Abstract
1. The soma membrane of cat motoneurones was voltage-clamped in vivo using intracellular current and voltage electrodes whose tips were separated by at least 5 micrometer. 2. Depolarization activates two separate, non-interacting K conductance systems whose rates of activation and decay differ by a factor of about 10. These conductances have a similar reversal potential, in the range of -6 to -21 mV (these and all subsequent voltages are expressed relative to the resting potential). Both conductances show linear 'instantaneous' current-voltage relationships. The steady-state magnitudes of both conductances increase with increasing depolarization. Neither conductance inactivates substantially during prolonged depolarizations. 3. The faster K conductance is similar to that described for squid axons and frog node. Activation begins at about +30 mV and is more than 90% complete within 5 msec of a depolarizing voltage step to +50 mV. Activation kinetics appear to be nonlinear. This fast K conductance contributes to the fast falling phase of the action potential. Following repolarization, this conductance decays with a time constant of 2-4 msec. 4. The slower K conductance activates during depolarizations of 10 mV or greater. The activation and decay of this conductance can be described by first-order exponential functions with time constants ranging from 20 to 50 msec. The slow K conductance underlies the prolonged hyperpolarization that follows motoneurone action potentials. Evidence from other studies suggests that this slow K conductance is regulated by intracellular Ca ions. 5. In addition to the two K conductance systems activated by depolarization, motoneurones exhibit another distinct conductance system that is activated by hyperpolarization. This third system has