Abstract
1. The vaseline-gap voltage-clamp method has been applied to the study of ionic currents in cut pieces from innervated and 5--7 days denervated rat skeletal muscle fibres. 2. Kinetic analysis of sodium currents in innervated rat muscle showed them to be similar to those in frog muscle, except that rat sodium channels are activated at slightly more negative potentials. Peak sodium conductances of 40--50 m-mho/cm2 were measured, corresponding to values of GNa of 100--120 m-mho/cm2. 3. The permeability sequence of the sodium channel to several organic and inorganic cations is Li+ greater than Na+ greater than hydroxylammonium greater than hydrazinium greater than guanidinium approximately ammonium greater than K+. TMA+ and Ca2+ were not measurably permeant. 4. Denervation appears to shift activation and inactivation parameters of sodium currents by approximately 10 mV to more negative potentials, but does not appreciably affect the maximum peak sodium conductance or the time constants for activation and inactivation. 5. Dose--response curves for block by tetrodotoxin in innervated fibres are fitted well by assuming binding of toxin to a single population of channels with a dissociation constant of about 5 nM. In denervated fibres there appears in addition a second population of channels with a dissociation constant in the micromolar range. These relatively toxin-insensitive channels respond less rapidly to potential changes, and can contribute up to 25--30% of the total sodium conductance. 