Abstract
1. Renal cortical slices from rat, rabbit, and guinea-pig were incubated in media in which choline, caesium or rubidium replaced sodium.
2. Slices of rabbit and guinea-pig renal cortex incubated in oxygenated choline Ringer decreased in volume initially and did not swell over 3 hr at 25° C. There was a steady loss of potassium. Inhibition of metabolism (N2 + 1 mM iodoacetamide) caused some swelling. Ouabain, 10 mM, in choline Ringer affected neither loss of potassium nor tissue water content.
3. Slices of rat renal cortex similarly incubated in choline Ringer swelled over 3 hr at 25° C whether or not metabolism was inhibited; ouabain (15 mM) affected neither tissue potassium loss nor tissue water content.
4. Incubation in choline Ringer containing either 0·2 mM p-chloromercuribenzoic acid, or 1 mM ethacrynic acid increased the tissue water content of guinea-pig renal cortical slices.
5. Depletion of cellular potassium (by preliminary incubation in oxygenated potassium-free sodium Ringer with 10 mM ouabain at 30° C) resulted in increased tissue water content when rabbit renal cortical slices were subsequently incubated in oxygenated choline Ringer at 25° C for 3 hr.
6. There was no evidence of energy-dependent extrusion of water or ions from either equilibrated rat or rabbit renal cortical slices leached at 0·5° C and then reincubated at 25° C in choline Ringer.
7. Rat and guinea-pig renal cortical slices leached at 0.5° C and reincubated at 25° C swelled in rubidium Ringer and in caesium