a reversal potential near the resting potential. Activation of this conductance during a hyperpolarizing voltage step can be fitted by a single exponential function with a time constant of 50-60 msec over the range -20 to -50 mV. This hyperpolarization-activated conductance accounts for some aspects of the anomalous rectification reported in cat motoneurones. 6. When the clamp circuit was turned off and the motoneurones were stimulated to discharge repetitively by depolarizing current steps, the apparent soma threshold voltage increased as the applied current (and discharge frequency) increased. 7. The basic features of the motoneurone action potential were reconstructed by simulations based on voltage clamp measurements of the voltage dependent conductance systems and previous measurements of passive membrane properties. These simulations assumed that the kinetics of the fast Na and K conductance systems in motoneurones can be described by equations of the same form as the Hodgkin-Huxley equations. These action potential reconstructions indicated that a major portion of the delayed depolarization following the action potential is attributable to capacitative currents from the dendrites...
Full text
PDF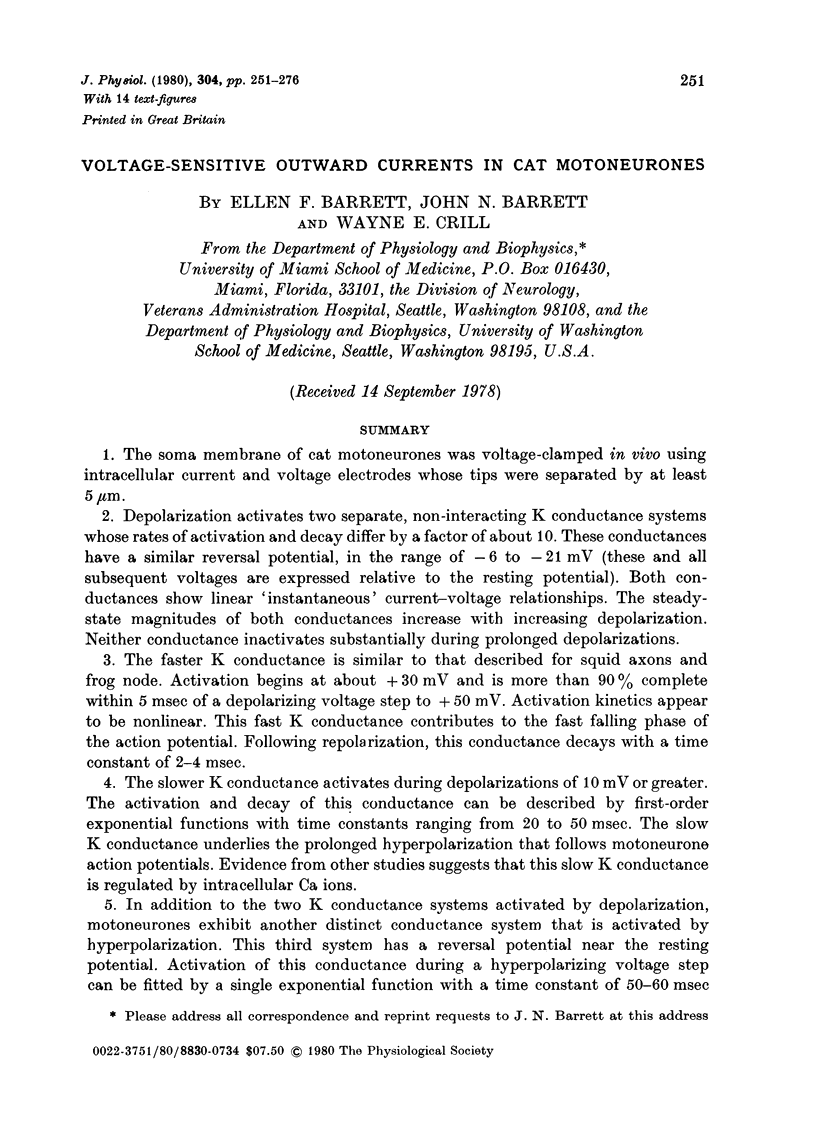
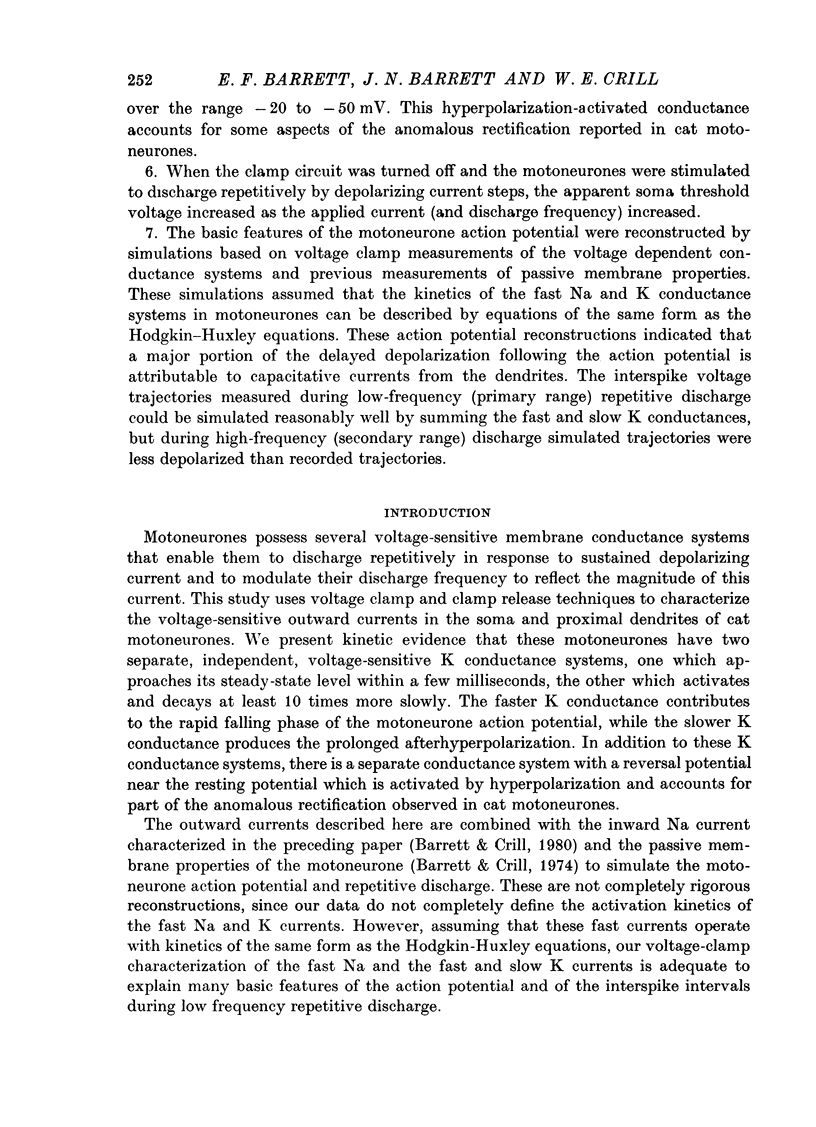
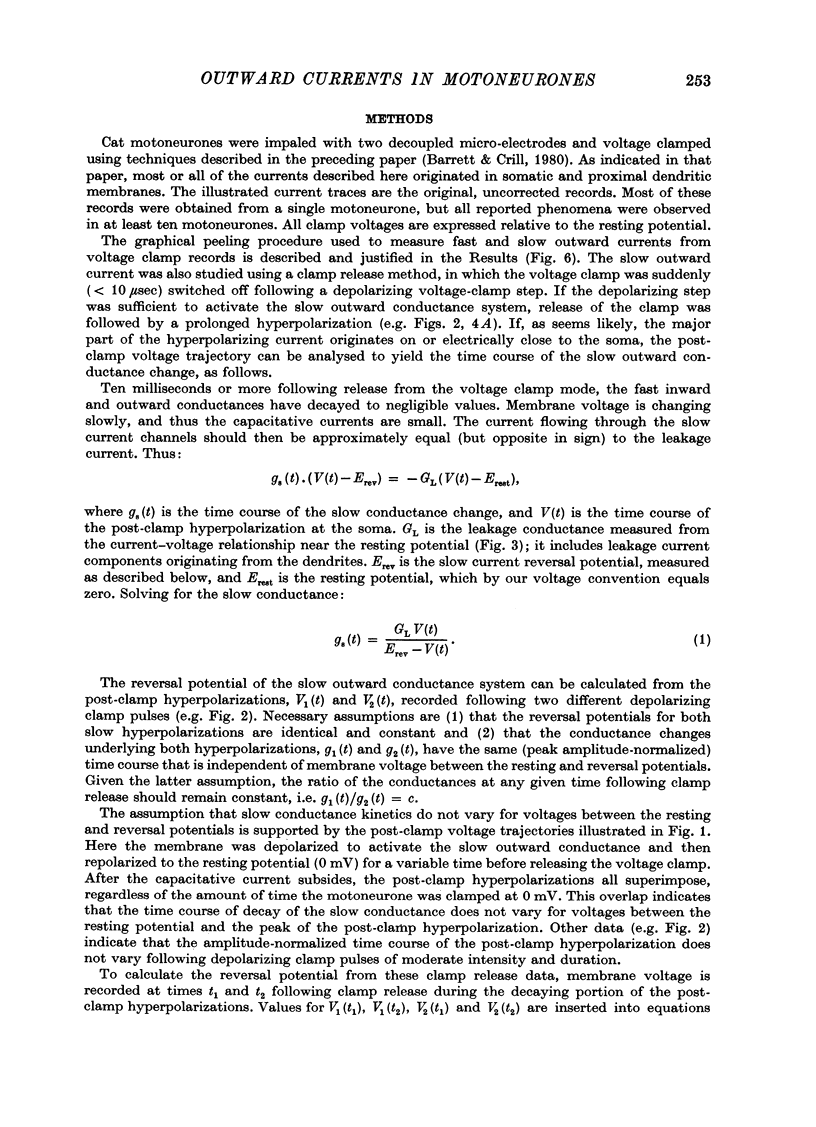
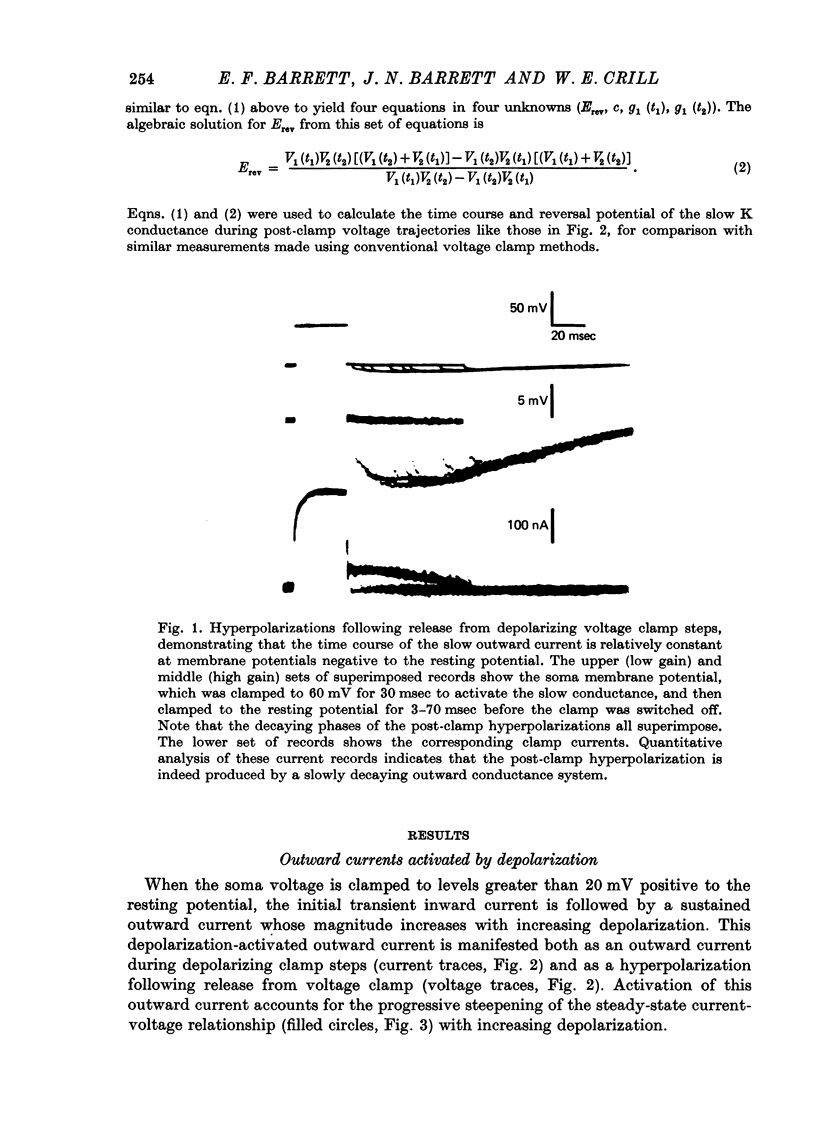
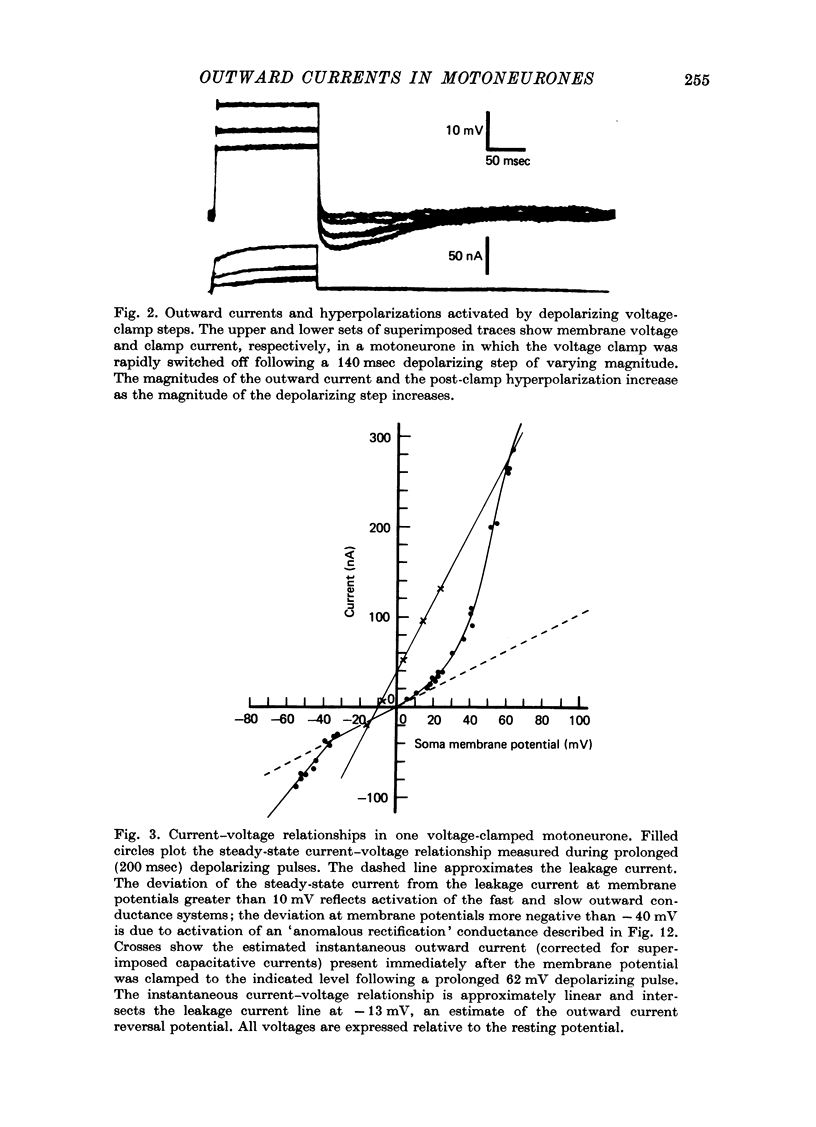
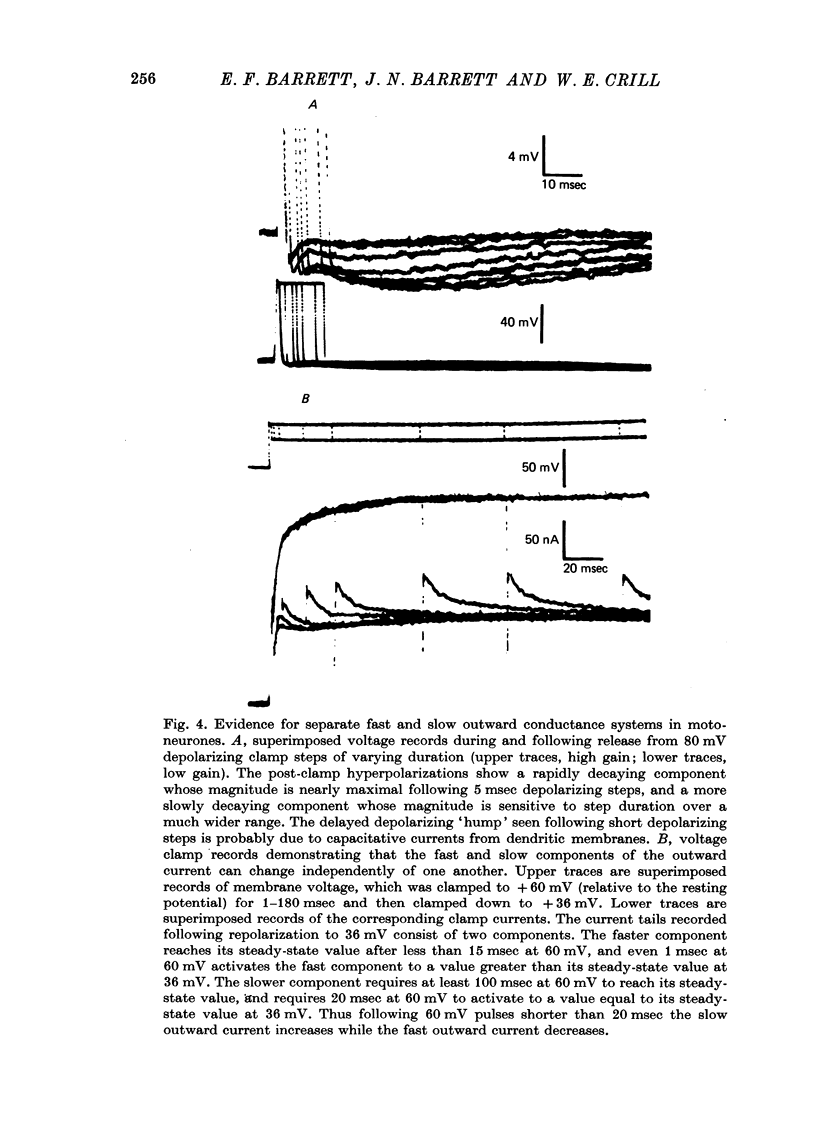
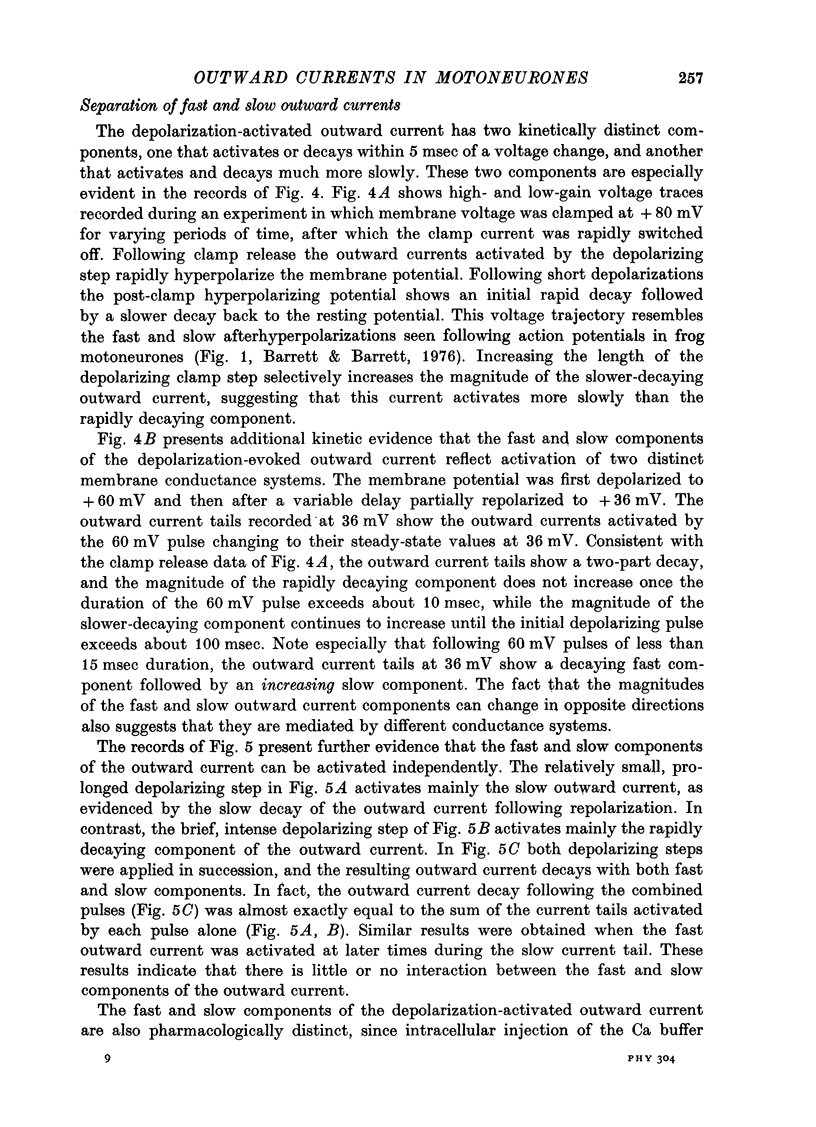
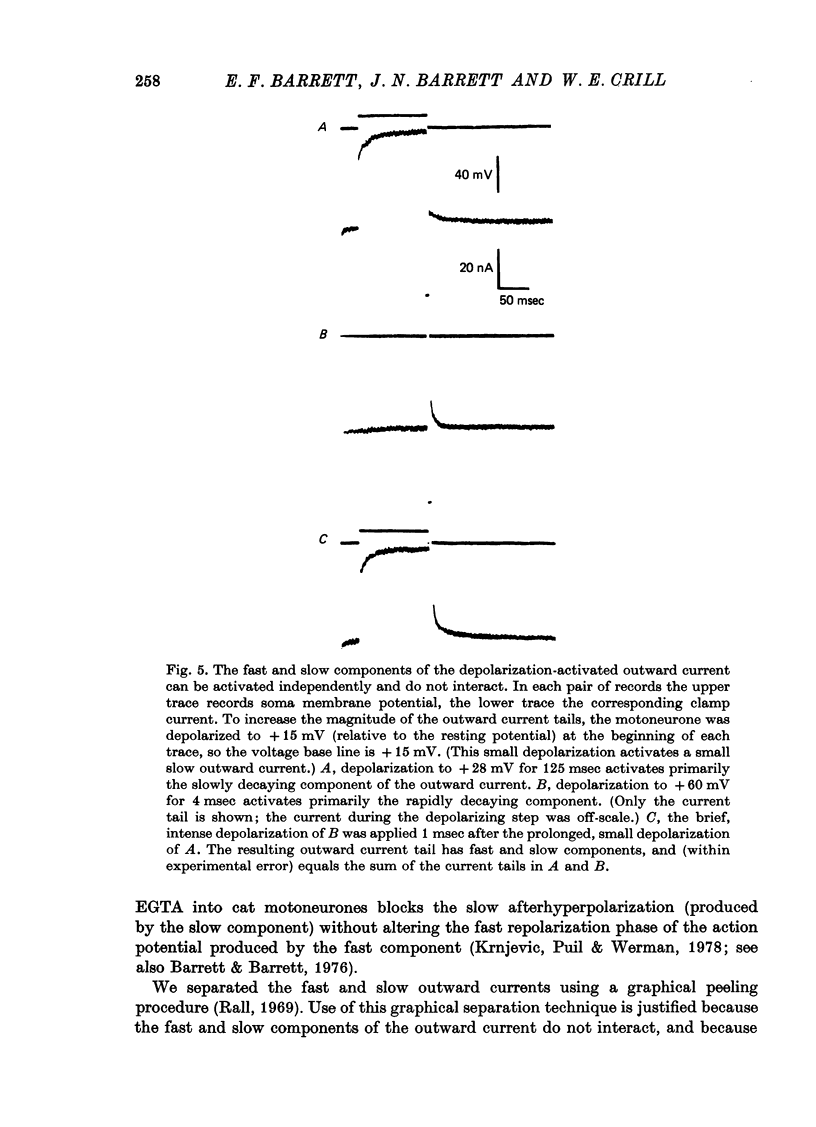
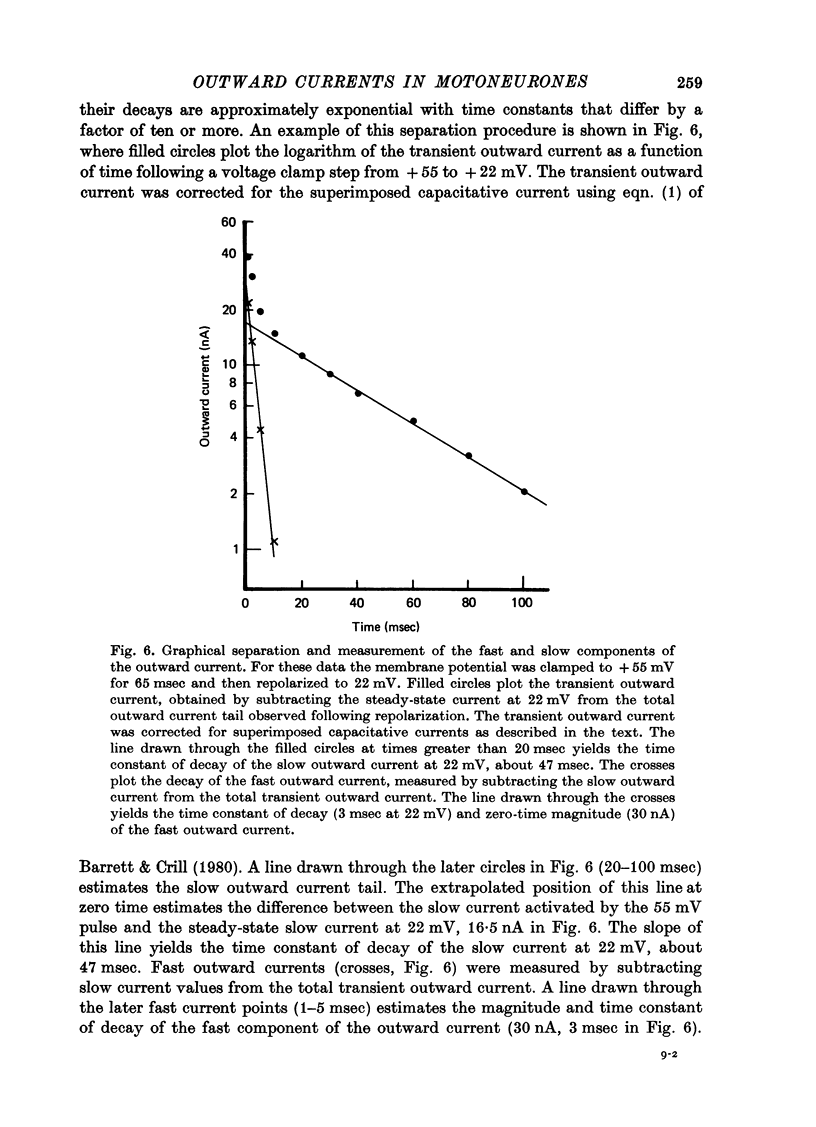
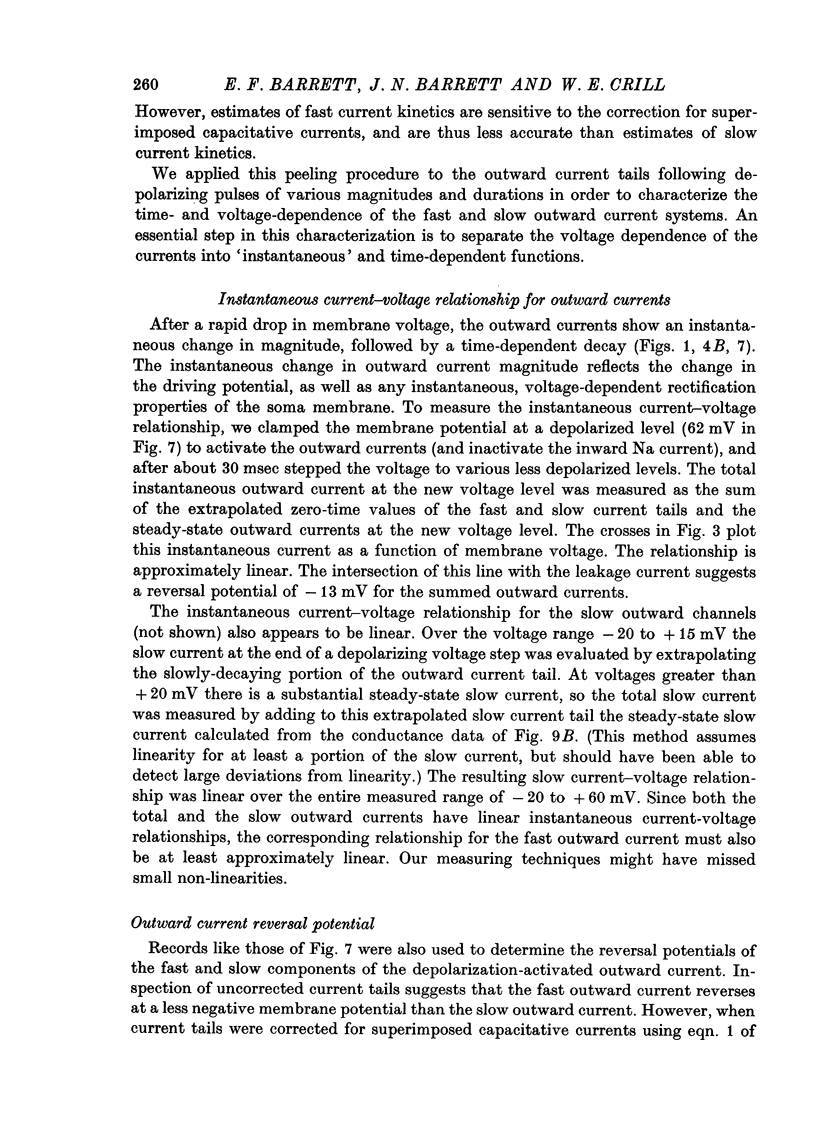
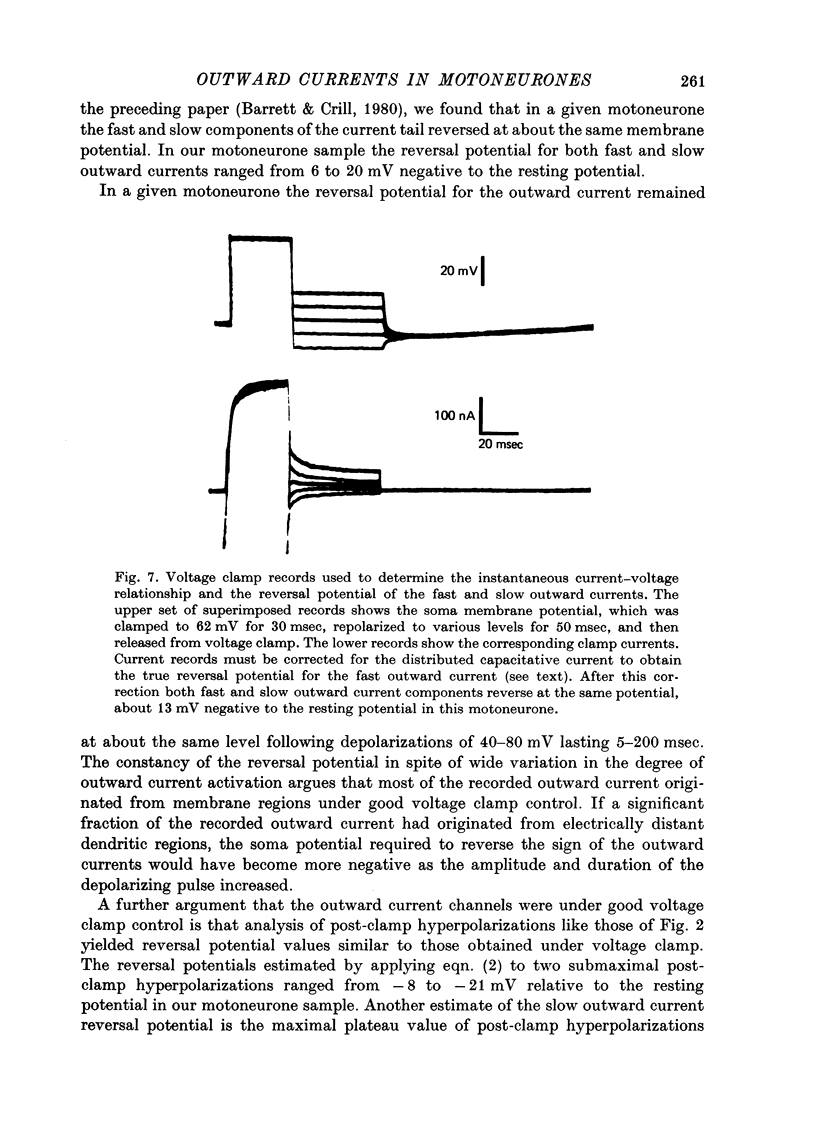
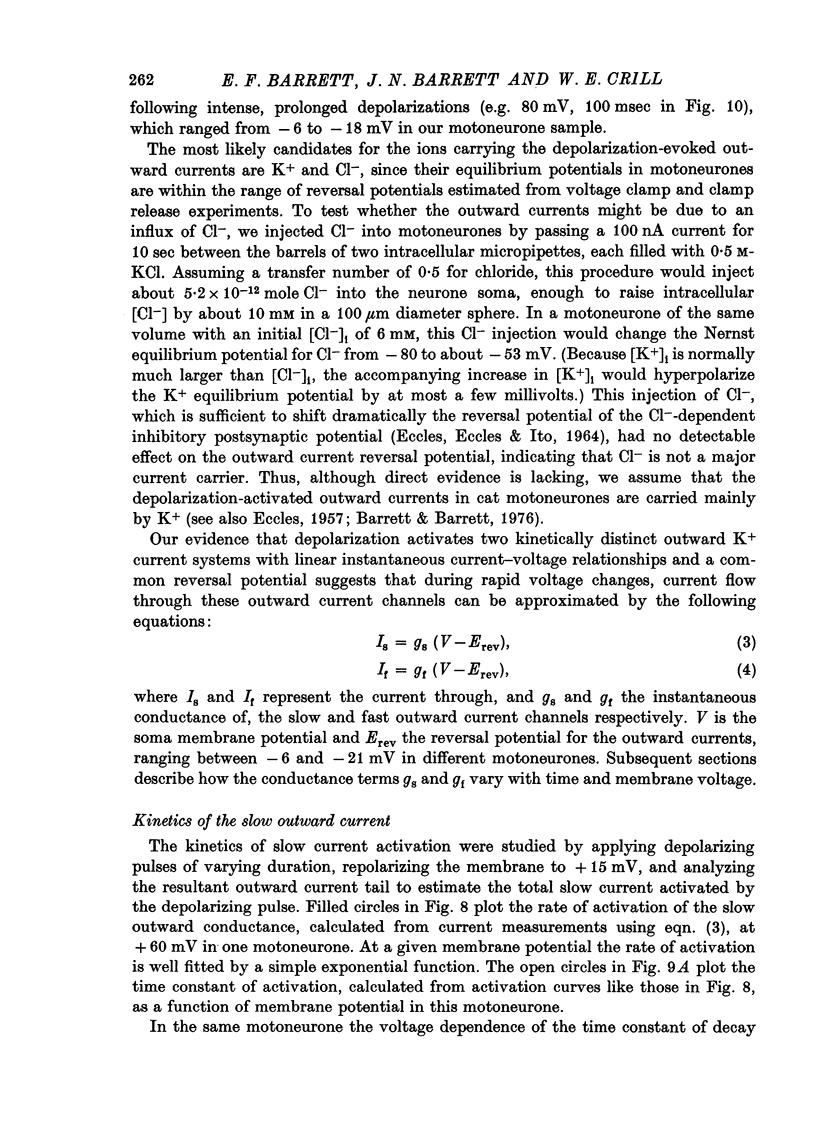
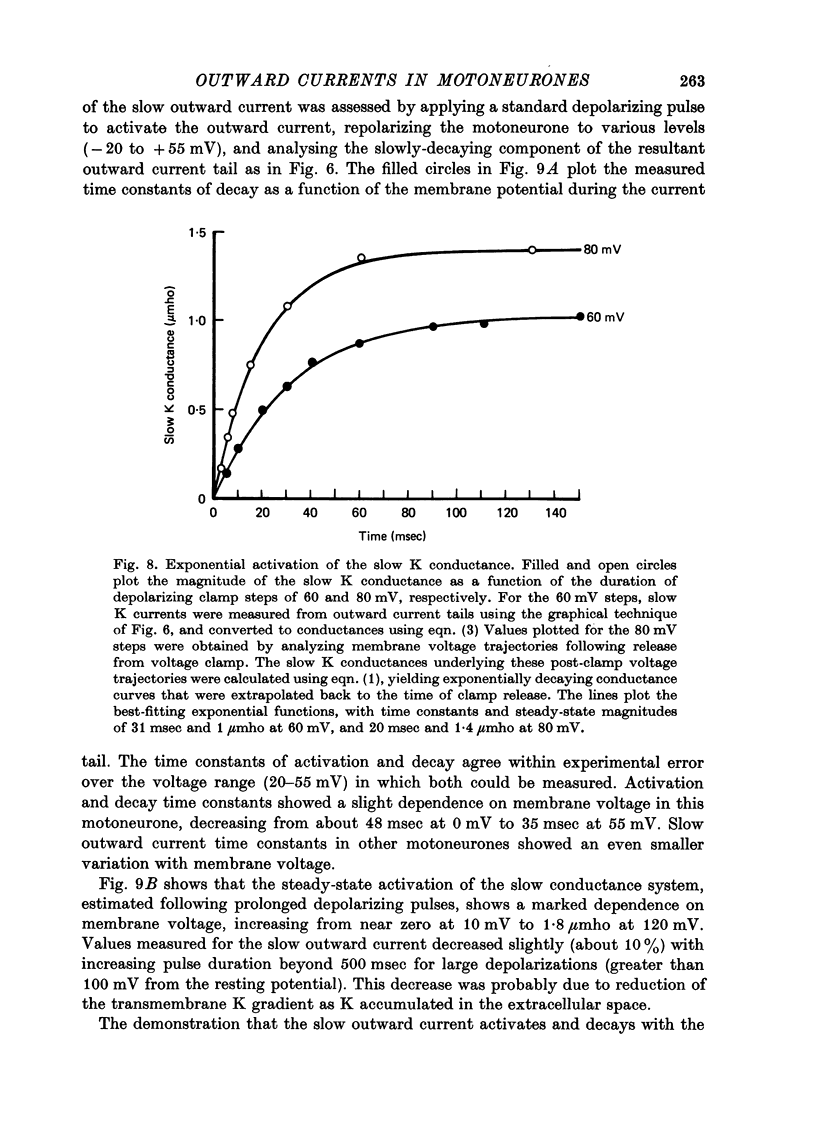
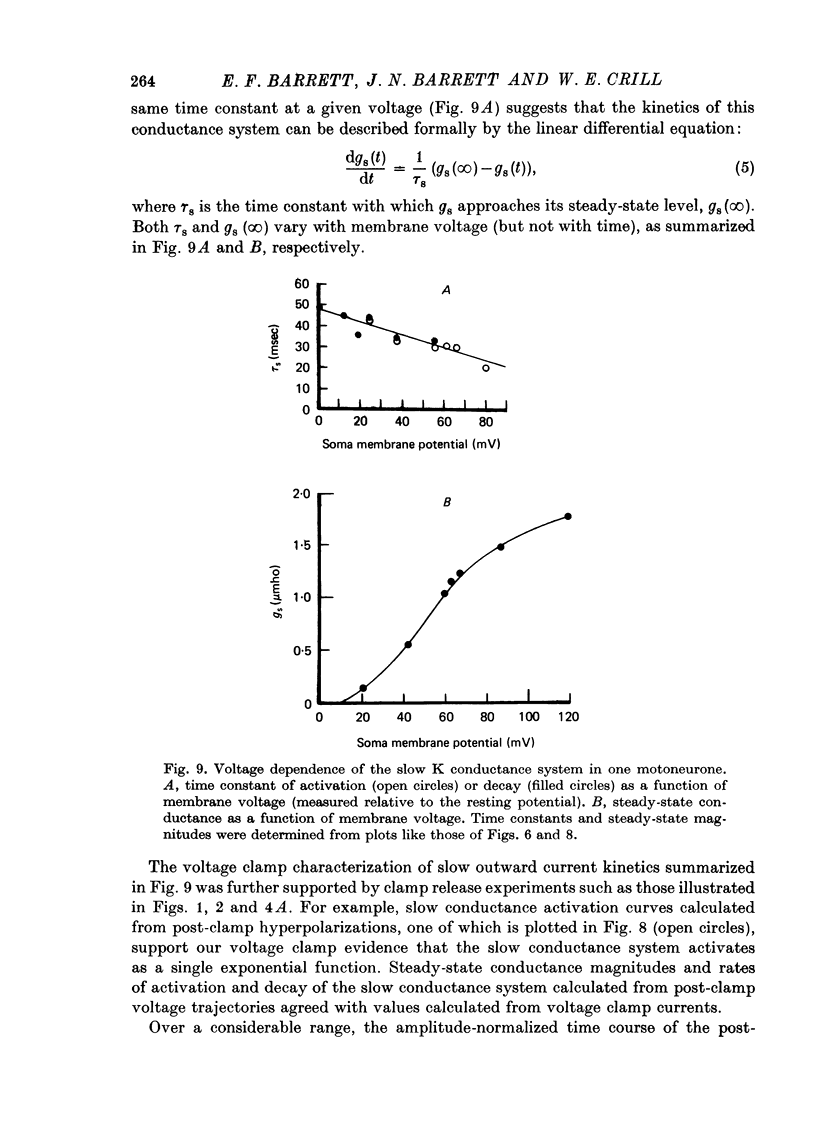