6. The potassium currents of innervated rat muscle were similar to those of frog muscle in their voltage dependence of activation. 7. The time constant for inactivation of the sodium current, tau h, at -13 mV showed a temperature dependence measured between 10 and 20 degrees C equivalent to an average Q10 of 2 . 3. The Q10 for the time constant of activation of the potassium current tau n, averaged 2 . 5 between -40 mV and +40 mV, measured over the same temperature range.
Full text
PDF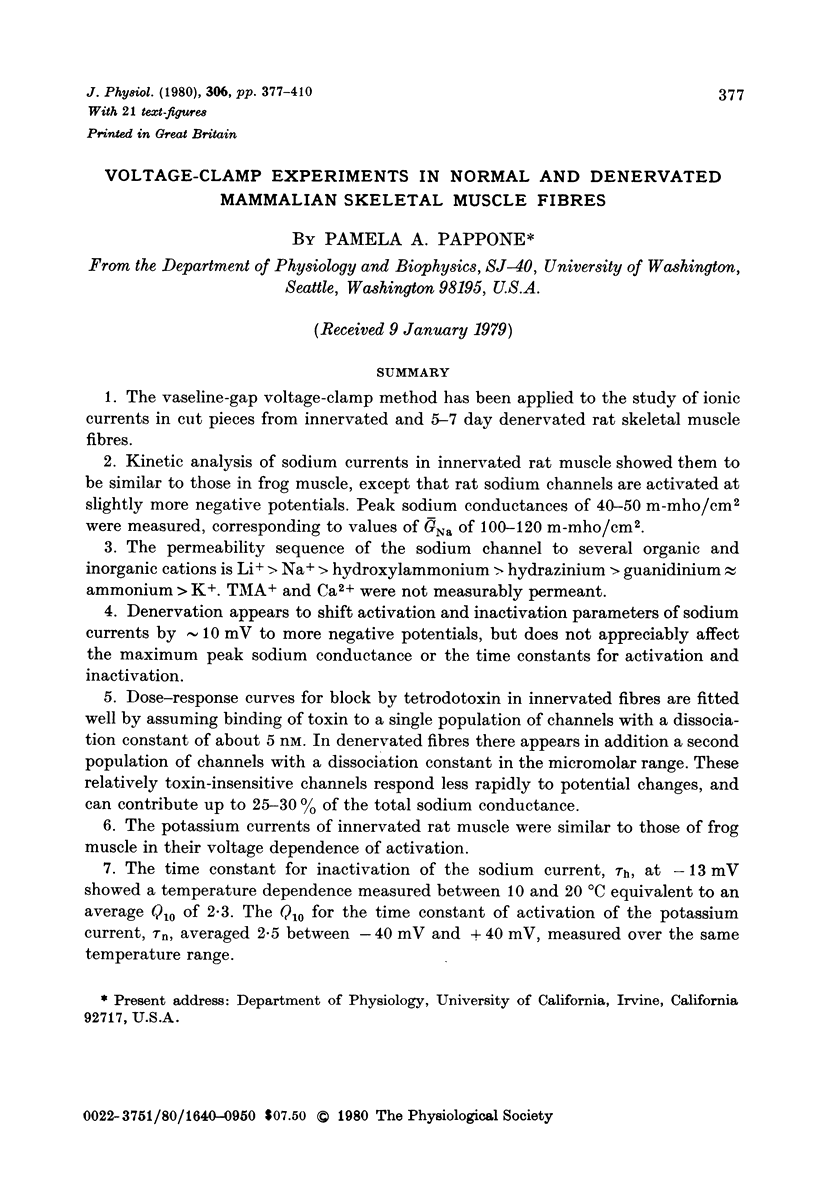
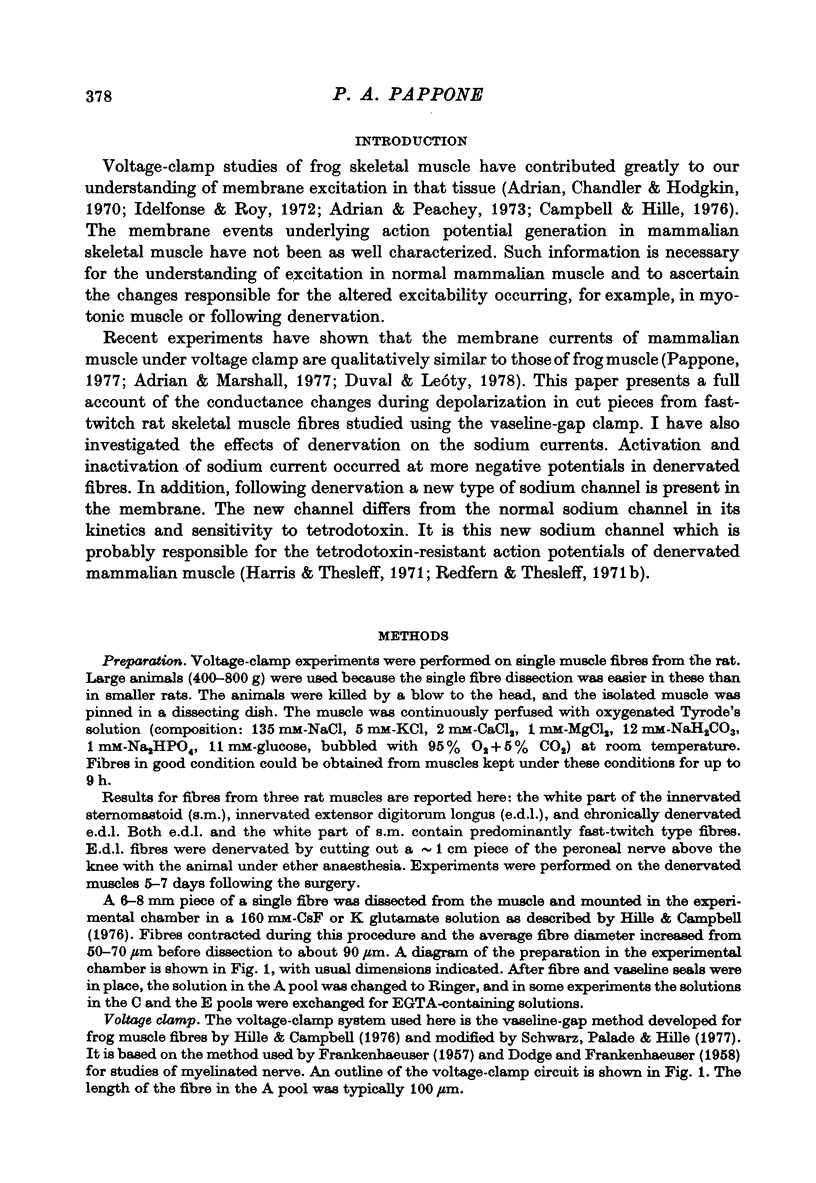
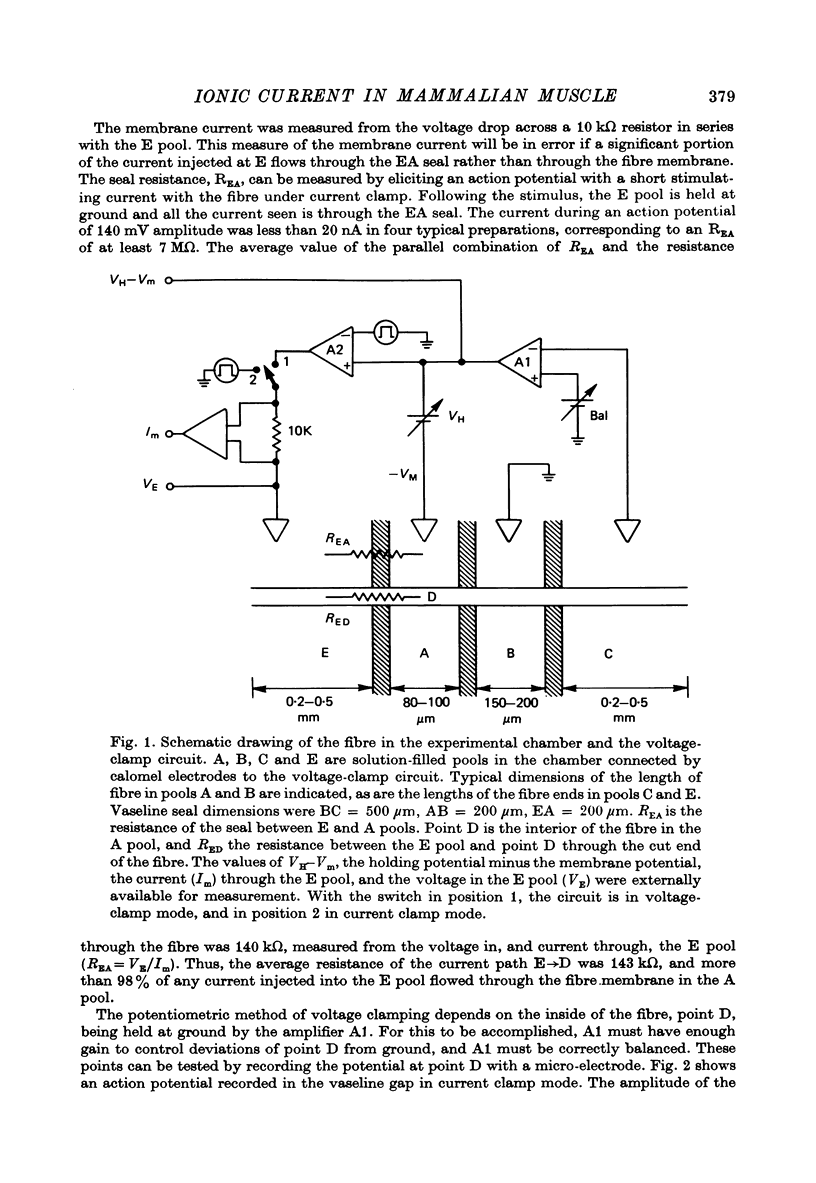
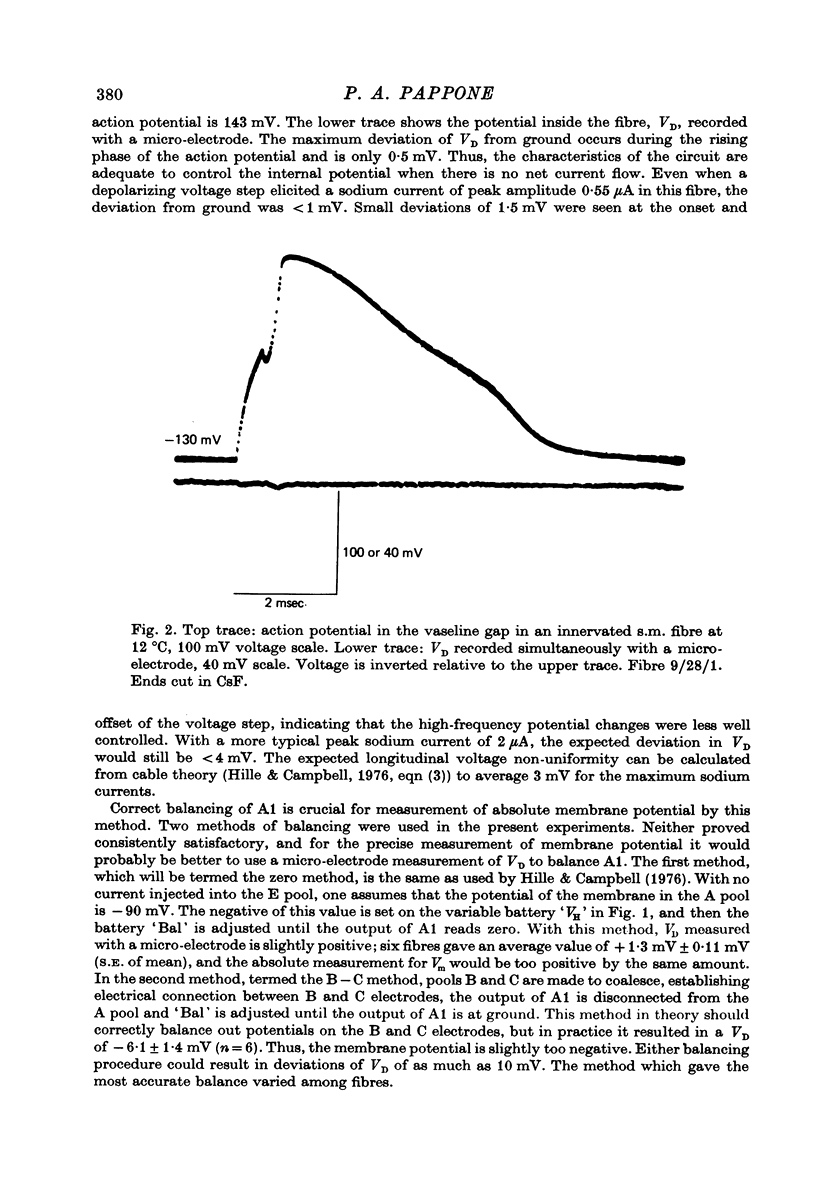
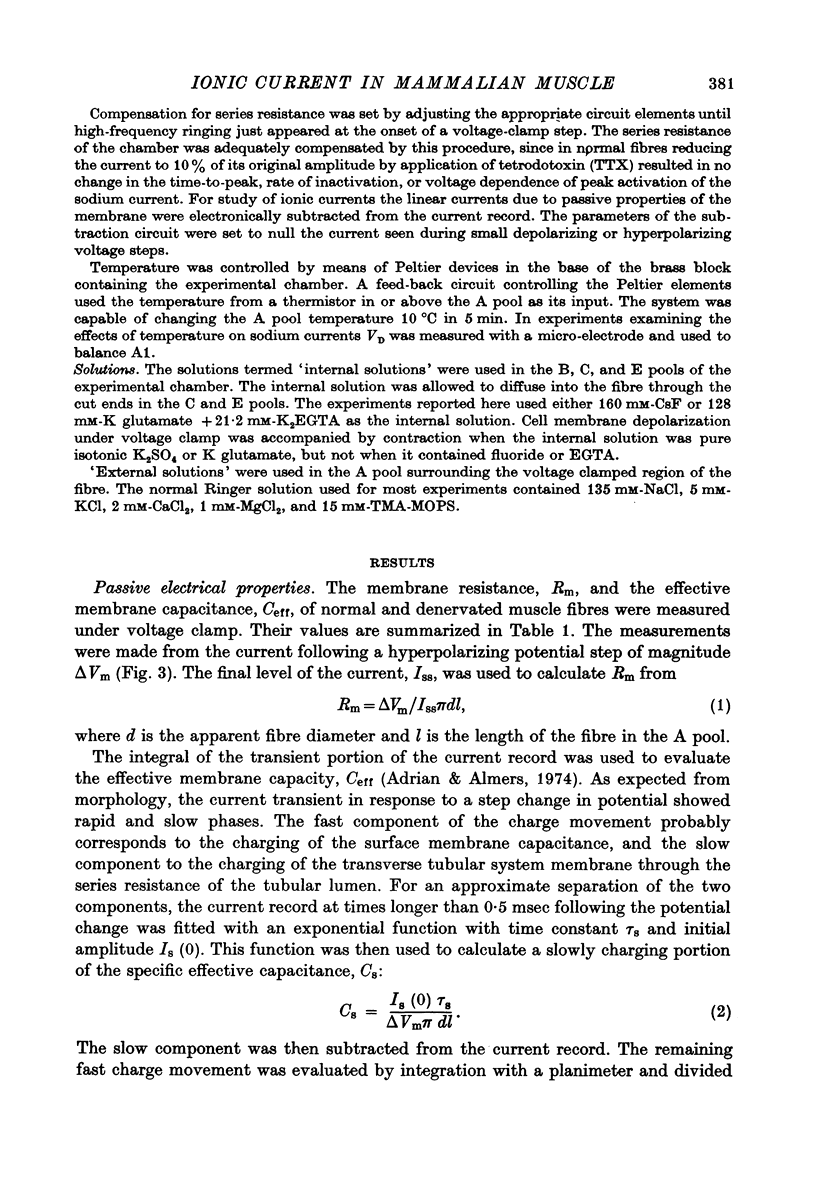
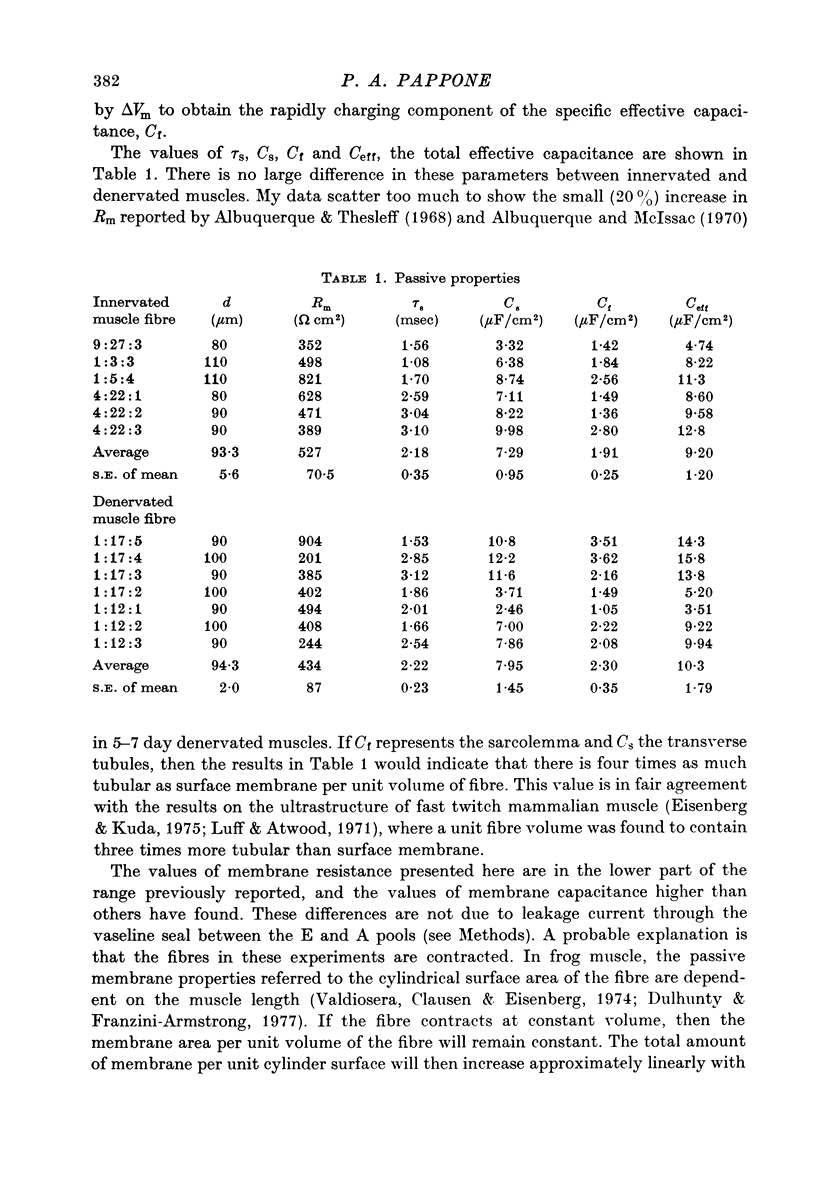
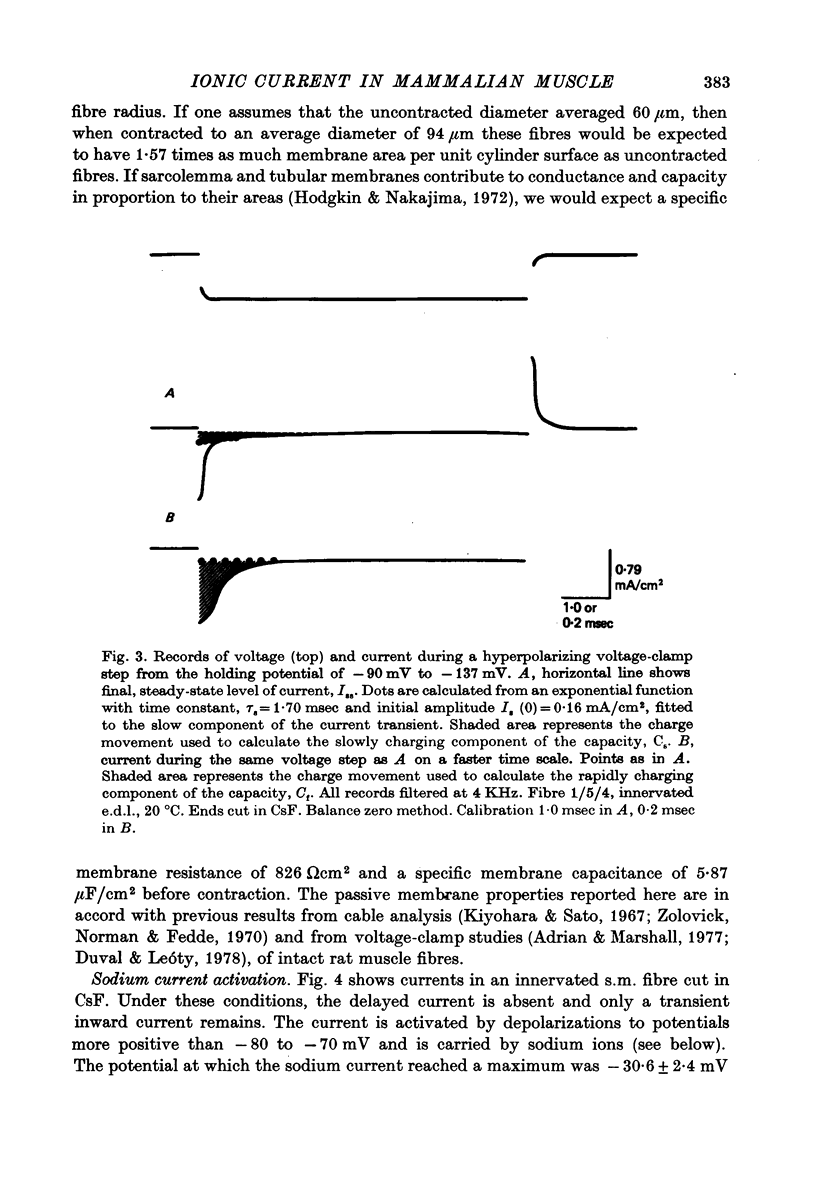
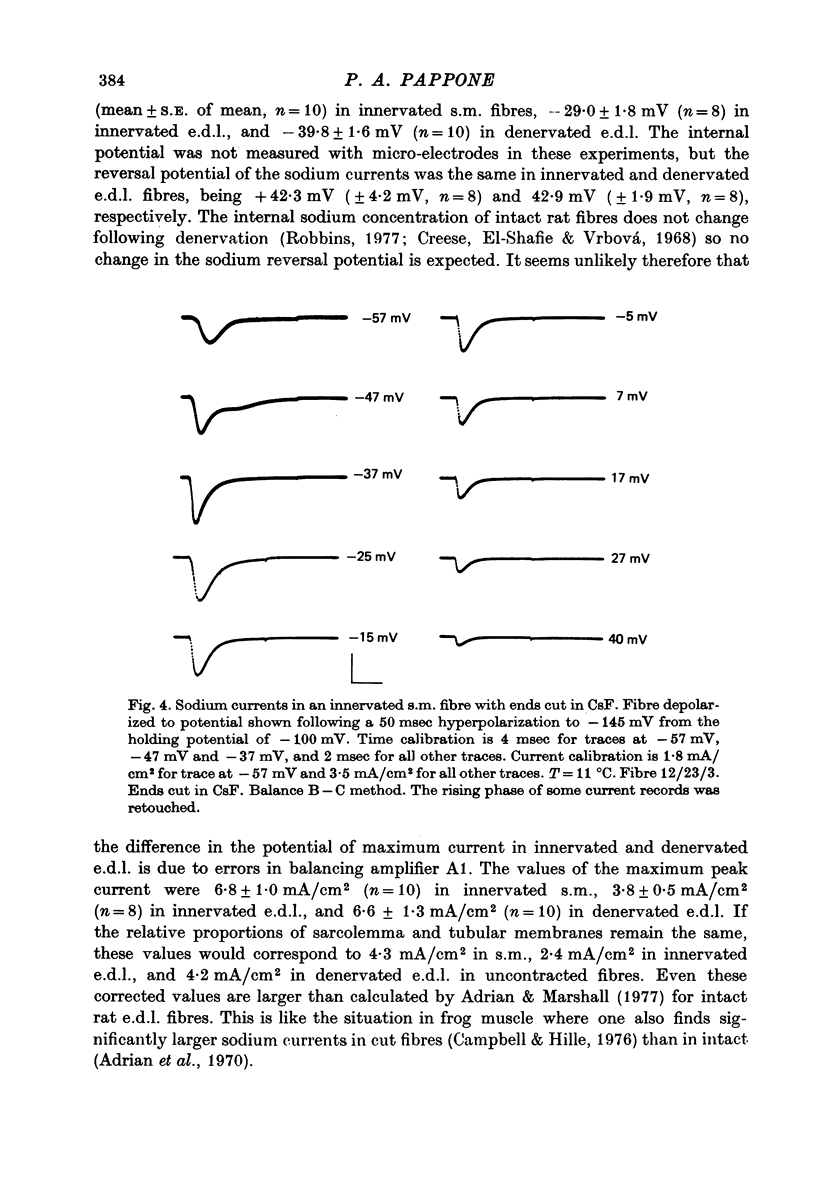
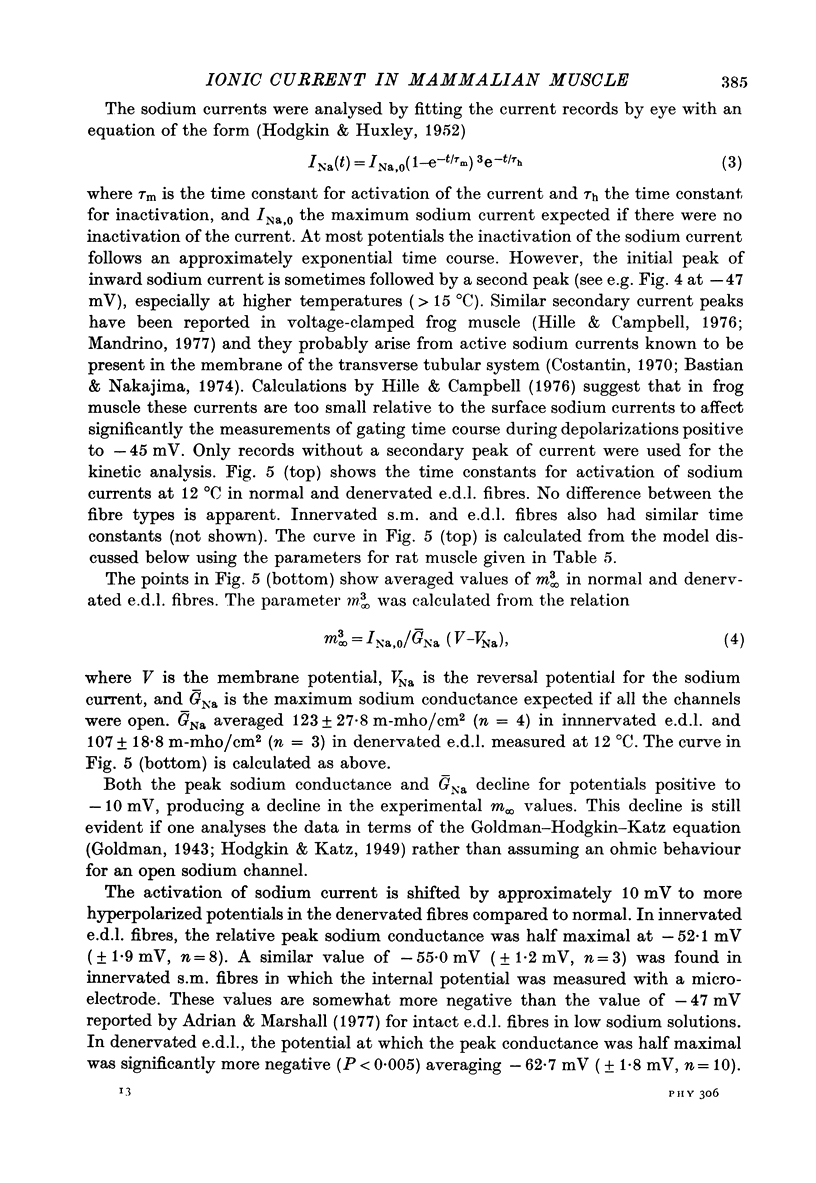
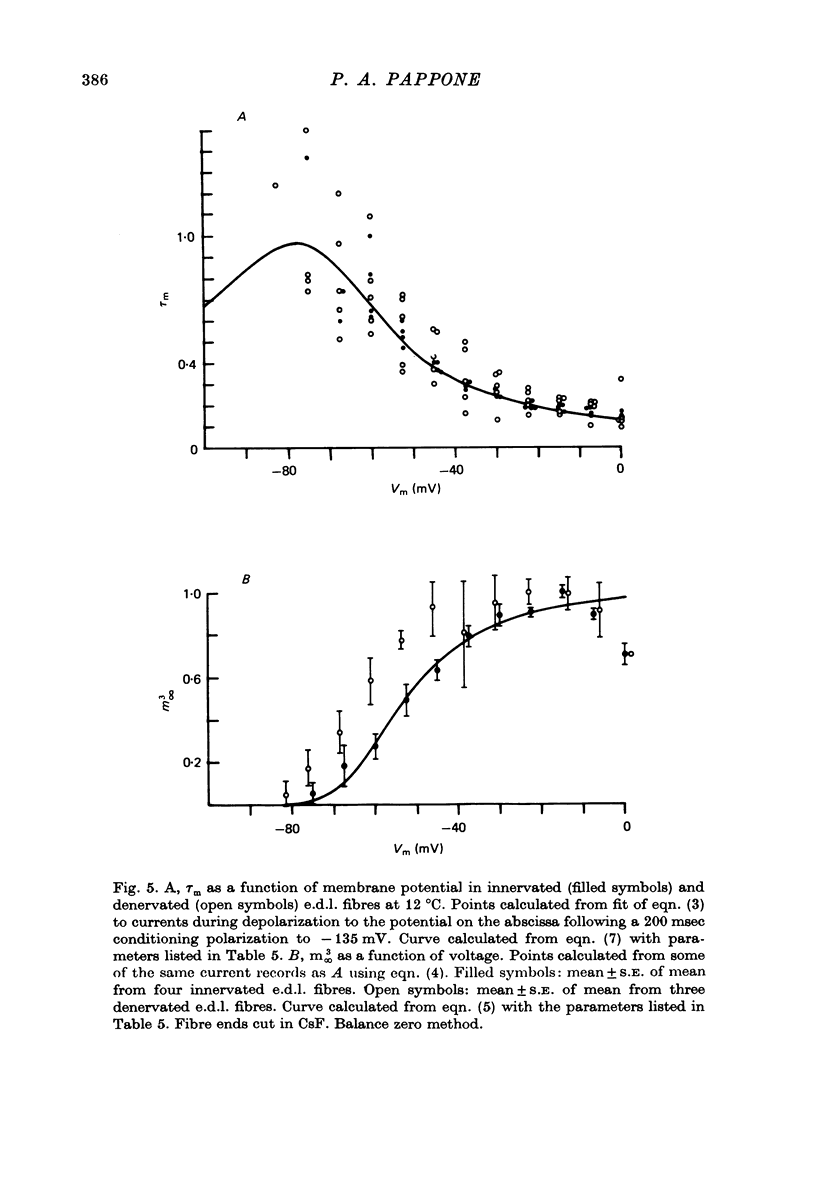
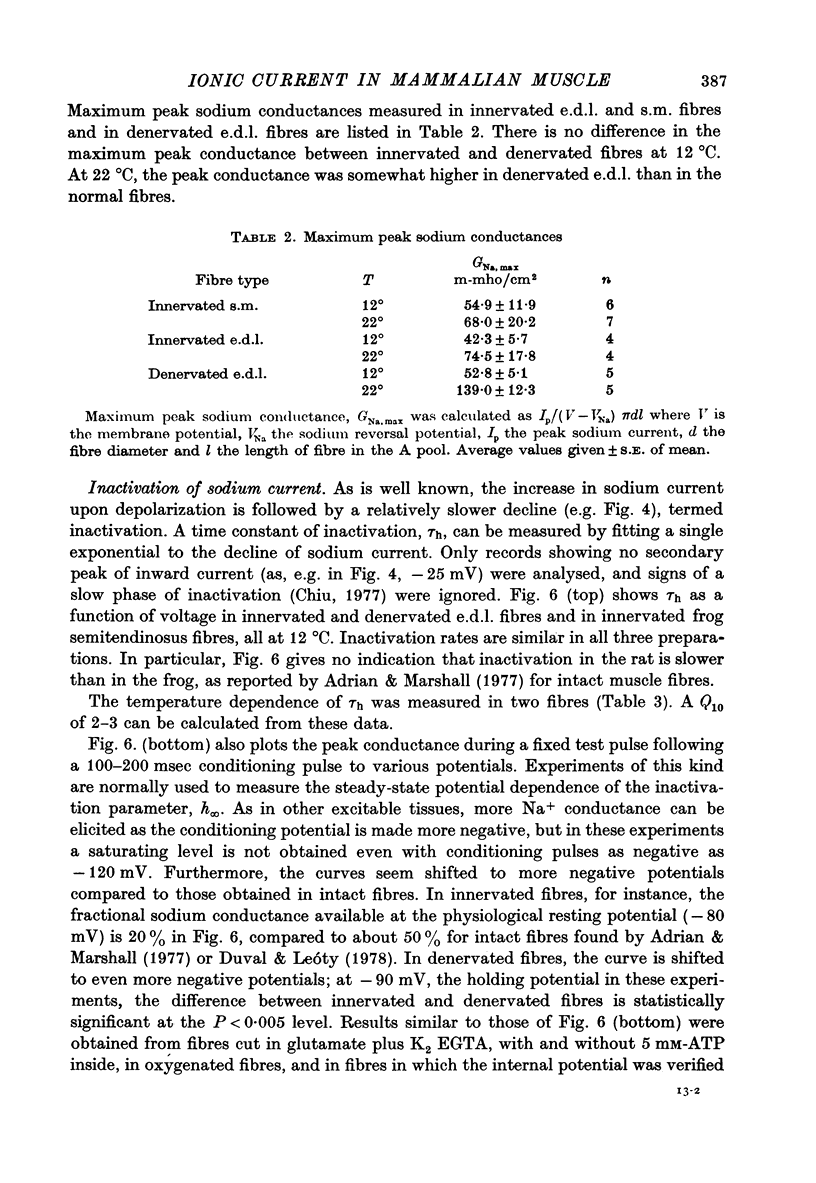
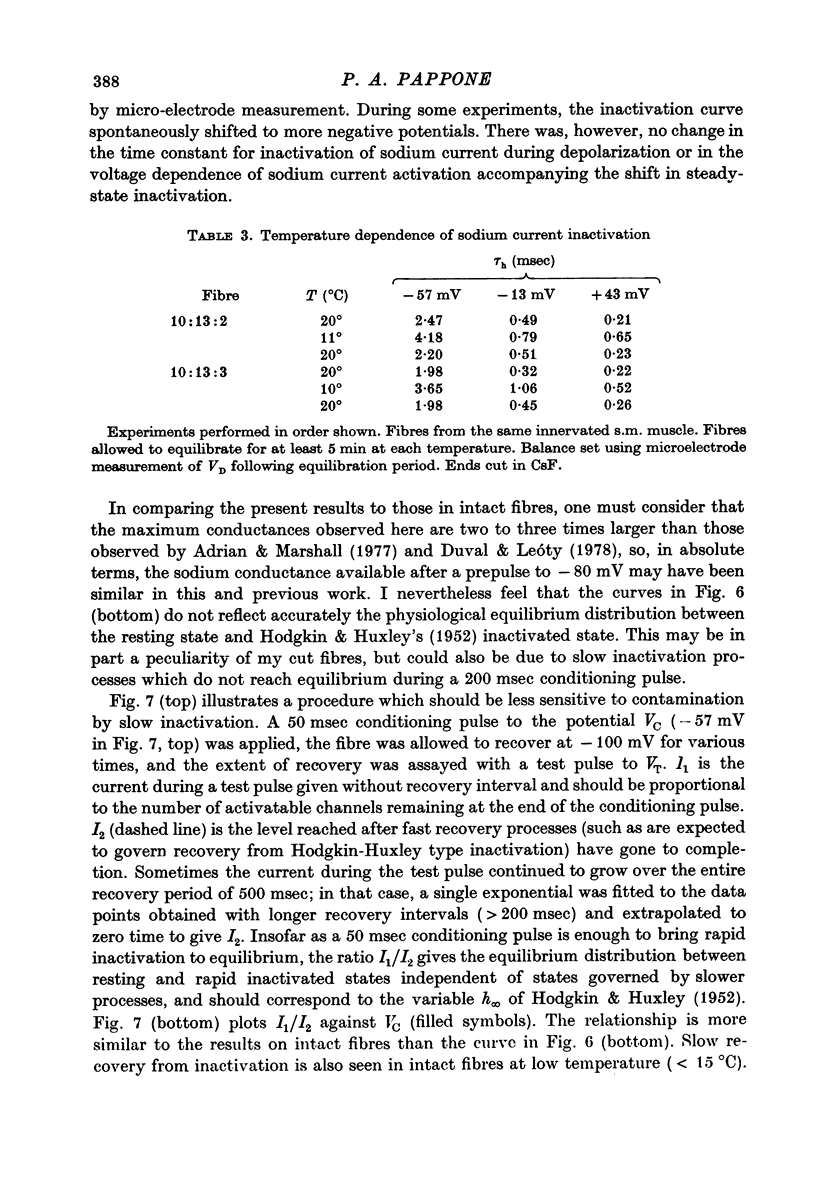