Ringer. There was no evidence of energy-dependent water or ion extrusion when metabolism was restored after leaching in either of these media. Metabolizing rat slices but not guinea-pig slices swelled faster than slices whose metabolism was inhibited.
8. These results lend no support to the mechano-chemical hypothesis which ascribes cellular volume regulation to a contractile mechanism squeezing isotonic extracellular fluid from the cells. Instead it is suggested that cellular water content in these experiments reflects the balance between the rate of loss of potassium (and chloride) from the cells and the rate of uptake of extracellular cation (and chloride) into the cells — these rates reflecting both the electrochemical potential gradients of the ions and membrane permeability to them. The implications in relation to the hypothesis of ouabain-insensitive cellular volume regulation are discussed.
Full text
PDF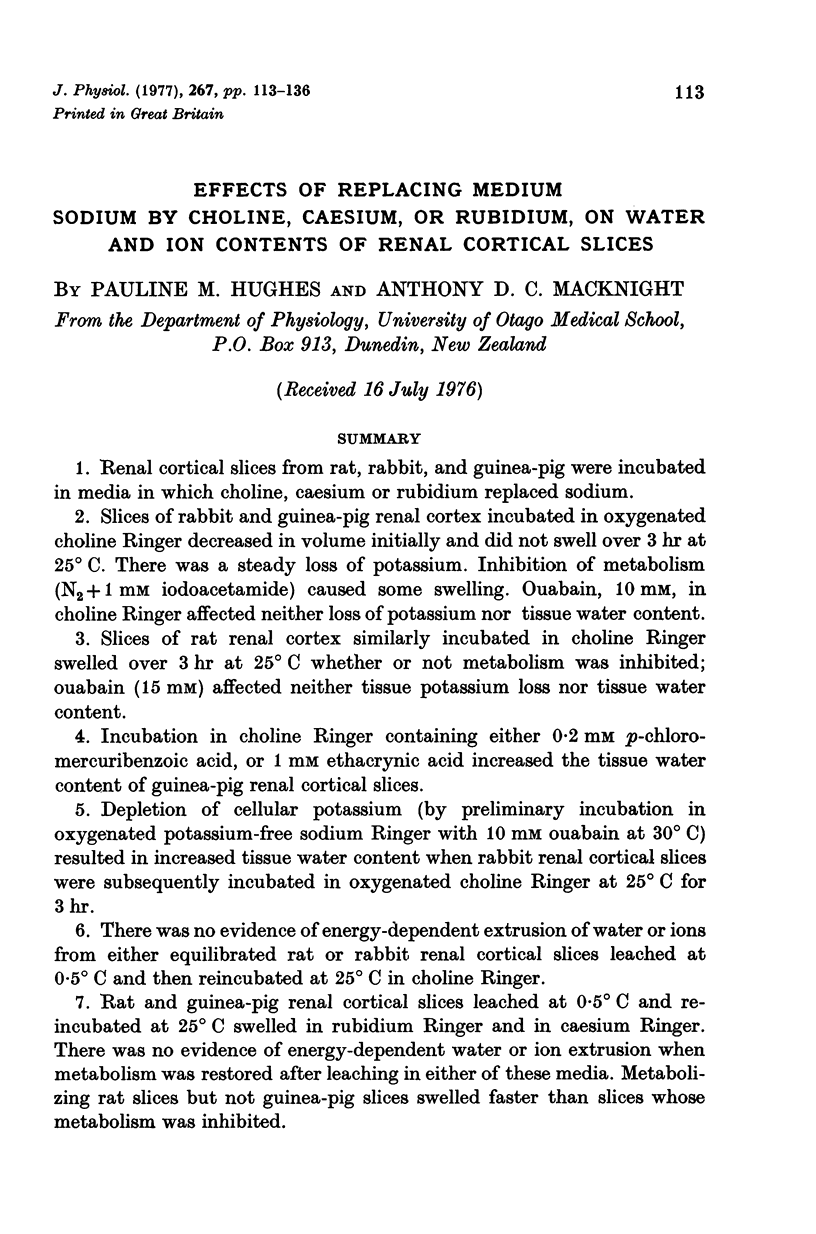
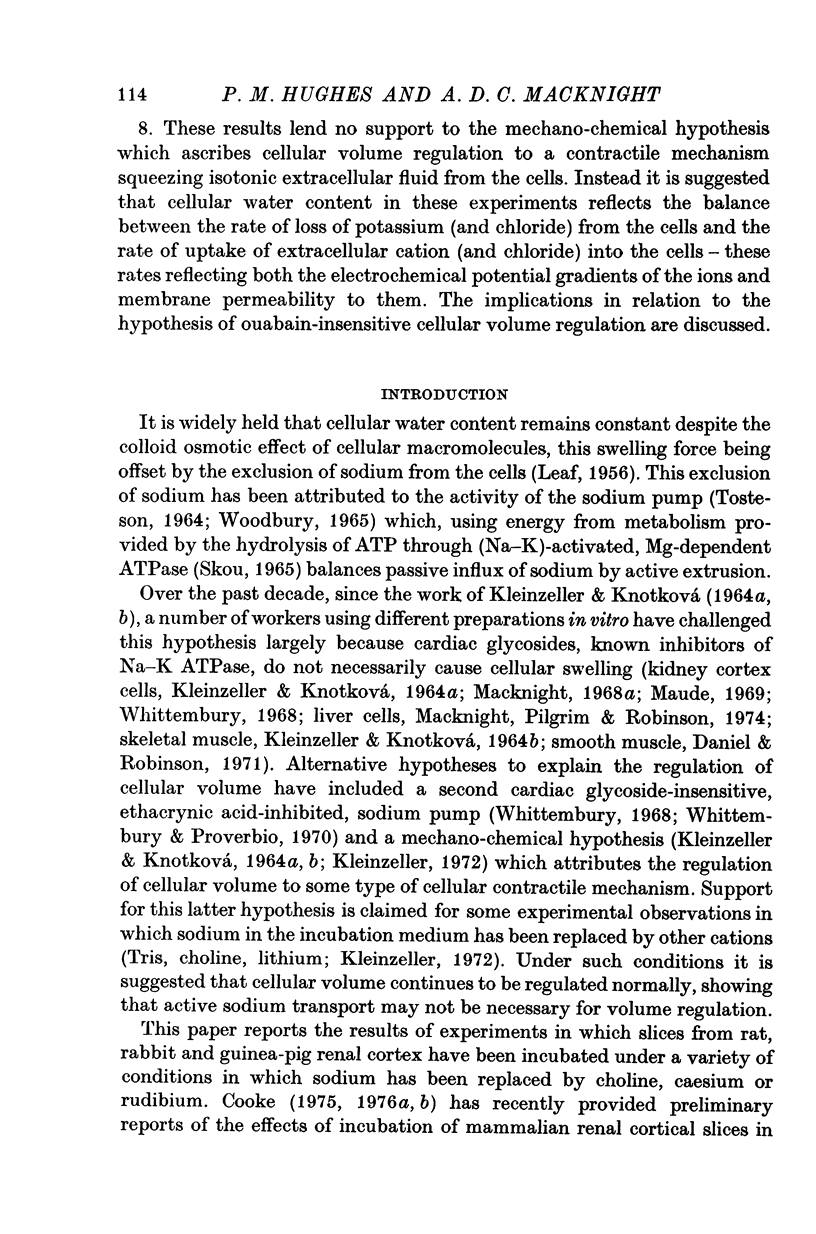