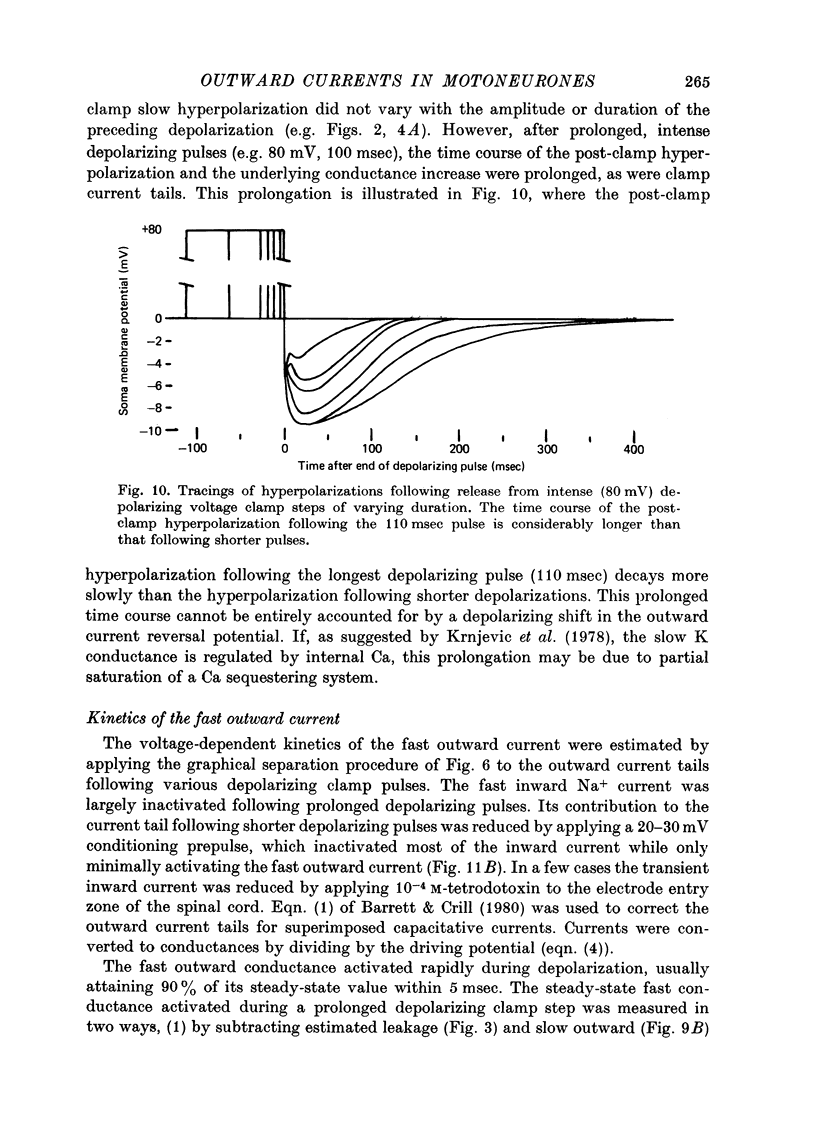
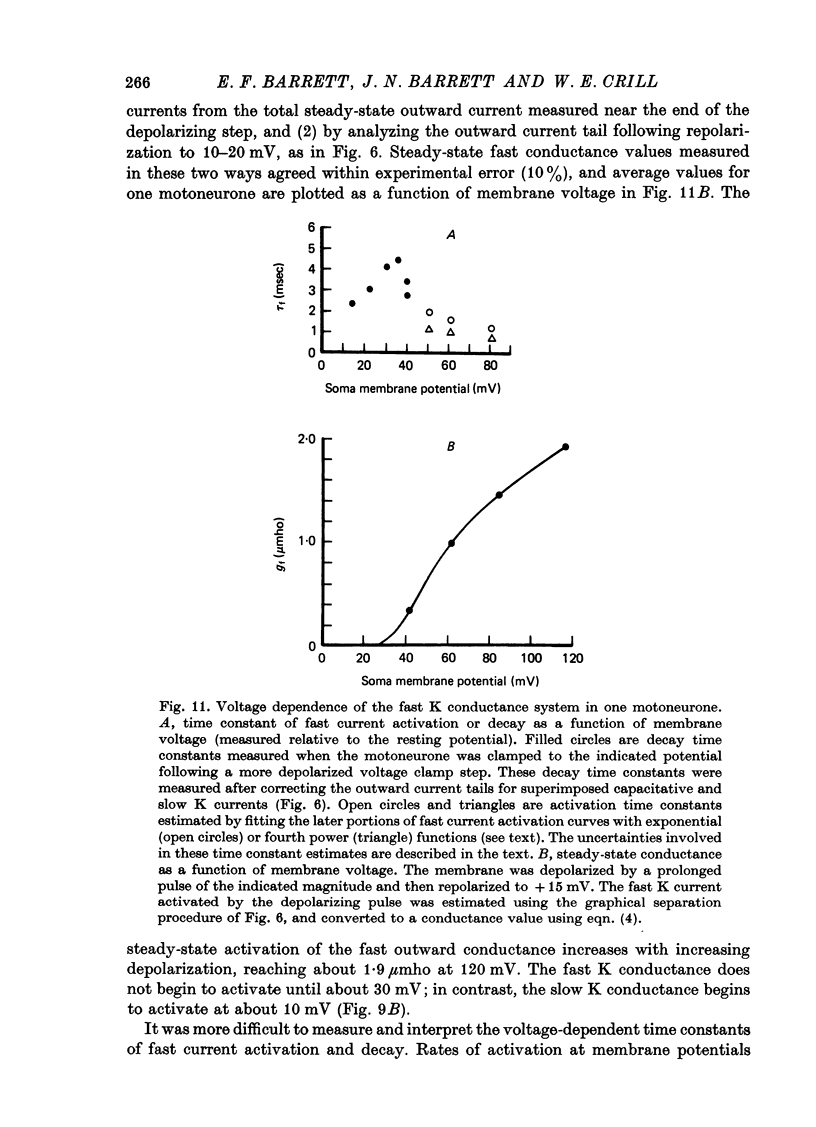
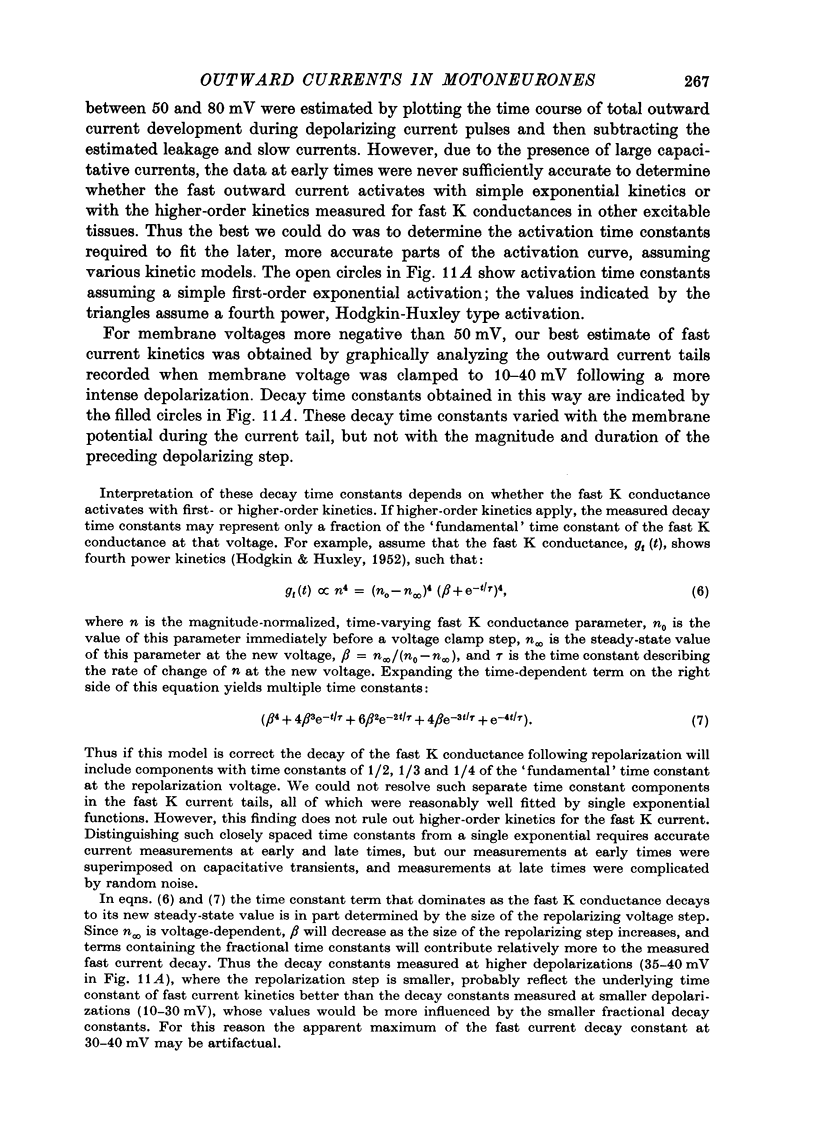
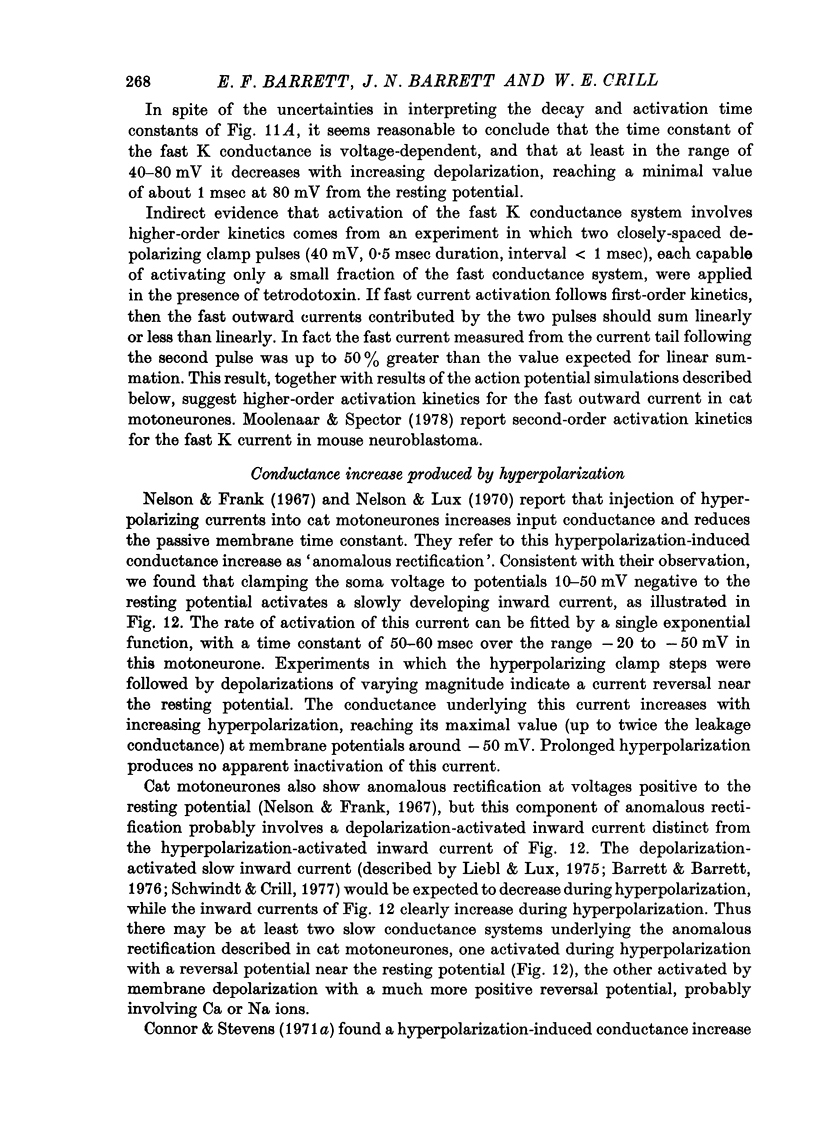
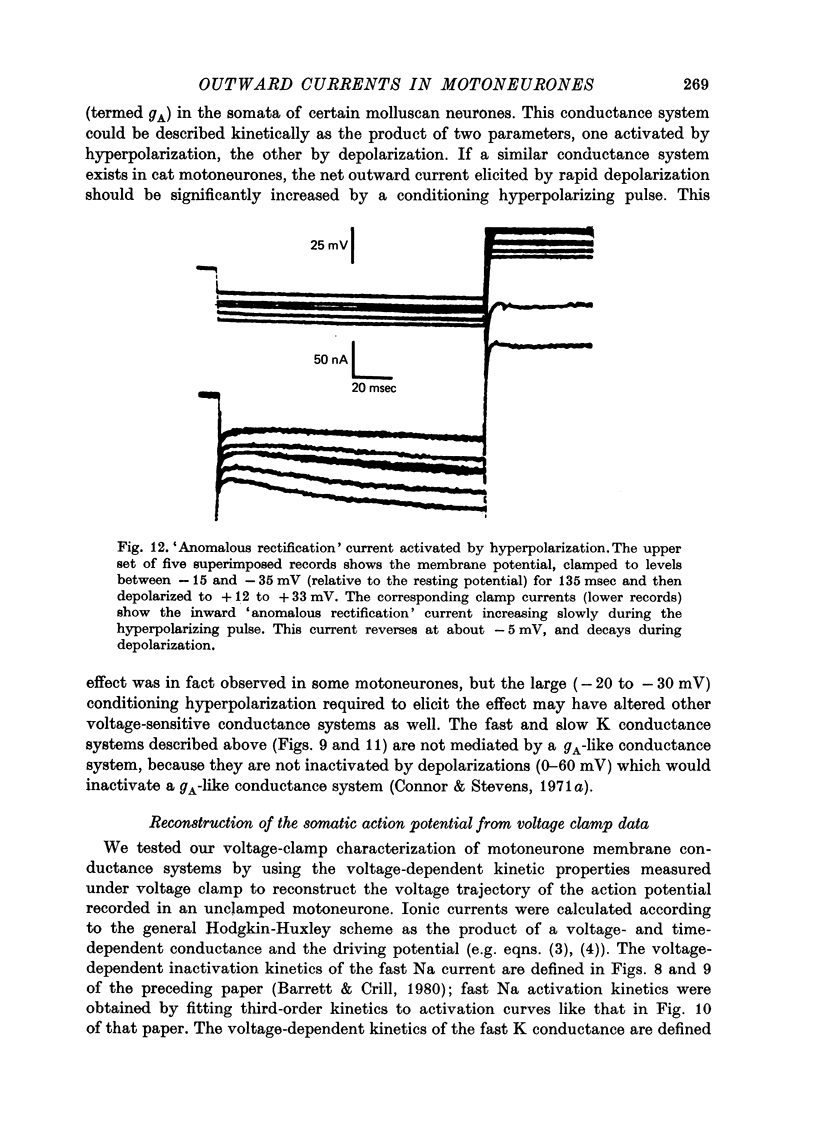
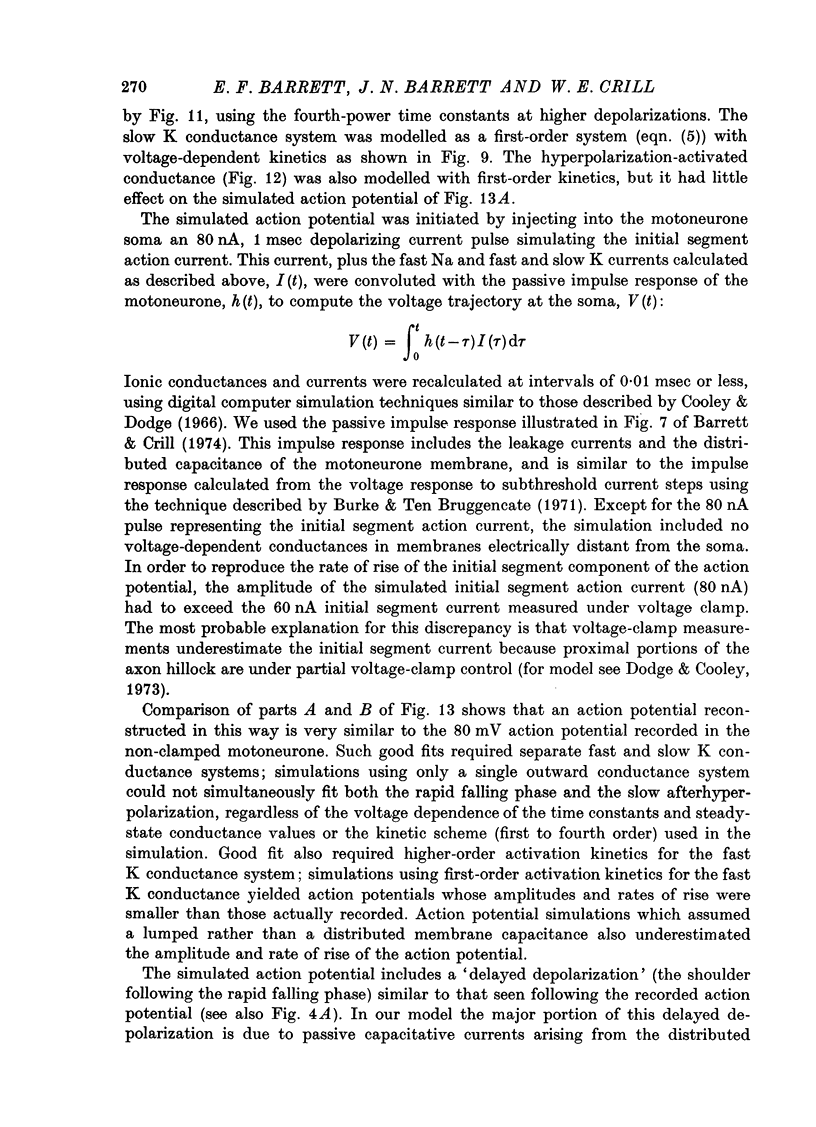
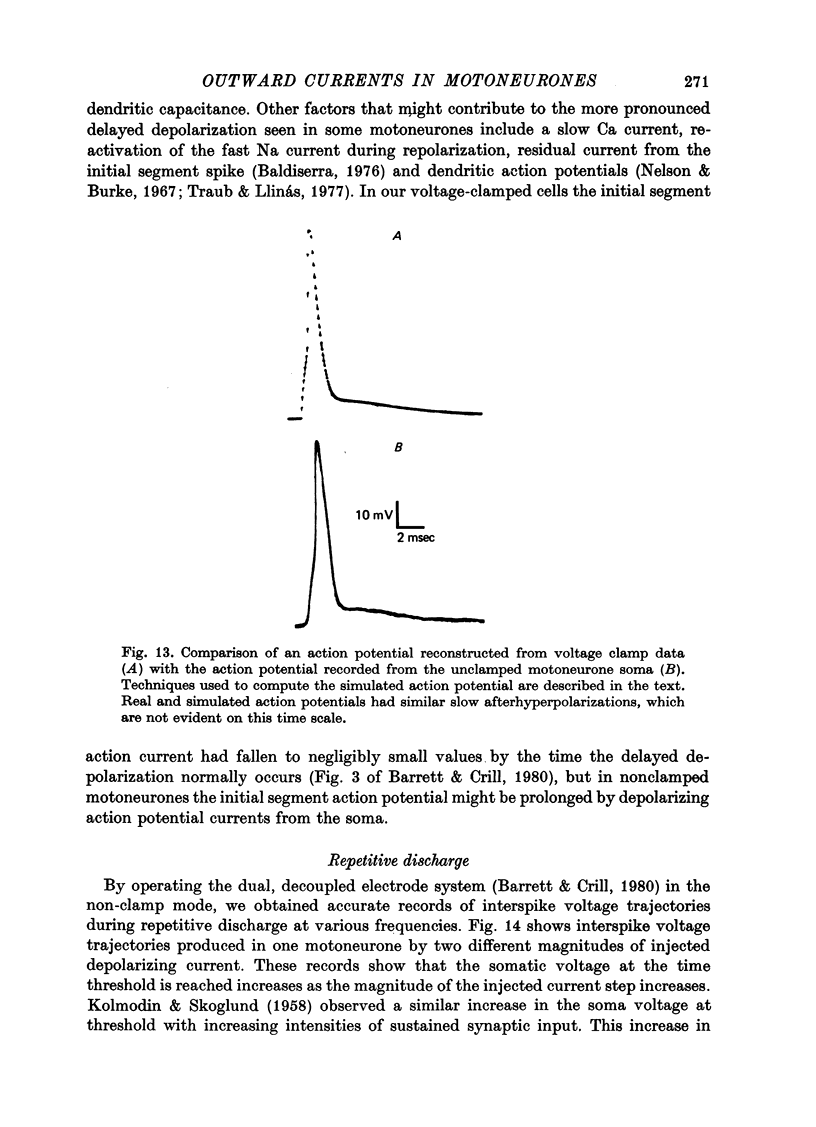
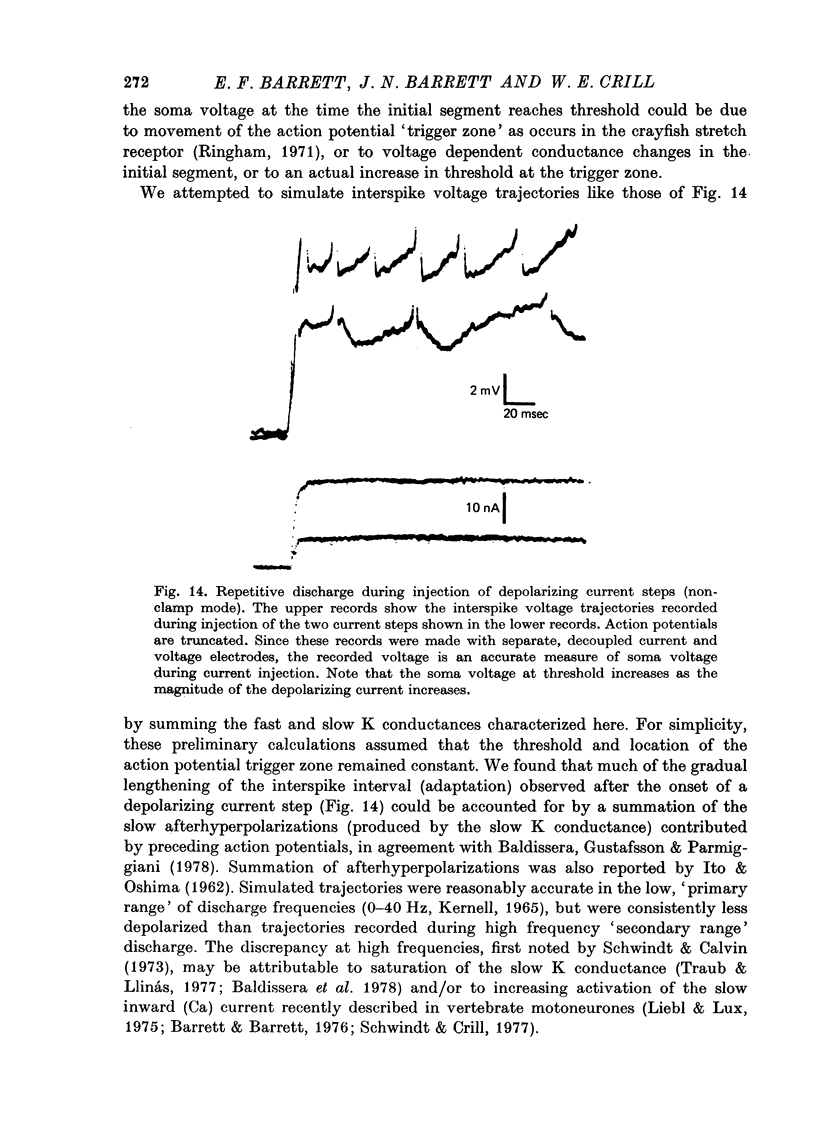
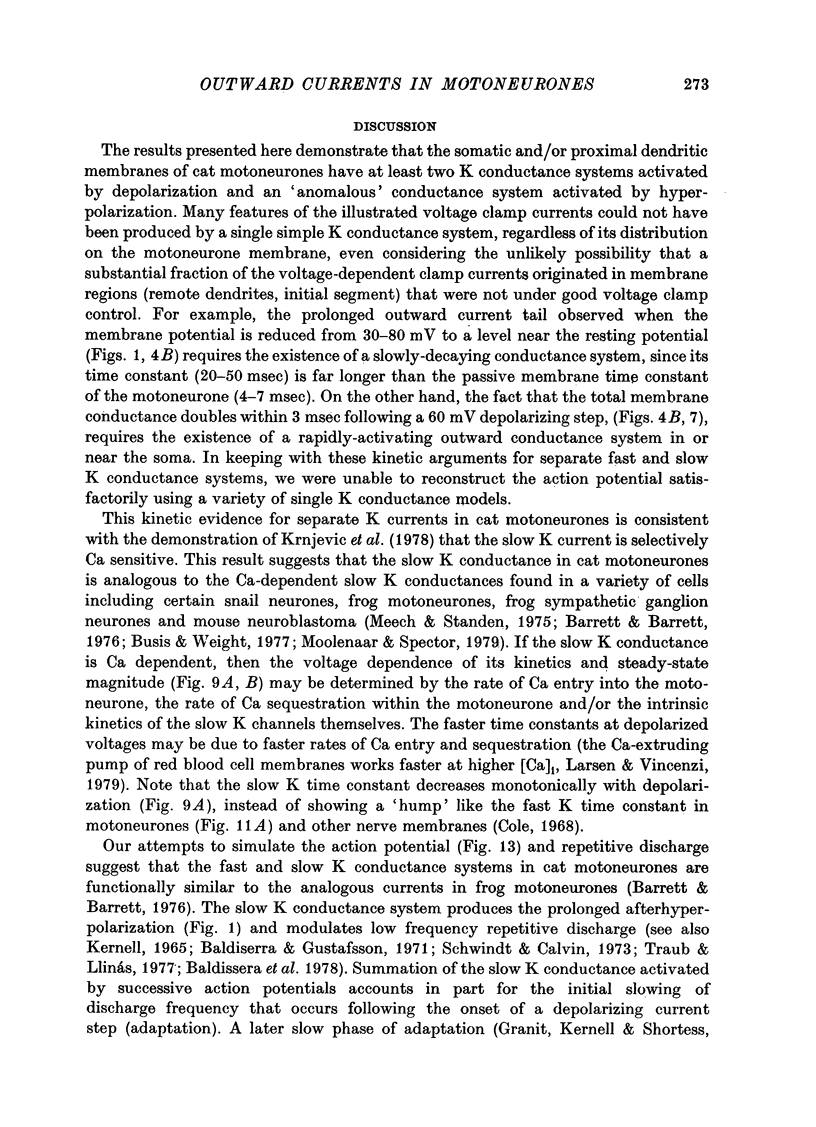
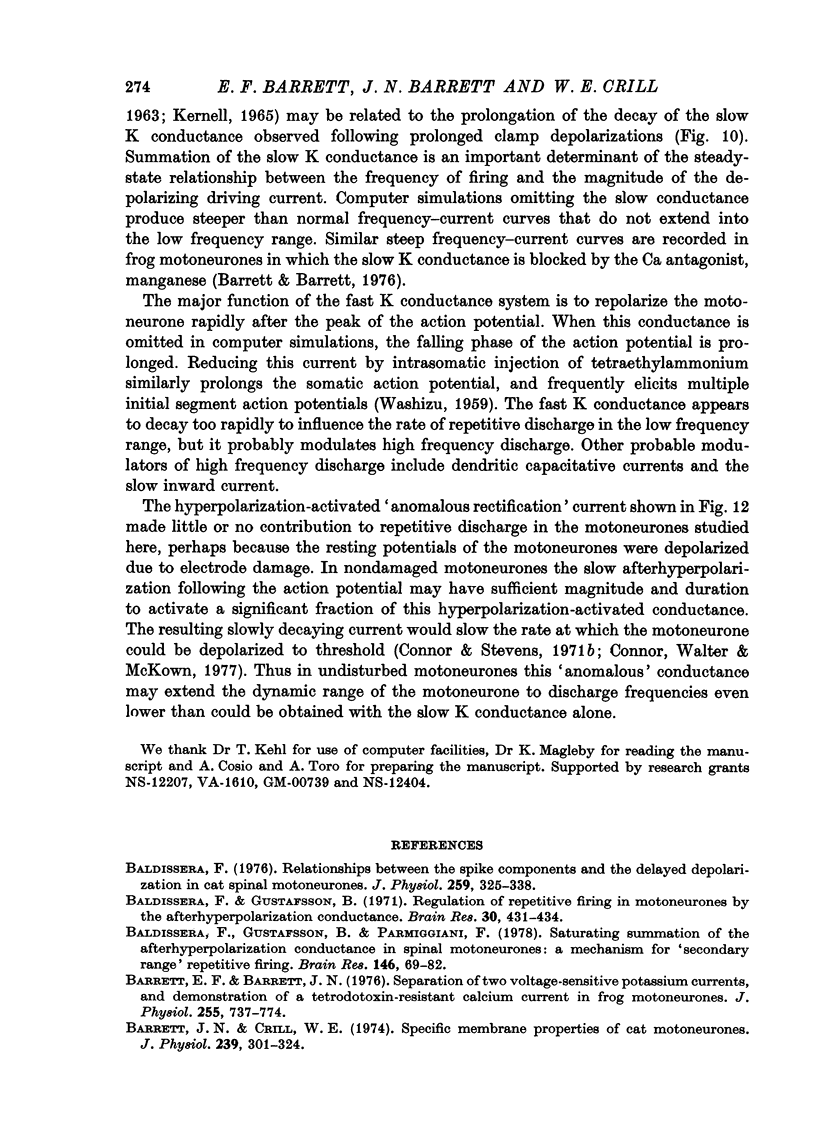
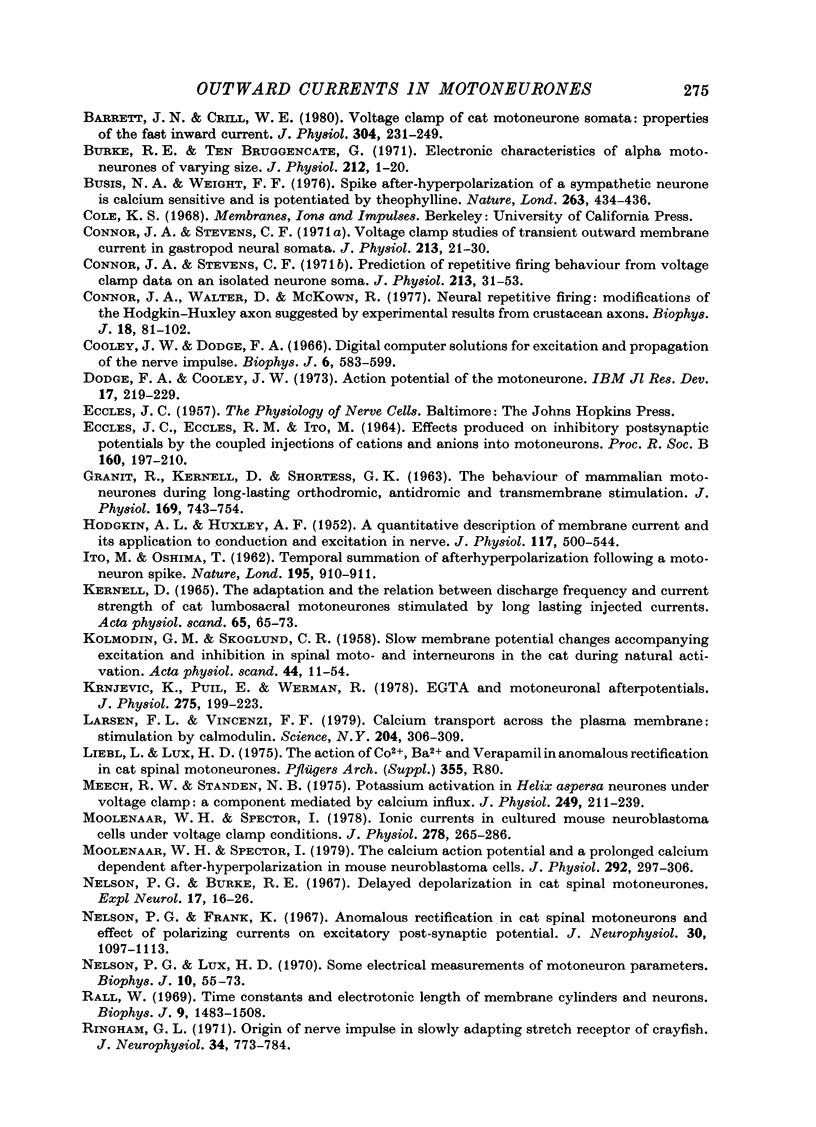
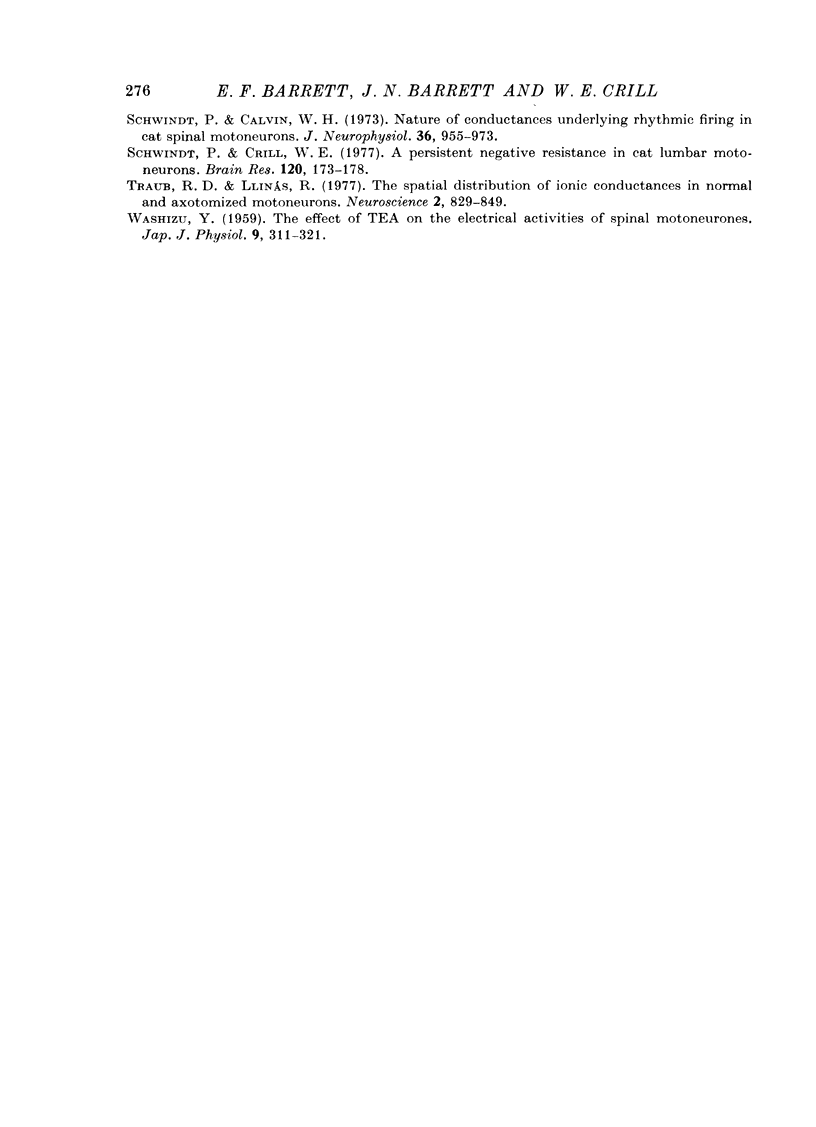
Selected References
These references are in PubMed. This may not be the complete list of references from this article.
