Abstract
1. The time sequence of the development of acetylcholinesterase (AChE), acetylcholine (ACh) receptors and functional synapses on the embryonic muscle membrane in a tunicate larva (Halocynthia roretzi) was investigated in vivo.
2. The fertilized tunicate egg was incubated in natural sea water at 9° C. Sixty-eight hr after fertilization the free-swimming larva was hatched, which had six striated muscle fibres in the tail. The developmental stage of the embryo was indicated by the developmental hours after fertilization.
3. The transmitter at the neuromuscular junction in the hatched larva is ACh. (i) Neuromuscular transmission was completely blocked by D-tubocurarine (1-5 × 10-5 M). (ii) Eserine (5-10 × 10-7 M) approximately doubled the time constant of the falling phase of miniature excitatory junctional currents (e.j.c.s). (iii) The reversal potential of the membrane response to iontophoretically applied ACh was -10 mV and similar to that of e.j.c.s. (iv) AChE was present on the muscle membrane surface.
4. AChE activity became visible histochemically on the embryonic cell membrane in the presumptive muscle region as early as the late gastrula stage (27 hr after fertilization, 12 hr before the ACh response appeared).
5. The response to iontophoretically applied ACh was present at 39 hr after fertilization but could not be evoked at 38 hr.
6. Between 39 and 41 hr after fertilization, the ACh responses increased rapidly, then remained relatively unchanged until larval hatching.