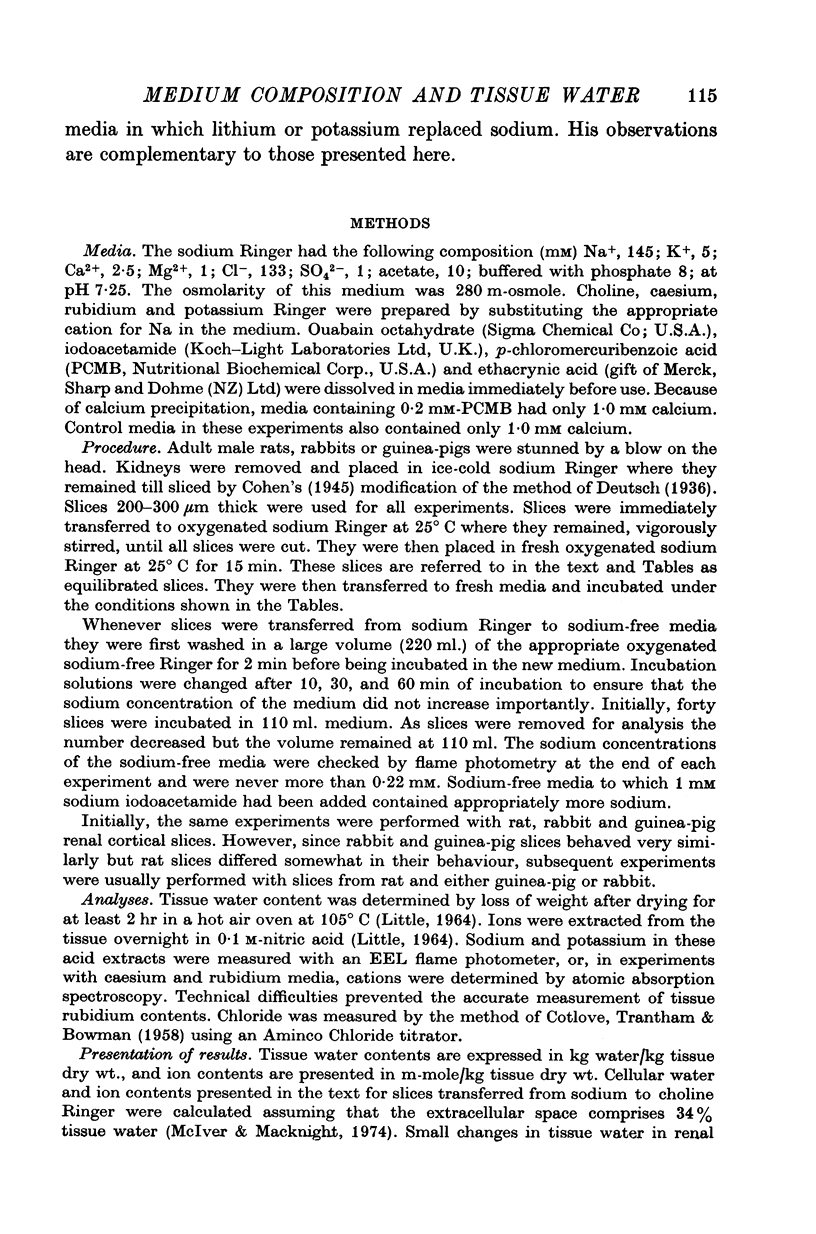
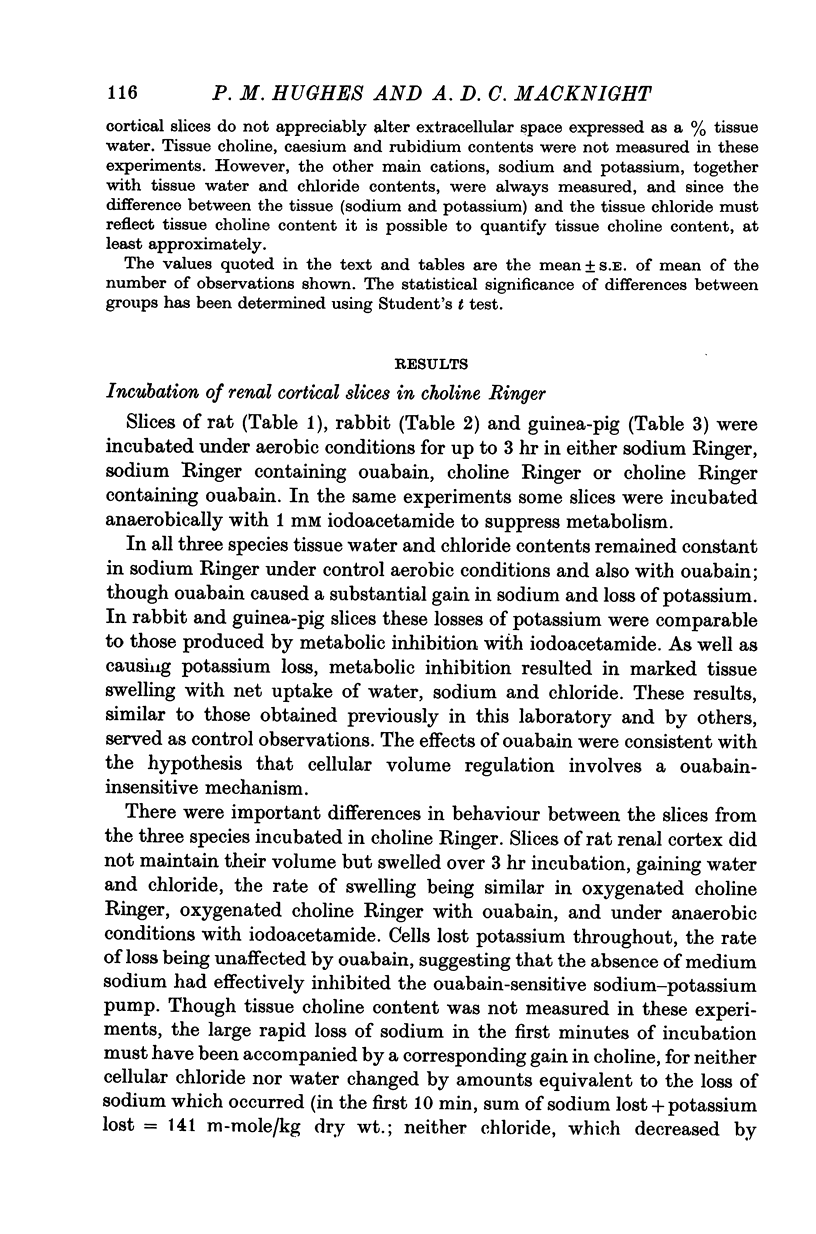
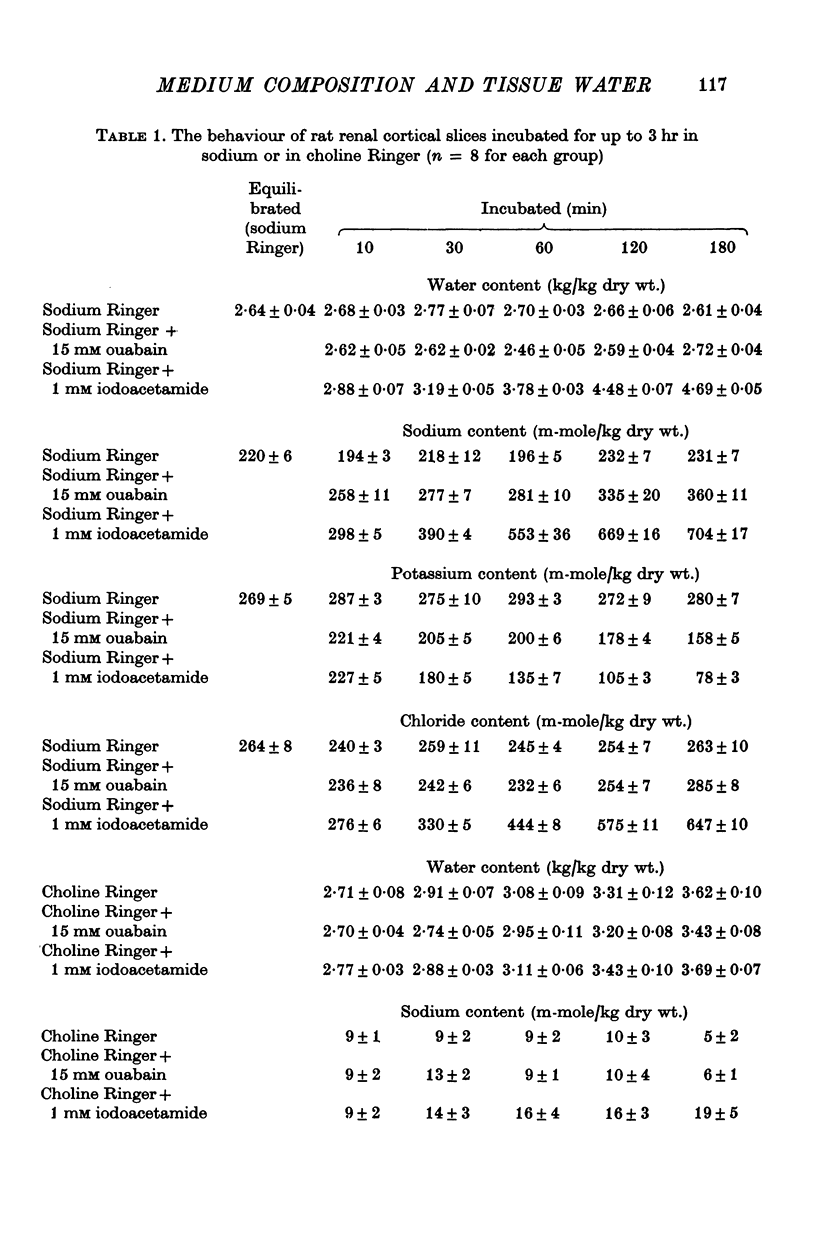
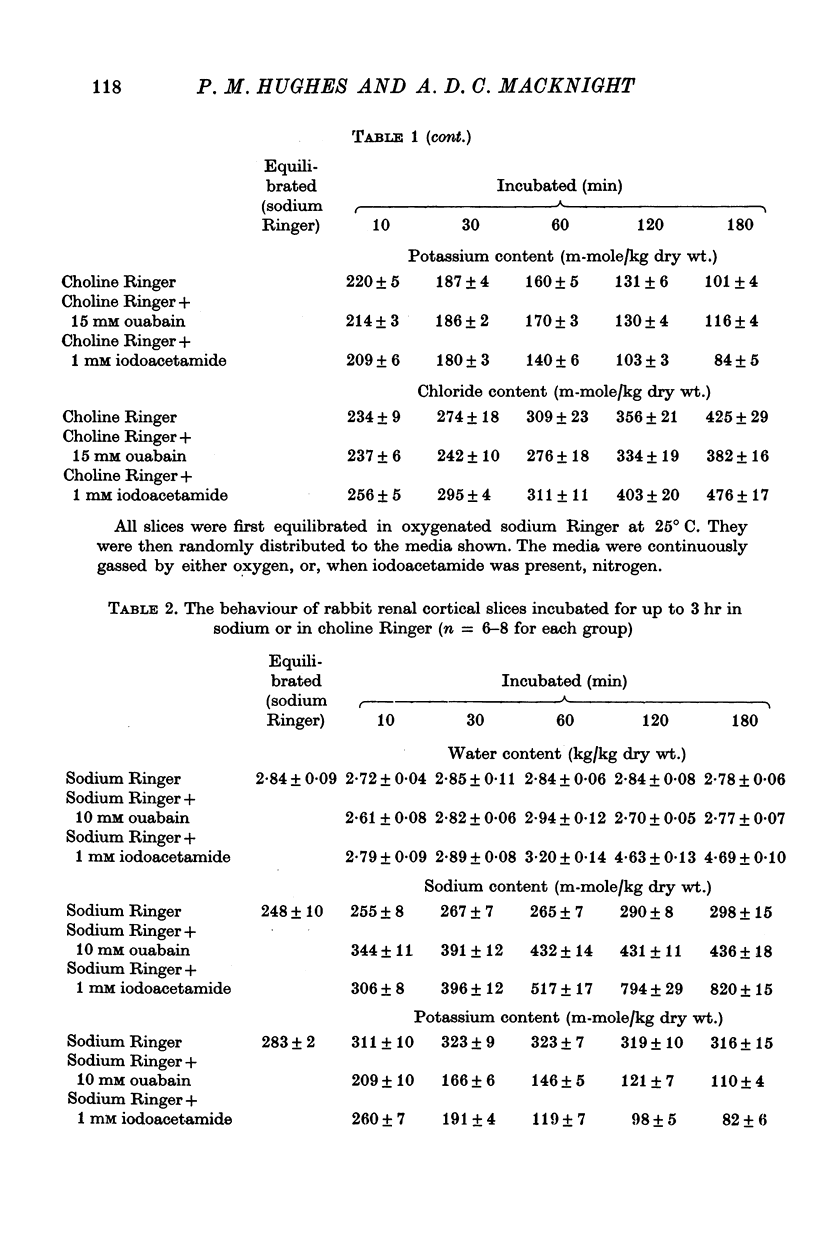
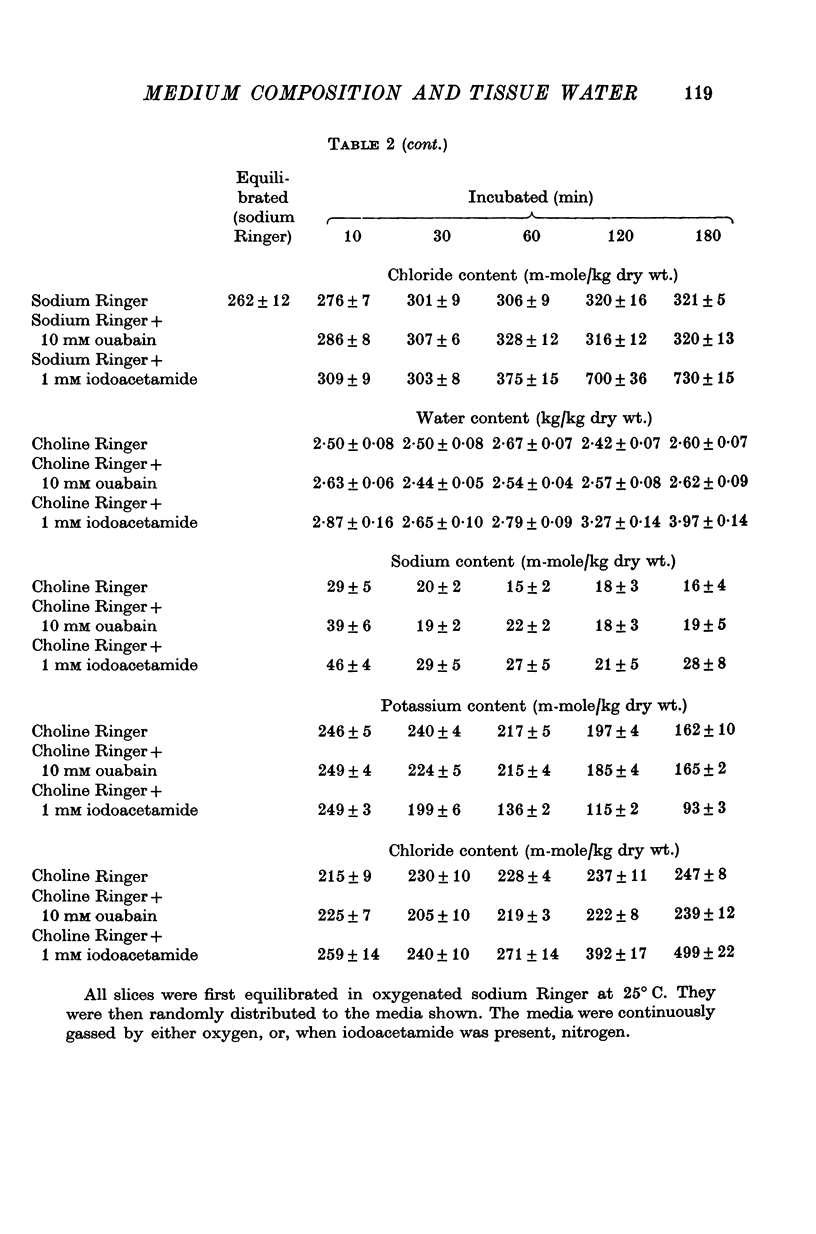
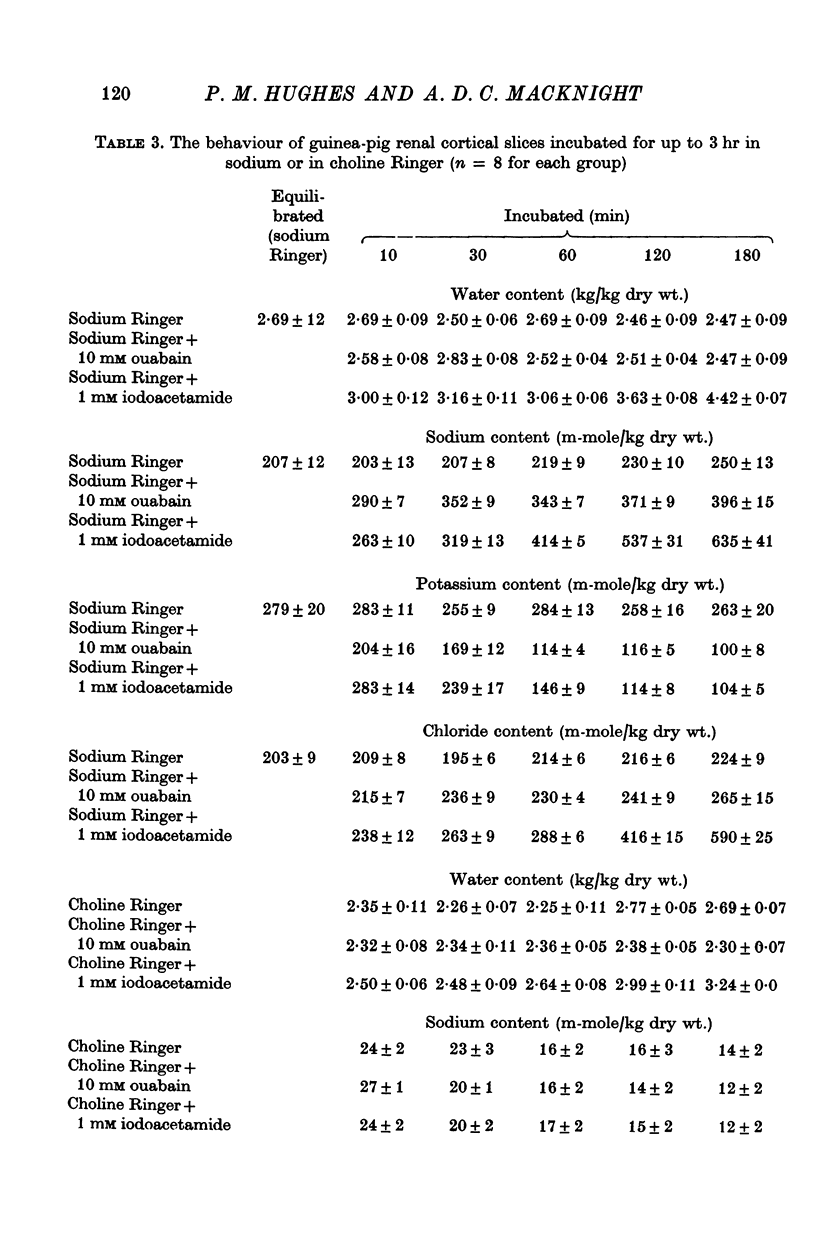
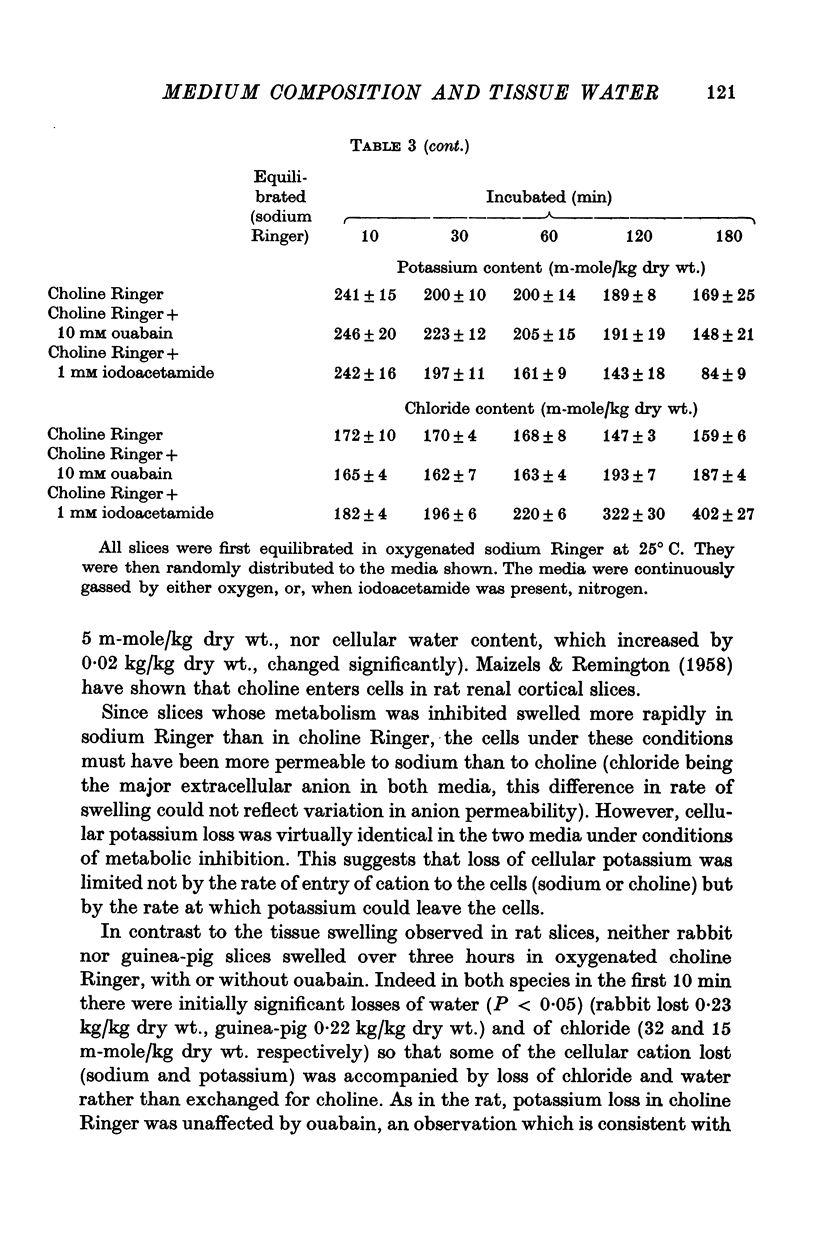
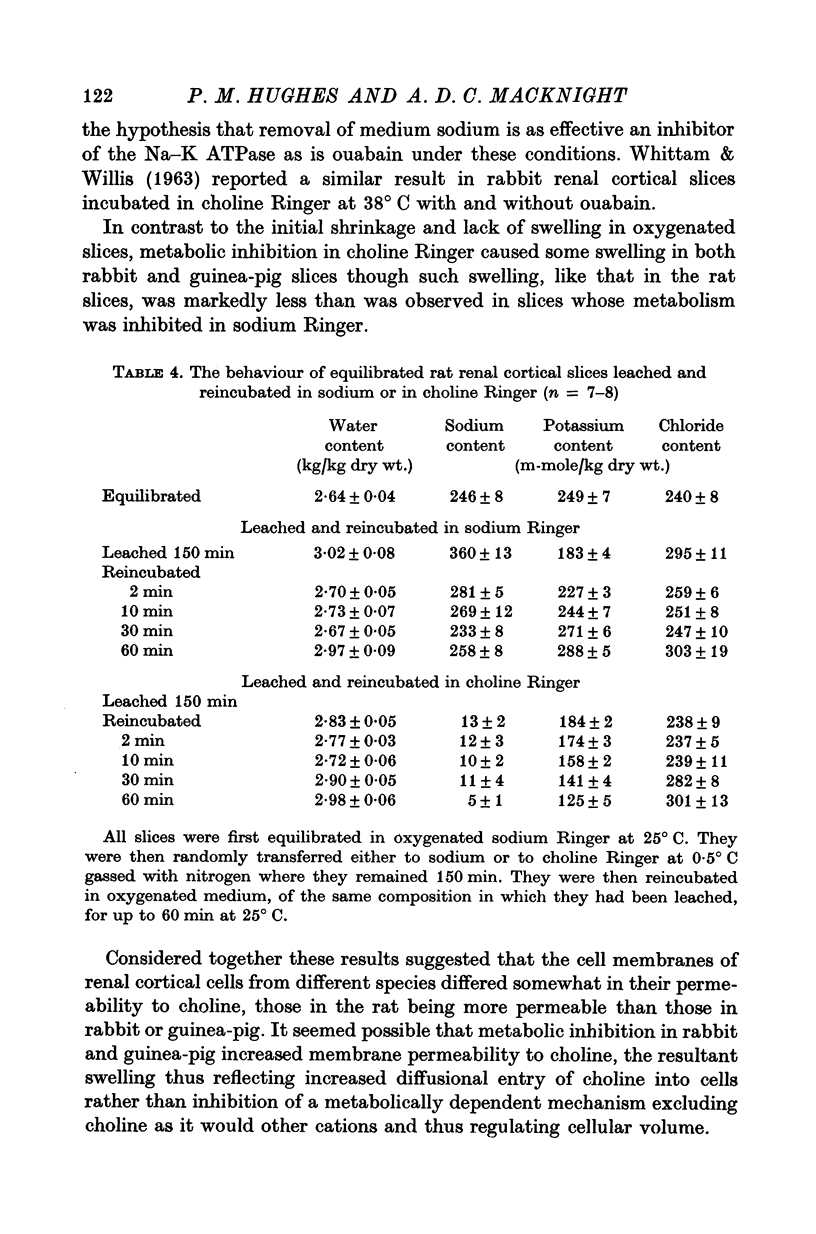
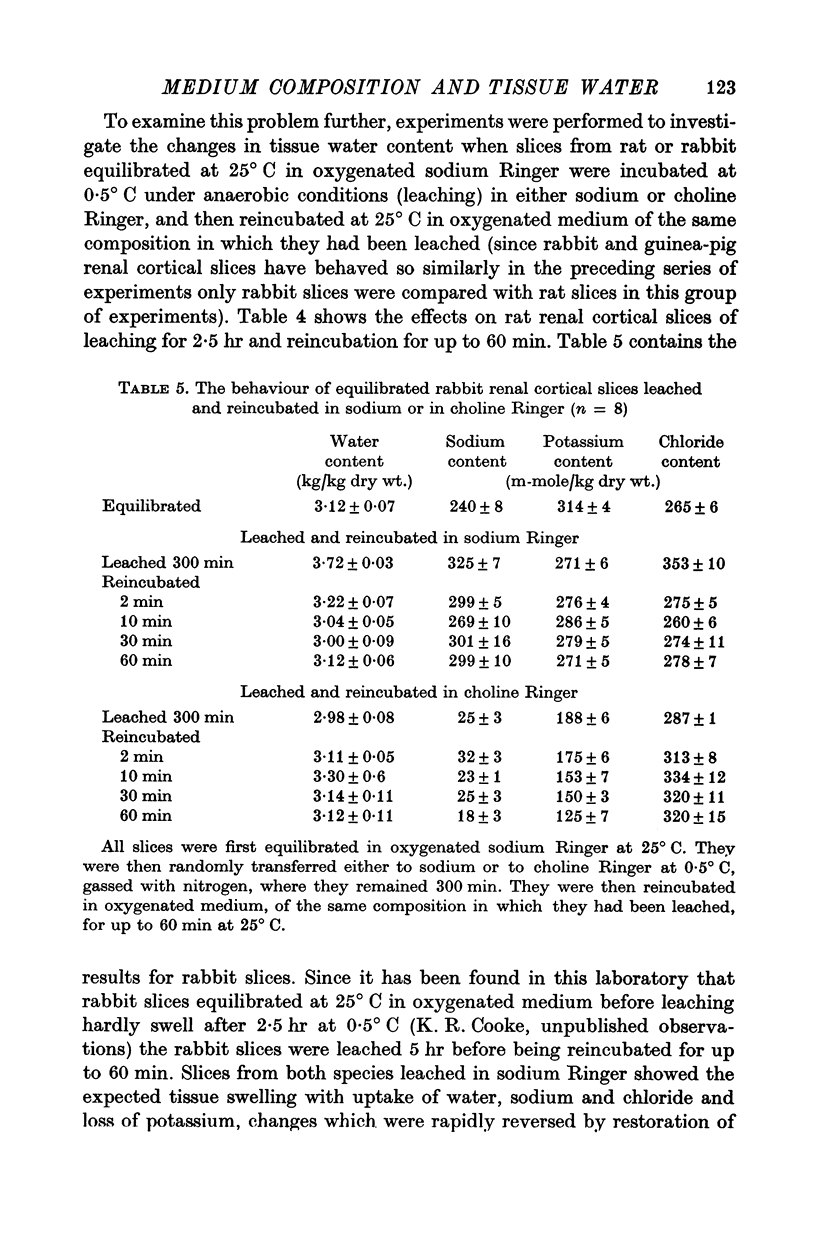
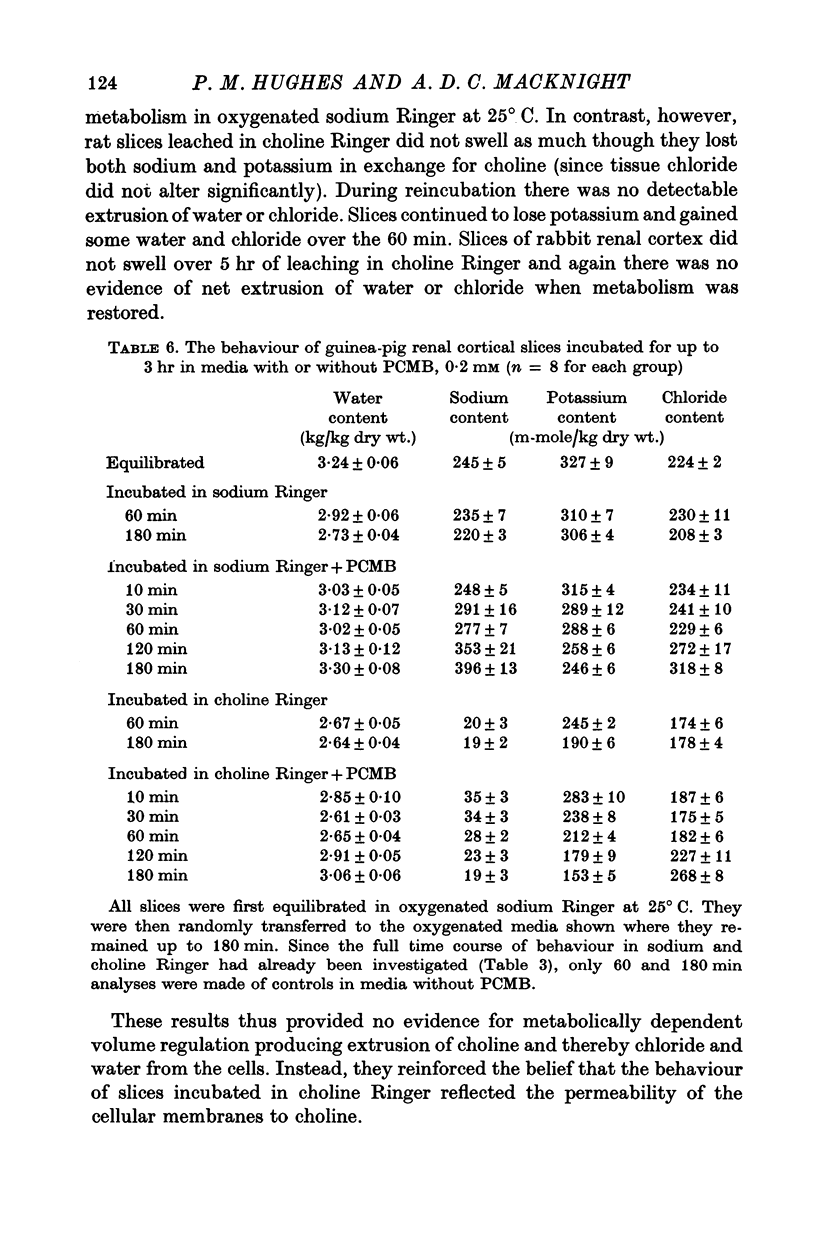
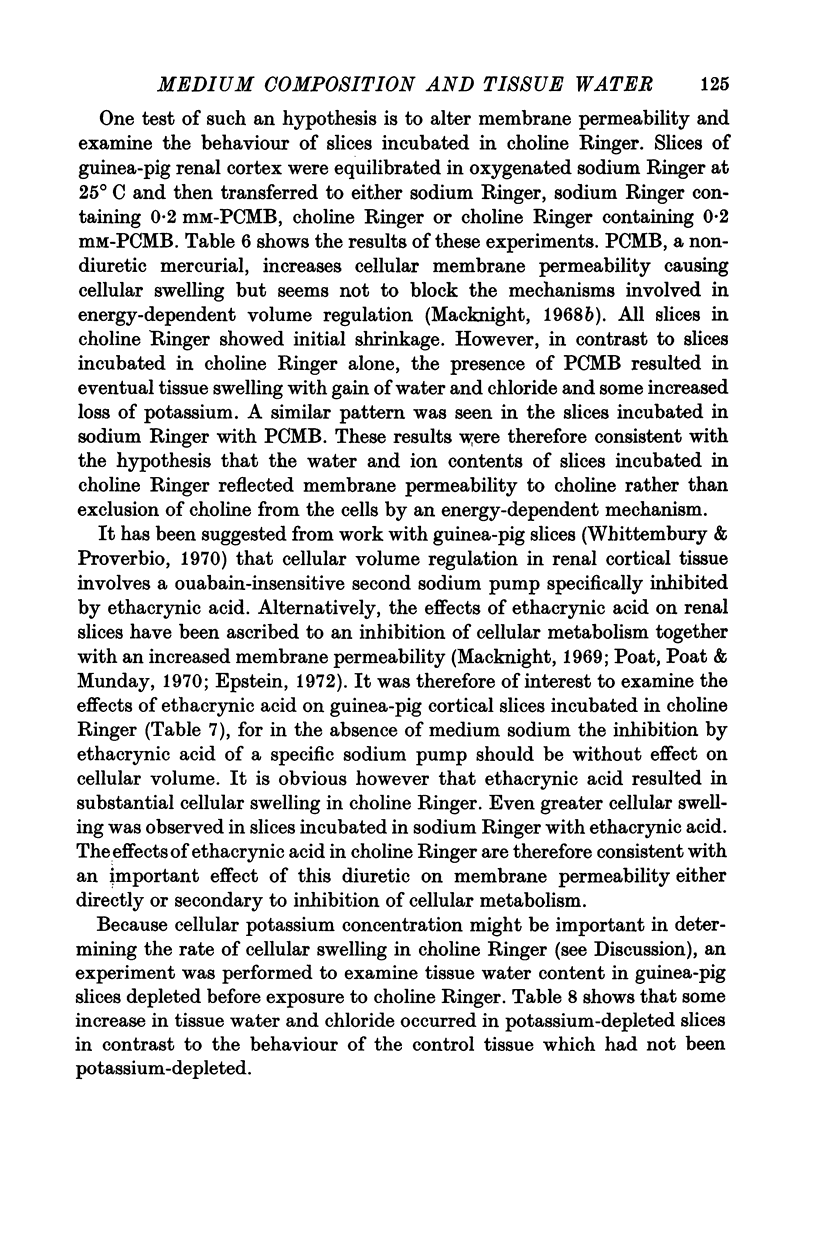
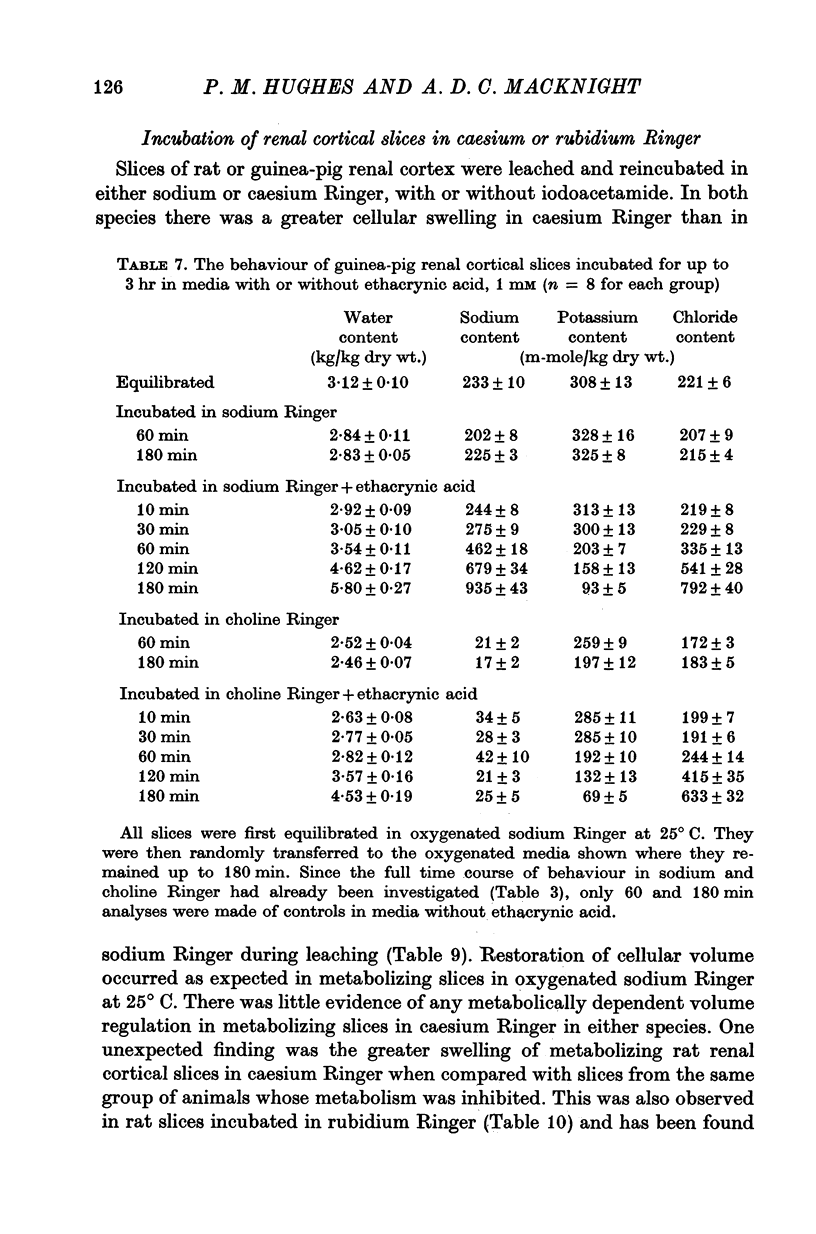
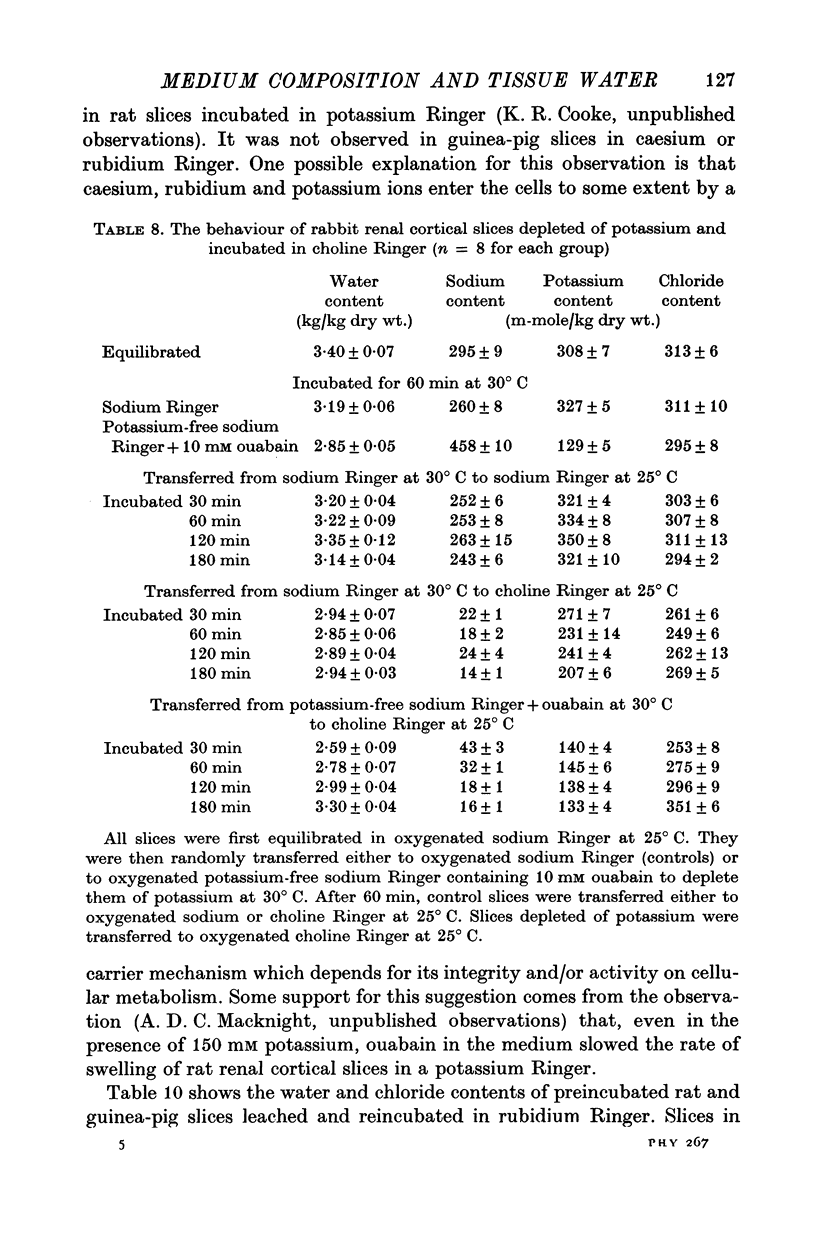