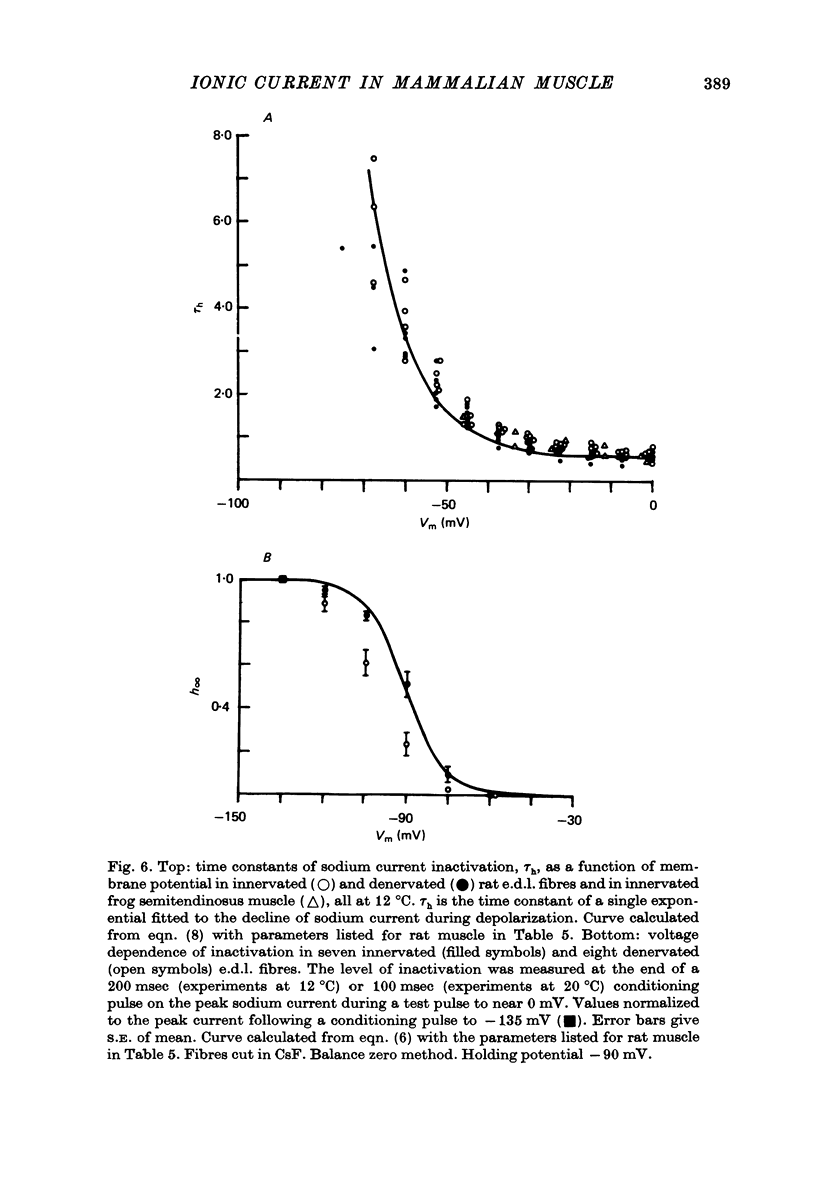
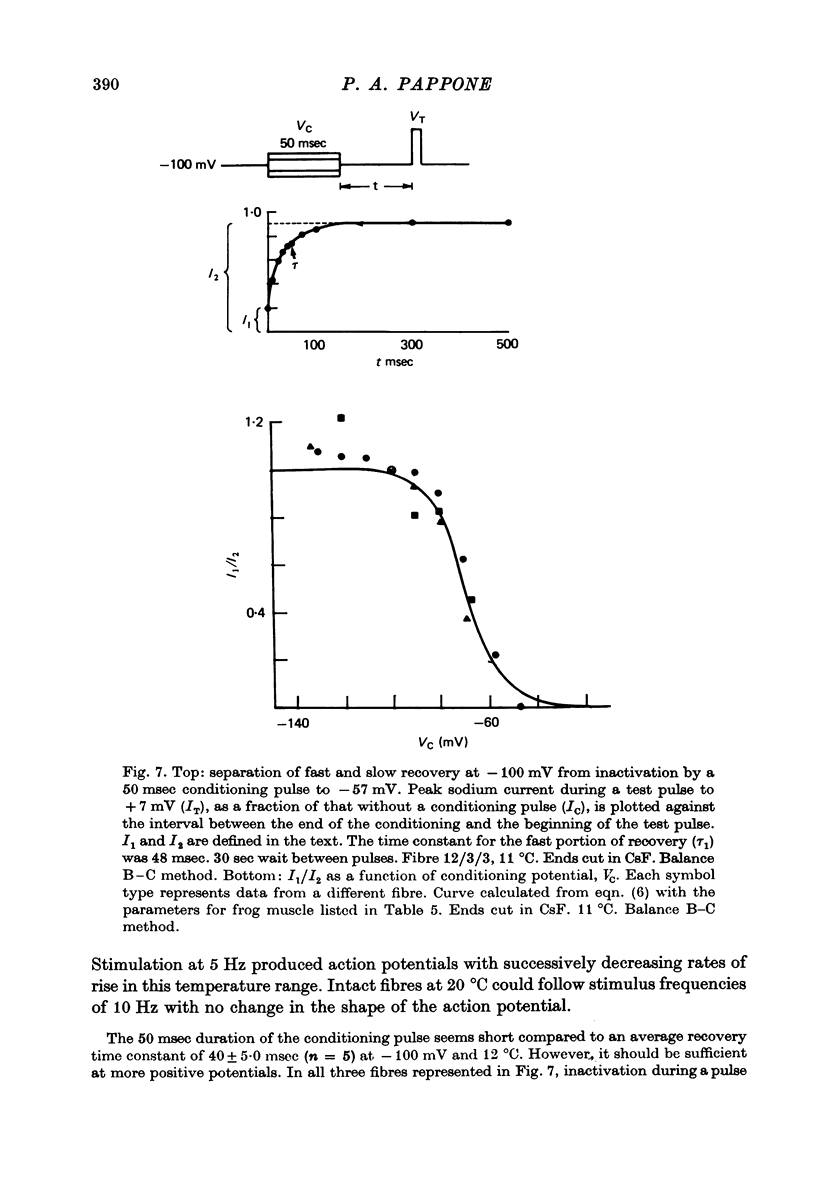
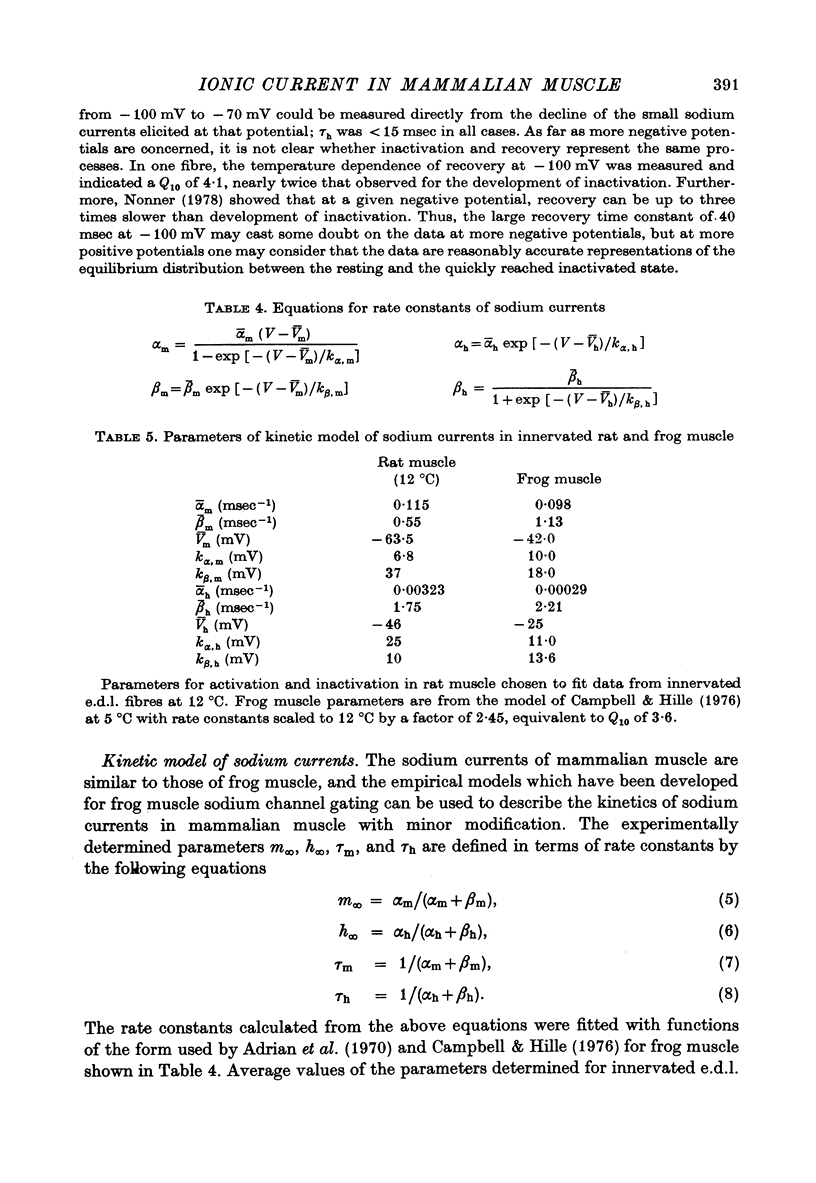
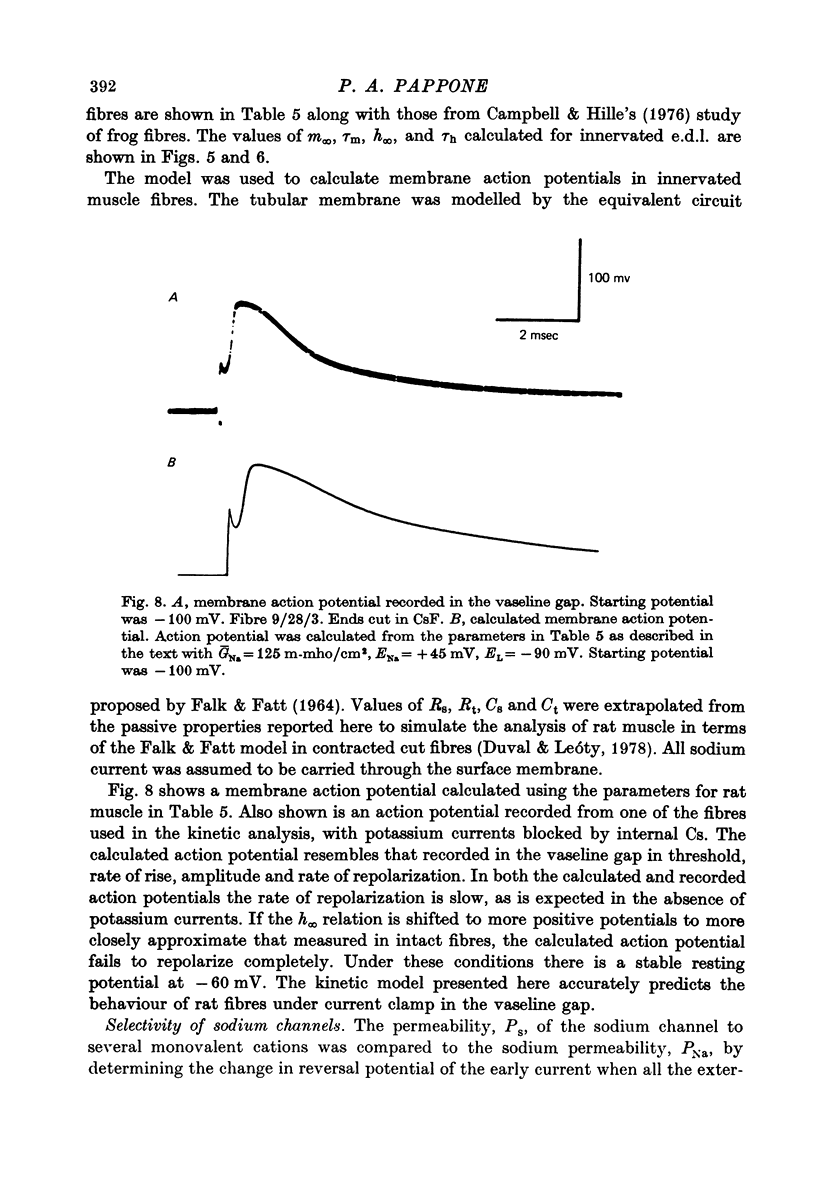
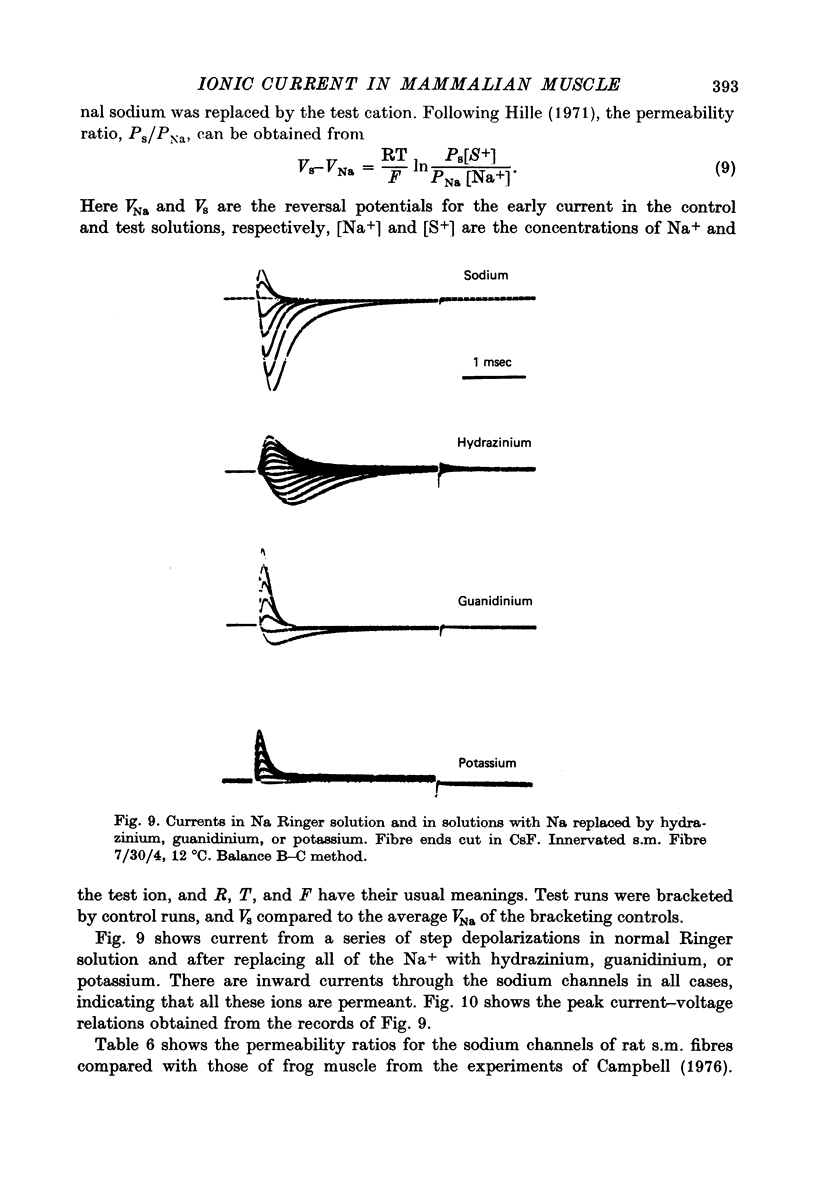
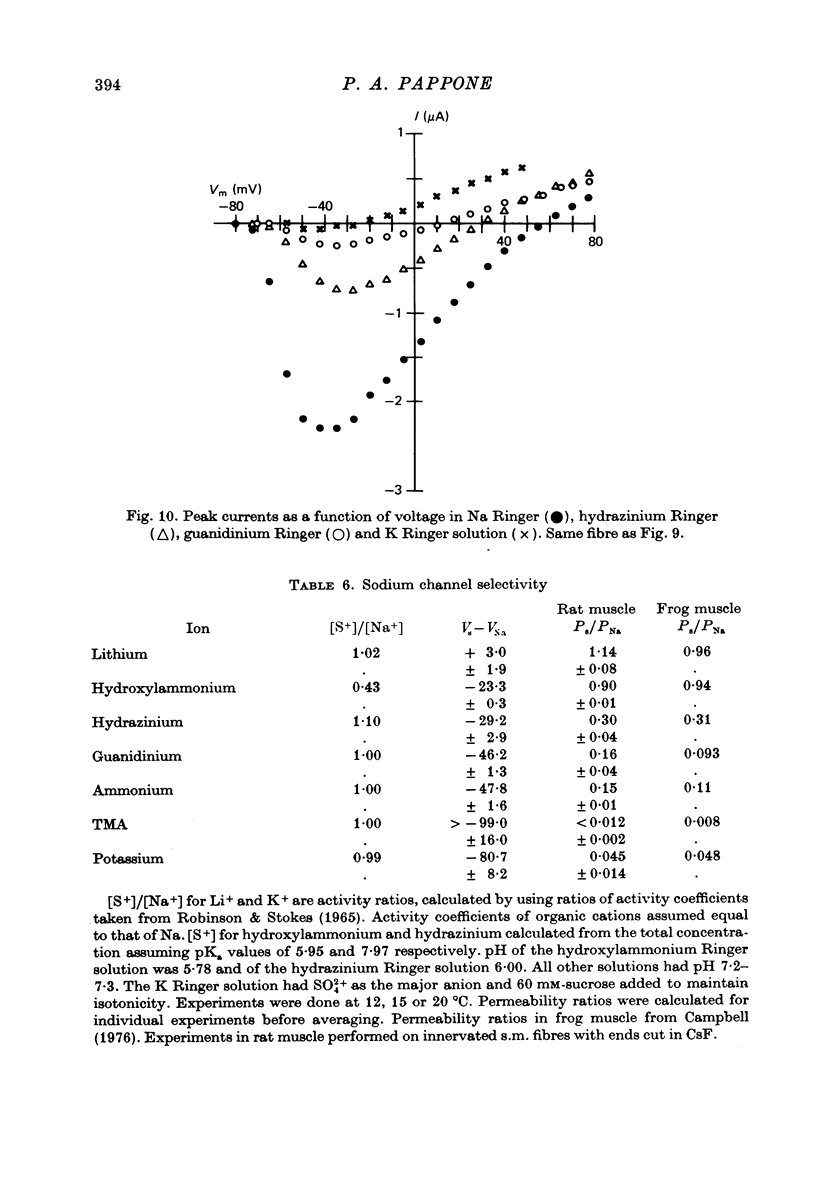
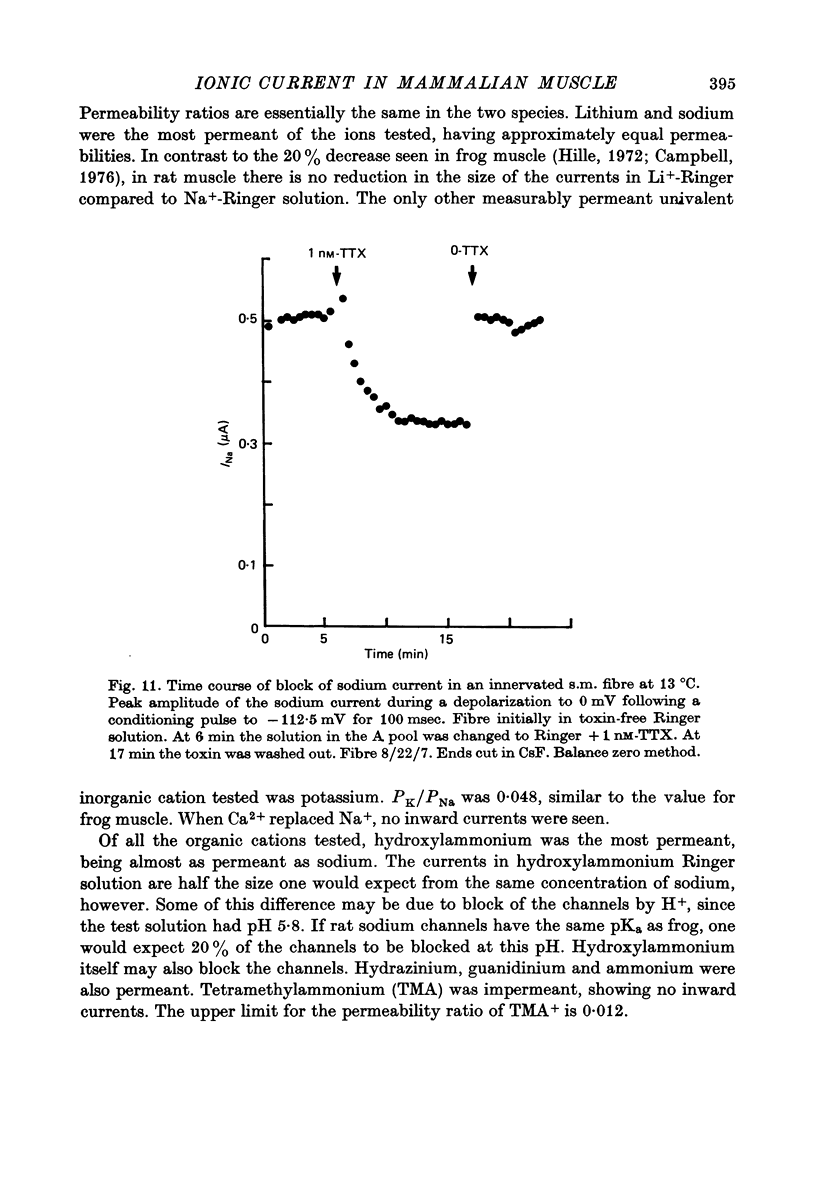
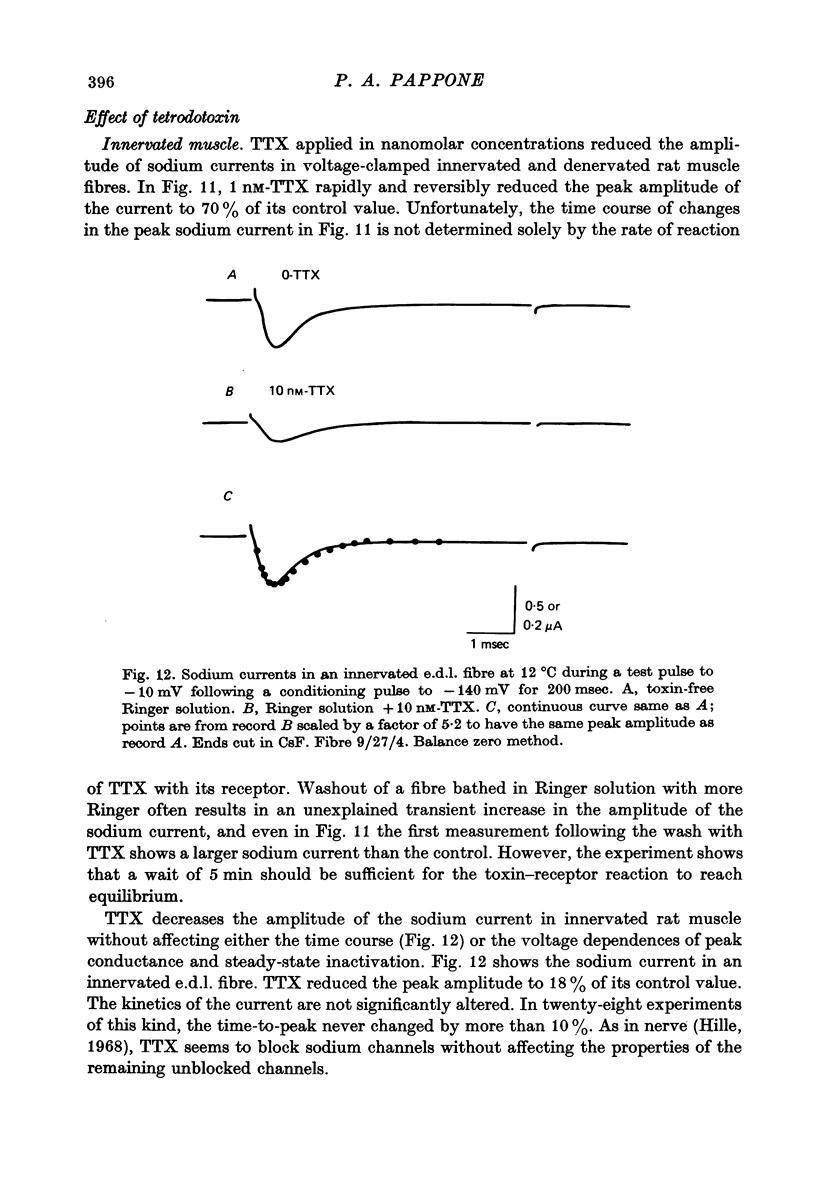
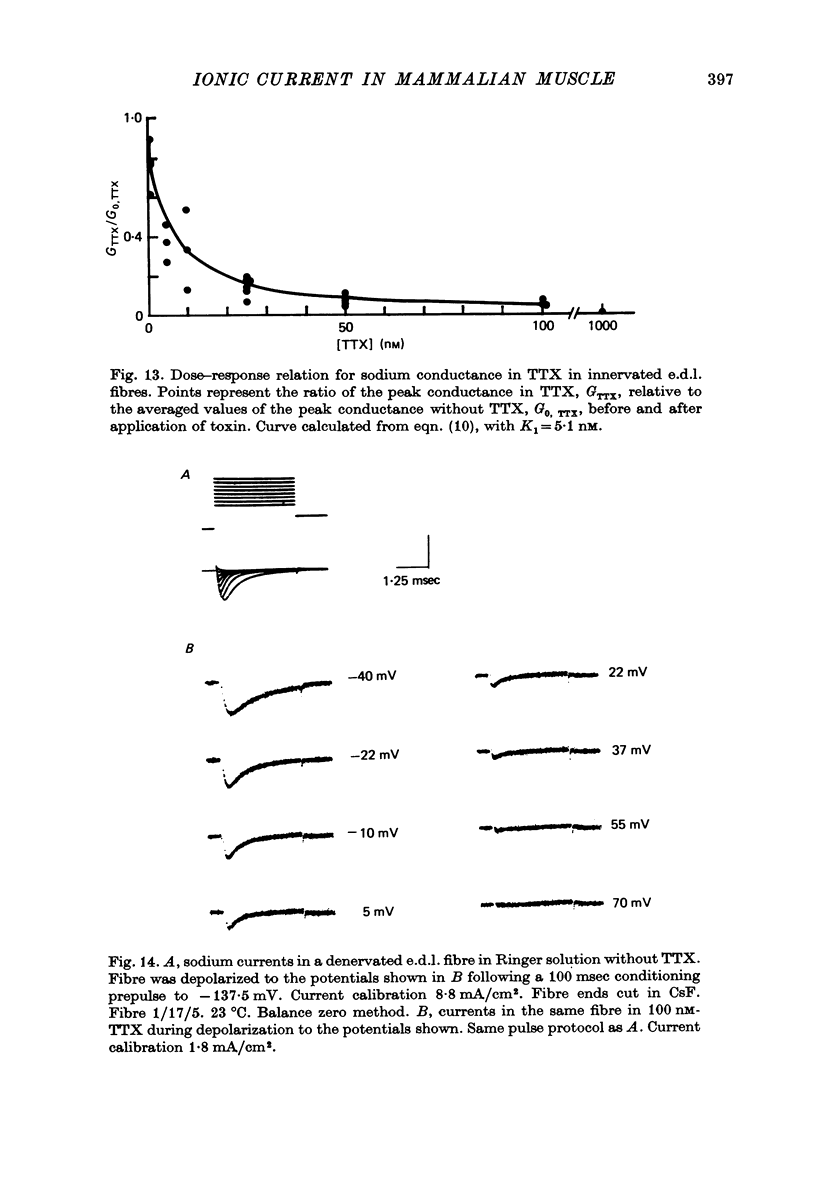
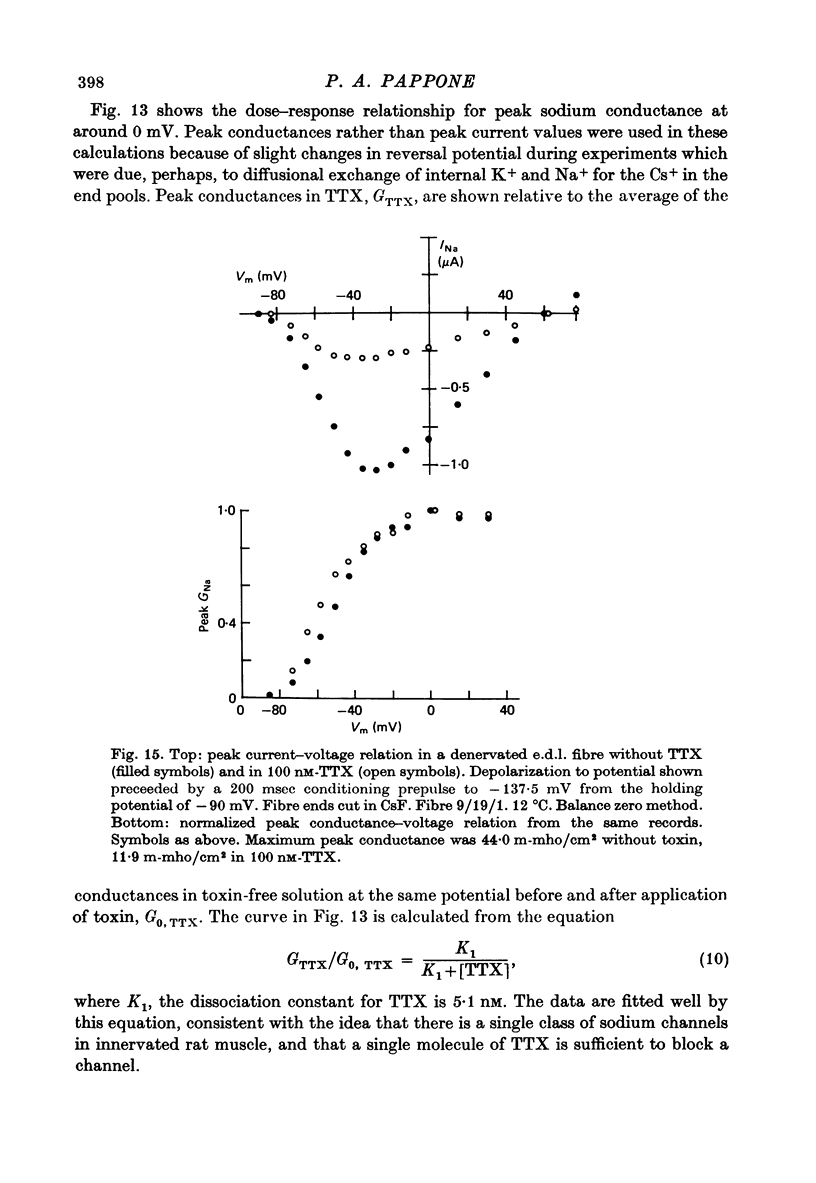
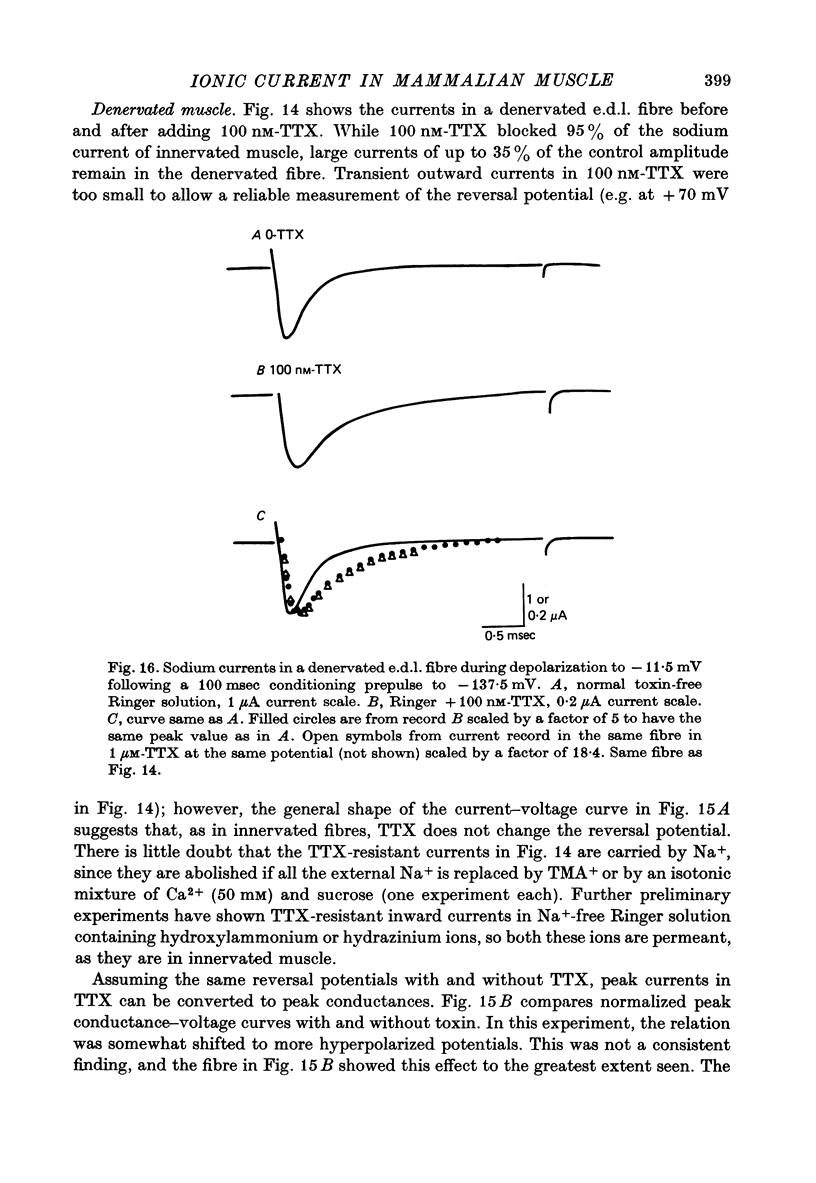
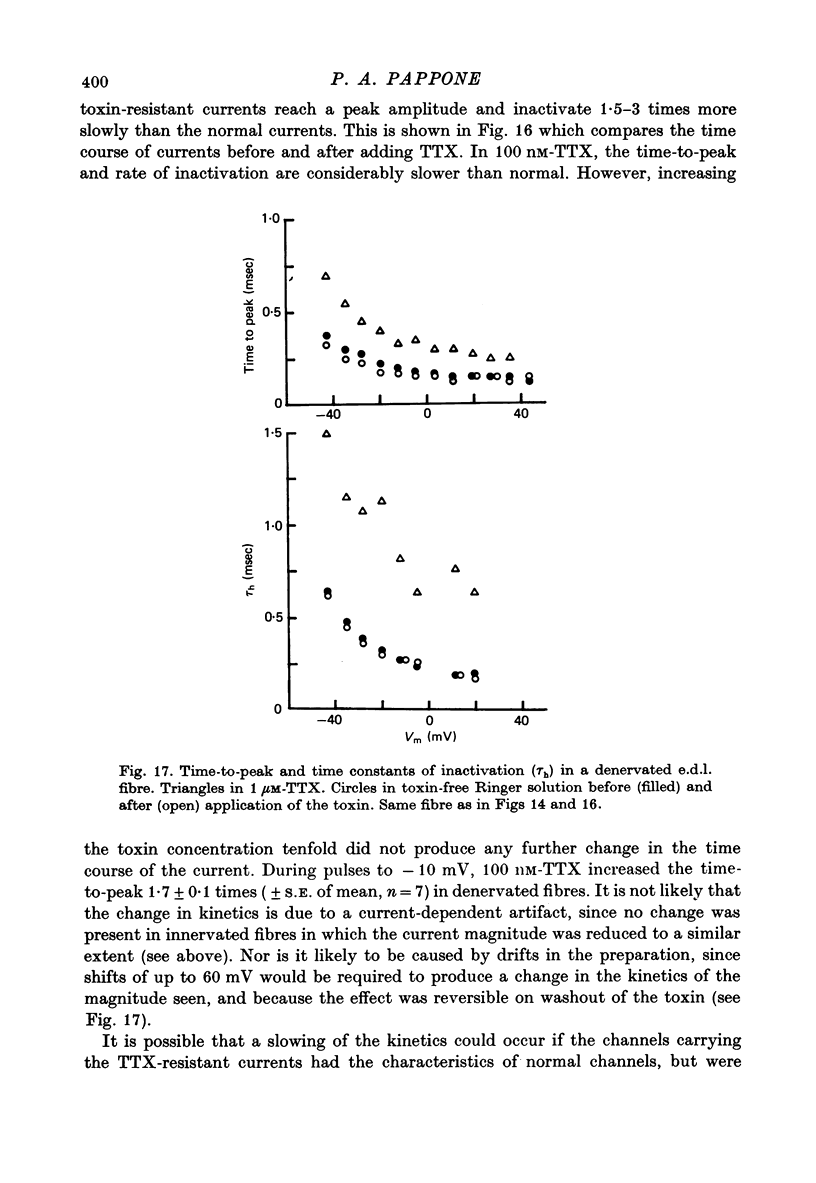
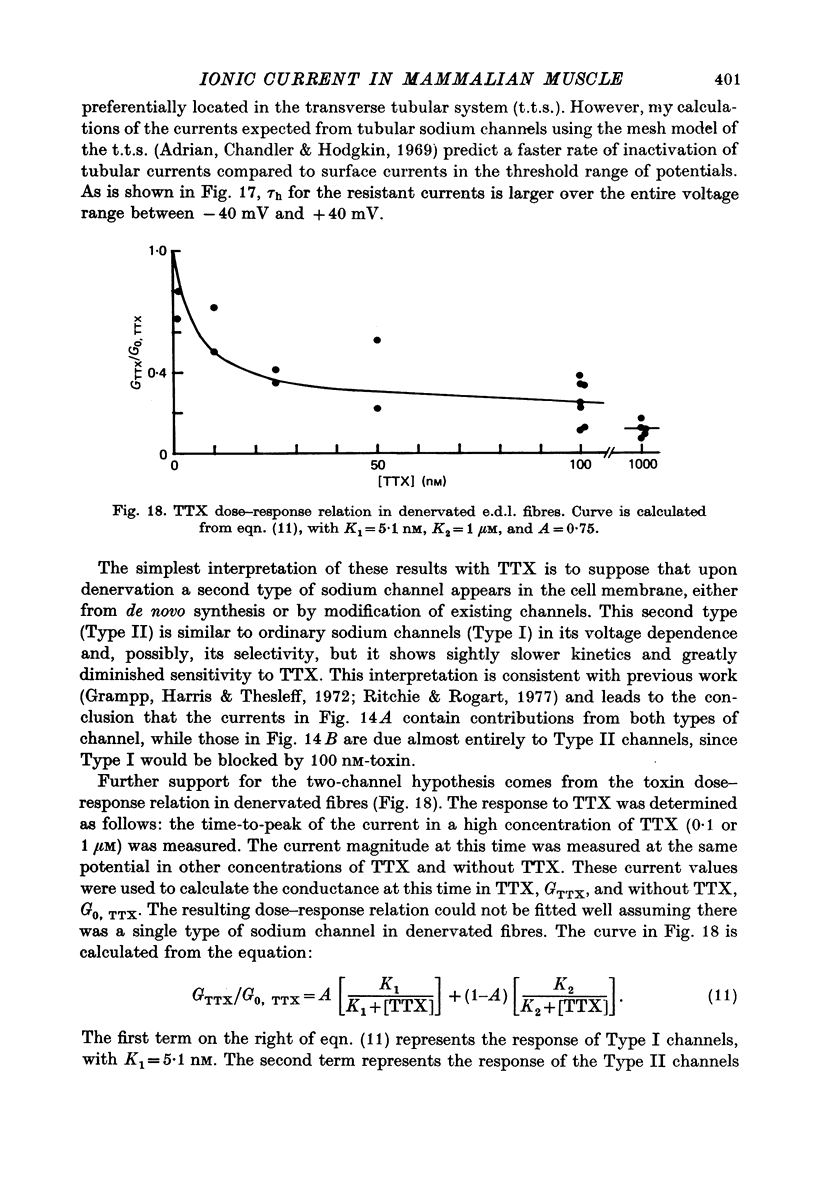