7. The stage of the initial appearance of the ACh response corresponded to the stage when the Ca current abruptly increased in the muscle membrane.
8. The first sign of neuromuscular transmission was appearance of a giant excitatory junctional potential (e.j.p.) with uniform amplitude (about 15-20 mV) and slow time course (time constant of the falling phase of a giant e.j.c. was 23·4 ± 6·9 msec, mean and S.D., at -60 mV and 11° C).
9. Within a few hours, these giant e.j.p.s disappeared and were successively replaced by medium-sized e.j.p.s and then e.j.p.s similar to those seen in hatched larvae (time constant of the falling phase of a miniature e.j.c. was 8·5 ± 1·8 msec at -60 mV and 11° C).
Full text
PDF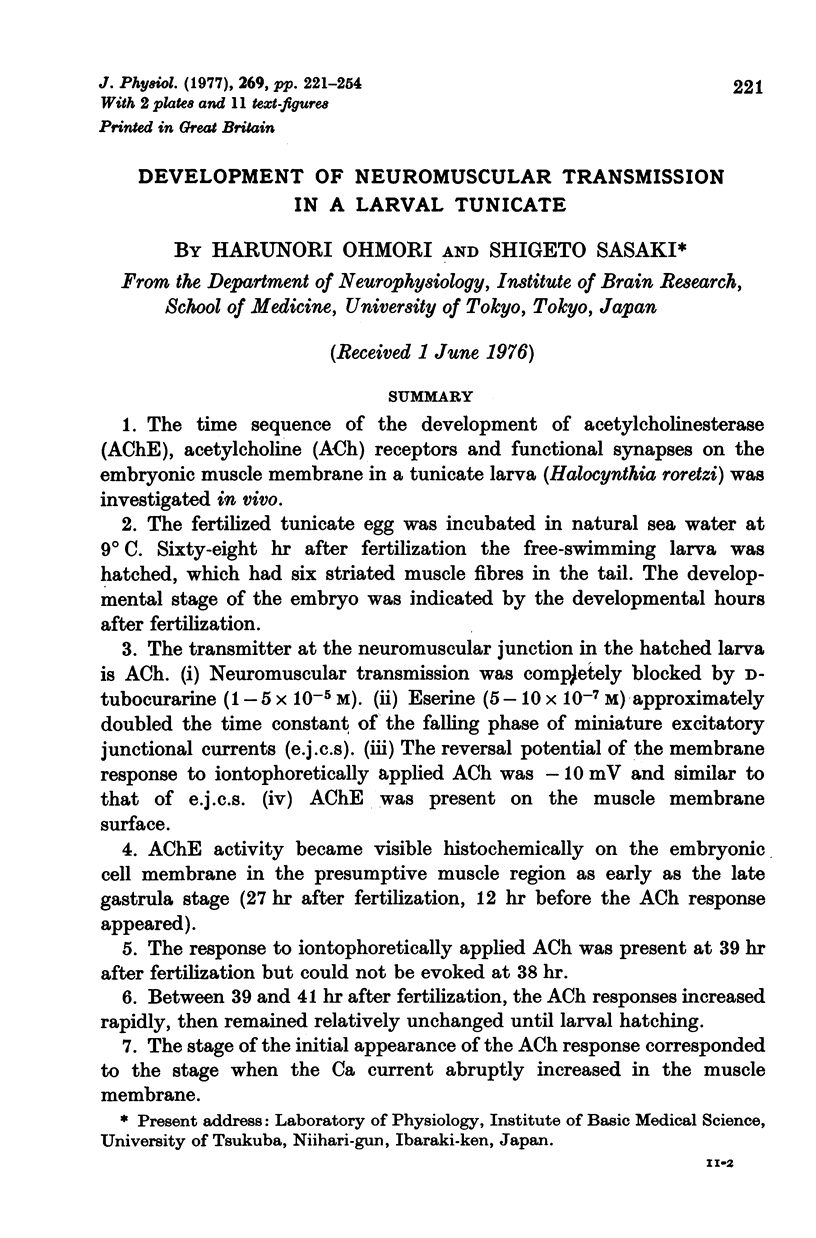
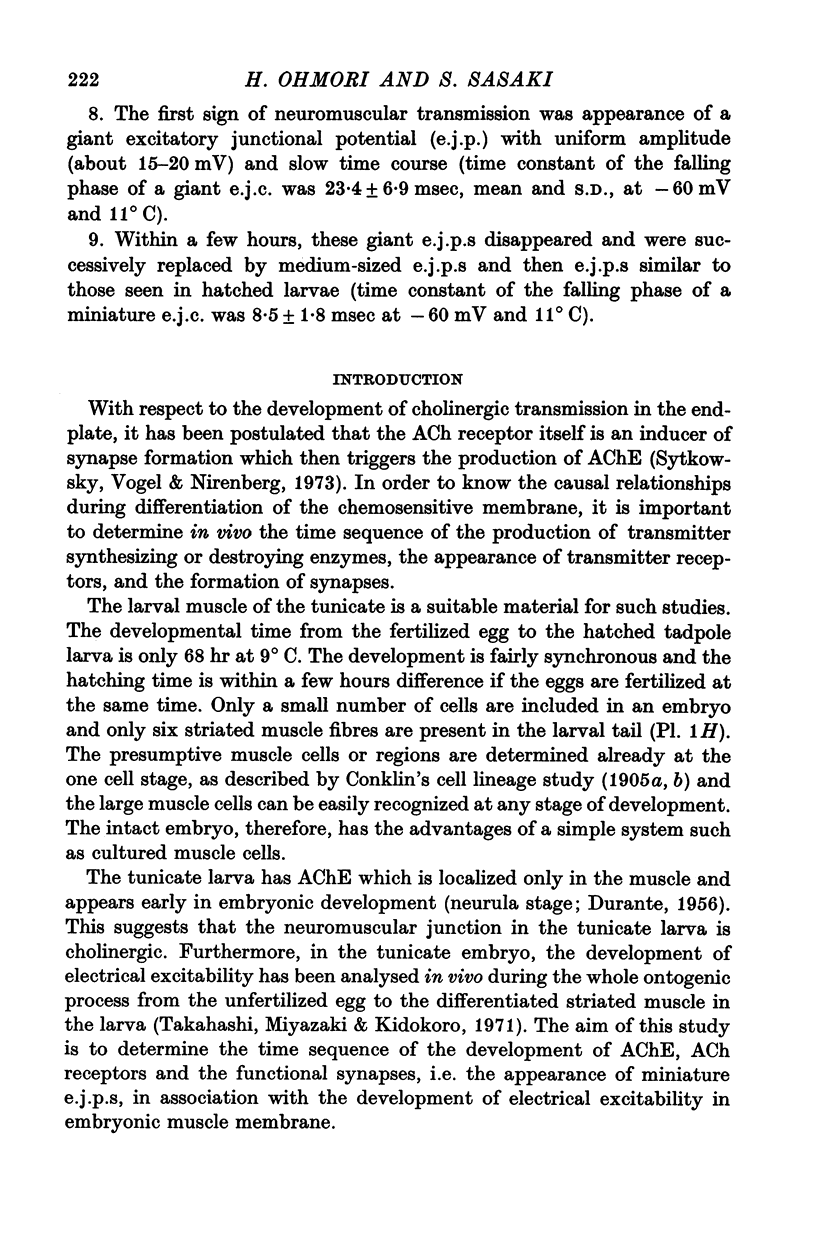