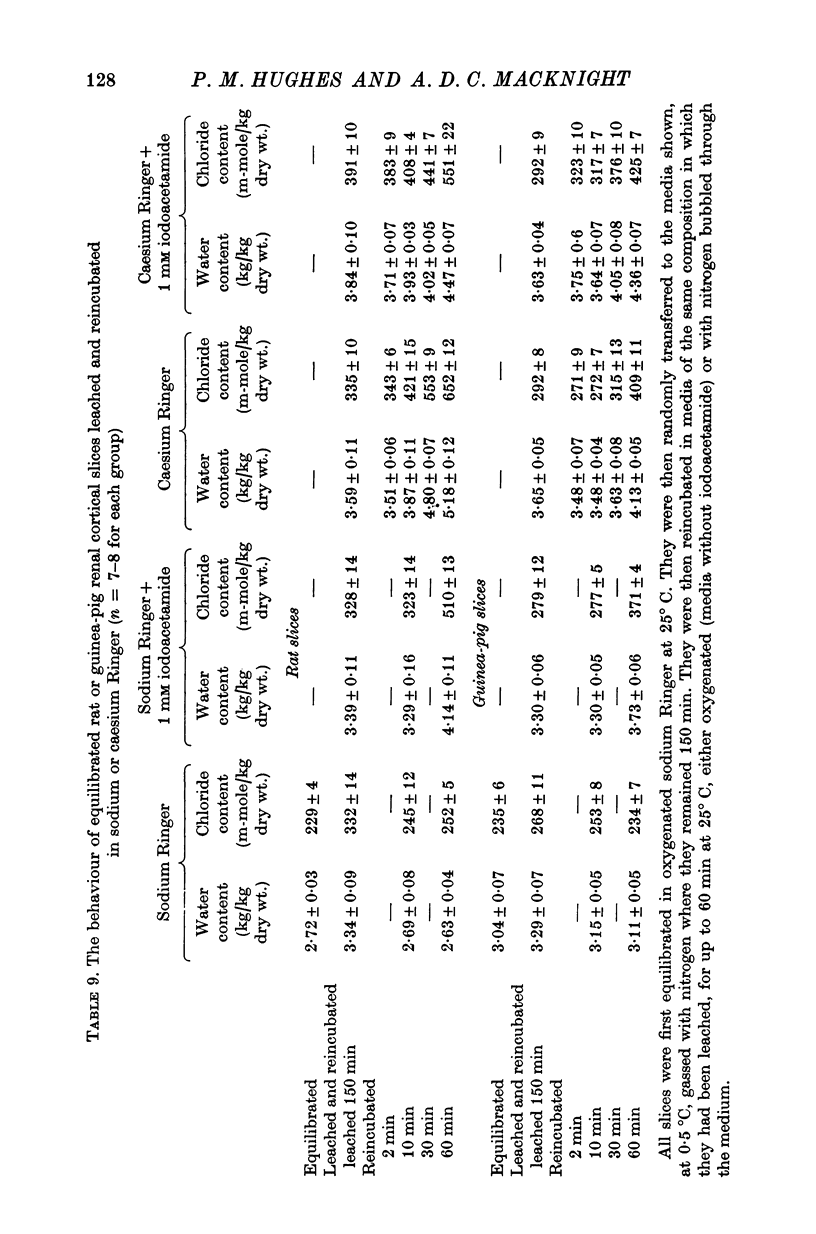
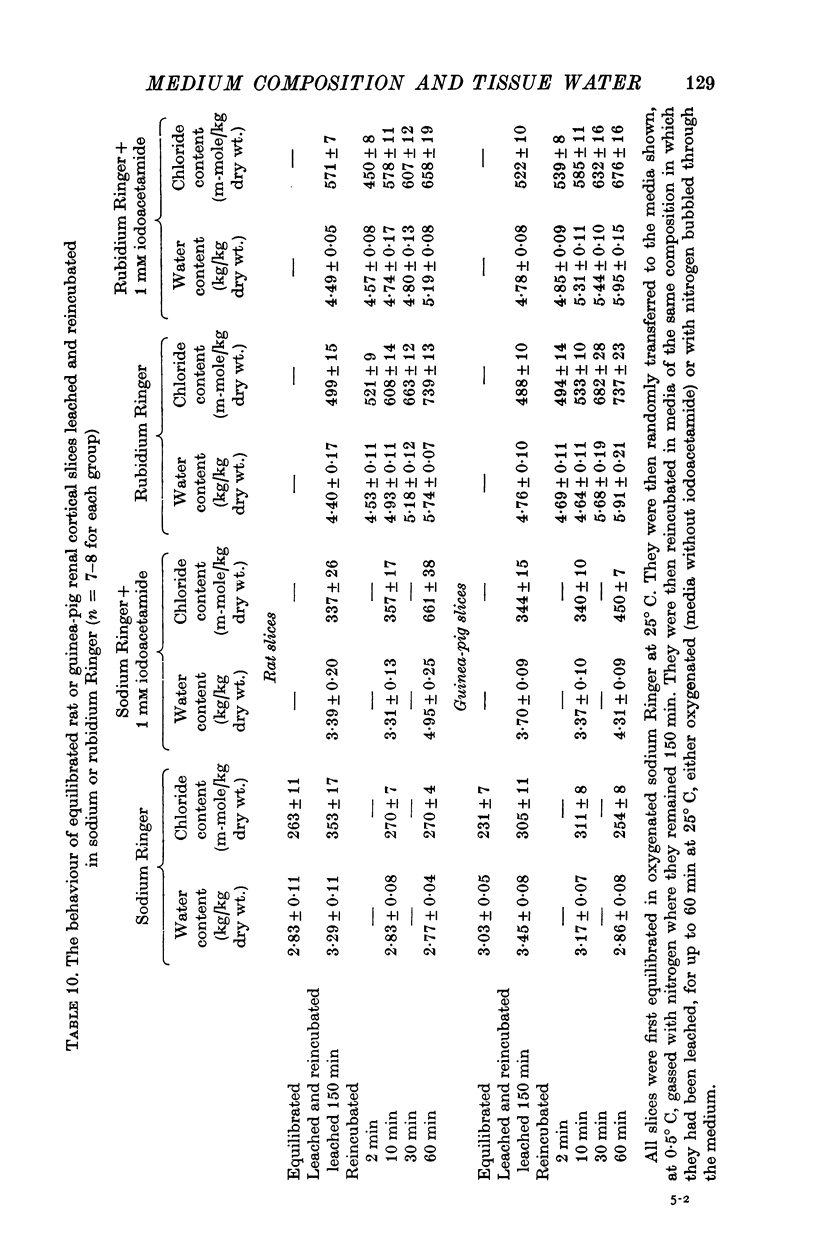
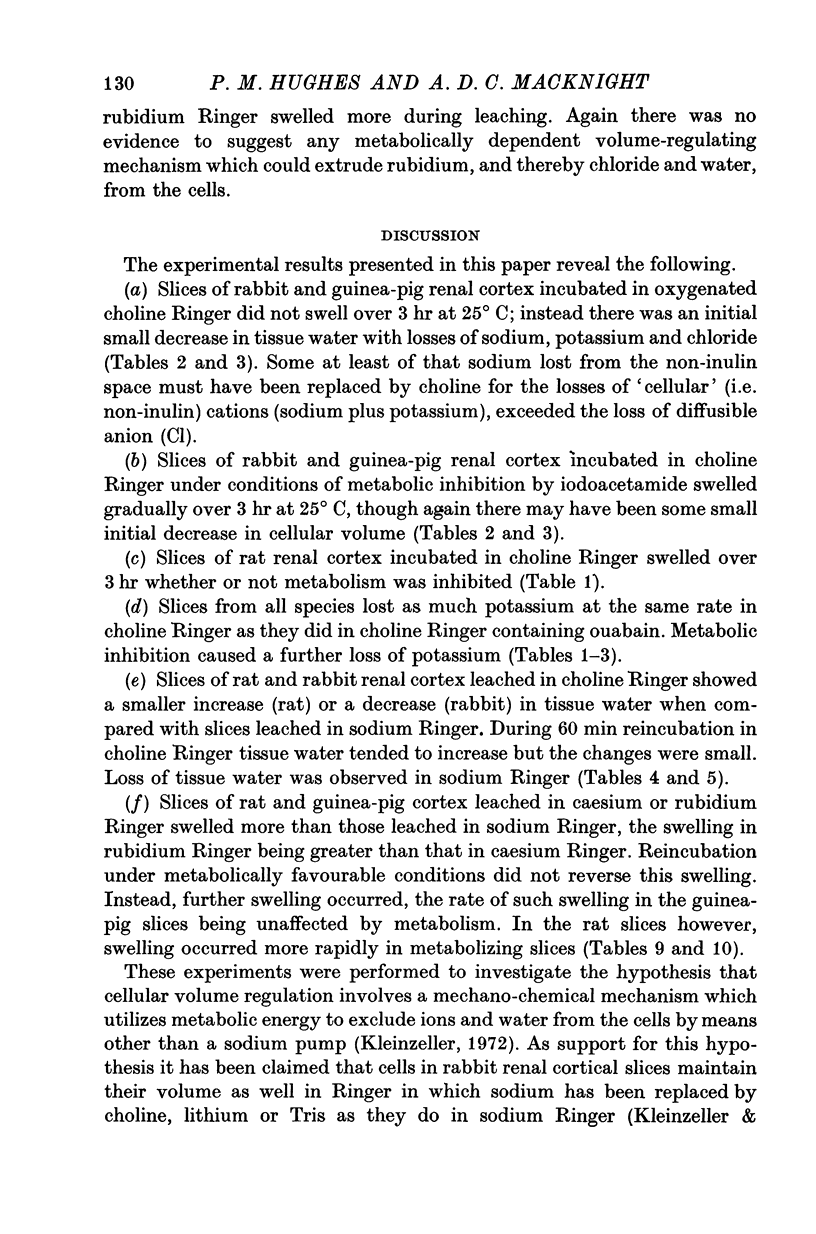
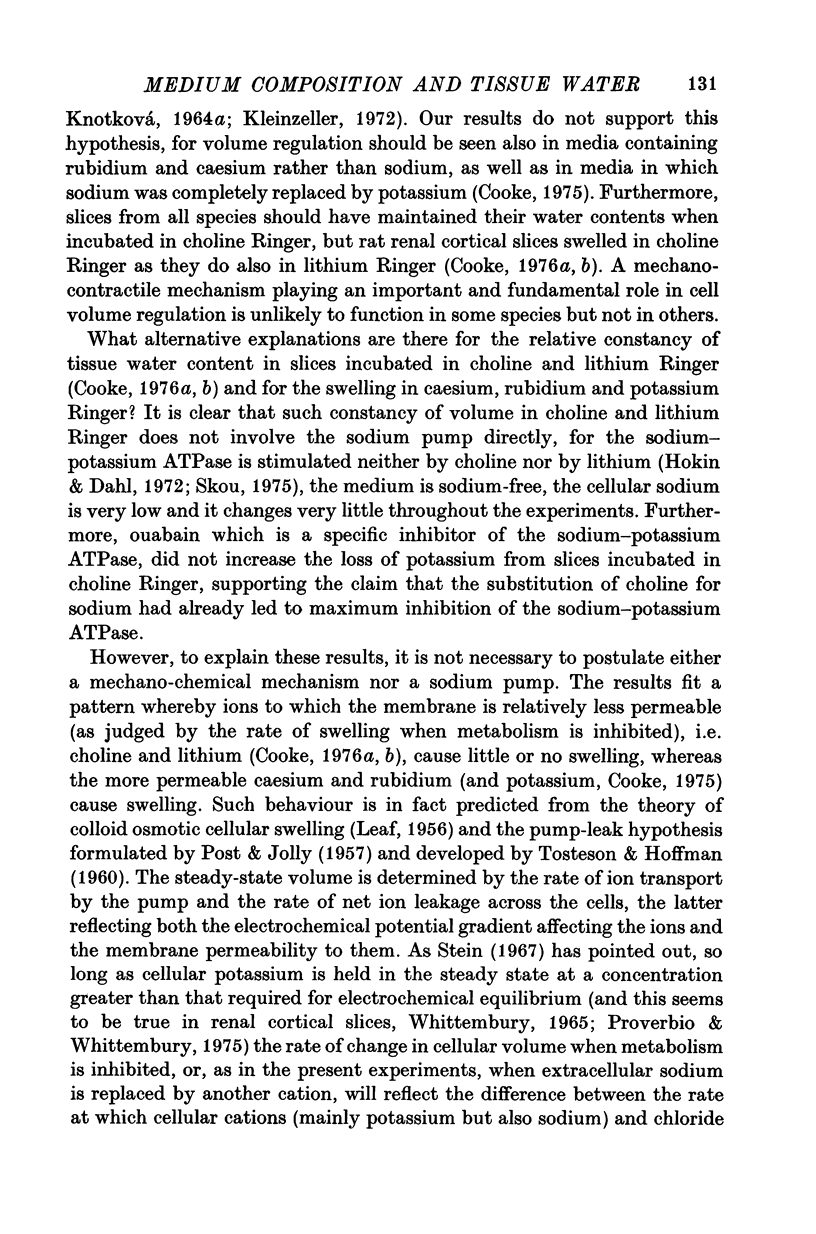
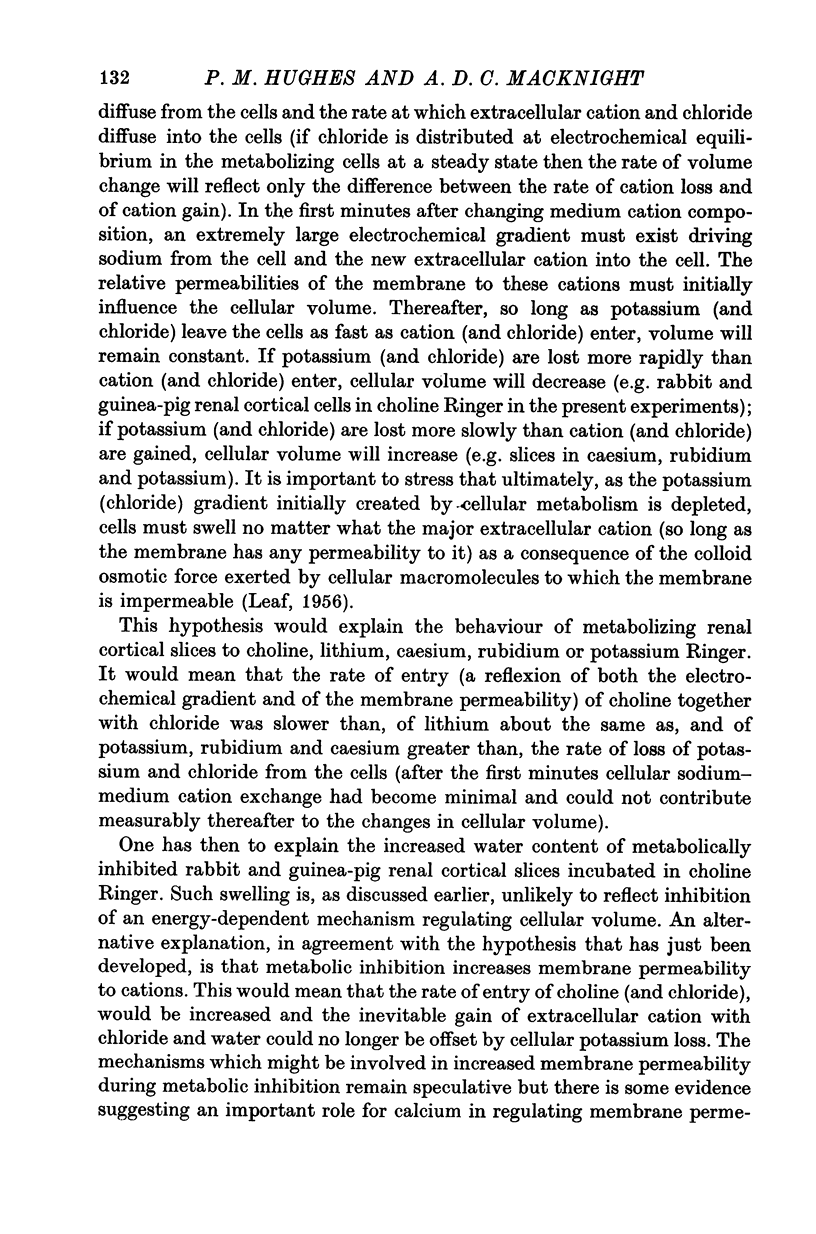
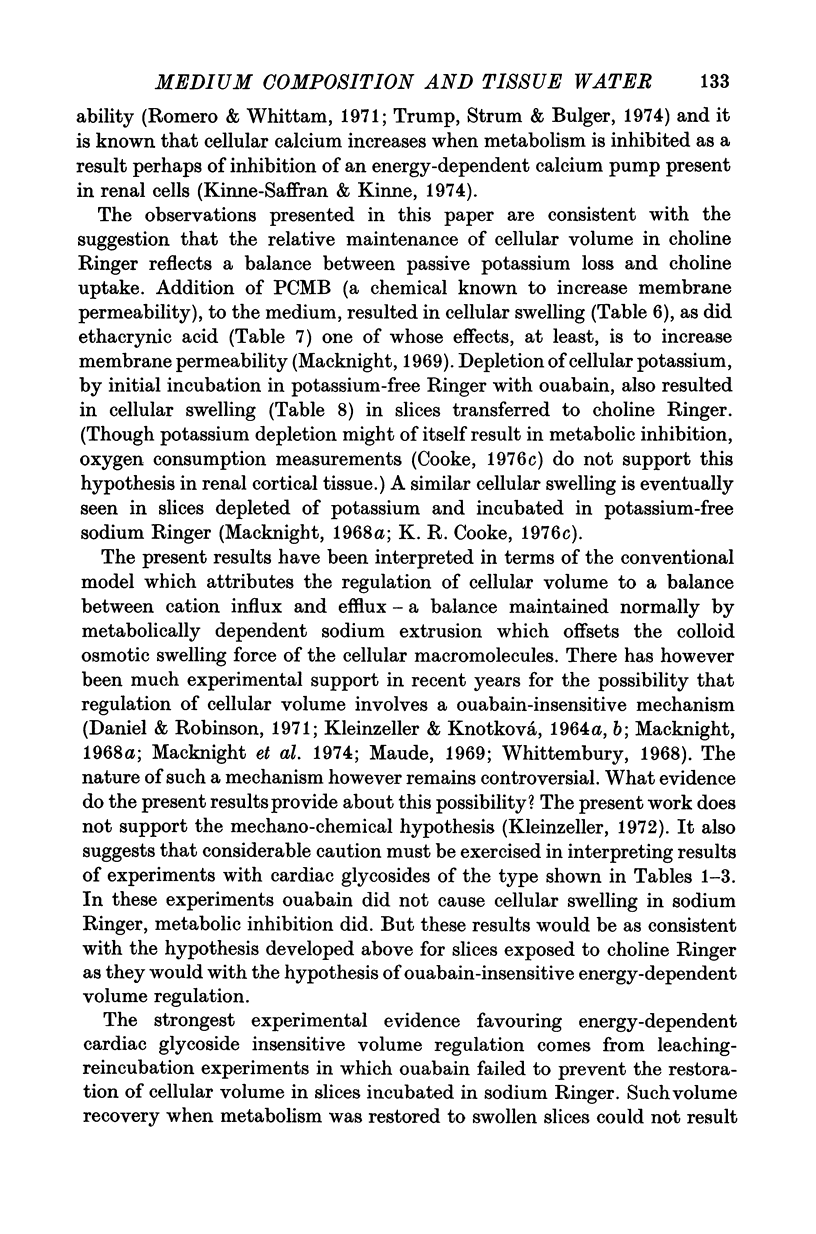
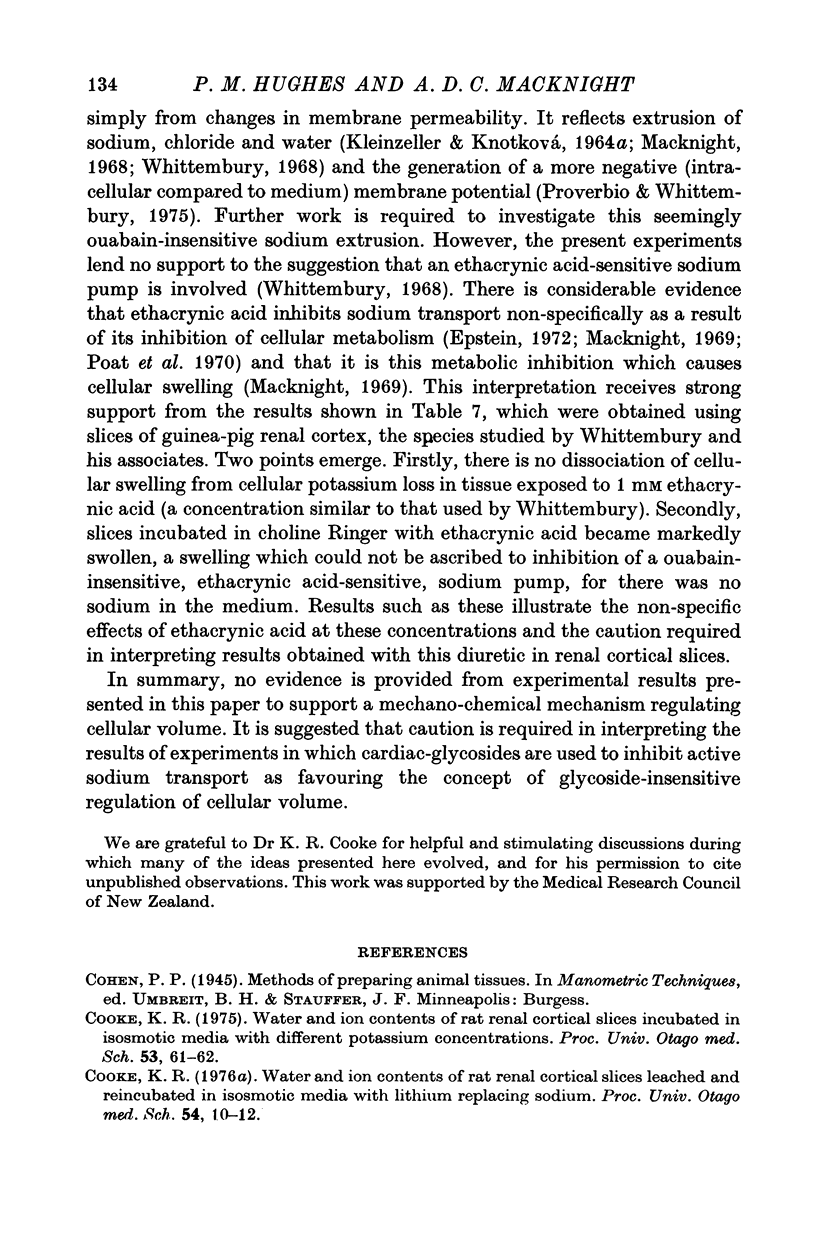
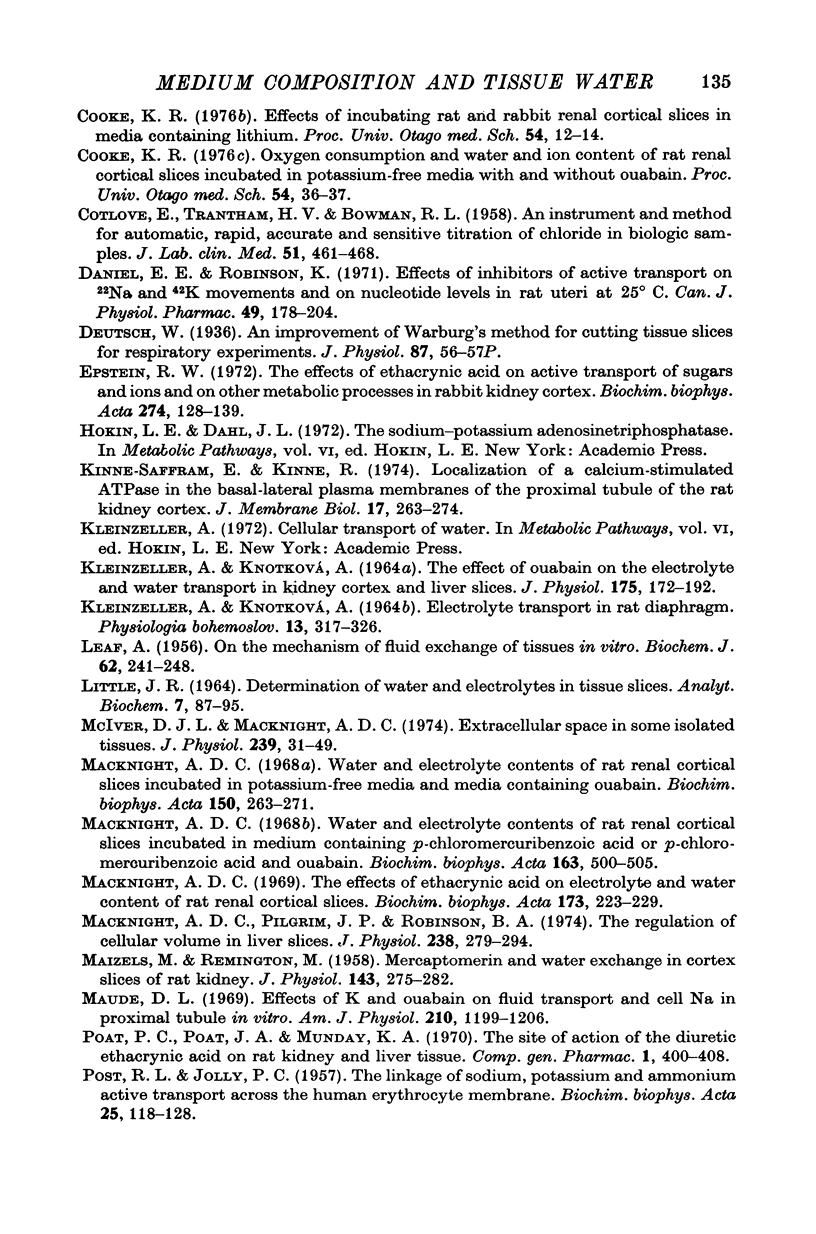
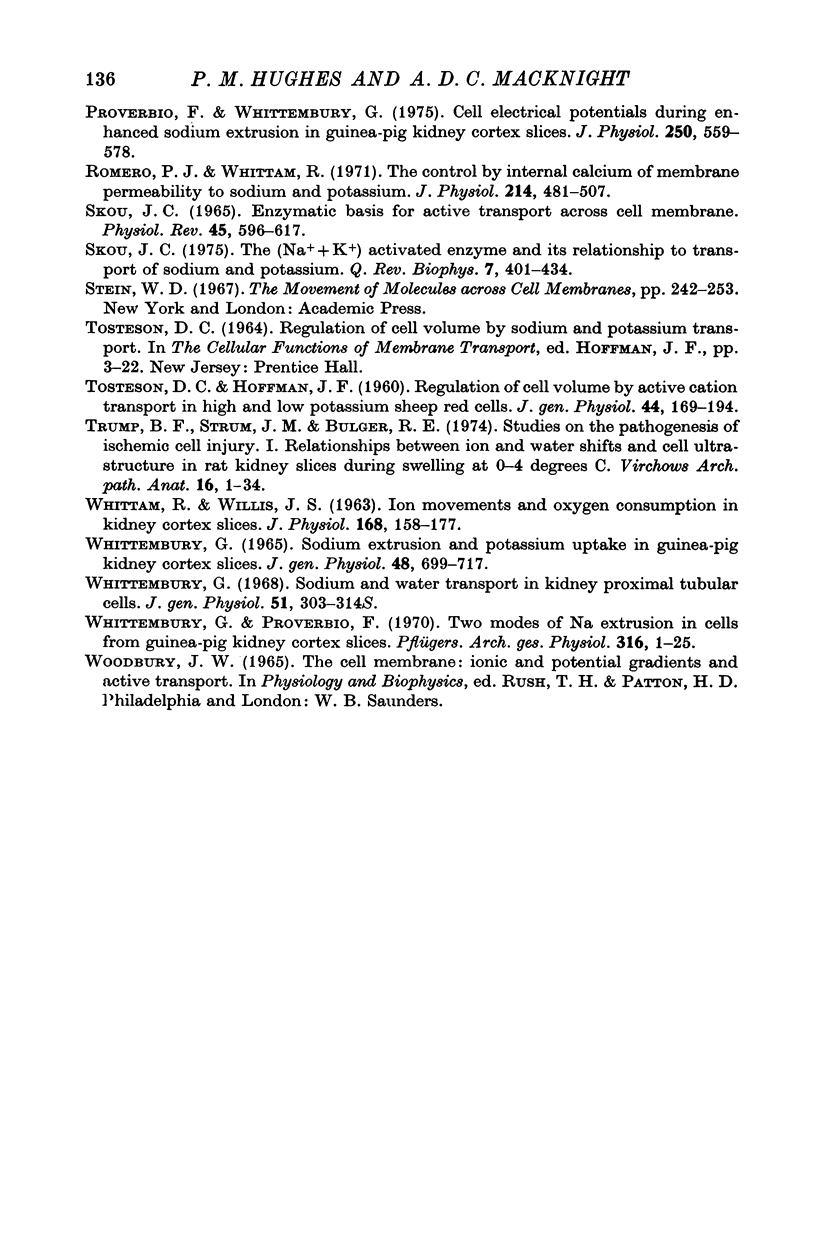
Selected References
These references are in PubMed. This may not be the complete list of references from this article.