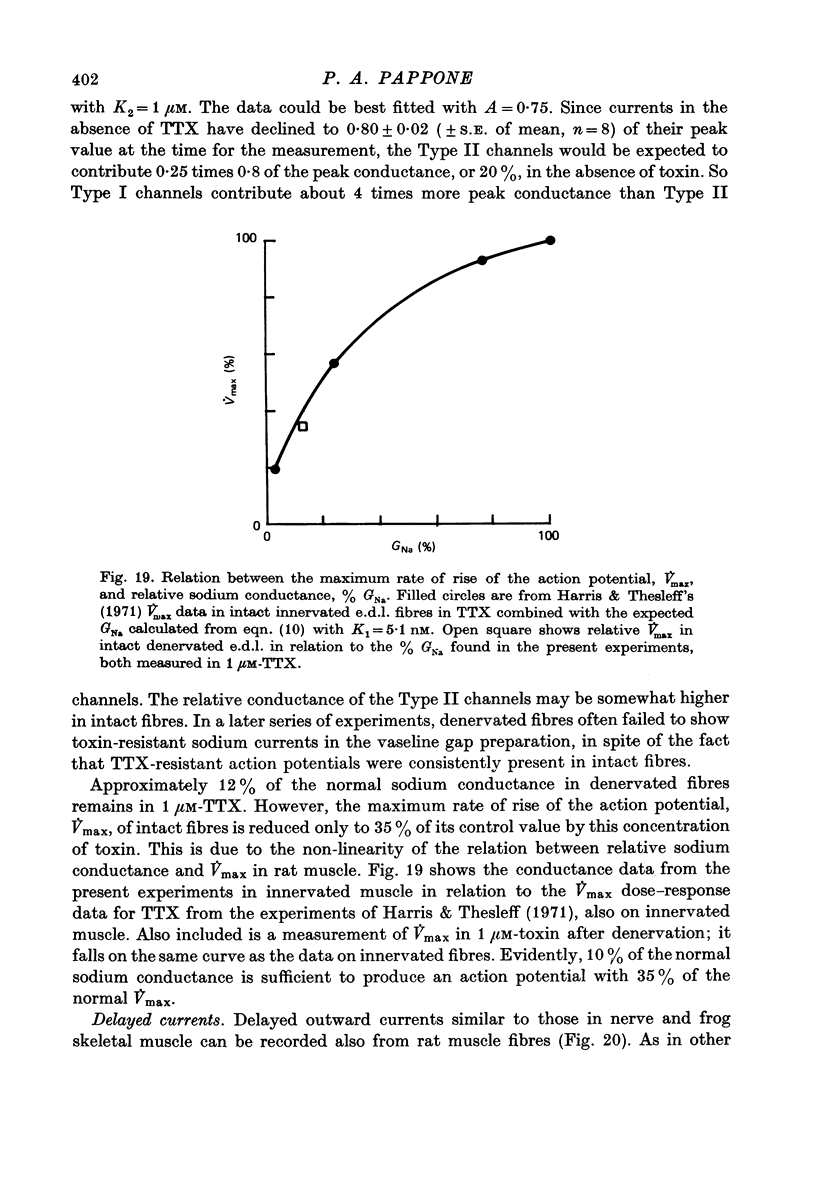
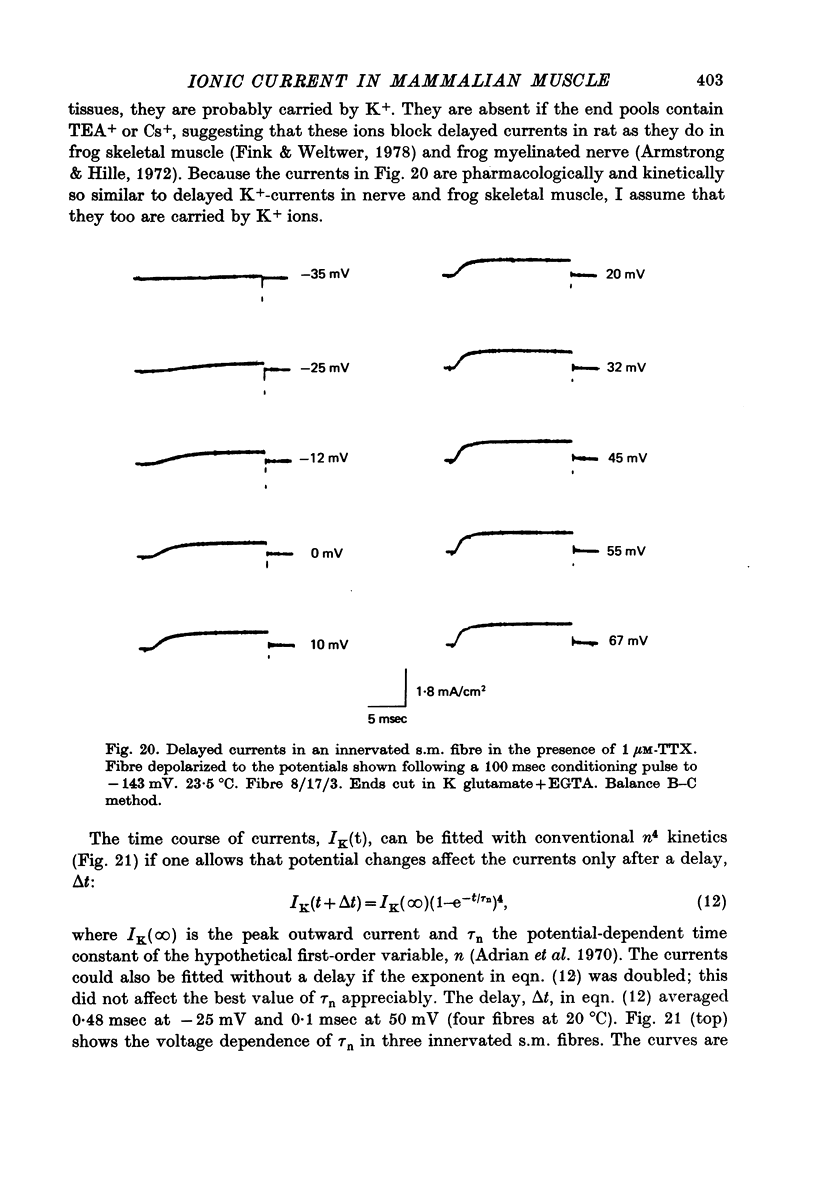
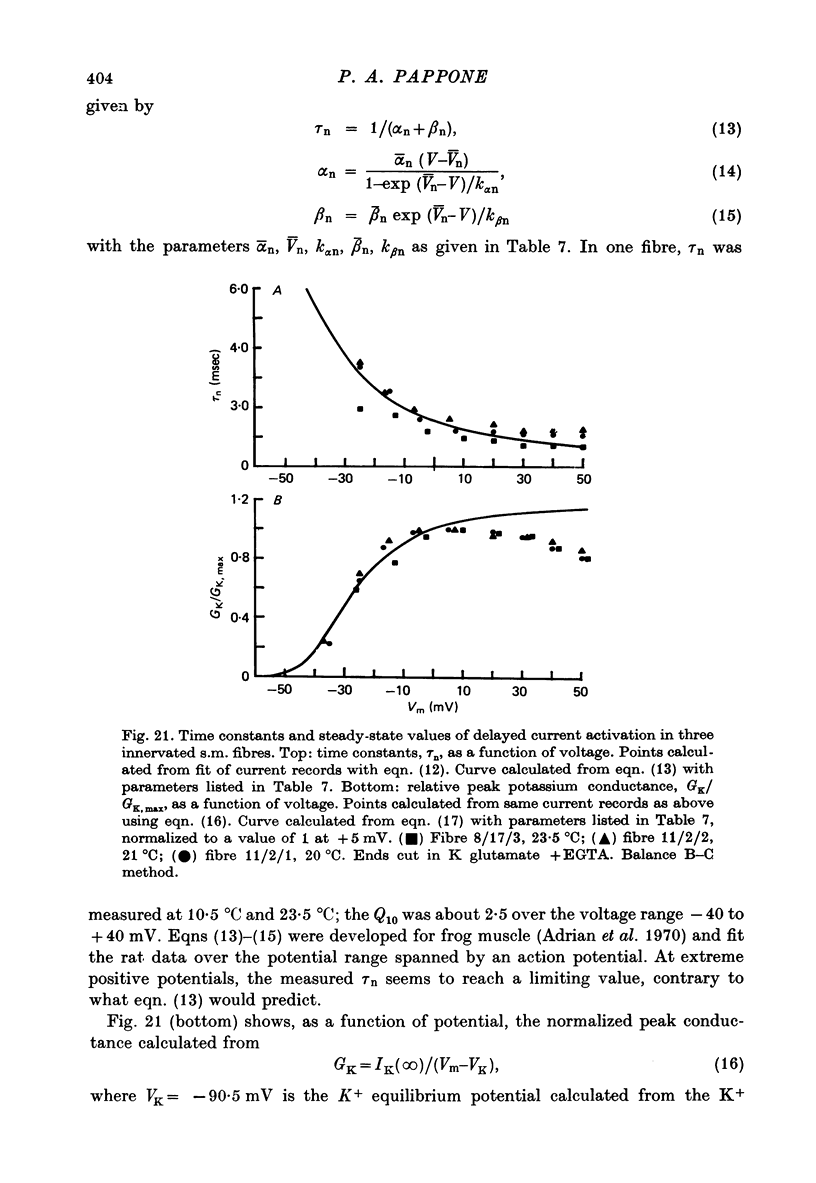
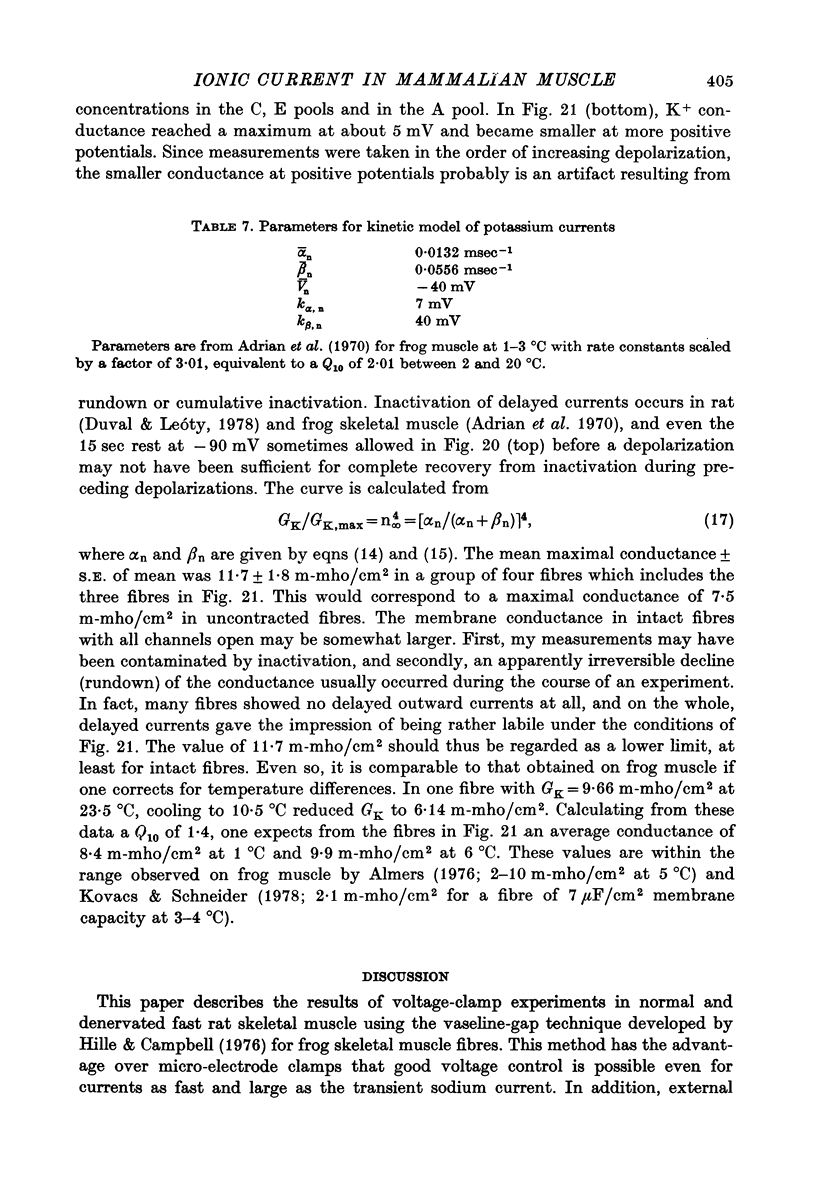
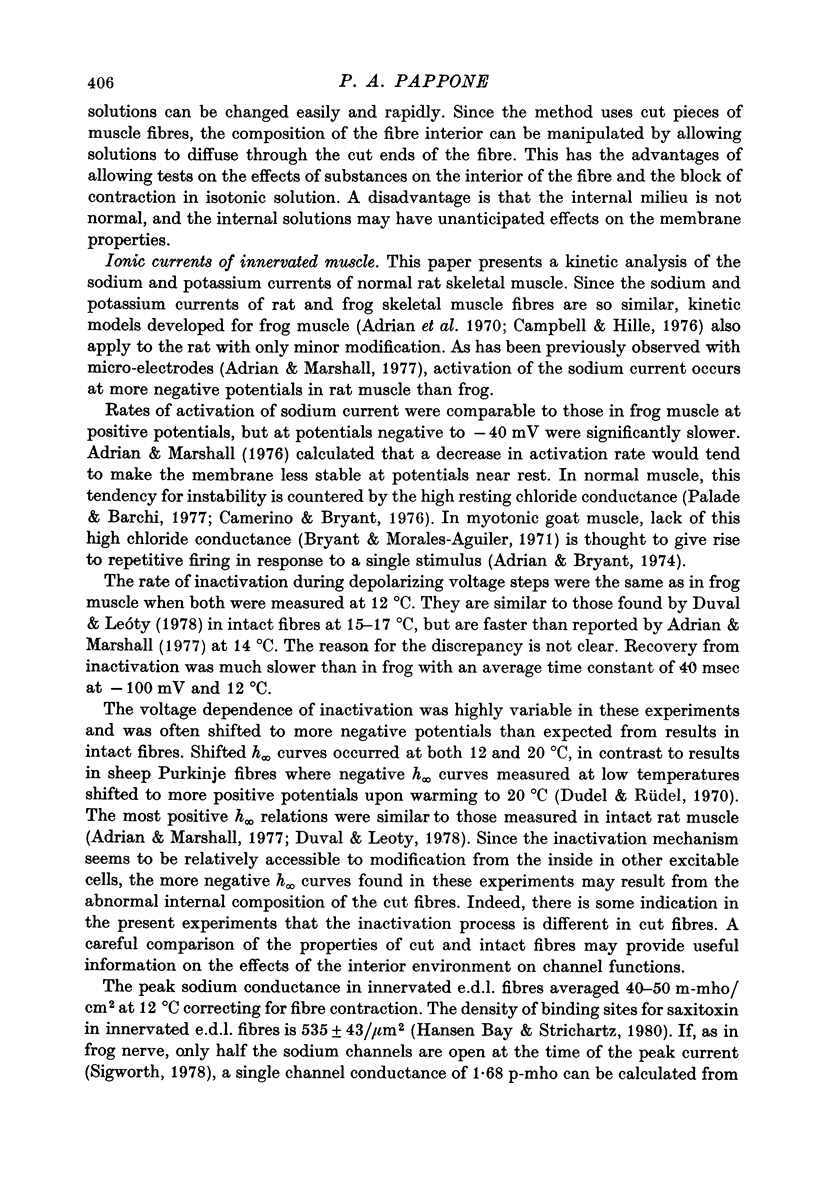
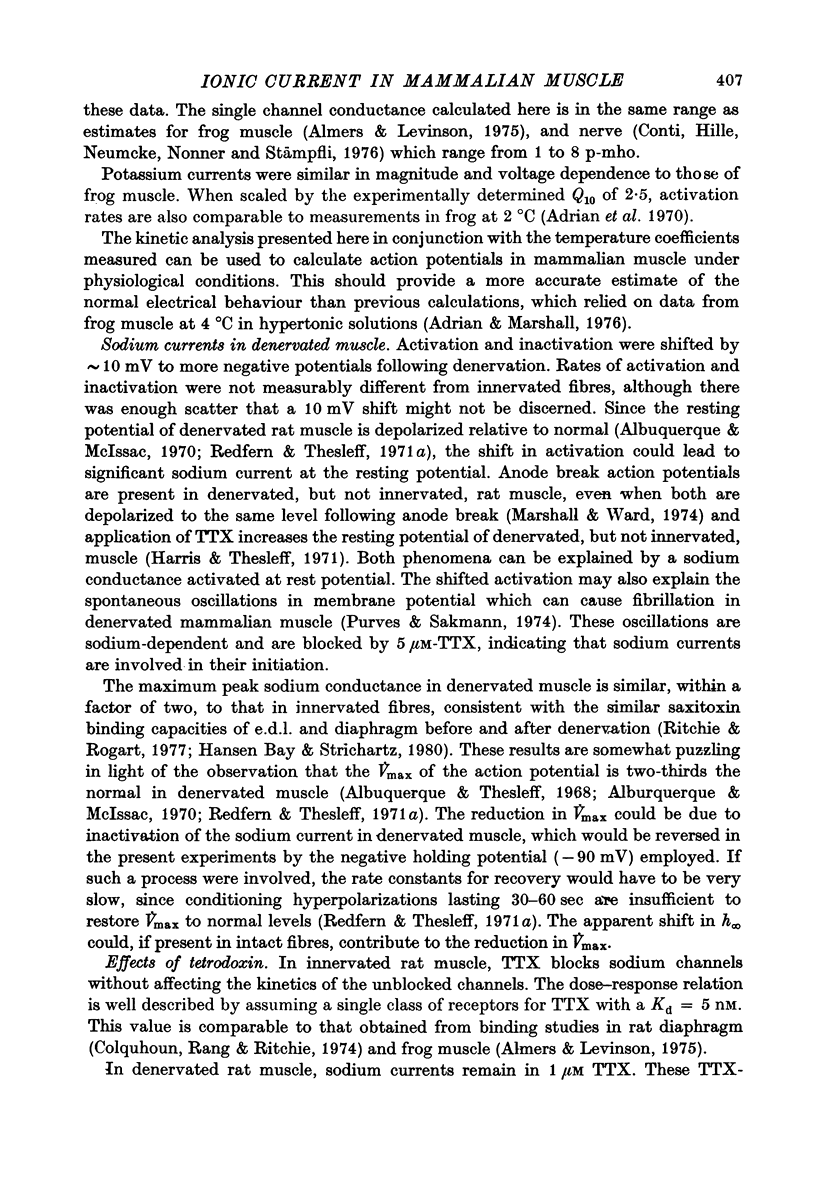
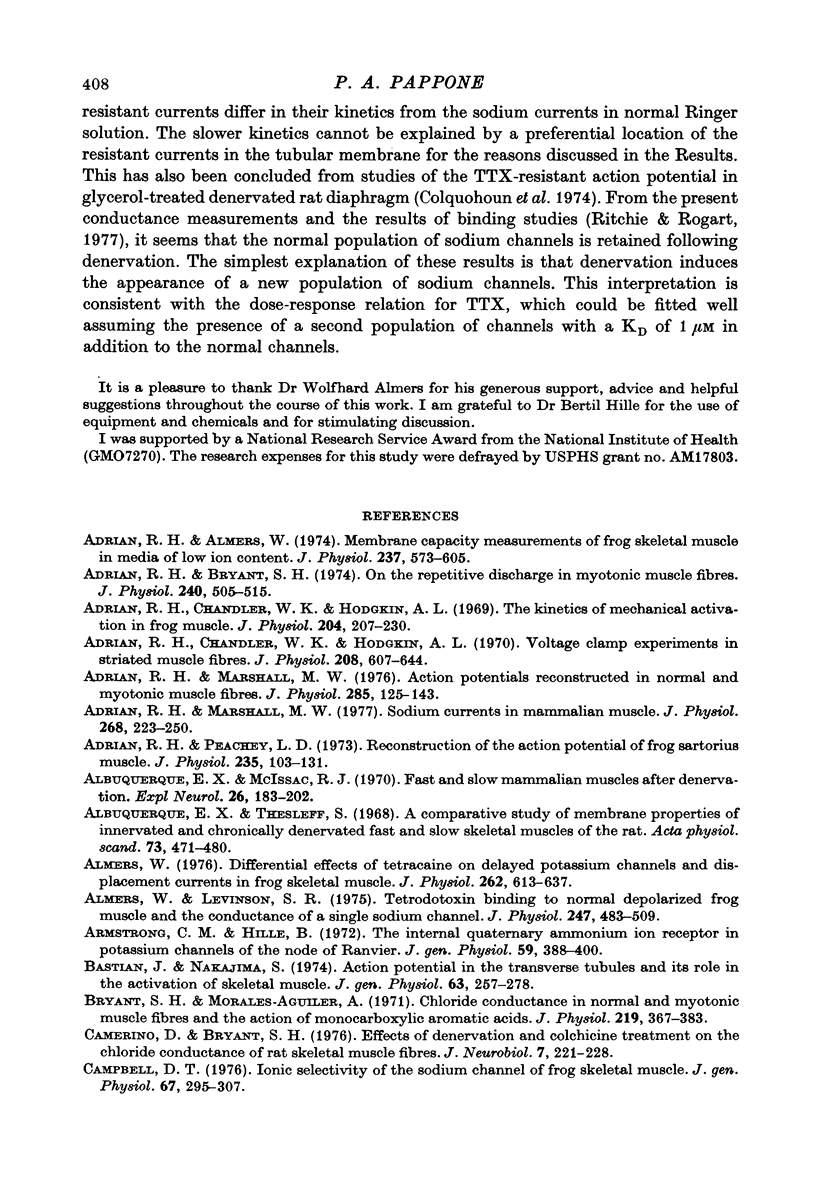
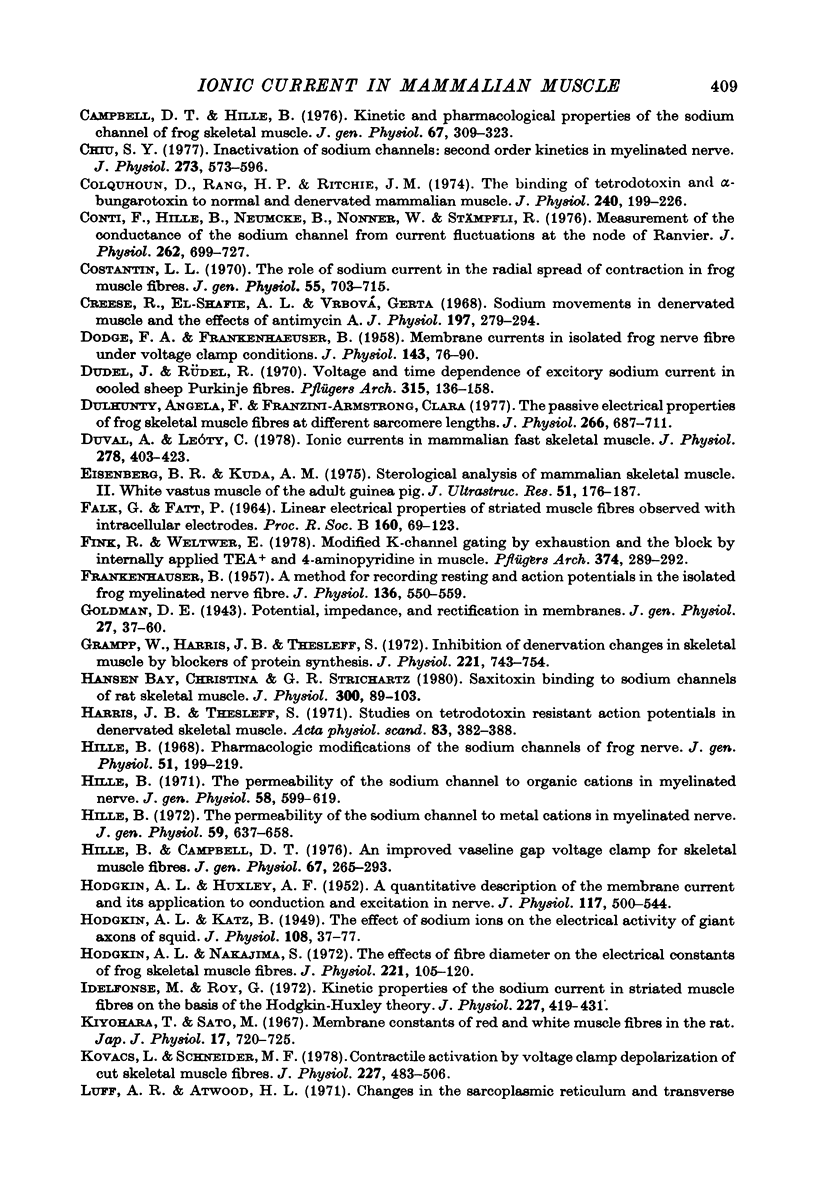
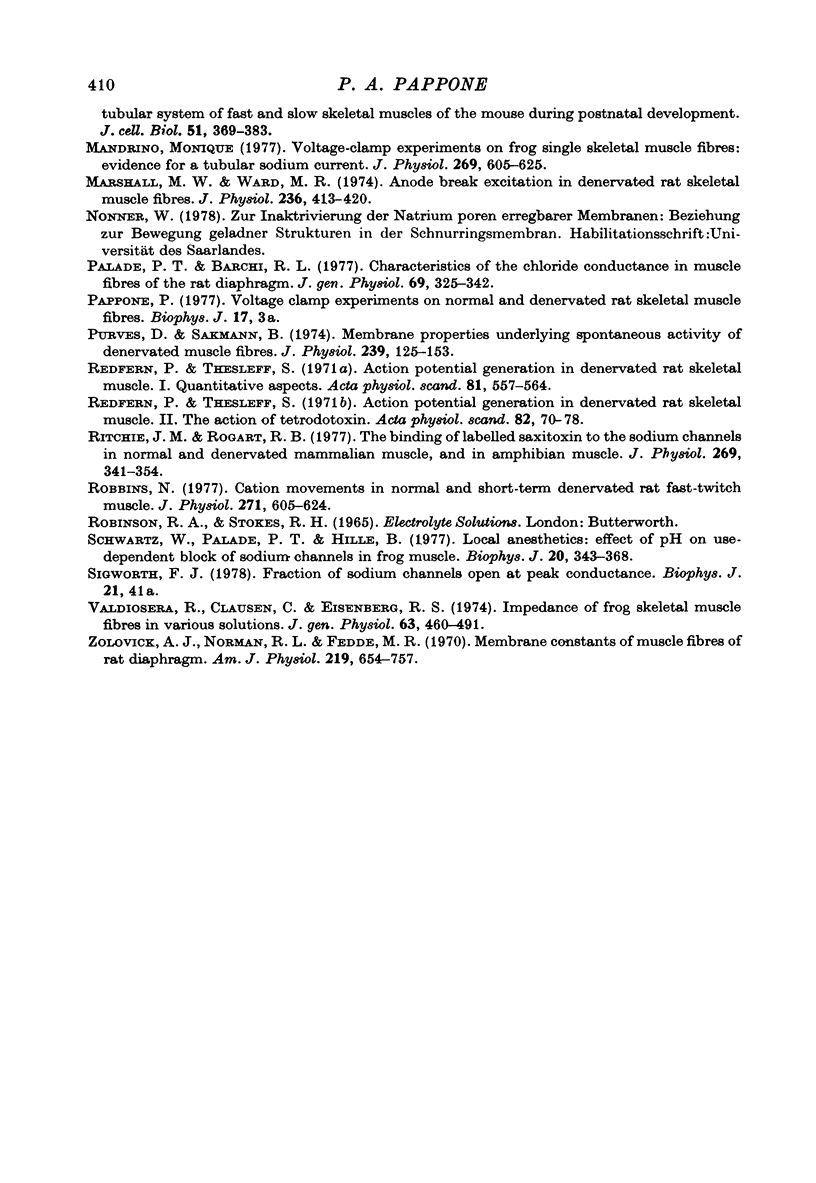
Selected References
These references are in PubMed. This may not be the complete list of references from this article.
