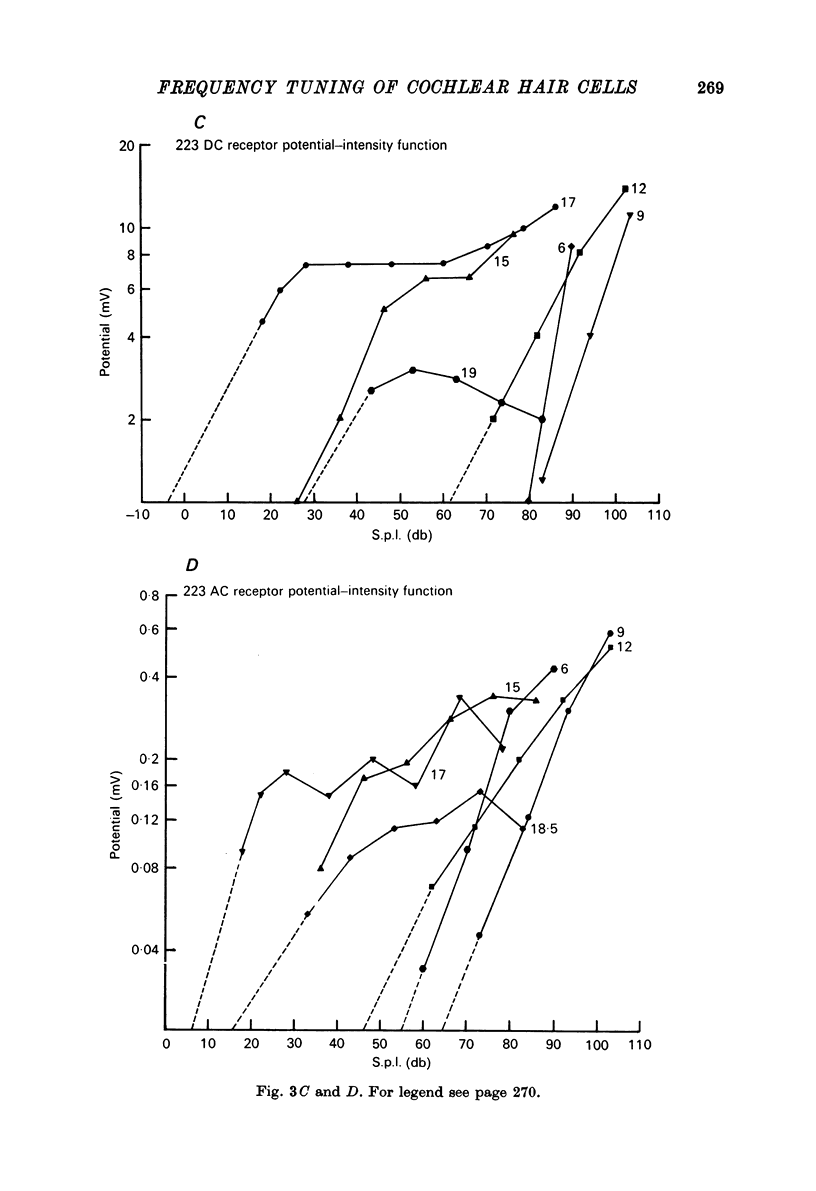
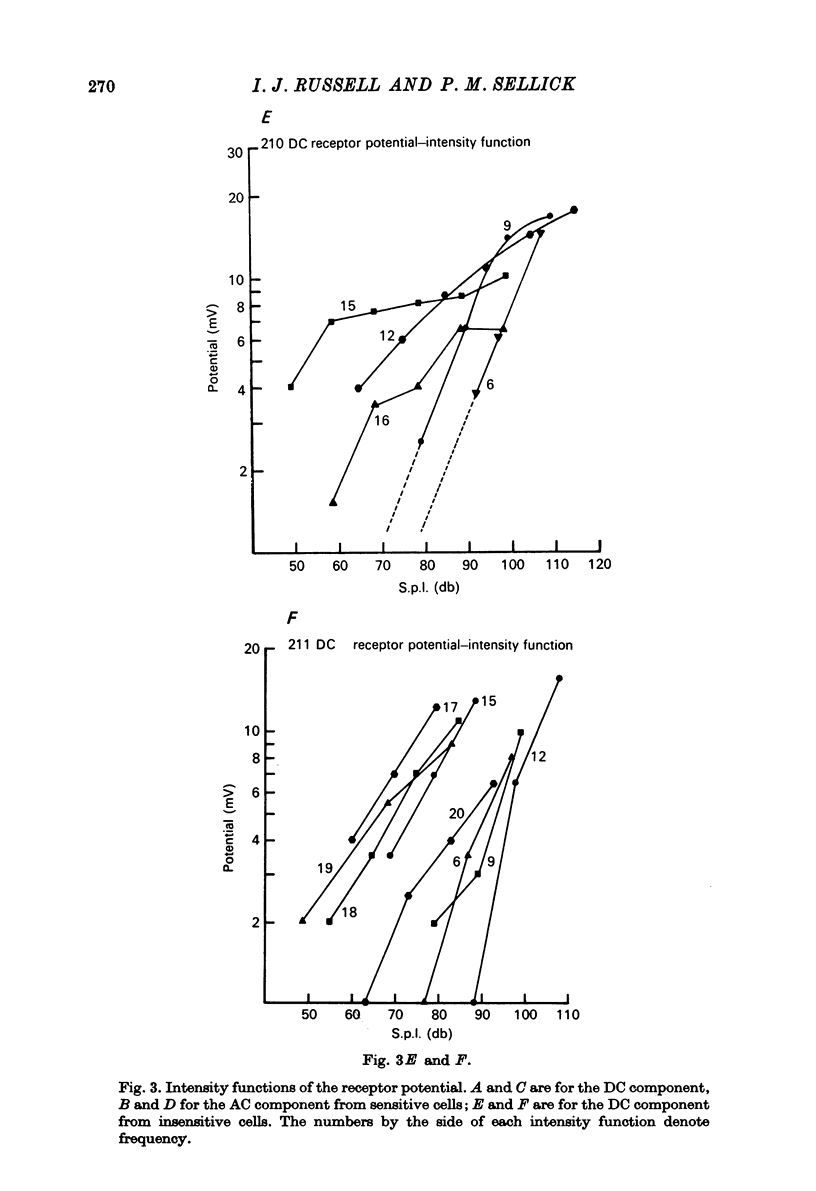
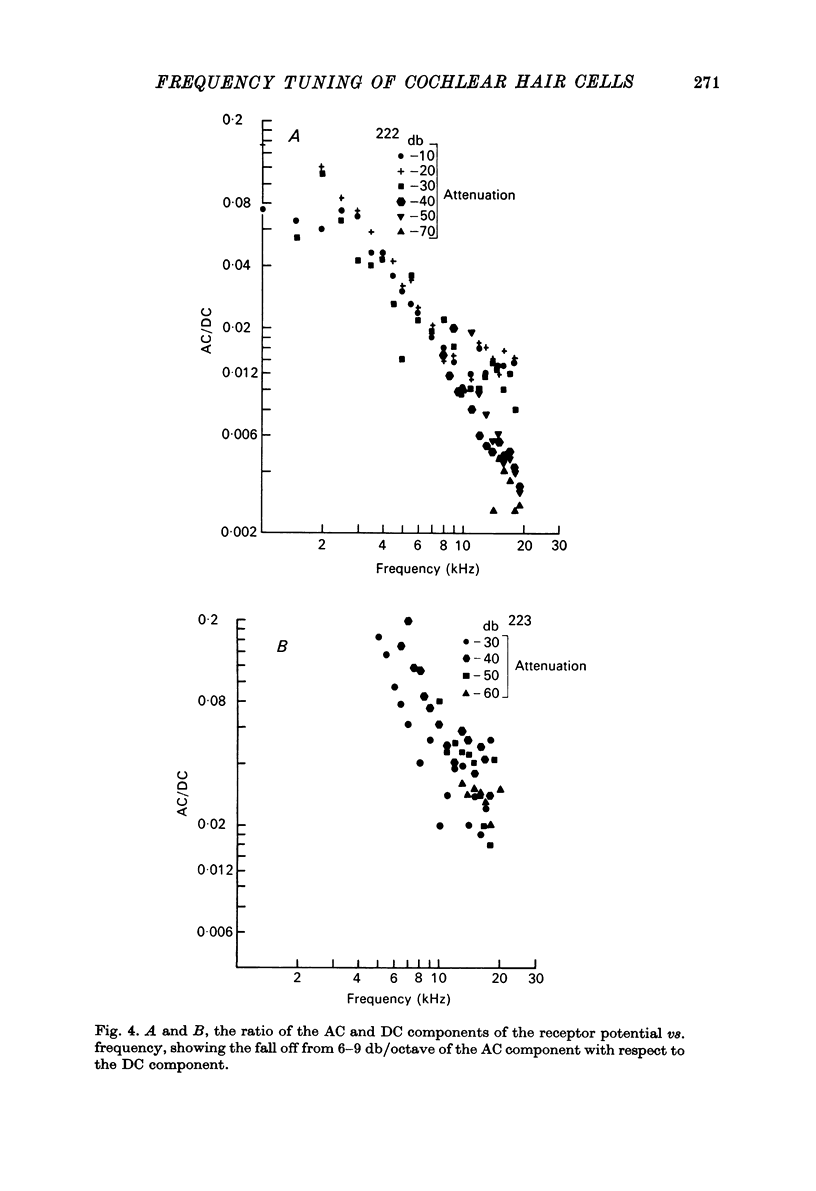
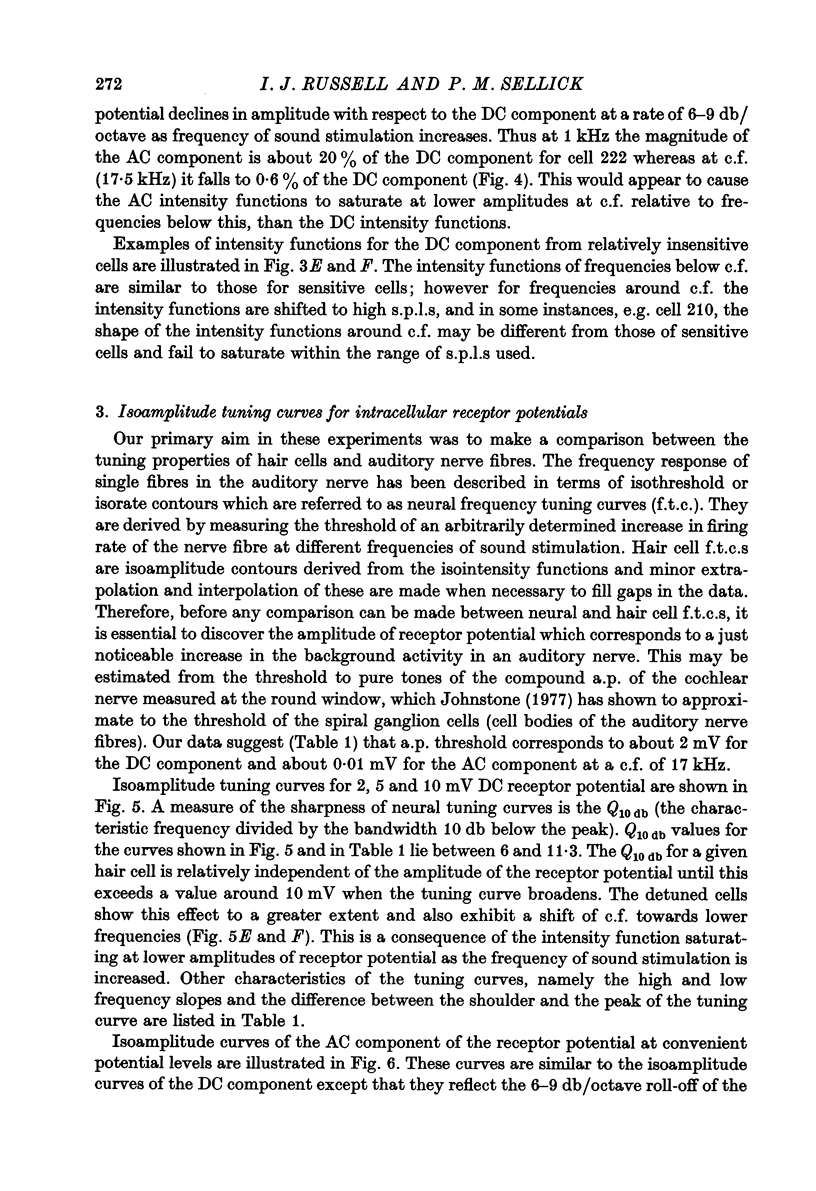
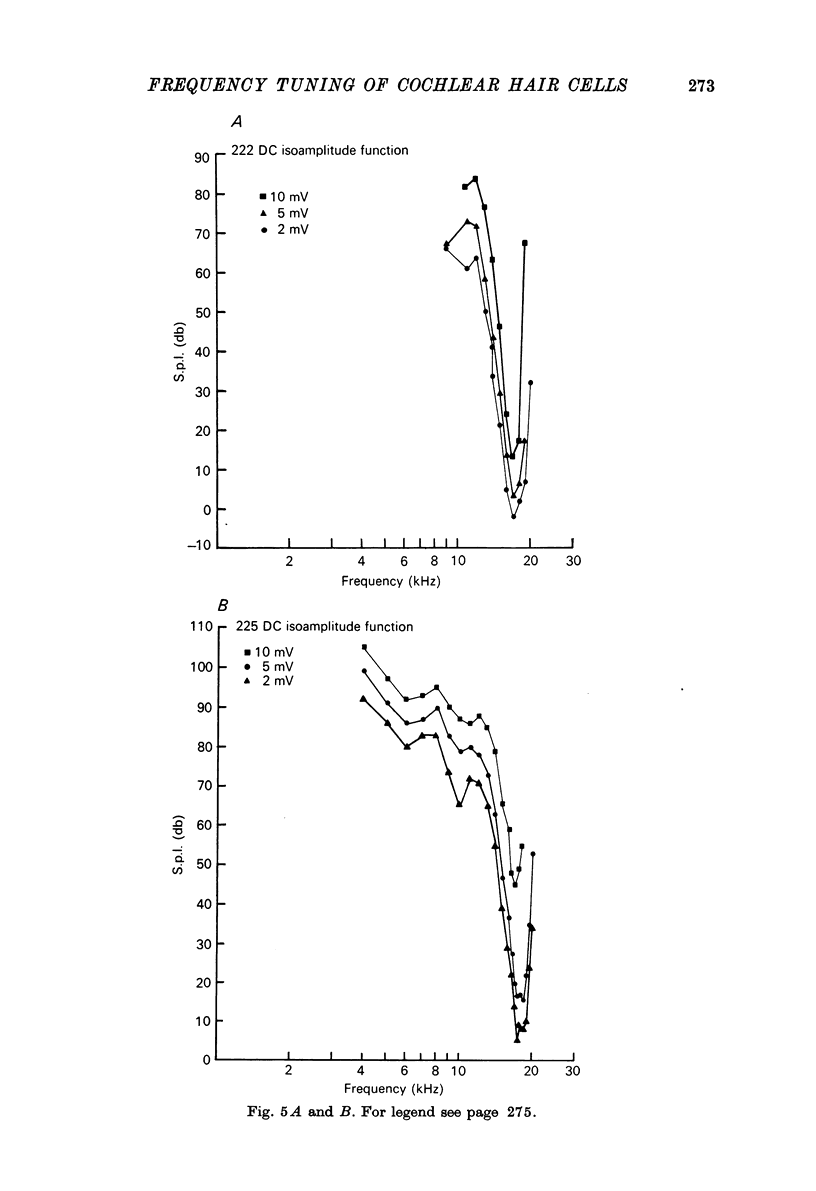
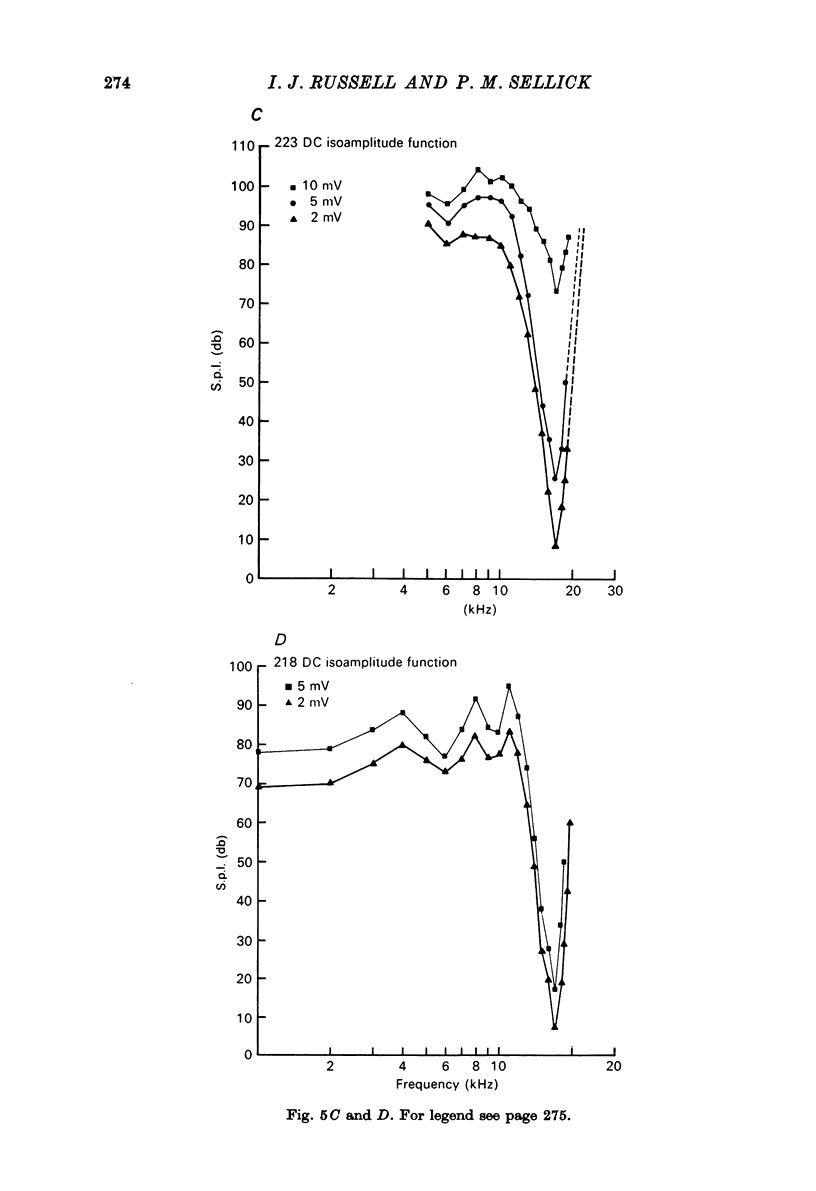
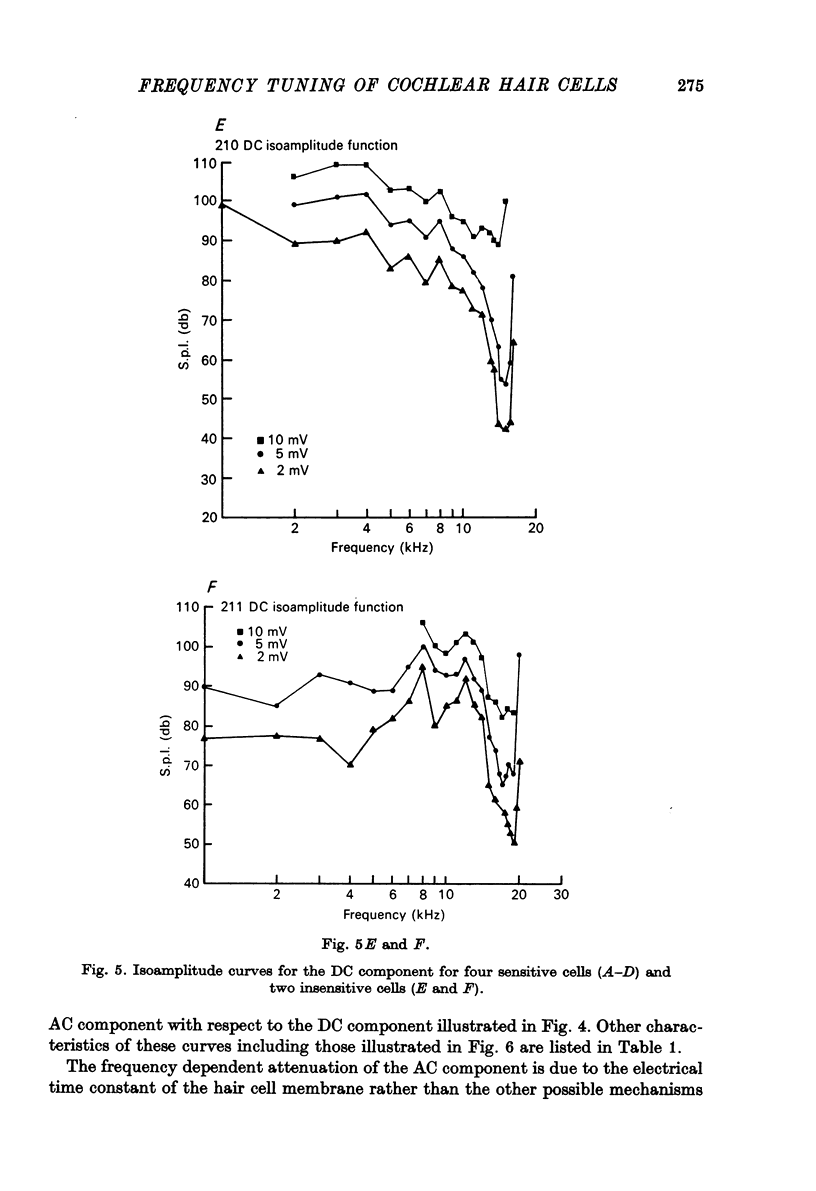
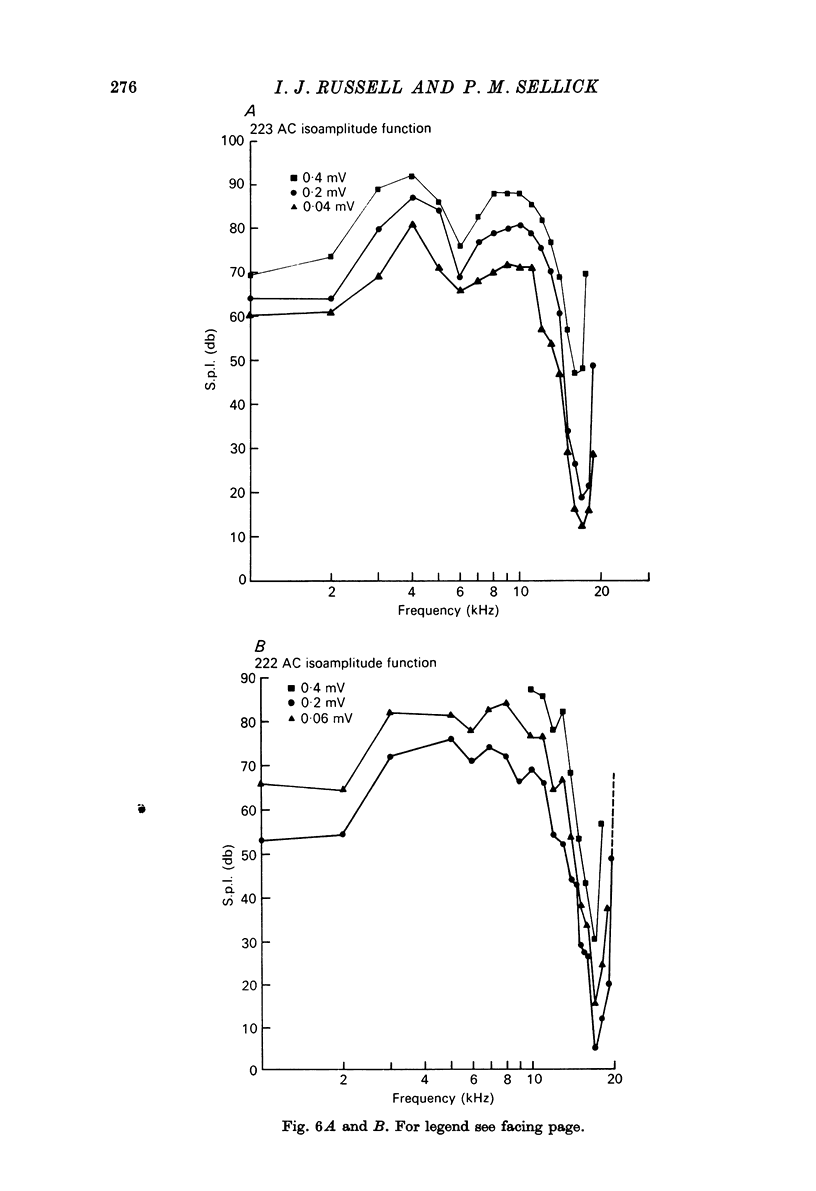
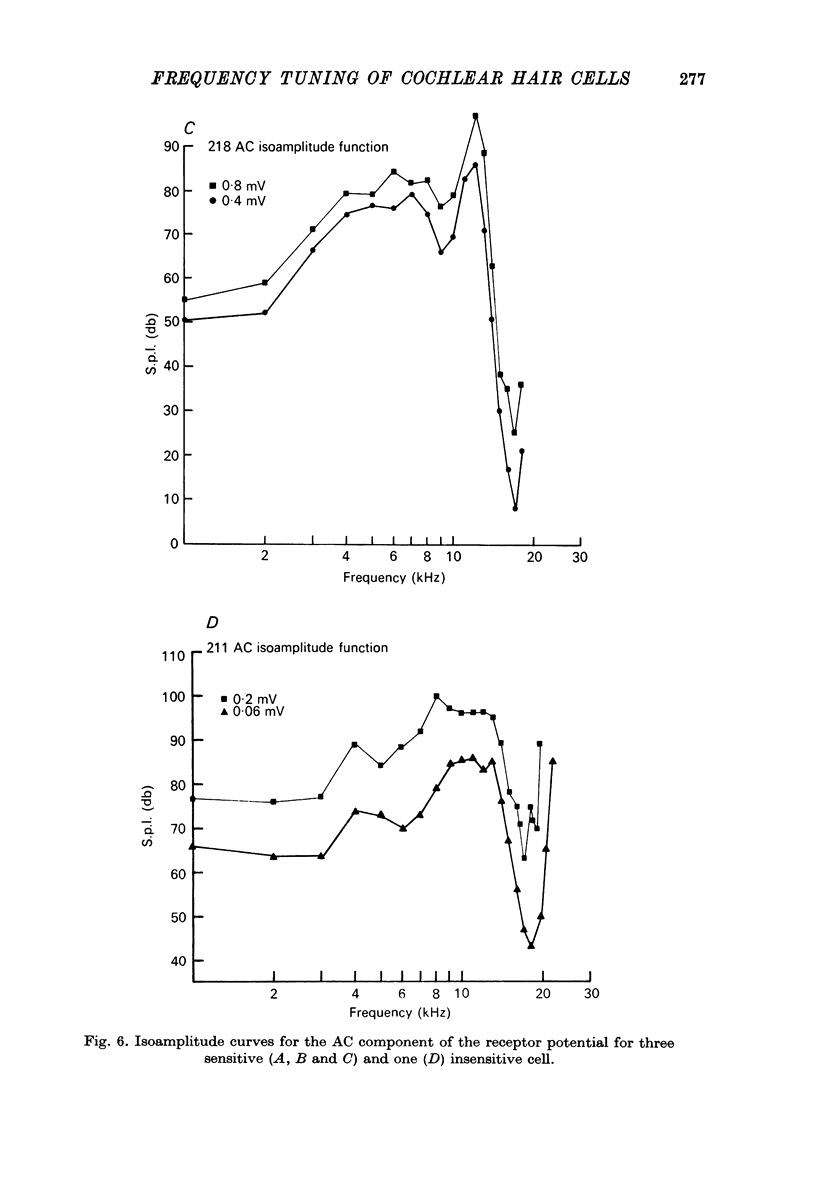
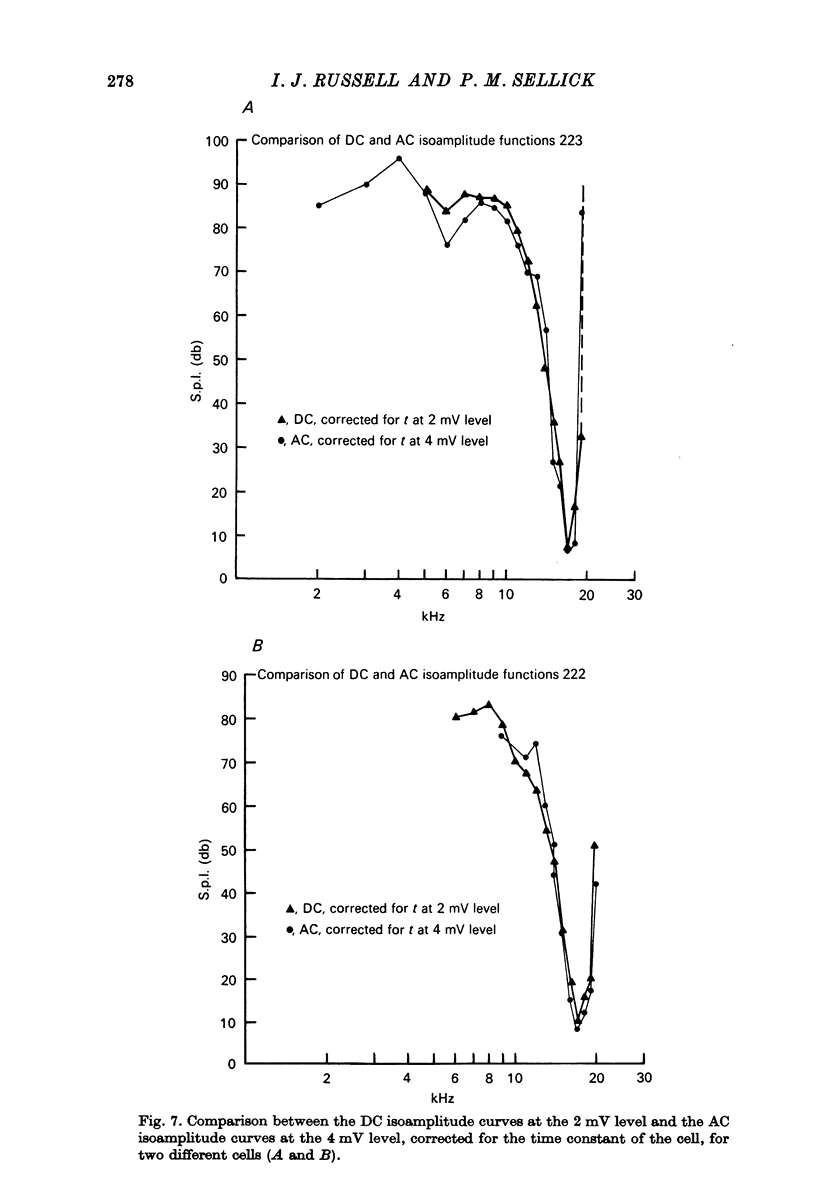
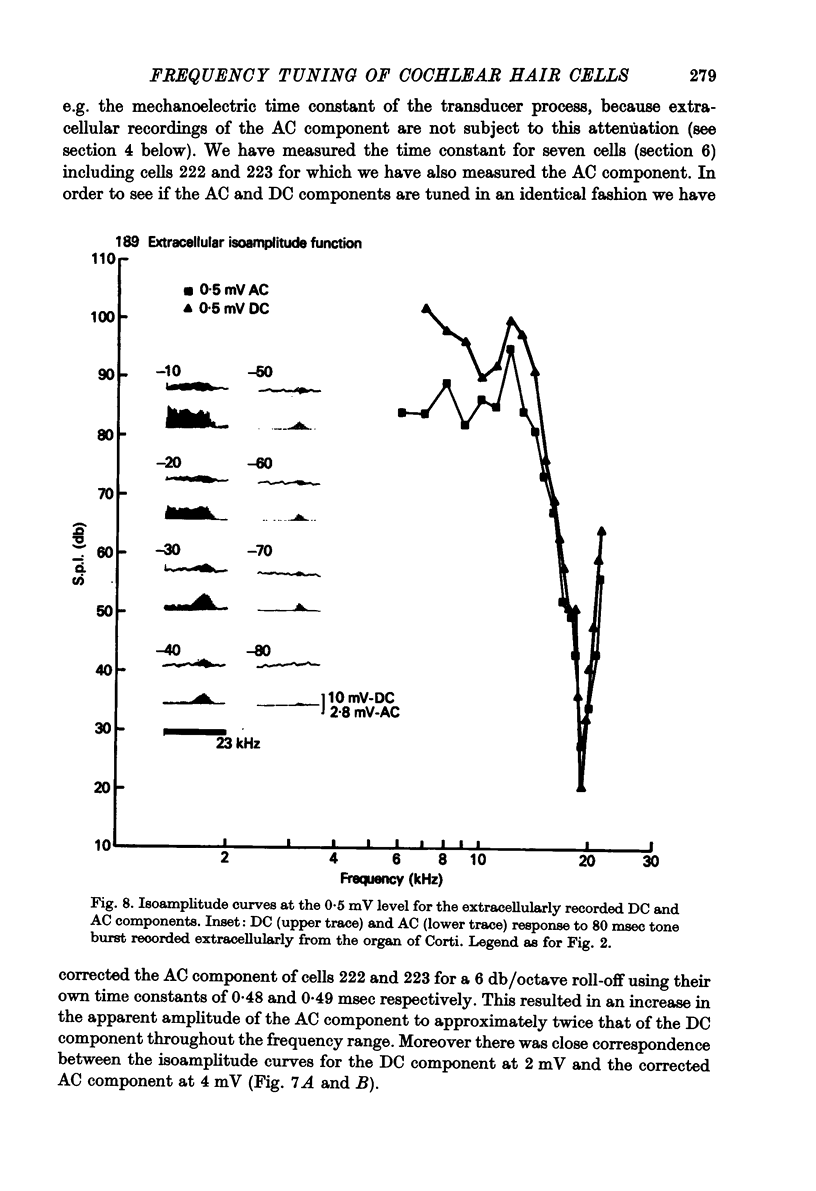
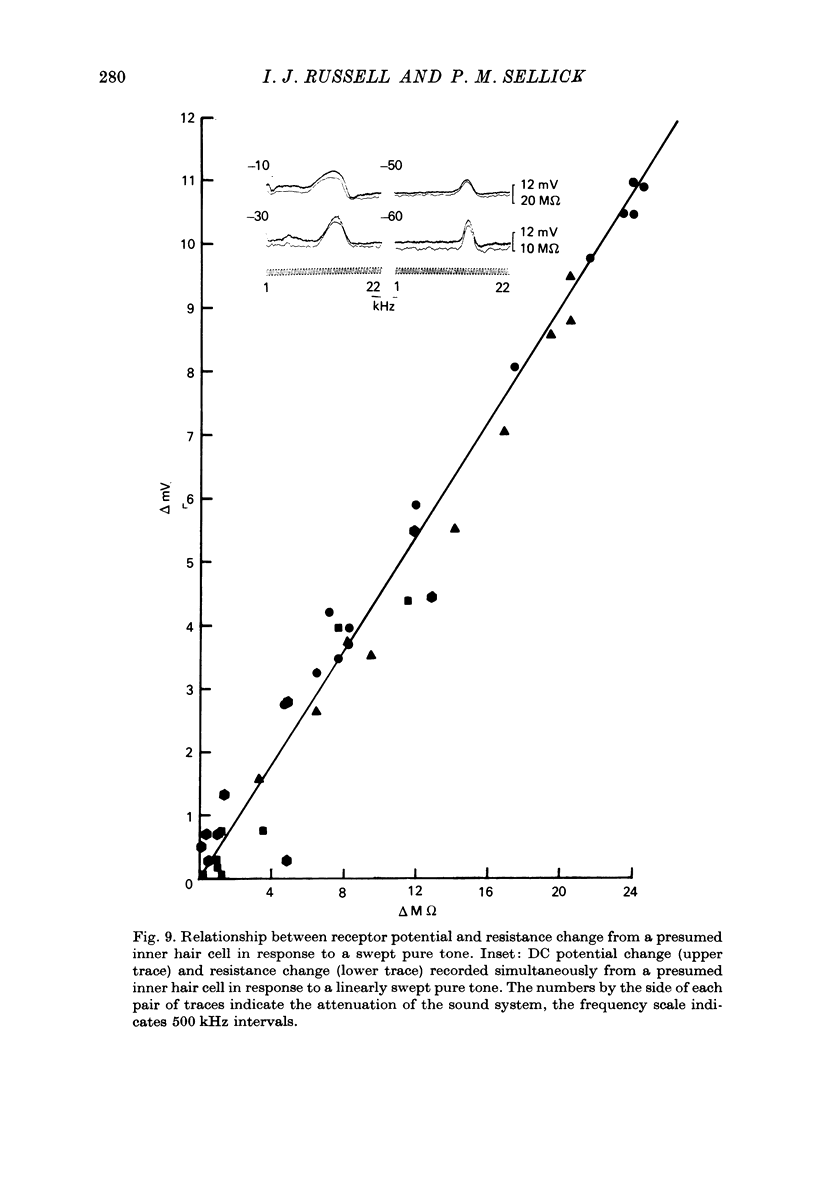
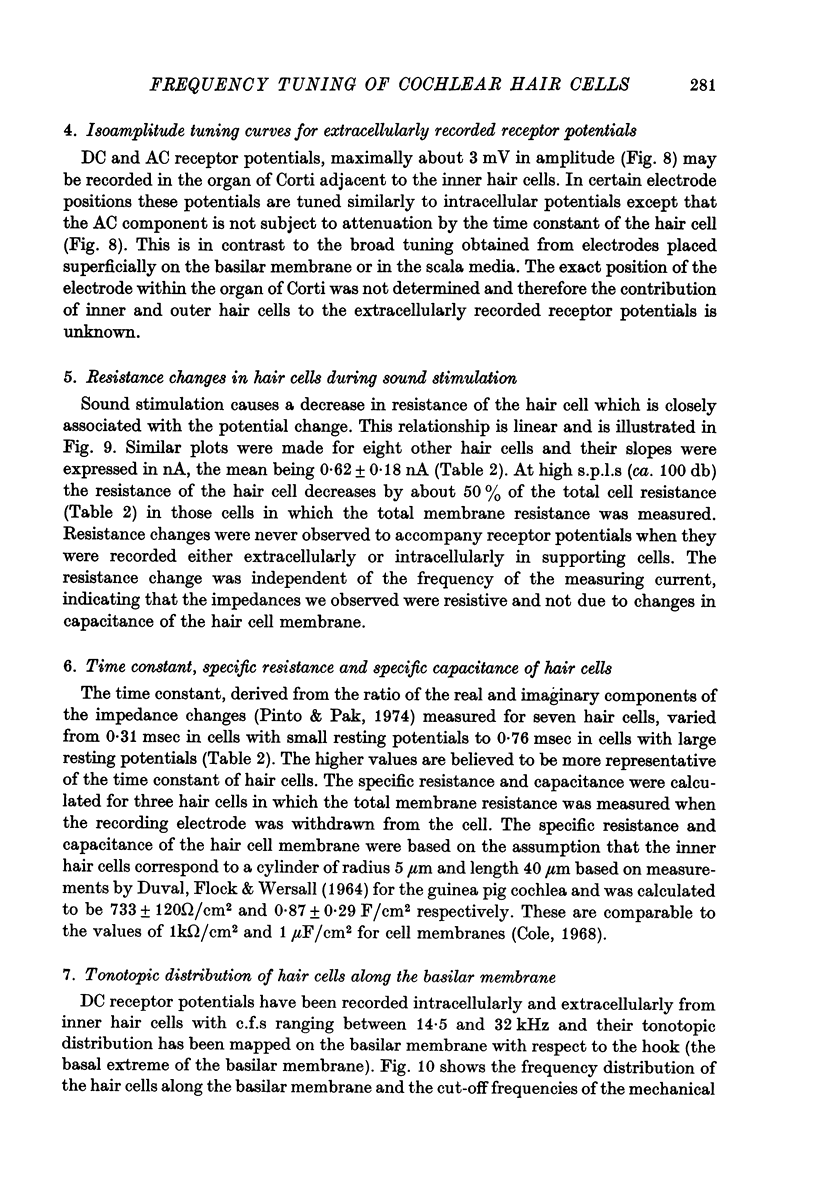
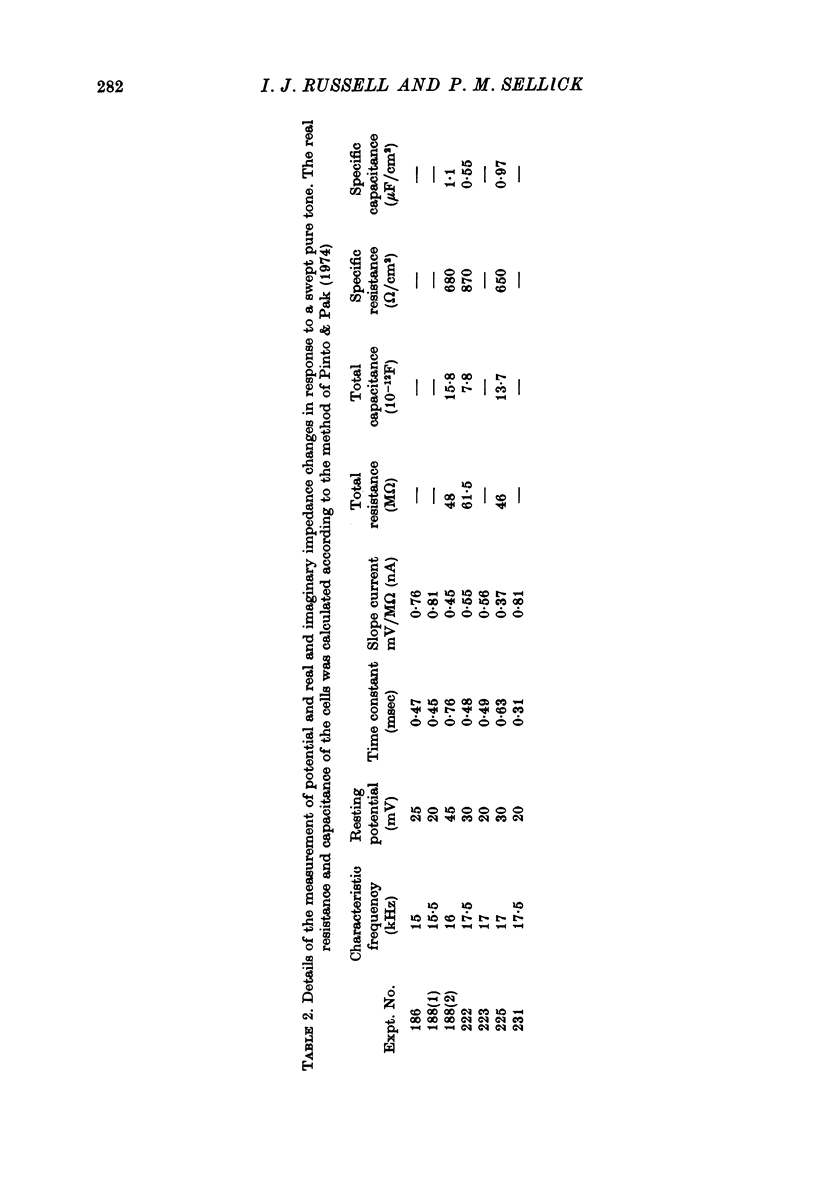
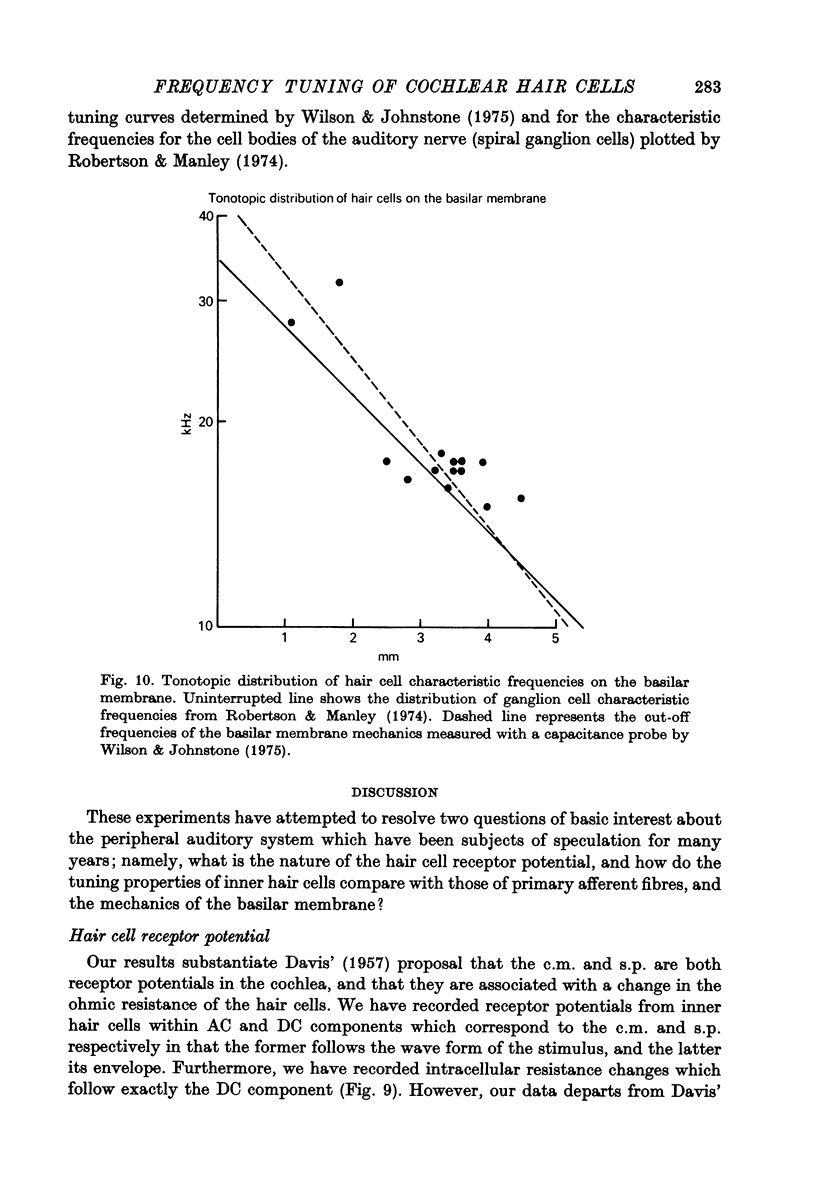
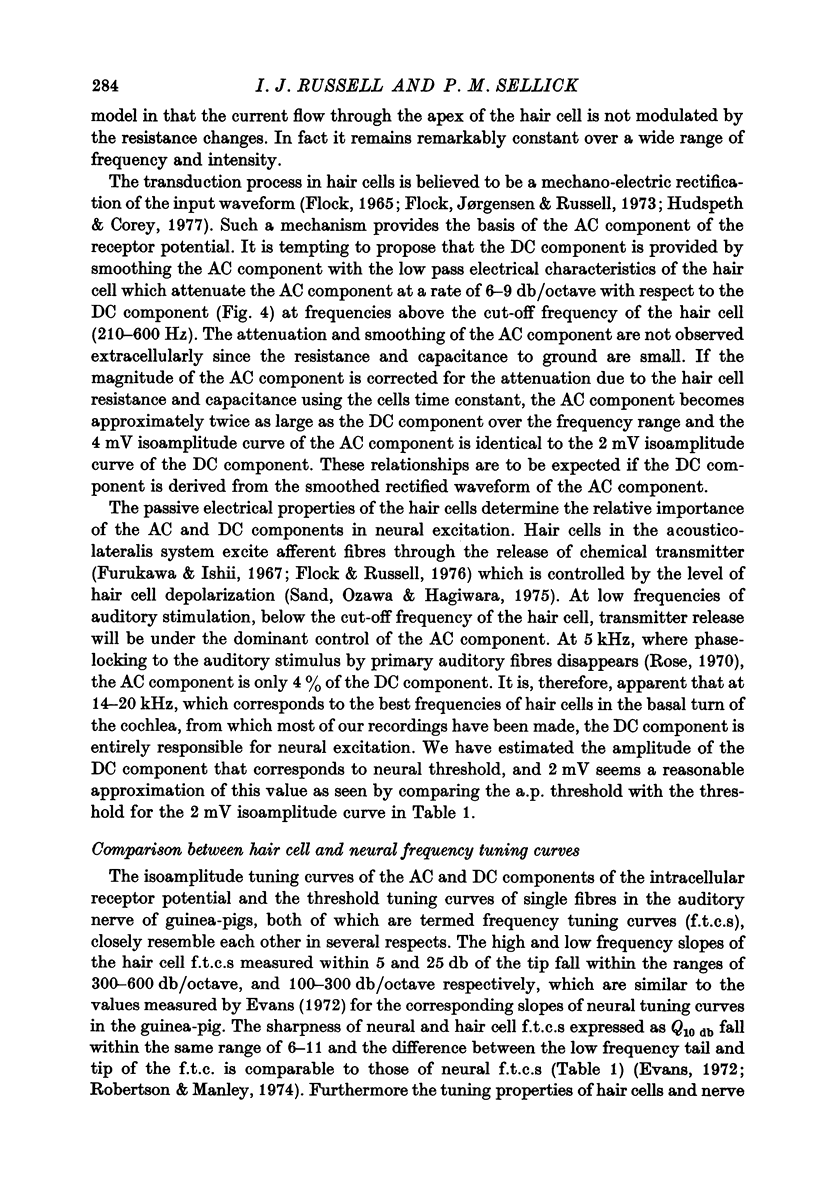
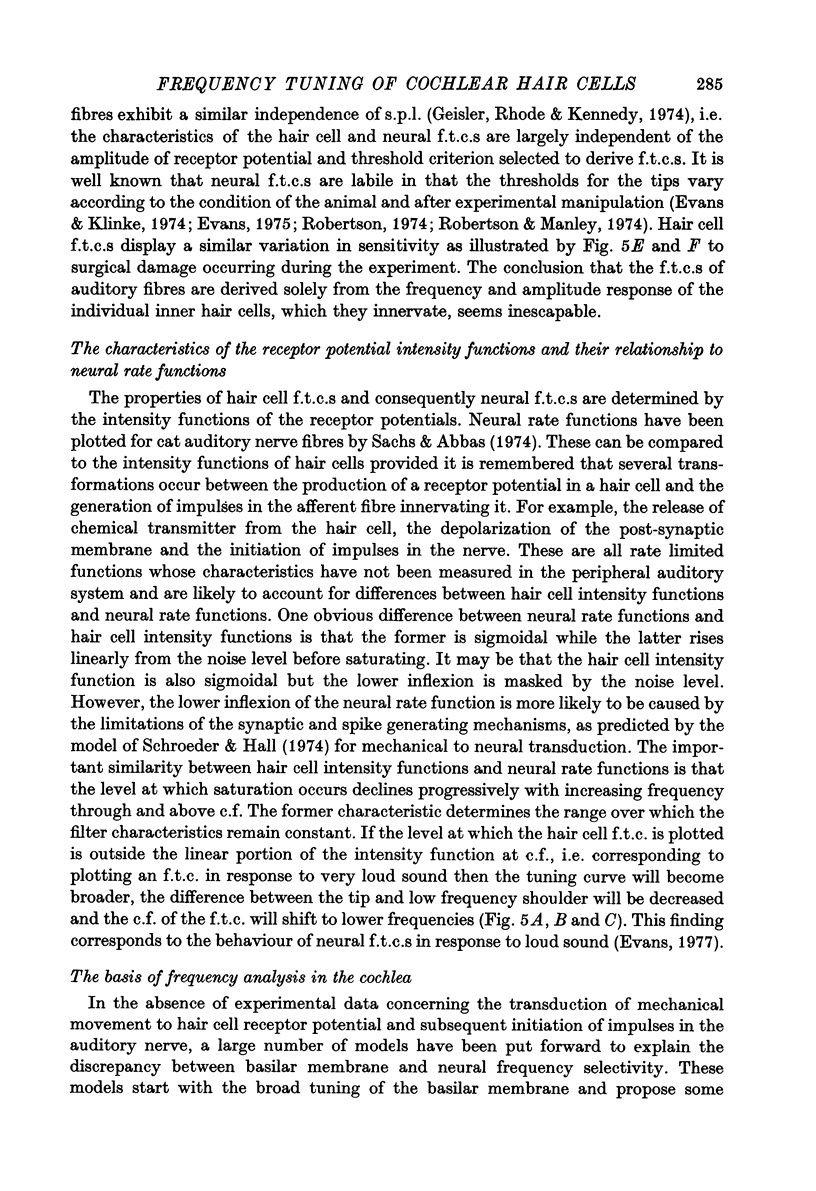
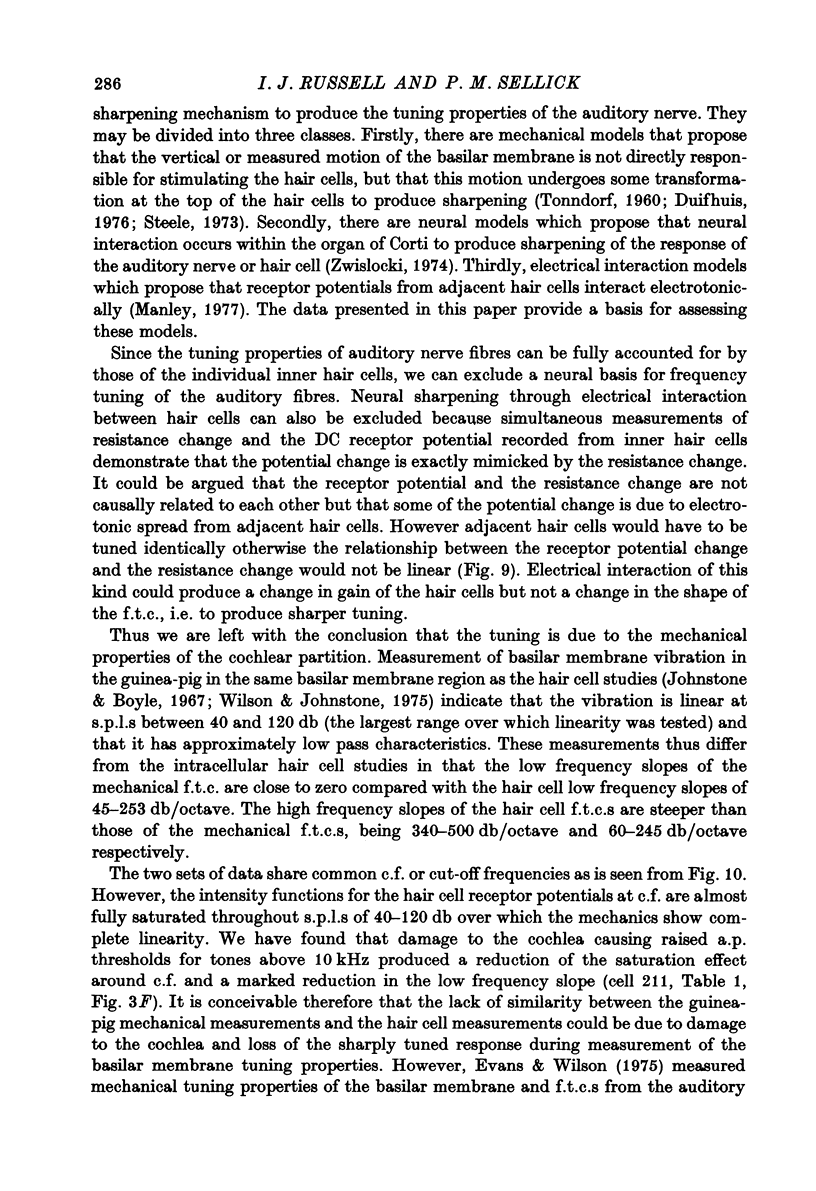