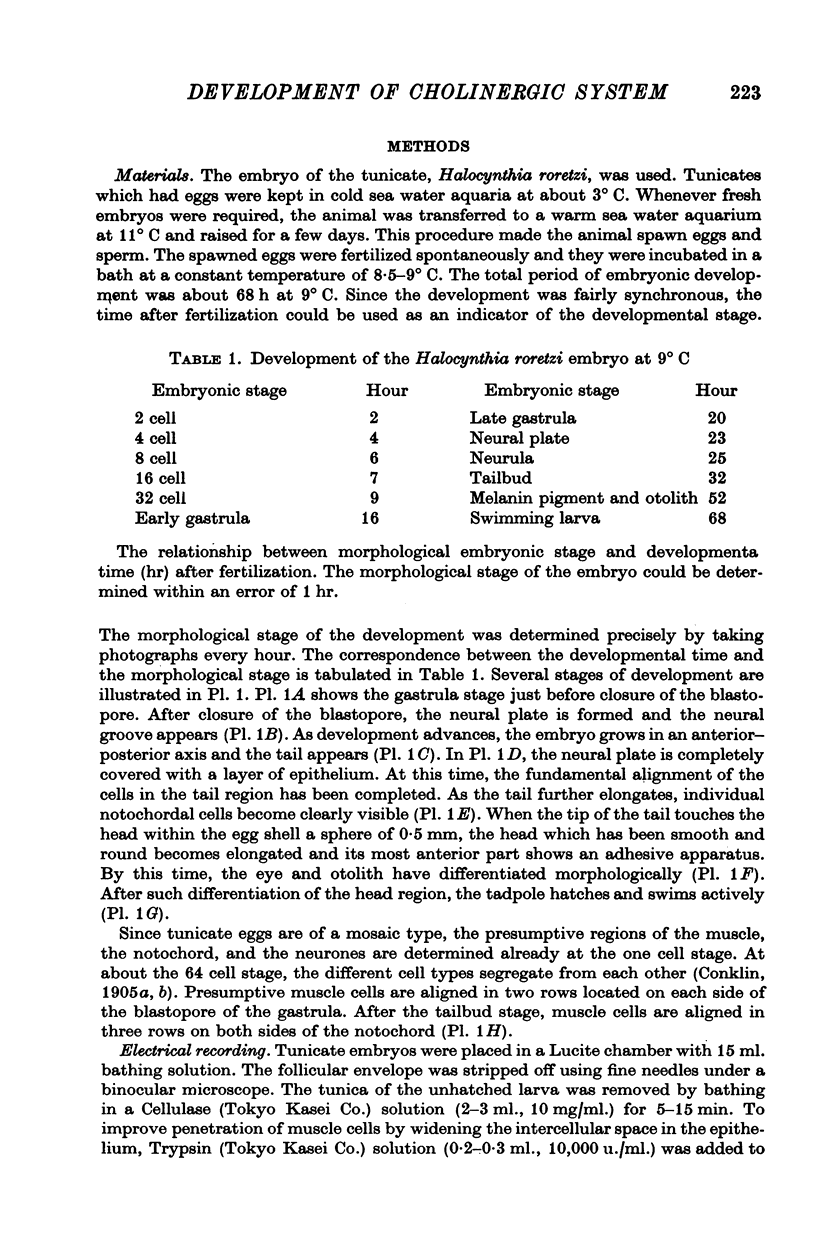
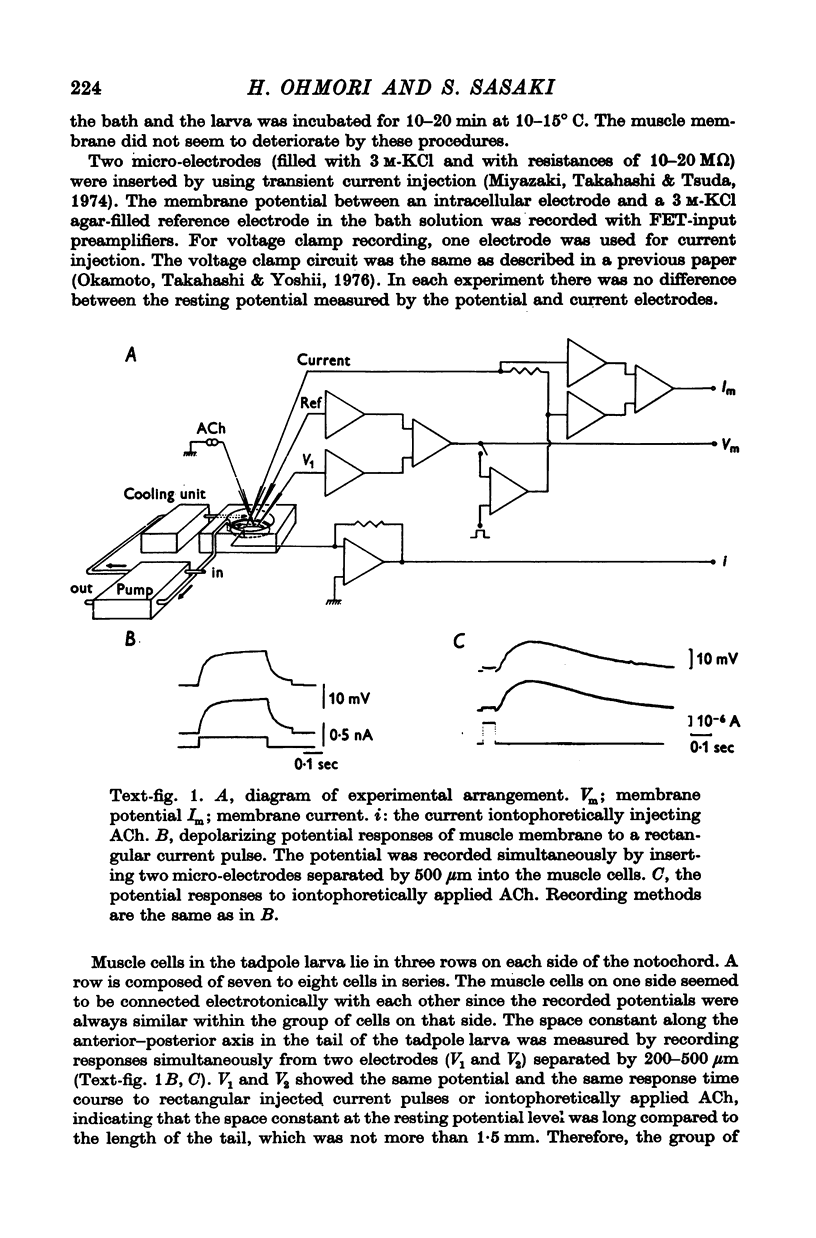
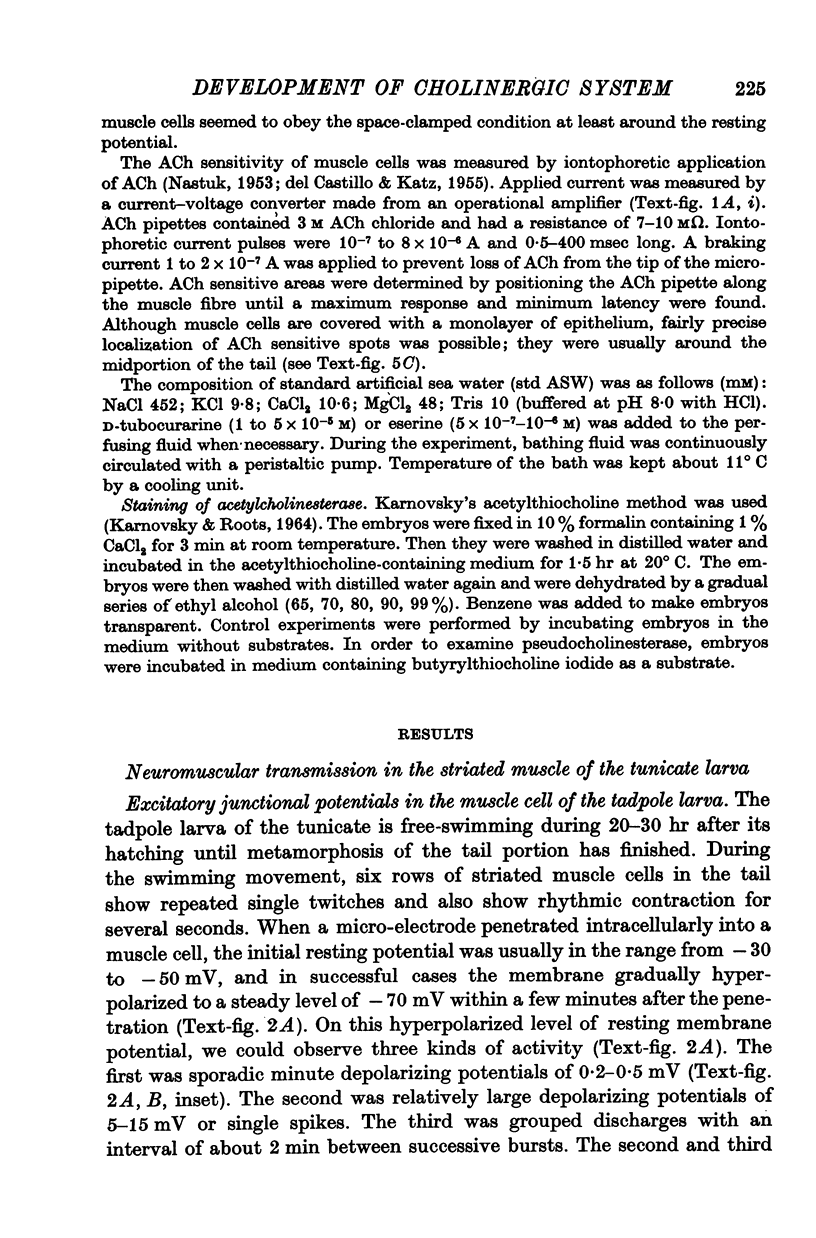
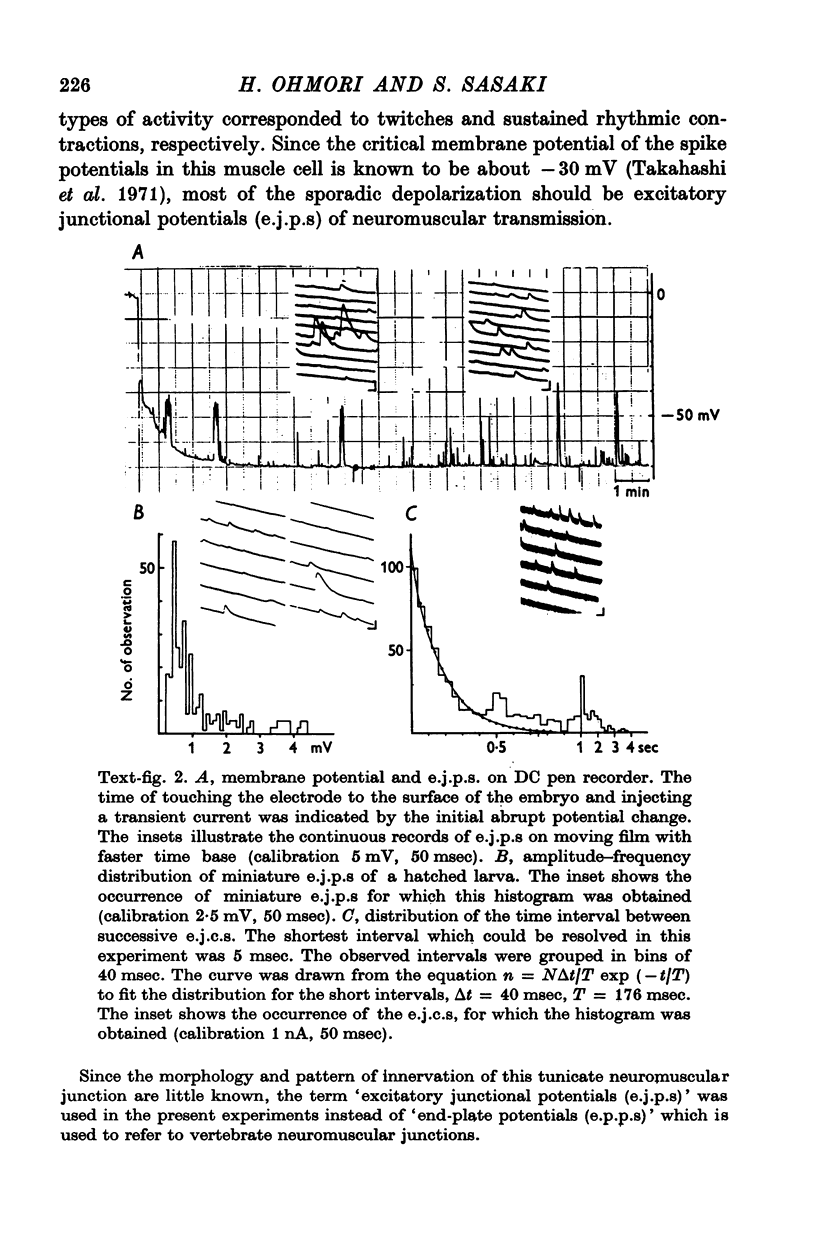
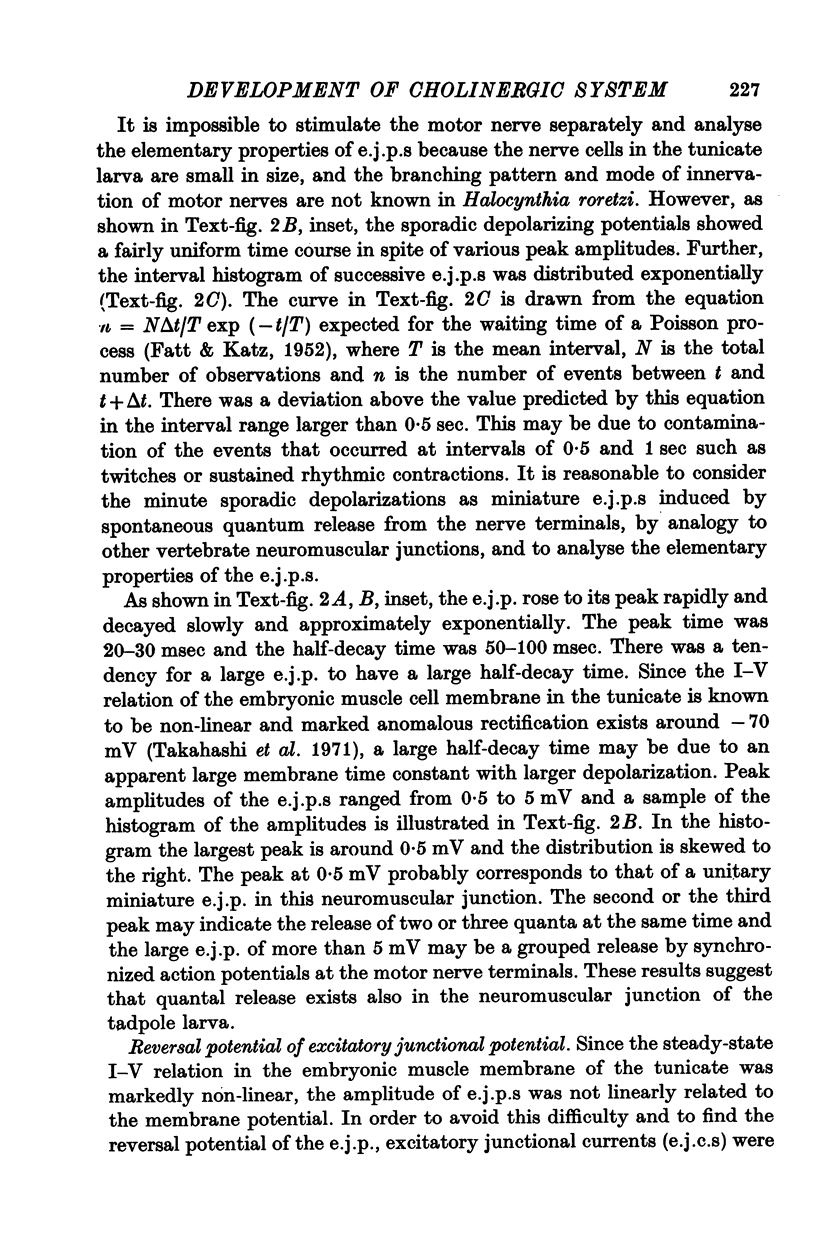
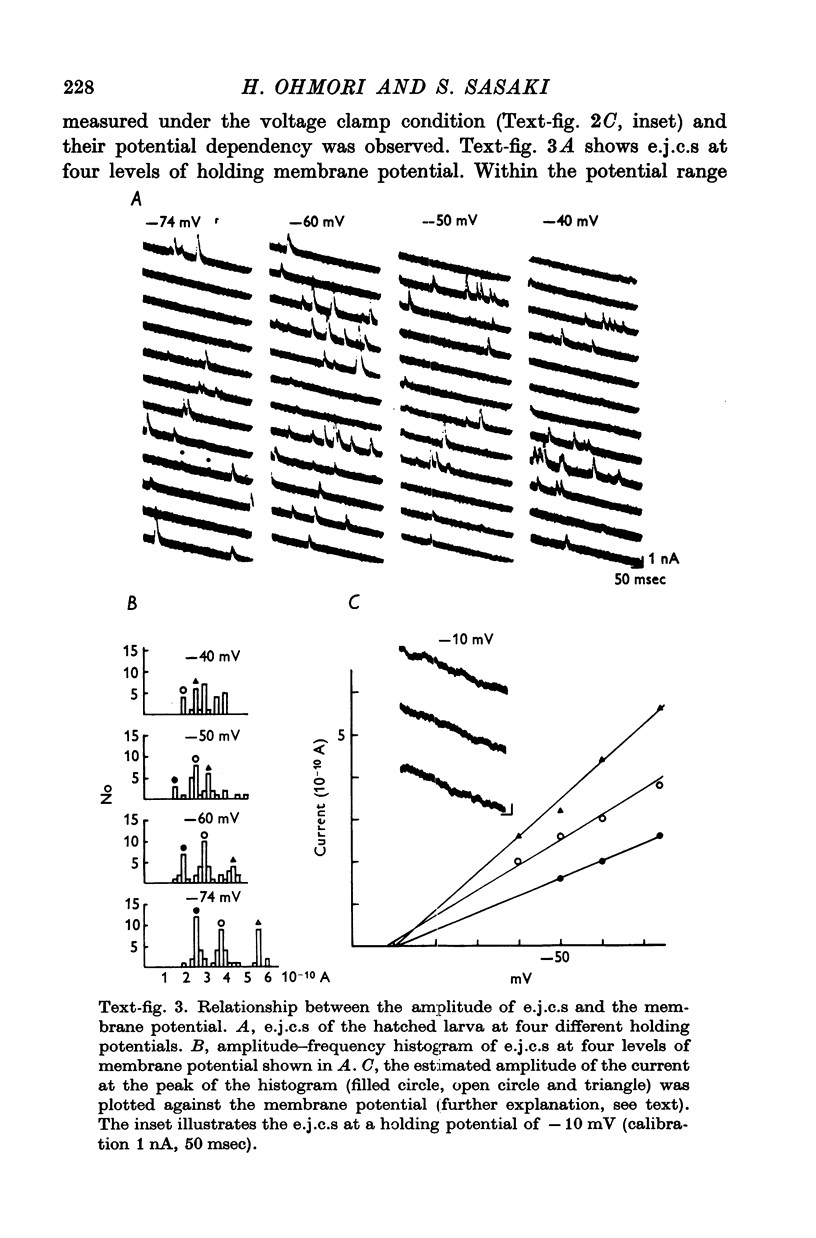
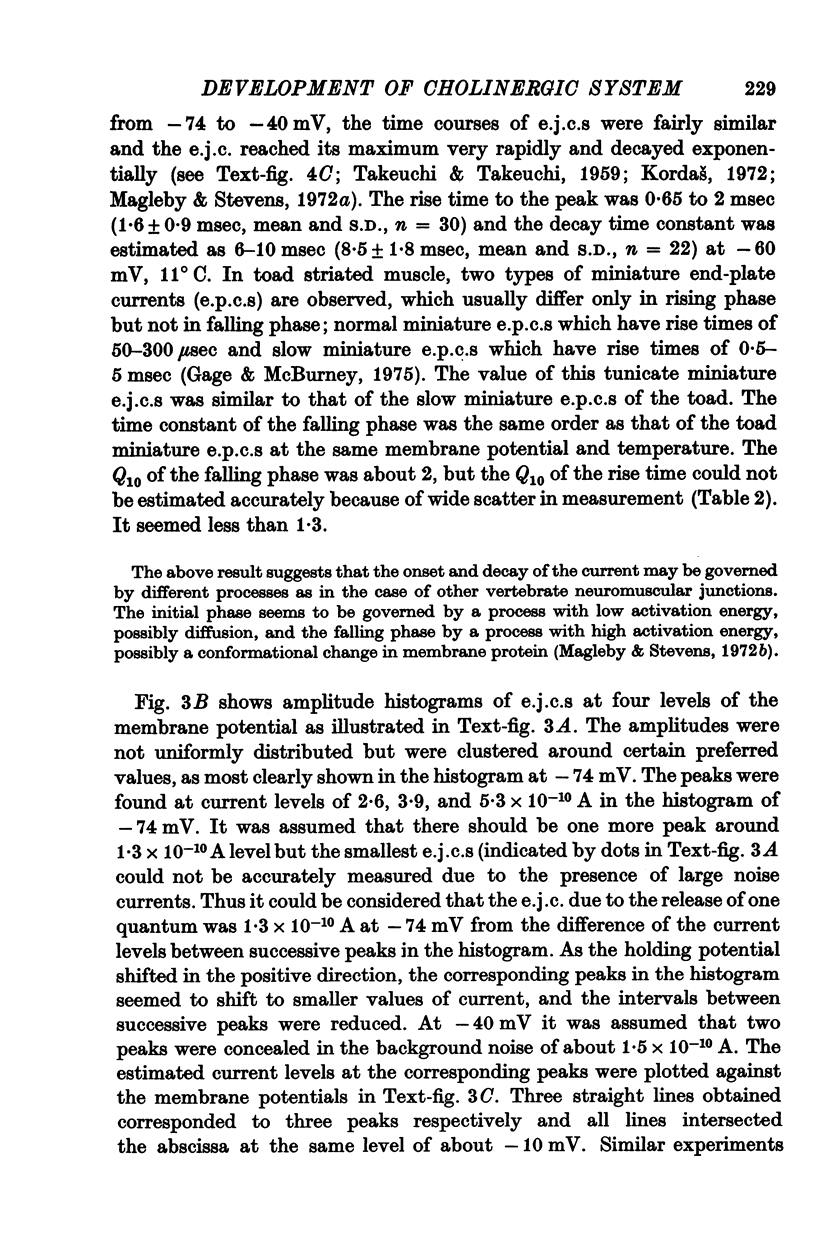
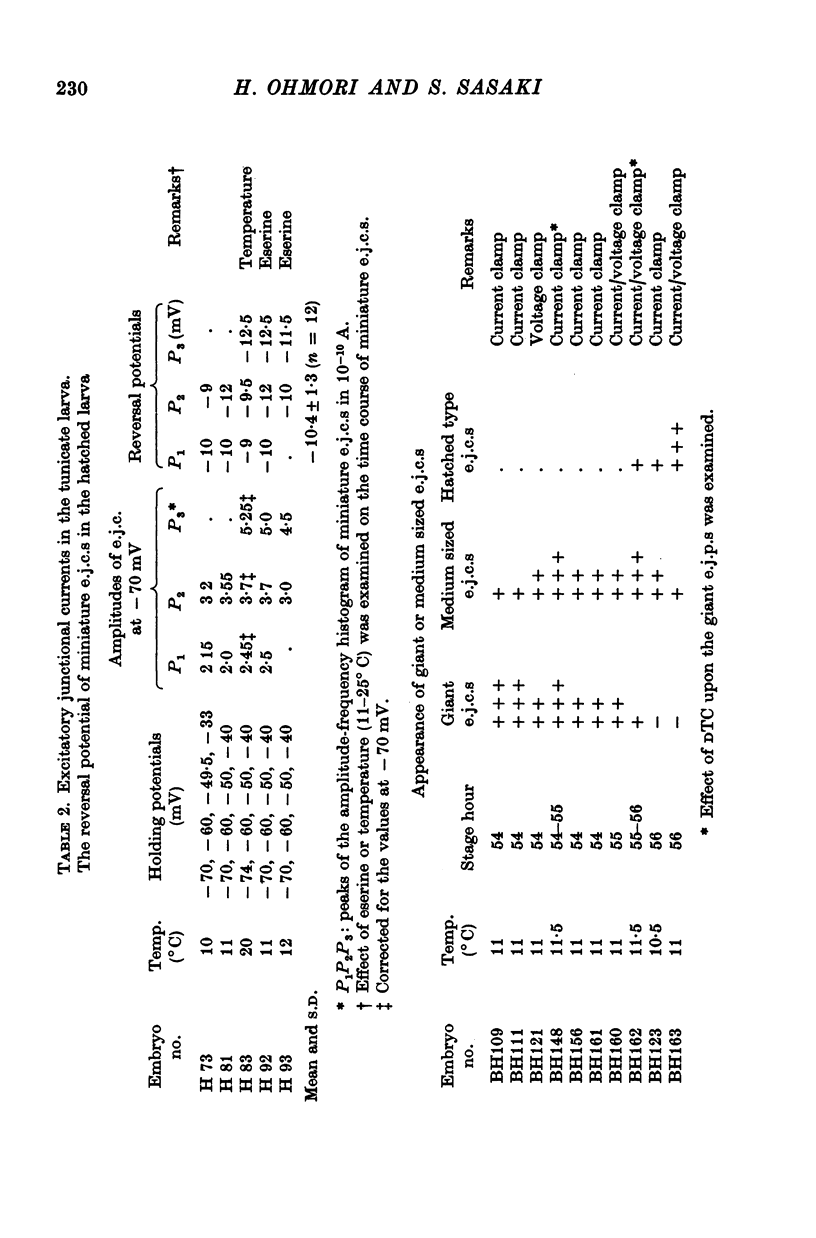
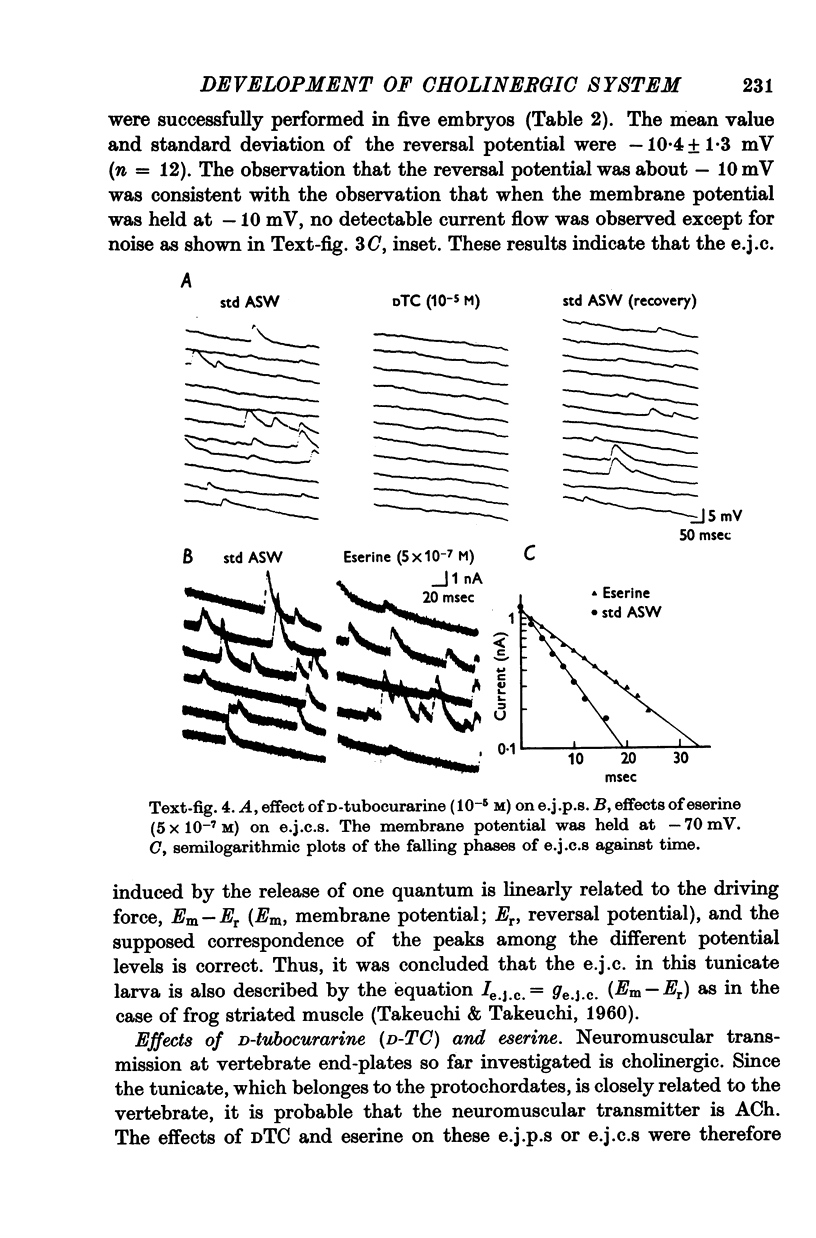
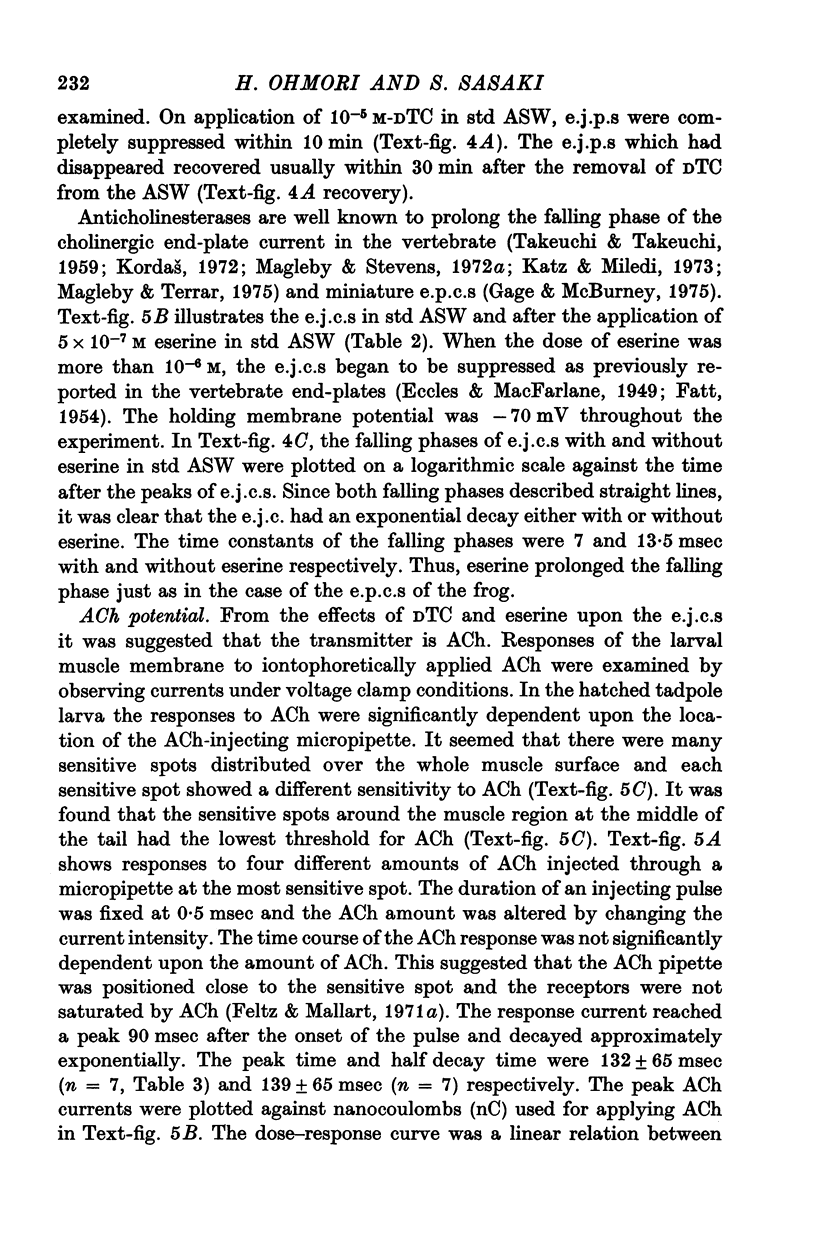