- Adrian R. H., Almers W. Membrane capacity measurements on frog skeletal muscle in media of low ion content. J Physiol. 1974 Mar;237(3):573–605. doi: 10.1113/jphysiol.1974.sp010499. [DOI] [PMC free article] [PubMed] [Google Scholar]
- Adrian R. H., Bryant S. H. On the repetitive discharge in myotonic muscle fibres. J Physiol. 1974 Jul;240(2):505–515. doi: 10.1113/jphysiol.1974.sp010620. [DOI] [PMC free article] [PubMed] [Google Scholar]
- Adrian R. H., Chandler W. K., Hodgkin A. L. The kinetics of mechanical activation in frog muscle. J Physiol. 1969 Sep;204(1):207–230. doi: 10.1113/jphysiol.1969.sp008909. [DOI] [PMC free article] [PubMed] [Google Scholar]
- Adrian R. H., Chandler W. K., Hodgkin A. L. Voltage clamp experiments in striated muscle fibres. J Physiol. 1970 Jul;208(3):607–644. doi: 10.1113/jphysiol.1970.sp009139. [DOI] [PMC free article] [PubMed] [Google Scholar]
- Adrian R. H., Marshall M. W. Action potentials reconstructed in normal and myotonic muscle fibres. J Physiol. 1976 Jun;258(1):125–143. doi: 10.1113/jphysiol.1976.sp011410. [DOI] [PMC free article] [PubMed] [Google Scholar]
- Adrian R. H., Marshall M. W. Sodium currents in mammalian muscle. J Physiol. 1977 Jun;268(1):223–250. doi: 10.1113/jphysiol.1977.sp011855. [DOI] [PMC free article] [PubMed] [Google Scholar]
- Adrian R. H., Peachey L. D. Reconstruction of the action potential of frog sartorius muscle. J Physiol. 1973 Nov;235(1):103–131. doi: 10.1113/jphysiol.1973.sp010380. [DOI] [PMC free article] [PubMed] [Google Scholar]
- Albuquerque E. X., McIsaac R. J. Fast and slow mammalian muscles after denervation. Exp Neurol. 1970 Jan;26(1):183–202. doi: 10.1016/0014-4886(70)90099-3. [DOI] [PubMed] [Google Scholar]
- Albuquerque E. X., Thesleff S. A comparative study of membrane properties of innervated and chronically denervated fast and slow skeletal muscles of the rat. Acta Physiol Scand. 1968 Aug;73(4):471–480. doi: 10.1111/j.1365-201x.1968.tb10886.x. [DOI] [PubMed] [Google Scholar]
- Almers W. Differential effects of tetracaine on delayed potassium channels and displacement currents in frog skeletal muscle. J Physiol. 1976 Nov;262(3):613–637. doi: 10.1113/jphysiol.1976.sp011612. [DOI] [PMC free article] [PubMed] [Google Scholar]
- Almers W., Levinson S. R. Tetrodotoxin binding to normal depolarized frog muscle and the conductance of a single sodium channel. J Physiol. 1975 May;247(2):483–509. doi: 10.1113/jphysiol.1975.sp010943. [DOI] [PMC free article] [PubMed] [Google Scholar]
- Armstrong C. M., Hille B. The inner quaternary ammonium ion receptor in potassium channels of the node of Ranvier. J Gen Physiol. 1972 Apr;59(4):388–400. doi: 10.1085/jgp.59.4.388. [DOI] [PMC free article] [PubMed] [Google Scholar]
- Bastian J., Nakajima S. Action potential in the transverse tubules and its role in the activation of skeletal muscle. J Gen Physiol. 1974 Feb;63(2):257–278. doi: 10.1085/jgp.63.2.257. [DOI] [PMC free article] [PubMed] [Google Scholar]
- Bay C. M., Strichartz G. R. Saxitoxin binding to sodium channels of rat skeletal muscles. J Physiol. 1980 Mar;300:89–103. doi: 10.1113/jphysiol.1980.sp013153. [DOI] [PMC free article] [PubMed] [Google Scholar]
- Bryant S. H., Morales-Aguilera A. Chloride conductance in normal and myotonic muscle fibres and the action of monocarboxylic aromatic acids. J Physiol. 1971 Dec;219(2):367–383. doi: 10.1113/jphysiol.1971.sp009667. [DOI] [PMC free article] [PubMed] [Google Scholar]
- Camerino D., Bryant S. H. Effects of denervation and colchicine treatment on the chloride conductance of rat skeletal muscle fibers. J Neurobiol. 1976 May;7(3):221–228. doi: 10.1002/neu.480070305. [DOI] [PubMed] [Google Scholar]
- Campbell D. T., Hille B. Kinetic and pharmacological properties of the sodium channel of frog skeletal muscle. J Gen Physiol. 1976 Mar;67(3):309–323. doi: 10.1085/jgp.67.3.309. [DOI] [PMC free article] [PubMed] [Google Scholar]
- Campbell D. T. Ionic selectivity of the sodium channel of frog skeletal muscle. J Gen Physiol. 1976 Mar;67(3):295–307. doi: 10.1085/jgp.67.3.295. [DOI] [PMC free article] [PubMed] [Google Scholar]
- Colquhoun D., Rang H. P., Ritchie J. M. The binding of tetrodotoxin and alpha-bungarotoxin to normal and denervated mammalian muscle. J Physiol. 1974 Jul;240(1):199–226. doi: 10.1113/jphysiol.1974.sp010607. [DOI] [PMC free article] [PubMed] [Google Scholar]
- Conti F., Hille B., Neumcke B., Nonner W., Stämpfli R. Measurement of the conductance of the sodium channel from current fluctuations at the node of Ranvier. J Physiol. 1976 Nov;262(3):699–727. doi: 10.1113/jphysiol.1976.sp011616. [DOI] [PMC free article] [PubMed] [Google Scholar]
- Creese R., el-Shafie A. L., Vrbová G. Sodium movements in denervated muscle and the effects of antimycin A. J Physiol. 1968 Jul;197(2):279–294. doi: 10.1113/jphysiol.1968.sp008559. [DOI] [PMC free article] [PubMed] [Google Scholar]
- DODGE F. A., FRANKENHAEUSER B. Membrane currents in isolated frog nerve fibre under voltage clamp conditions. J Physiol. 1958 Aug 29;143(1):76–90. doi: 10.1113/jphysiol.1958.sp006045. [DOI] [PMC free article] [PubMed] [Google Scholar]
- Dudel J., Rüdel R. Voltage and time dependence of excitatory sodium current in cooled sheep Purkinje fibres. Pflugers Arch. 1970;315(2):136–158. doi: 10.1007/BF00586657. [DOI] [PubMed] [Google Scholar]
- Duval A., Léoty C. Ionic currents in mammalian fast skeletal muscle. J Physiol. 1978 May;278:403–423. doi: 10.1113/jphysiol.1978.sp012312. [DOI] [PMC free article] [PubMed] [Google Scholar]
- Eisenberg B. R., Kuda A. M. Stereological analysis of mammalian skeletal muscle. II. White vastus muscle of the adult guinea pig. J Ultrastruct Res. 1975 May;51(2):176–187. doi: 10.1016/s0022-5320(75)80146-8. [DOI] [PubMed] [Google Scholar]
- FALK G., FATT P. LINEAR ELECTRICAL PROPERTIES OF STRIATED MUSCLE FIBRES OBSERVED WITH INTRACELLULAR ELECTRODES. Proc R Soc Lond B Biol Sci. 1964 Apr 14;160:69–123. doi: 10.1098/rspb.1964.0030. [DOI] [PubMed] [Google Scholar]
- Grampp W., Harris J. B., Thesleff S. Inhibition of denervation changes in skeletal muscle by blockers of protein synthesis. J Physiol. 1972 Mar;221(3):743–754. doi: 10.1113/jphysiol.1972.sp009780. [DOI] [PMC free article] [PubMed] [Google Scholar]
- HODGKIN A. L., HUXLEY A. F. A quantitative description of membrane current and its application to conduction and excitation in nerve. J Physiol. 1952 Aug;117(4):500–544. doi: 10.1113/jphysiol.1952.sp004764. [DOI] [PMC free article] [PubMed] [Google Scholar]
- HODGKIN A. L., KATZ B. The effect of sodium ions on the electrical activity of giant axon of the squid. J Physiol. 1949 Mar 1;108(1):37–77. doi: 10.1113/jphysiol.1949.sp004310. [DOI] [PMC free article] [PubMed] [Google Scholar]
- Harris J. B., Thesleff S. Studies on tetrodotoxin resistant action potentials in denervated skeletal muscle. Acta Physiol Scand. 1971 Nov;83(3):382–388. doi: 10.1111/j.1748-1716.1971.tb05091.x. [DOI] [PubMed] [Google Scholar]
- Hille B., Campbell D. T. An improved vaseline gap voltage clamp for skeletal muscle fibers. J Gen Physiol. 1976 Mar;67(3):265–293. doi: 10.1085/jgp.67.3.265. [DOI] [PMC free article] [PubMed] [Google Scholar]
- Hille B. Pharmacological modifications of the sodium channels of frog nerve. J Gen Physiol. 1968 Feb;51(2):199–219. doi: 10.1085/jgp.51.2.199. [DOI] [PMC free article] [PubMed] [Google Scholar]
- Hodgkin A. L., Nakajima S. The effect of diameter on the electrical constants of frog skeletal muscle fibres. J Physiol. 1972 Feb;221(1):105–120. doi: 10.1113/jphysiol.1972.sp009742. [DOI] [PMC free article] [PubMed] [Google Scholar]
- Ildefonse M., Roy G. Kinetic properties of the sodium current in striated muscle fibres on the basis of the Hodgkin-Huxley theory. J Physiol. 1972 Dec;227(2):419–431. doi: 10.1113/jphysiol.1972.sp010040. [DOI] [PMC free article] [PubMed] [Google Scholar]
- Kiyohara T., Sato M. Membrane constants of red and white muscle fibers in the rat. Jpn J Physiol. 1967 Dec 15;17(6):720–725. doi: 10.2170/jjphysiol.17.720. [DOI] [PubMed] [Google Scholar]
- Kovács L., Schneider M. F. Contractile activation by voltage clamp depolarization of cut skeletal muscle fibres. J Physiol. 1978 Apr;277:483–506. doi: 10.1113/jphysiol.1978.sp012286. [DOI] [PMC free article] [PubMed] [Google Scholar]
- Luff A. R., Atwood H. L. Changes in the sarcoplasmic reticulum and transverse tubular system of fast and slow skeletal muscles of the mouse during postnatal development. J Cell Biol. 1971 Nov;51(21):369–383. doi: 10.1083/jcb.51.2.369. [DOI] [PMC free article] [PubMed] [Google Scholar]
- Mandrino M. Voltage-clamp experiments on frog single skeletal muscle fibres: evidence for a tubular sodium current. J Physiol. 1977 Aug;269(3):605–625. doi: 10.1113/jphysiol.1977.sp011918. [DOI] [PMC free article] [PubMed] [Google Scholar]
- Marshall M. W., Ward M. R. Anode break excitation in denervated rat skeletal muscle fibres. J Physiol. 1974 Jan;236(2):413–420. doi: 10.1113/jphysiol.1974.sp010443. [DOI] [PMC free article] [PubMed] [Google Scholar]
- Palade P. T., Barchi R. L. Characteristics of the chloride conductance in muscle fibers of the rat diaphragm. J Gen Physiol. 1977 Mar;69(3):325–342. doi: 10.1085/jgp.69.3.325. [DOI] [PMC free article] [PubMed] [Google Scholar]
- Purves D., Sakmann B. Membrane properties underlying spontaneous activity of denervated muscle fibres. J Physiol. 1974 May;239(1):125–153. doi: 10.1113/jphysiol.1974.sp010559. [DOI] [PMC free article] [PubMed] [Google Scholar]
- Redfern P., Thesleff S. Action potential generation in denervated rat skeletal muscle. I. Quantitative aspects. Acta Physiol Scand. 1971 Apr;81(4):557–564. doi: 10.1111/j.1748-1716.1971.tb04932.x. [DOI] [PubMed] [Google Scholar]
- Redfern P., Thesleff S. Action potential generation in denervated rat skeletal muscle. II. The action of tetrodotoxin. Acta Physiol Scand. 1971 May;82(1):70–78. doi: 10.1111/j.1748-1716.1971.tb04943.x. [DOI] [PubMed] [Google Scholar]
- Ritchie J. M., Rogart R. B. The binding of labelled saxitoxin to the sodium channels in normal and denervated mammalian muscle, and in amphibian muscle. J Physiol. 1977 Jul;269(2):341–354. doi: 10.1113/jphysiol.1977.sp011905. [DOI] [PMC free article] [PubMed] [Google Scholar]
- Robbins N. Cation movements in normal and short-term denervated rat fast twitch muscle. J Physiol. 1977 Oct;271(3):605–624. doi: 10.1113/jphysiol.1977.sp012017. [DOI] [PMC free article] [PubMed] [Google Scholar]
- Schwarz W., Palade P. T., Hille B. Local anesthetics. Effect of pH on use-dependent block of sodium channels in frog muscle. Biophys J. 1977 Dec;20(3):343–368. doi: 10.1016/S0006-3495(77)85554-9. [DOI] [PMC free article] [PubMed] [Google Scholar]
- Valdiosera R., Clausen C., Eisenberg R. S. Impedance of frog skeletal muscle fibers in various solutions. J Gen Physiol. 1974 Apr;63(4):460–491. doi: 10.1085/jgp.63.4.460. [DOI] [PMC free article] [PubMed] [Google Scholar]
- Zolovick A. J., Norman R. L., Fedde M. R. Membrane constants of muscle fibers of rat diaphragm. Am J Physiol. 1970 Sep;219(3):654–657. doi: 10.1152/ajplegacy.1970.219.3.654. [DOI] [PubMed] [Google Scholar]