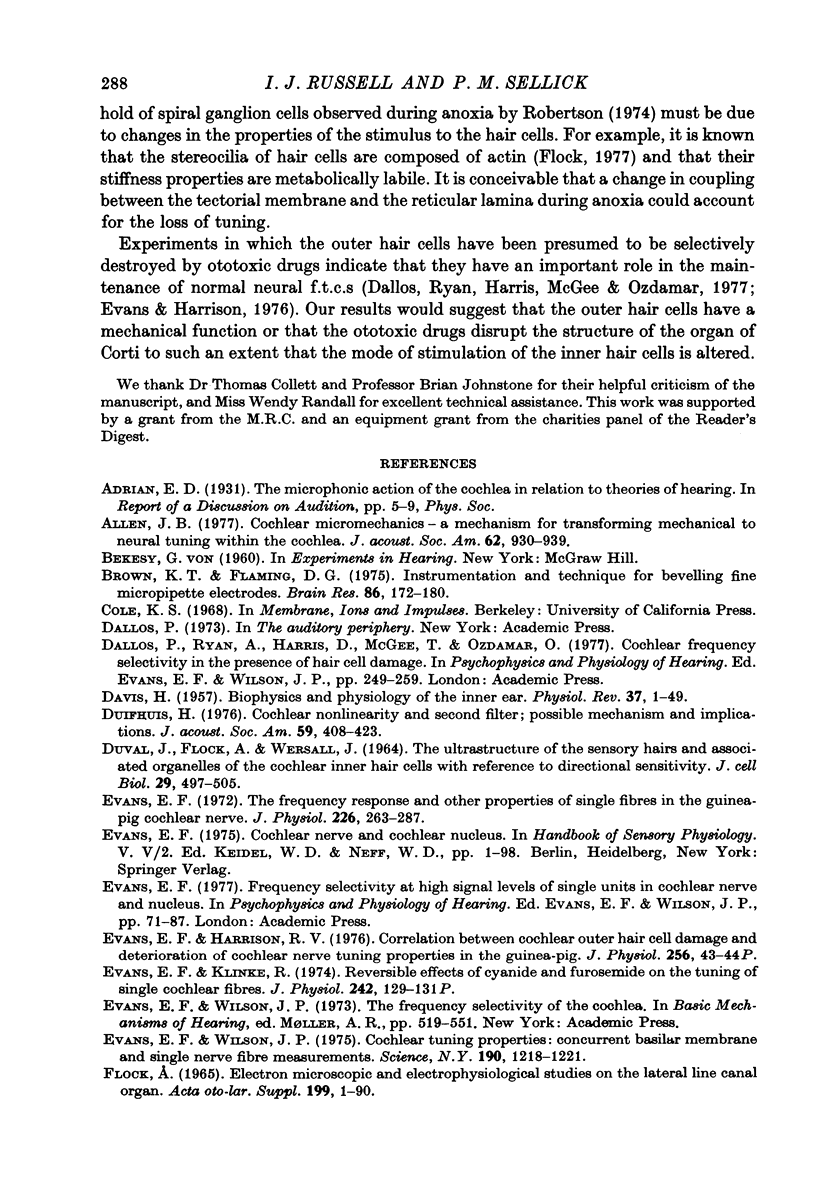
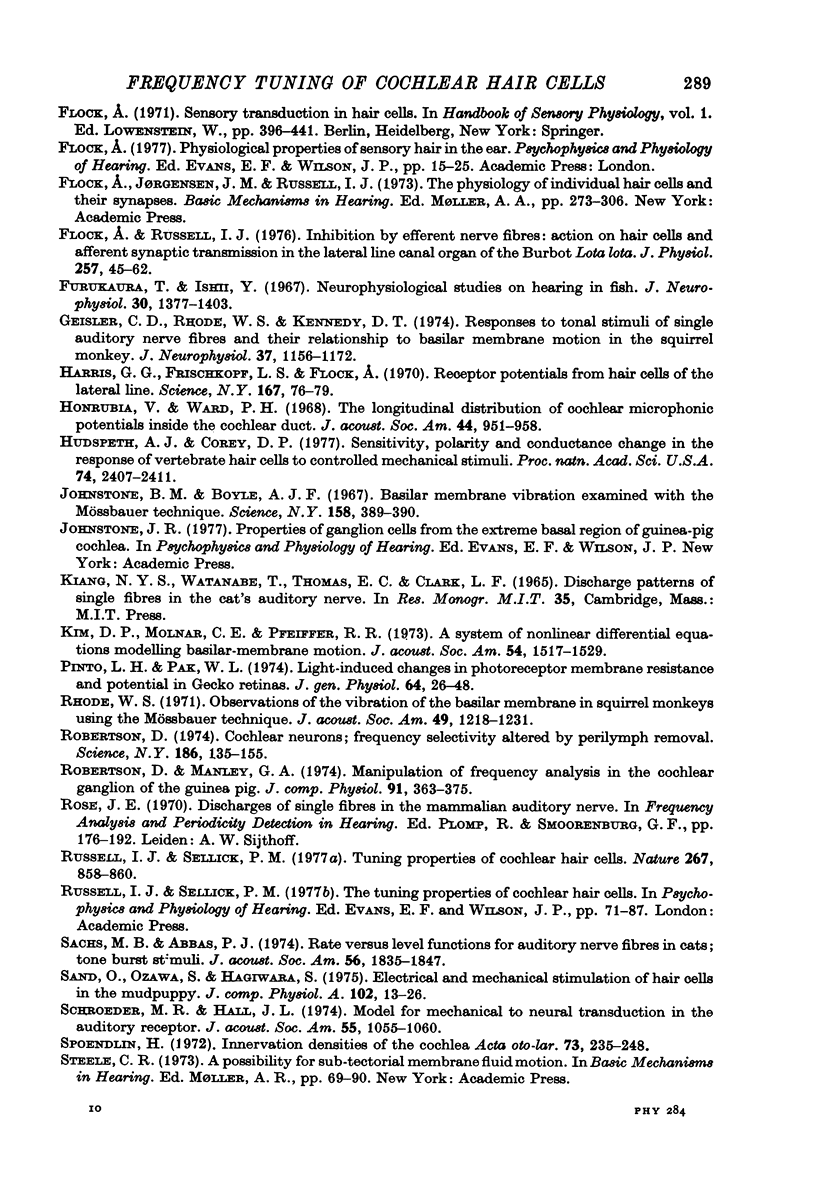
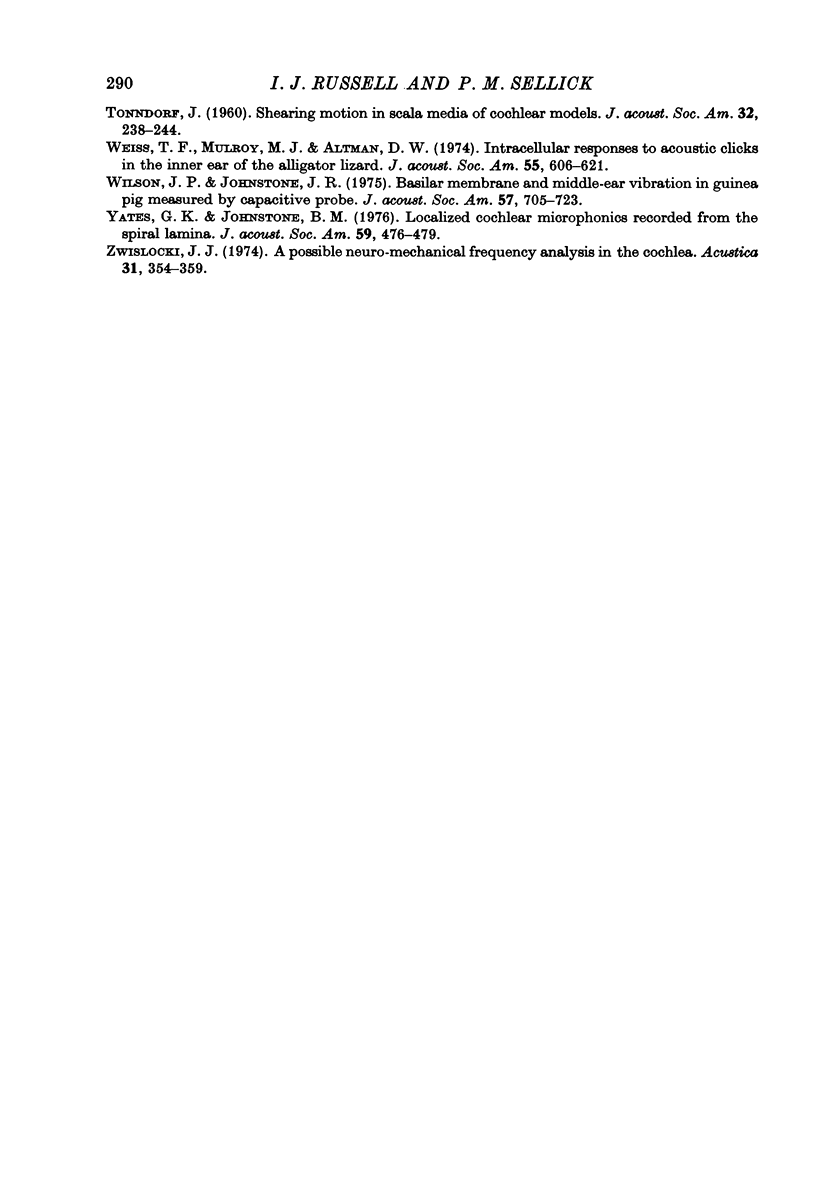
Selected References
These references are in PubMed. This may not be the complete list of references from this article.
- Allen J. B. Cochlear micromechanics--a mechanism for transforming mechanical to neural tuning within the cochlea. J Acoust Soc Am. 1977 Oct;62(4):930–939. doi: 10.1121/1.381586. [DOI] [PubMed] [Google Scholar]
- Brown K. T., Flaming D. G. Instrumentation and technique for beveling fine micropipette electrodes. Brain Res. 1975 Mar 14;86(1):172–180. doi: 10.1016/0006-8993(75)90652-6. [DOI] [PubMed] [Google Scholar]