- Baldissera F., Gustafsson B., Parmiggiani F. Saturating summation of the afterhyperpolarization conductance in spinal motoneurones: a mechanism for 'secondary range' repetitive firing. Brain Res. 1978 May 5;146(1):69–82. doi: 10.1016/0006-8993(78)90218-4. [DOI] [PubMed] [Google Scholar]
- Baldissera F., Gustafsson B. Regulation of repetitive firing in motoneurones by the afterhyperpolarization conductance. Brain Res. 1971 Jul 23;30(2):431–434. doi: 10.1016/0006-8993(71)90096-5. [DOI] [PubMed] [Google Scholar]
- Baldissera F. Relationships between the spike components and the delayed depolarization in cat spinal neurones. J Physiol. 1976 Jul;259(2):325–338. doi: 10.1113/jphysiol.1976.sp011468. [DOI] [PMC free article] [PubMed] [Google Scholar]
- Barrett E. F., Barret J. N. Separation of two voltage-sensitive potassium currents, and demonstration of a tetrodotoxin-resistant calcium current in frog motoneurones. J Physiol. 1976 Mar;255(3):737–774. doi: 10.1113/jphysiol.1976.sp011306. [DOI] [PMC free article] [PubMed] [Google Scholar]
- Barrett J. N., Crill W. E. Specific membrane properties of cat motoneurones. J Physiol. 1974 Jun;239(2):301–324. doi: 10.1113/jphysiol.1974.sp010570. [DOI] [PMC free article] [PubMed] [Google Scholar]
- Barrett J. N., Crill W. E. Voltage clamp of cat motoneurone somata: properties of the fast inward current. J Physiol. 1980 Jul;304:231–249. doi: 10.1113/jphysiol.1980.sp013322. [DOI] [PMC free article] [PubMed] [Google Scholar]
- Busis N. A., Weight F. F. Spike after-hyperpolarisation of a sympathetic neurone is calcium sensitive and is potentiated by theophylline. Nature. 1976 Sep 30;263(5576):434–436. doi: 10.1038/263434a0. [DOI] [PubMed] [Google Scholar]
- Connor J. A., Stevens C. F. Prediction of repetitive firing behaviour from voltage clamp data on an isolated neurone soma. J Physiol. 1971 Feb;213(1):31–53. doi: 10.1113/jphysiol.1971.sp009366. [DOI] [PMC free article] [PubMed] [Google Scholar]
- Connor J. A., Stevens C. F. Voltage clamp studies of a transient outward membrane current in gastropod neural somata. J Physiol. 1971 Feb;213(1):21–30. doi: 10.1113/jphysiol.1971.sp009365. [DOI] [PMC free article] [PubMed] [Google Scholar]
- Connor J. A., Walter D., McKown R. Neural repetitive firing: modifications of the Hodgkin-Huxley axon suggested by experimental results from crustacean axons. Biophys J. 1977 Apr;18(1):81–102. doi: 10.1016/S0006-3495(77)85598-7. [DOI] [PMC free article] [PubMed] [Google Scholar]
- Cooley J. W., Dodge F. A., Jr Digital computer solutions for excitation and propagation of the nerve impulse. Biophys J. 1966 Sep;6(5):583–599. doi: 10.1016/S0006-3495(66)86679-1. [DOI] [PMC free article] [PubMed] [Google Scholar]
- ECCLES J., ECCLES R. M., ITO M. EFFECTS PRODUCED ON INHIBITORY POSTSYNAPTIC POTENTIALS BY THE COUPLED INJECTIONS OF CATIONS AND ANIONS INTO MOTONEURONS. Proc R Soc Lond B Biol Sci. 1964 May 19;160:197–210. doi: 10.1098/rspb.1964.0036. [DOI] [PubMed] [Google Scholar]
- GRANIT R., KERNELL D., SHORTESS G. K. THE BEHAVIOUR OF MAMMALIAN MOTONEURONES DURING LONG-LASTING ORTHODROMIC, ANTIDROMIC AND TRANS-MEMBRANE STIMULATION. J Physiol. 1963 Dec;169:743–754. doi: 10.1113/jphysiol.1963.sp007293. [DOI] [PMC free article] [PubMed] [Google Scholar]
- HODGKIN A. L., HUXLEY A. F. A quantitative description of membrane current and its application to conduction and excitation in nerve. J Physiol. 1952 Aug;117(4):500–544. doi: 10.1113/jphysiol.1952.sp004764. [DOI] [PMC free article] [PubMed] [Google Scholar]
- KOLMODIN G. M., SKOGLUND C. R. Slow membrane potential changes accompanying excitation and inhibition in spinal moto- and interneurons in the cat during natural activation. Acta Physiol Scand. 1958 Oct 28;44(1):11–54. doi: 10.1111/j.1748-1716.1958.tb01607.x. [DOI] [PubMed] [Google Scholar]
- Krnjević K., Puil E., Werman R. EGTA and motoneuronal after-potentials. J Physiol. 1978 Feb;275:199–223. doi: 10.1113/jphysiol.1978.sp012186. [DOI] [PMC free article] [PubMed] [Google Scholar]
- Larsen F. L., Vincenzi F. F. Calcium transport across the plasma membrane: stimulation by calmodulin. Science. 1979 Apr 20;204(4390):306–309. doi: 10.1126/science.155309. [DOI] [PubMed] [Google Scholar]
- Meech R. W., Standen N. B. Potassium activation in Helix aspersa neurones under voltage clamp: a component mediated by calcium influx. J Physiol. 1975 Jul;249(2):211–239. doi: 10.1113/jphysiol.1975.sp011012. [DOI] [PMC free article] [PubMed] [Google Scholar]
- Moolenaar W. H., Spector I. Ionic currents in cultured mouse neuroblastoma cells under voltage-clamp conditions. J Physiol. 1978 May;278:265–286. doi: 10.1113/jphysiol.1978.sp012303. [DOI] [PMC free article] [PubMed] [Google Scholar]
- Moolenaar W. H., Spector I. The calcium action potential and a prolonged calcium dependent after-hyperpolarization in mouse neuroblastoma cells. J Physiol. 1979 Jul;292:297–306. doi: 10.1113/jphysiol.1979.sp012851. [DOI] [PMC free article] [PubMed] [Google Scholar]
- Nelson P. G., Burke R. E. Delayed depolarization in cat spinal motoneurons. Exp Neurol. 1967 Jan;17(1):16–26. doi: 10.1016/0014-4886(67)90118-5. [DOI] [PubMed] [Google Scholar]
- Nelson P. G., Frank K. Anomalous rectification in cat spinal motoneurons and effect of polarizing currents on excitatory postsynaptic potential. J Neurophysiol. 1967 Sep;30(5):1097–1113. doi: 10.1152/jn.1967.30.5.1097. [DOI] [PubMed] [Google Scholar]
- Nelson P. G., Lux H. D. Some electrical measurements of motoneuron parameters. Biophys J. 1970 Jan;10(1):55–73. doi: 10.1016/S0006-3495(70)86285-3. [DOI] [PMC free article] [PubMed] [Google Scholar]
- Rall W. Time constants and electrotonic length of membrane cylinders and neurons. Biophys J. 1969 Dec;9(12):1483–1508. doi: 10.1016/S0006-3495(69)86467-2. [DOI] [PMC free article] [PubMed] [Google Scholar]
- Ringham G. L. Origin of nerve impulse in slowly adapting stretch receptor of crayfish. J Neurophysiol. 1971 Sep;34(5):773–784. doi: 10.1152/jn.1971.34.5.773. [DOI] [PubMed] [Google Scholar]
- Schwindt P. C., Calvin W. H. Nature of conductances underlying rhythmic firing in cat spinal motoneurons. J Neurophysiol. 1973 Nov;36(6):955–973. doi: 10.1152/jn.1973.36.6.955. [DOI] [PubMed] [Google Scholar]
- Schwindt P., Crill W. E. A persistent negative resistance in cat lumbar motoneurons. Brain Res. 1977 Jan 14;120(1):173–178. doi: 10.1016/0006-8993(77)90510-8. [DOI] [PubMed] [Google Scholar]
- WASHIZU Y. The effect of TEA on the electrical activities of spinal motoneurons. Jpn J Physiol. 1959 Sep 15;9:311–321. doi: 10.2170/jjphysiol.9.311. [DOI] [PubMed] [Google Scholar]