- COTLOVE E., TRANTHAM H. V., BOWMAN R. L. An instrument and method for automatic, rapid, accurate, and sensitive titration of chloride in biologic samples. J Lab Clin Med. 1958 Mar;51(3):461–468. [PubMed] [Google Scholar]
- Daniel E. E., Robinson K. Effects of inhibitors of active transport on 22 Na and 42 K movements and on nucleotide levels in rat uteri at 25 degrees C. Can J Physiol Pharmacol. 1971 Mar;49(3):178–204. doi: 10.1139/y71-025. [DOI] [PubMed] [Google Scholar]
- Epstein R. W. The effects of ethacrynic acid on active transport of sugars and ions and on other metabolic processes in rabbit kidney cortex. Biochim Biophys Acta. 1972 Jul 3;274(1):128–139. doi: 10.1016/0005-2736(72)90288-x. [DOI] [PubMed] [Google Scholar]
- Glaister D., Kerly M. The oxygen consumption and carbohydrate metabolism of the retractor muscle of the foot of Mytilus edulis. J Physiol. 1936 Jun 10;87(1):56–66. doi: 10.1113/jphysiol.1936.sp003388. [DOI] [PMC free article] [PubMed] [Google Scholar]
- KLEINZELLER A., KNOTKOVA A. ELECTROLYTE TRANSPORT IN RAT DIAPHRAGM. Physiol Bohemoslov. 1964;13:317–326. [PubMed] [Google Scholar]
- KLEINZELLER A., KNOTKOVA A. THE EFFECT OF OUABAIN ON THE ELECTROLYTE AND WATER TRANSPORT IN KIDNEY CORTEX AND LIVER SLICES. J Physiol. 1964 Dec;175:172–192. doi: 10.1113/jphysiol.1964.sp007510. [DOI] [PMC free article] [PubMed] [Google Scholar]
- Kinne-Saffran E., Kinne R. Localization of a calcium-stimulated ATPase in the basal-lateral plasma membranes of the proximal tubule of rat kidney cortex. J Membr Biol. 1974 Jul 12;17(3):263–274. doi: 10.1007/BF01870187. [DOI] [PubMed] [Google Scholar]
- LEAF A. On the mechanism of fluid exchange of tissues in vitro. Biochem J. 1956 Feb;62(2):241–248. doi: 10.1042/bj0620241. [DOI] [PMC free article] [PubMed] [Google Scholar]
- LITTLE J. R. DETERMINATION OF WATER AND ELECTROLYTES IN TISSUE SLICES. Anal Biochem. 1964 Jan;7:87–95. doi: 10.1016/0003-2697(64)90122-8. [DOI] [PubMed] [Google Scholar]
- MAIZELS M., REMINGTON M. Mercaptomerin and water exchange in cortex slices of rat kidney. J Physiol. 1958 Sep 23;143(2):275–282. doi: 10.1113/jphysiol.1958.sp006058. [DOI] [PMC free article] [PubMed] [Google Scholar]
- Macknight A. D., Pilgrim J. P., Robinson B. A. The regulation of cellular volume in liver slices. J Physiol. 1974 Apr;238(2):279–294. doi: 10.1113/jphysiol.1974.sp010524. [DOI] [PMC free article] [PubMed] [Google Scholar]
- Macknight A. D. The effects of ethacrynic acid on the electrolyte and water contents of rat renal cortical slices. Biochim Biophys Acta. 1969 Mar 11;173(2):223–233. doi: 10.1016/0005-2736(69)90106-0. [DOI] [PubMed] [Google Scholar]
- Macknight A. D. Water and electrolyte contents of rat renal cortical slices incubated in medium containing p-chloromercuri-benzoic acid or p-chloromercuribenzoic acid and ouabain. Biochim Biophys Acta. 1968 Dec 10;163(4):500–505. doi: 10.1016/0005-2736(68)90079-5. [DOI] [PubMed] [Google Scholar]
- Macknight A. D. Water and electrolyte contents of rat renal cortical slices incubated in potassium-free media and media containing ouabain. Biochim Biophys Acta. 1968 Mar 1;150(2):263–270. doi: 10.1016/0005-2736(68)90169-7. [DOI] [PubMed] [Google Scholar]
- Maude D. L. Effects of K and ouabain on fluid transport and cell Na in proximal tubule in vitro. Am J Physiol. 1969 May;216(5):1199–1206. doi: 10.1152/ajplegacy.1969.216.5.1199. [DOI] [PubMed] [Google Scholar]
- McIver D. J., Macknight A. D. Extracellular space in some isolated tissues. J Physiol. 1974 May;239(1):31–49. doi: 10.1113/jphysiol.1974.sp010554. [DOI] [PMC free article] [PubMed] [Google Scholar]
- POST R. L., JOLLY P. C. The linkage of sodium, potassium, and ammonium active transport across the human erythrocyte membrane. Biochim Biophys Acta. 1957 Jul;25(1):118–128. doi: 10.1016/0006-3002(57)90426-2. [DOI] [PubMed] [Google Scholar]
- Poat P. C., Poat J. A., Munday K. A. The site of action of the diuretic ethacrynic acid on rat kidney and liver tissue. Comp Gen Pharmacol. 1970 Dec;1(4):400–408. doi: 10.1016/0010-4035(70)90064-9. [DOI] [PubMed] [Google Scholar]
- Proverbio F., Whittembury G. Cell electrical potentials during enhanced sodium extrusion in guinea-pig kidney cortex slices. J Physiol. 1975 Sep;250(3):559–578. doi: 10.1113/jphysiol.1975.sp011070. [DOI] [PMC free article] [PubMed] [Google Scholar]
- Romero P. J., Whittam R. The control by internal calcium of membrane permeability to sodium and potassium. J Physiol. 1971 May;214(3):481–507. doi: 10.1113/jphysiol.1971.sp009445. [DOI] [PMC free article] [PubMed] [Google Scholar]
- SKOU J. C. ENZYMATIC BASIS FOR ACTIVE TRANSPORT OF NA+ AND K+ ACROSS CELL MEMBRANE. Physiol Rev. 1965 Jul;45:596–617. doi: 10.1152/physrev.1965.45.3.596. [DOI] [PubMed] [Google Scholar]
- Skou J. C. The (Na++K+) activated enzyme system and its relationship to transport of sodium and potassium. Q Rev Biophys. 1974 Jul;7(3):401–434. doi: 10.1017/s0033583500001475. [DOI] [PubMed] [Google Scholar]
- TOSTESON D. C., HOFFMAN J. F. Regulation of cell volume by active cation transport in high and low potassium sheep red cells. J Gen Physiol. 1960 Sep;44:169–194. doi: 10.1085/jgp.44.1.169. [DOI] [PMC free article] [PubMed] [Google Scholar]
- Trump B. F., Strum J. M., Bulger R. E. Studies on the pathogenesis of ischemic cell injury. I. Relations between ion and water shifts and cell ultrastructure in rat kidney slices during swelling at 0-4 degrees C. Virchows Arch B Cell Pathol. 1974;16(1):1–34. doi: 10.1007/BF02894060. [DOI] [PubMed] [Google Scholar]
- WHITTAM R., WILLIS J. S. ION MOVEMENTS AND OXYGEN CONSUMPTION IN KIDNEY CORTEX SLICES. J Physiol. 1963 Aug;168:158–177. doi: 10.1113/jphysiol.1963.sp007184. [DOI] [PMC free article] [PubMed] [Google Scholar]
- WHITTEMBURY G. SODIUM EXTRUSION AND POTASSIUM UPTAKE IN GUINEA PIG KIDNEY CORTEX SLICES. J Gen Physiol. 1965 Mar;48:699–717. doi: 10.1085/jgp.48.4.699. [DOI] [PMC free article] [PubMed] [Google Scholar]
- Whittembury G., Proverbio F. Two modes of Na extrusion in cells from guinea pig kidney cortex slices. Pflugers Arch. 1970;316(1):1–25. doi: 10.1007/BF00587893. [DOI] [PubMed] [Google Scholar]