- DAVIS H. Biophysics and physiology of the inner ear. Physiol Rev. 1957 Jan;37(1):1–49. doi: 10.1152/physrev.1957.37.1.1. [DOI] [PubMed] [Google Scholar]
- Duvall A. J., 3rd, Flock A., Wersäll J. The ultrastructure of the sensory hairs and associated organelles of the cochlear inner hair cell, with reference to directional sensitivity. J Cell Biol. 1966 Jun;29(3):497–505. doi: 10.1083/jcb.29.3.497. [DOI] [PMC free article] [PubMed] [Google Scholar]
- Evans E. F., Harrison R. V. Proceedings: Correlation between cochlear outer hair cell damage and deterioration of cochlear nerve tuning properties in the guinea-pig. J Physiol. 1976 Mar;256(1):43P–44P. [PubMed] [Google Scholar]
- Evans E. F. The frequency response and other properties of single fibres in the guinea-pig cochlear nerve. J Physiol. 1972 Oct;226(1):263–287. doi: 10.1113/jphysiol.1972.sp009984. [DOI] [PMC free article] [PubMed] [Google Scholar]
- Evans E. F., Wilson J. P. Cochlear tuning properties: concurrent basilar membrane and single nerve fiber measurements. Science. 1975 Dec 19;190(4220):1218–1221. doi: 10.1126/science.1198110. [DOI] [PubMed] [Google Scholar]
- Flock A., Russell I. Inhibition by efferent nerve fibres: action on hair cells and afferent synaptic transmission in the lateral line canal organ of the burbot Lota lota. J Physiol. 1976 May;257(1):45–62. doi: 10.1113/jphysiol.1976.sp011355. [DOI] [PMC free article] [PubMed] [Google Scholar]
- Furukawa T., Ishii Y. Neurophysiological studies on hearing in goldfish. J Neurophysiol. 1967 Nov;30(6):1377–1403. doi: 10.1152/jn.1967.30.6.1377. [DOI] [PubMed] [Google Scholar]
- Geisler C. D., Rhode W. S., Kennedy D. T. Responses to tonal stimuli of single auditory nerve fibers and their relationship to basilar membrane motion in the squirrel monkey. J Neurophysiol. 1974 Nov;37(6):1156–1172. doi: 10.1152/jn.1974.37.6.1156. [DOI] [PubMed] [Google Scholar]
- Harris G. G., Frishkopf L. S., Flock A. Receptor potentials from hair cells of the lateral line. Science. 1970 Jan 2;167(3914):76–79. doi: 10.1126/science.167.3914.76. [DOI] [PubMed] [Google Scholar]
- Honrubia V., Ward P. H. Longitudinal distribution of the cochlear microphonics inside the cochlear duct (guinea pig). J Acoust Soc Am. 1968 Oct;44(4):951–958. doi: 10.1121/1.1911234. [DOI] [PubMed] [Google Scholar]
- Hudspeth A. J., Corey D. P. Sensitivity, polarity, and conductance change in the response of vertebrate hair cells to controlled mechanical stimuli. Proc Natl Acad Sci U S A. 1977 Jun;74(6):2407–2411. doi: 10.1073/pnas.74.6.2407. [DOI] [PMC free article] [PubMed] [Google Scholar]
- Johnstone B. M., Boyle A. J. Basilar membrane vibration examined with the Mössbauer technique. Science. 1967 Oct 20;158(3799):389–390. doi: 10.1126/science.158.3799.389. [DOI] [PubMed] [Google Scholar]
- Kim D. O., Molnar C. E., Pfeiffer R. R. A system of nonlinear differential equations modeling basilar-membrane motion. J Acoust Soc Am. 1973 Dec;54(6):1517–1529. doi: 10.1121/1.1914449. [DOI] [PubMed] [Google Scholar]
- Rhode W. S. Observations of the vibration of the basilar membrane in squirrel monkeys using the Mössbauer technique. J Acoust Soc Am. 1971 Apr;49(4 Suppl):1218+–1218+. doi: 10.1121/1.1912485. [DOI] [PubMed] [Google Scholar]
- Robertson D. Cochlear neurons: frequency selectivity altered by perilymph removal. Science. 1974 Oct 11;186(4159):153–155. doi: 10.1126/science.186.4159.153. [DOI] [PubMed] [Google Scholar]
- Russell I. J., Sellick P. M. Tuning properties of cochlear hair cells. Nature. 1977 Jun 30;267(5614):858–860. doi: 10.1038/267858a0. [DOI] [PubMed] [Google Scholar]
- Schroeder M. R., Hall J. L. Model for mechanical to neural transduction in the auditory receptor. J Acoust Soc Am. 1974 May;55(5):1055–1060. doi: 10.1121/1.1914647. [DOI] [PubMed] [Google Scholar]
- Weiss T. F., Mulroy M. J., Altmann D. W. Intracellular responses to acoustic clicks in the inner ear of the alligator lizard. J Acoust Soc Am. 1974 Mar;55(3):606–619. doi: 10.1121/1.1914571. [DOI] [PubMed] [Google Scholar]
- Wilson J. P., Johnstone J. R. Basilar membrane and middle-ear vibration in guinea pig measured by capacitive probe. J Acoust Soc Am. 1975 Mar;57(3):705–723. doi: 10.1121/1.380472. [DOI] [PubMed] [Google Scholar]
- Yates G. K., Johnstone B. M. Localized cochlear microphonics recorded from the spiral lamina. J Acoust Soc Am. 1976 Feb;59(2):476–479. doi: 10.1121/1.380862. [DOI] [PubMed] [Google Scholar]