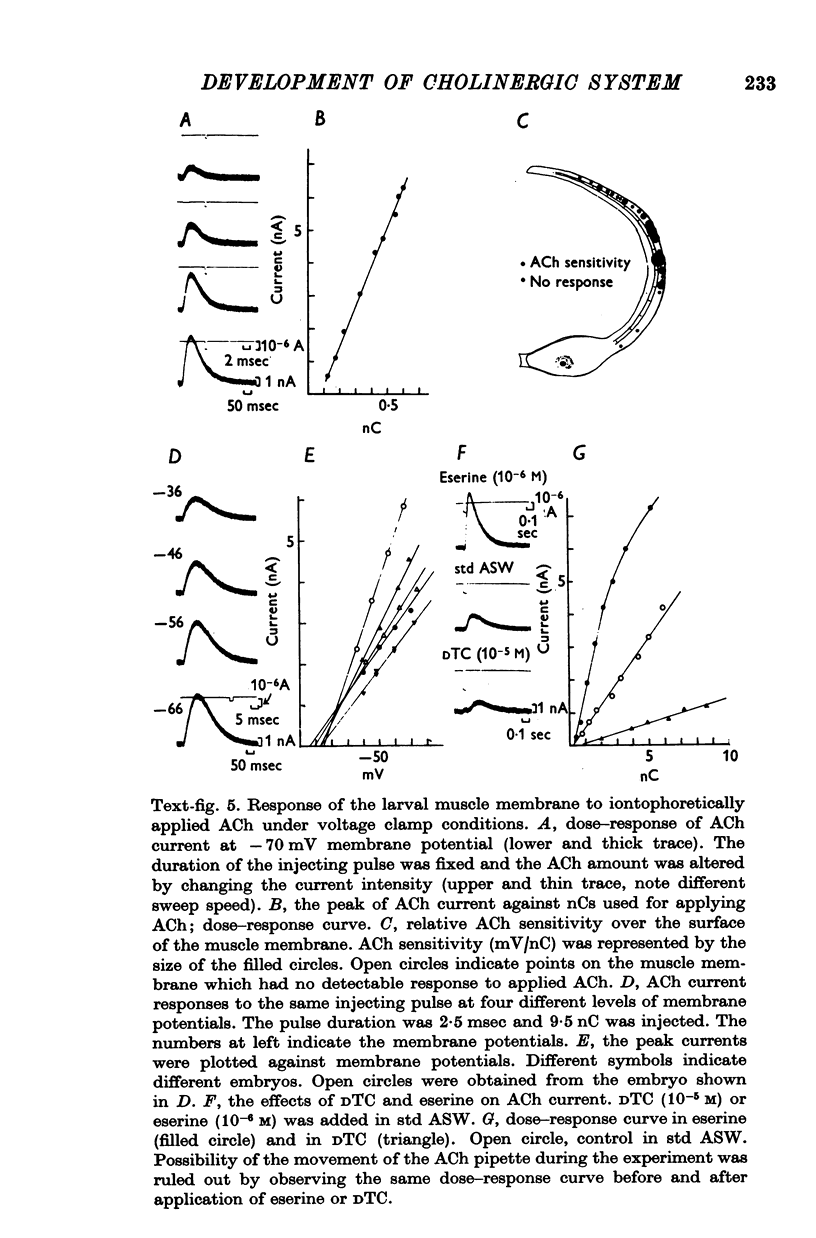
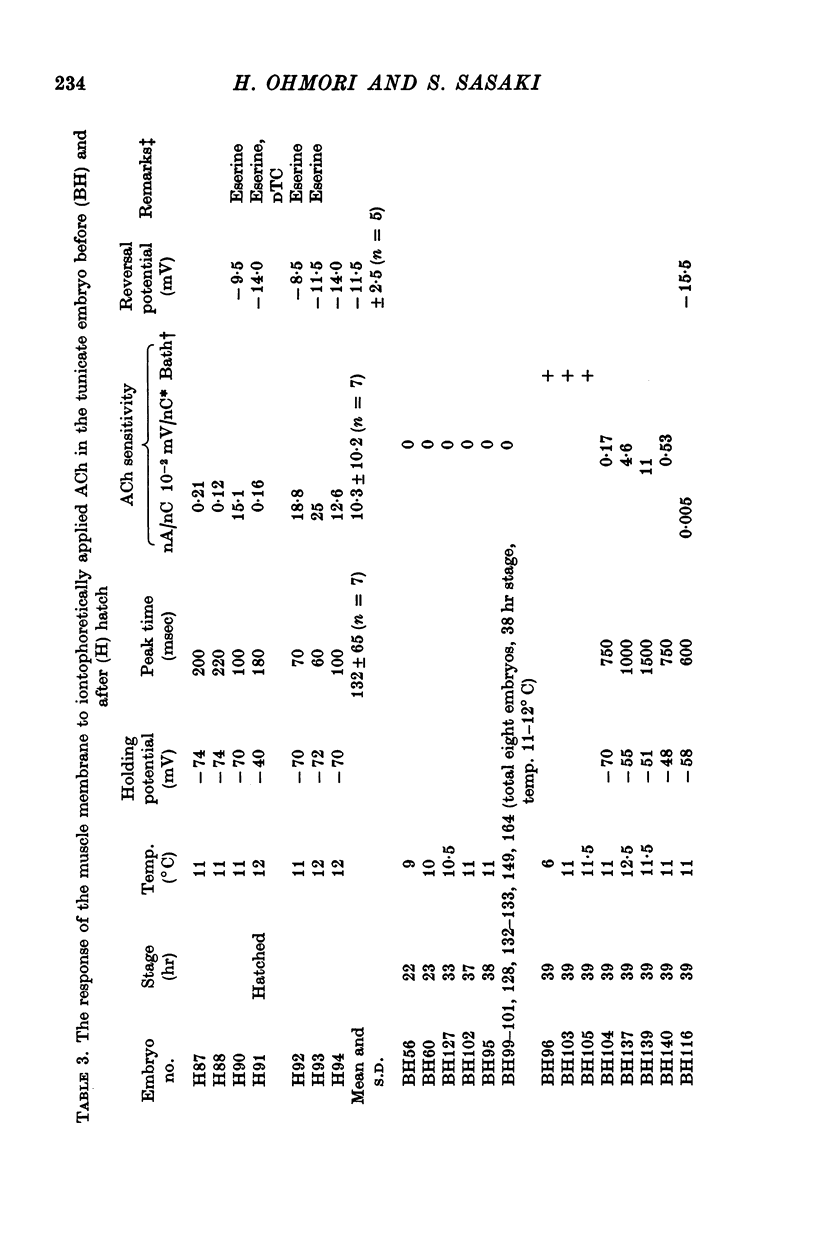
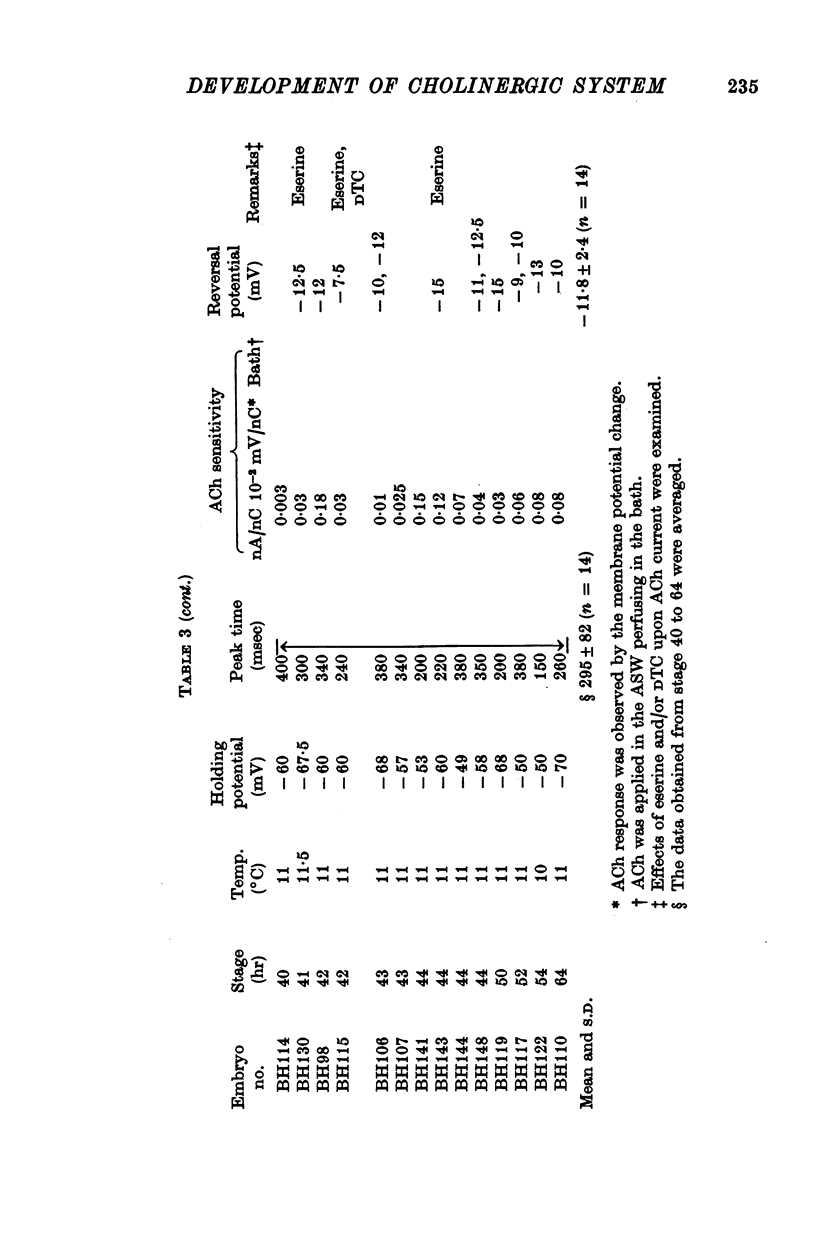
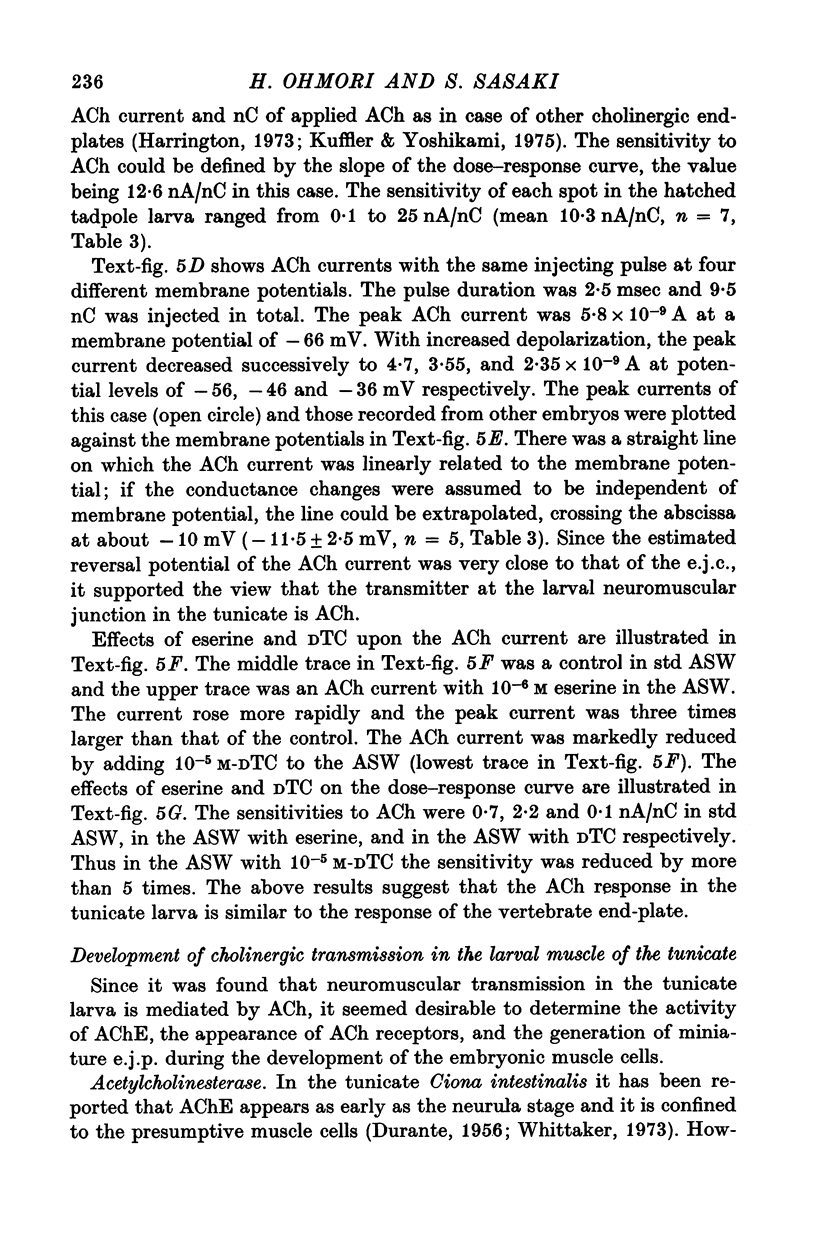
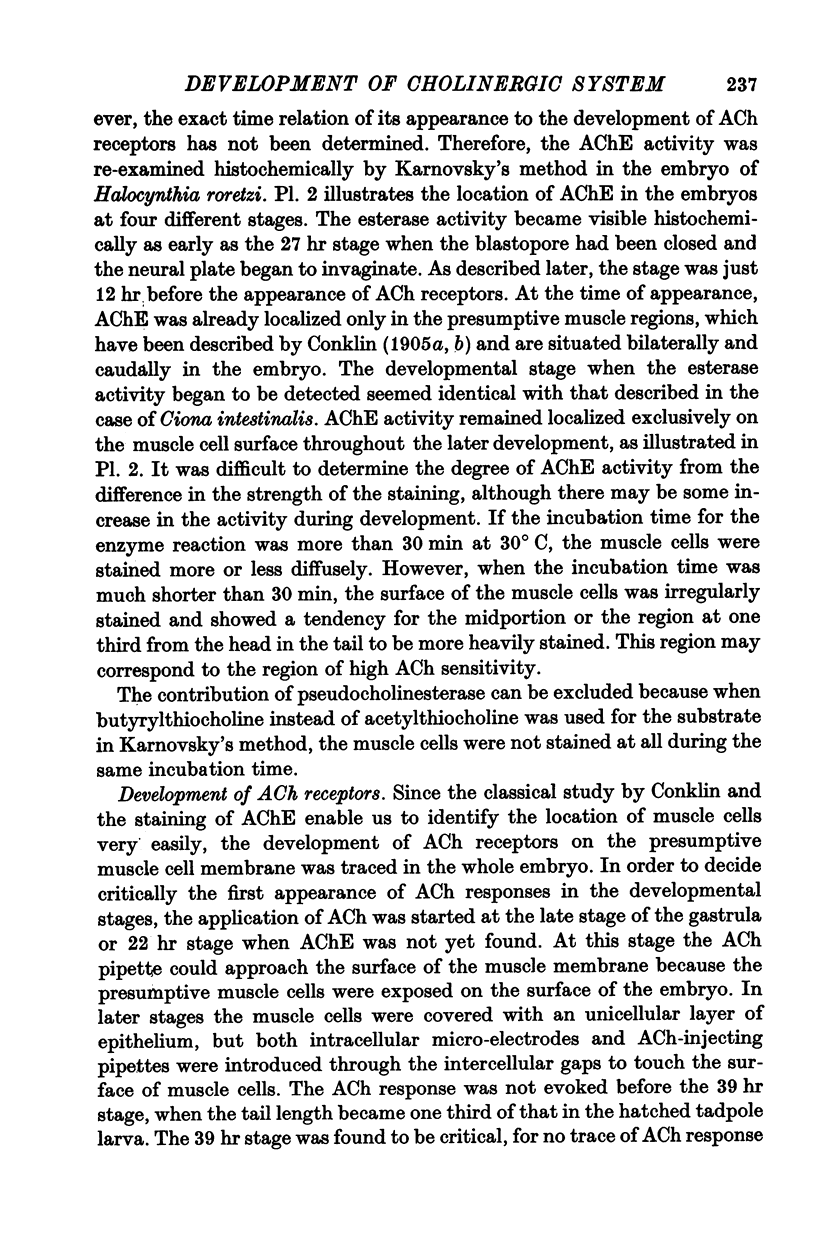
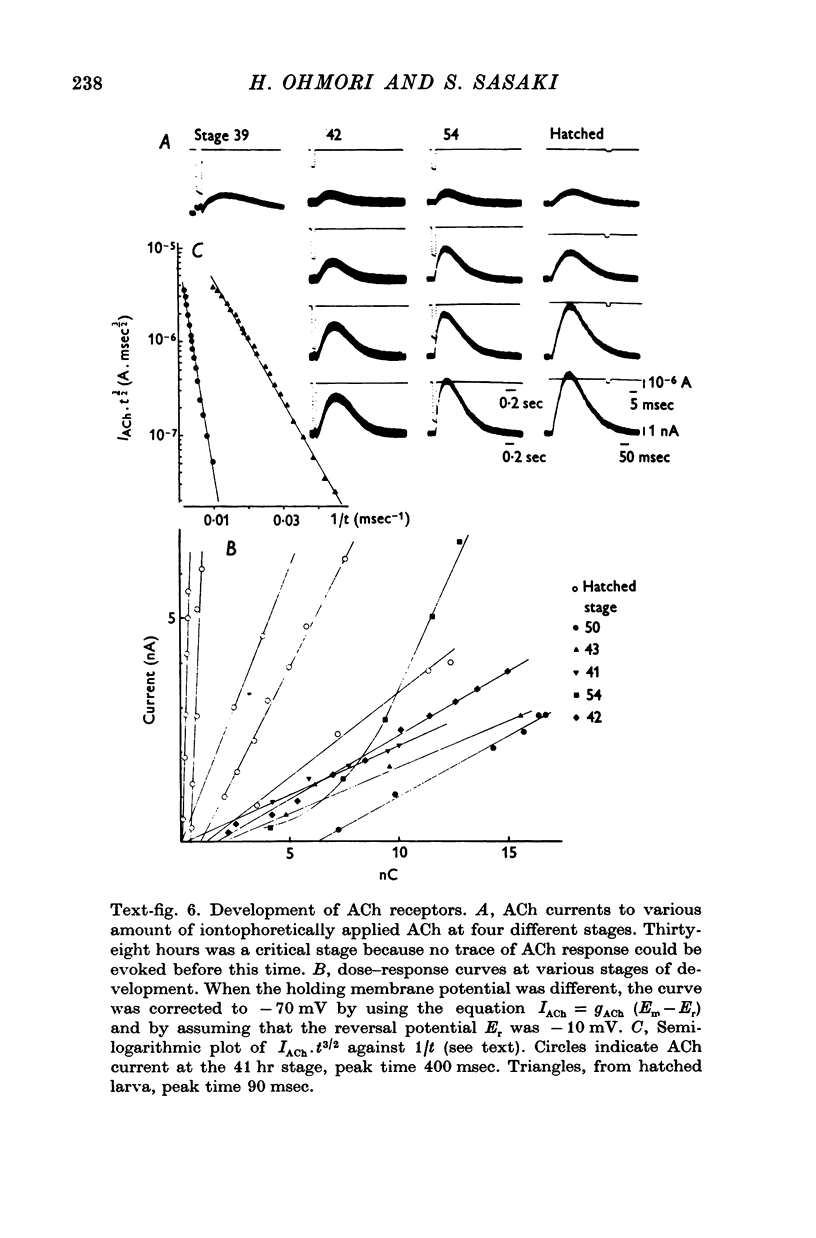
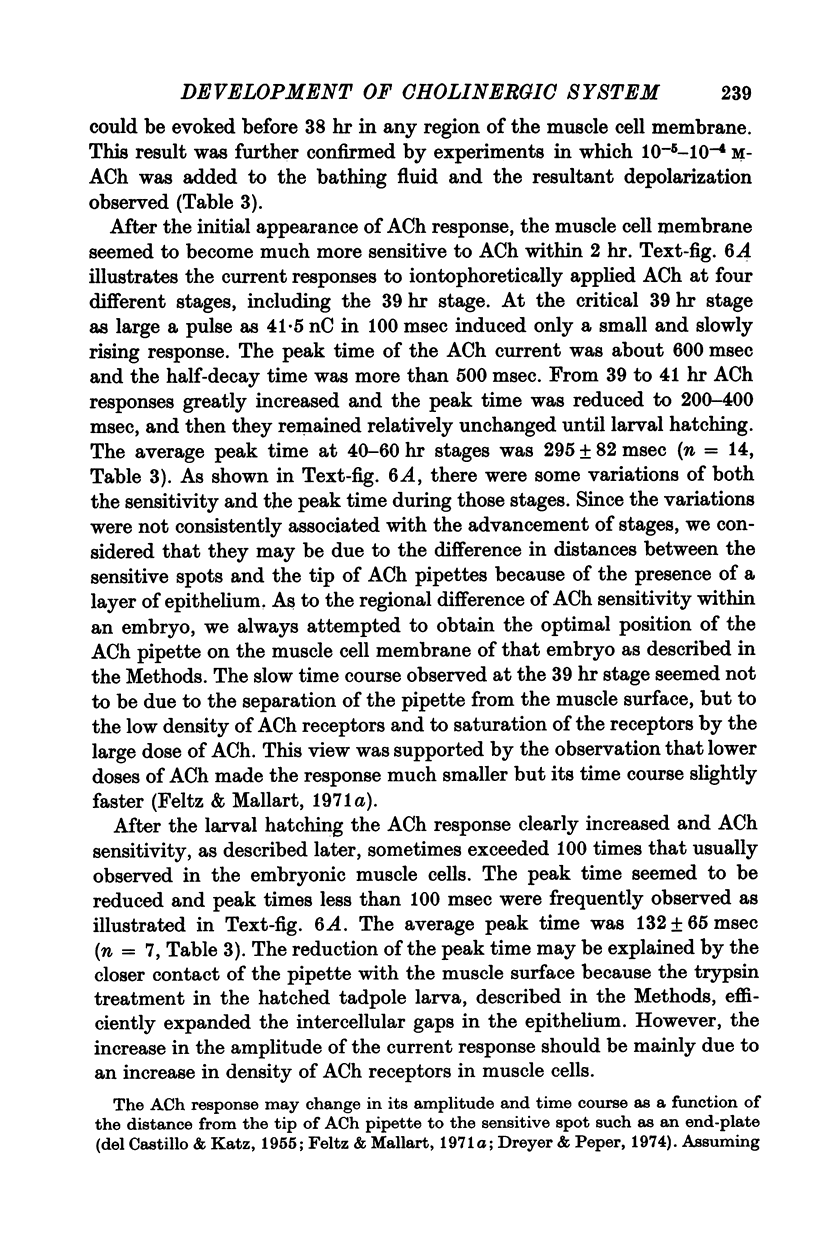
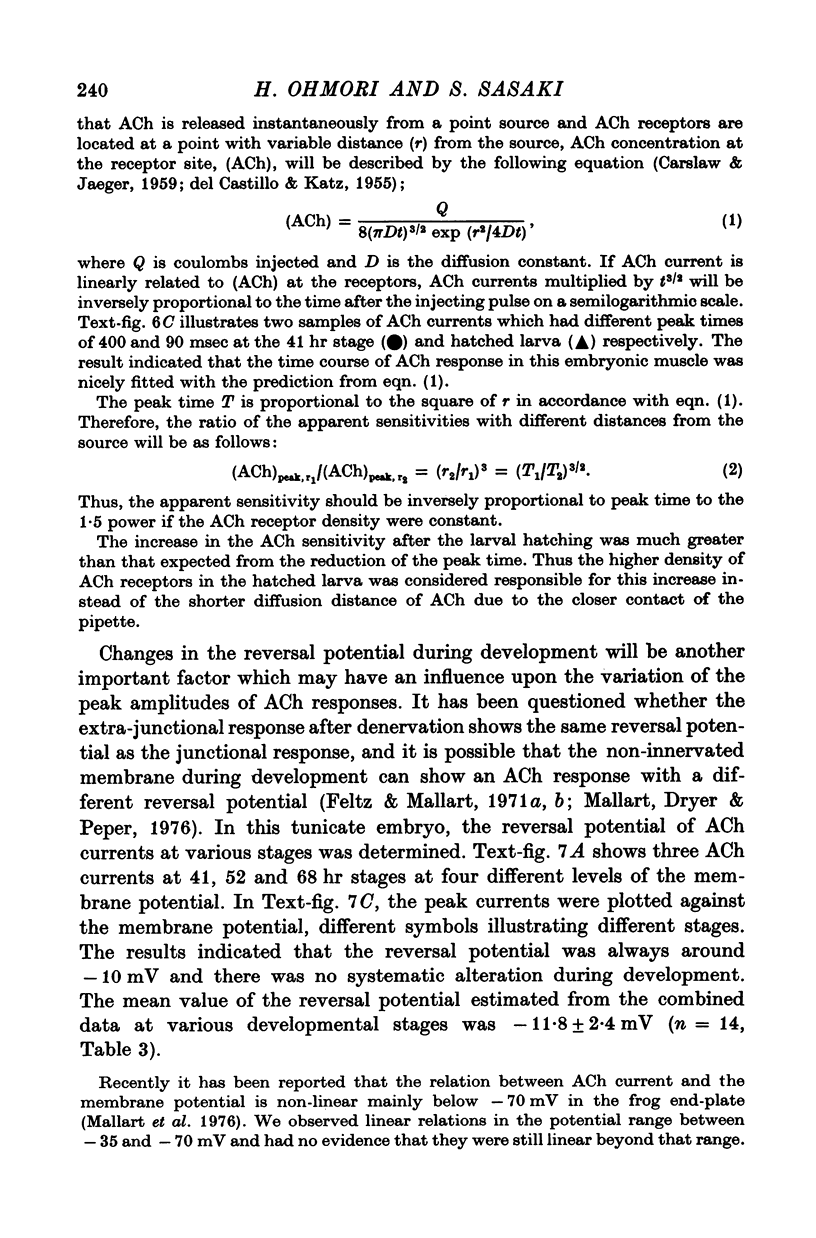
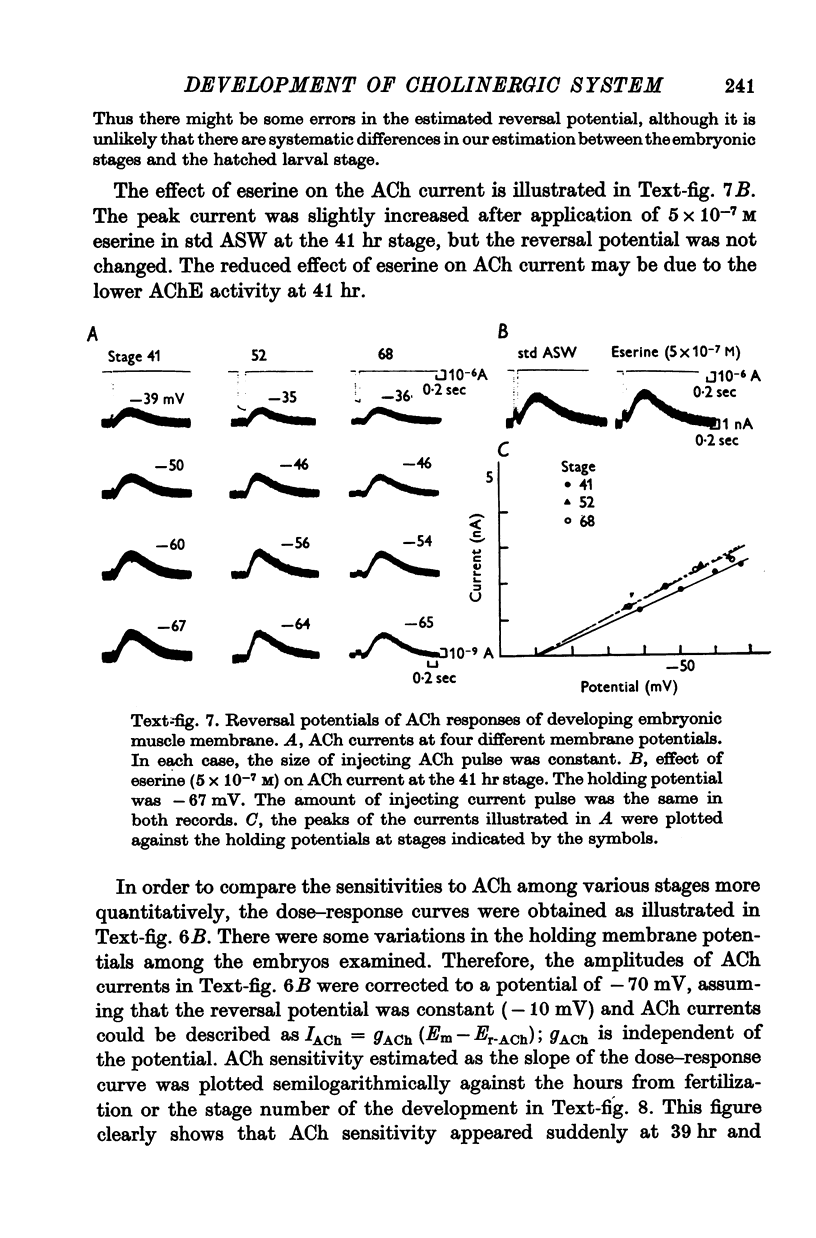
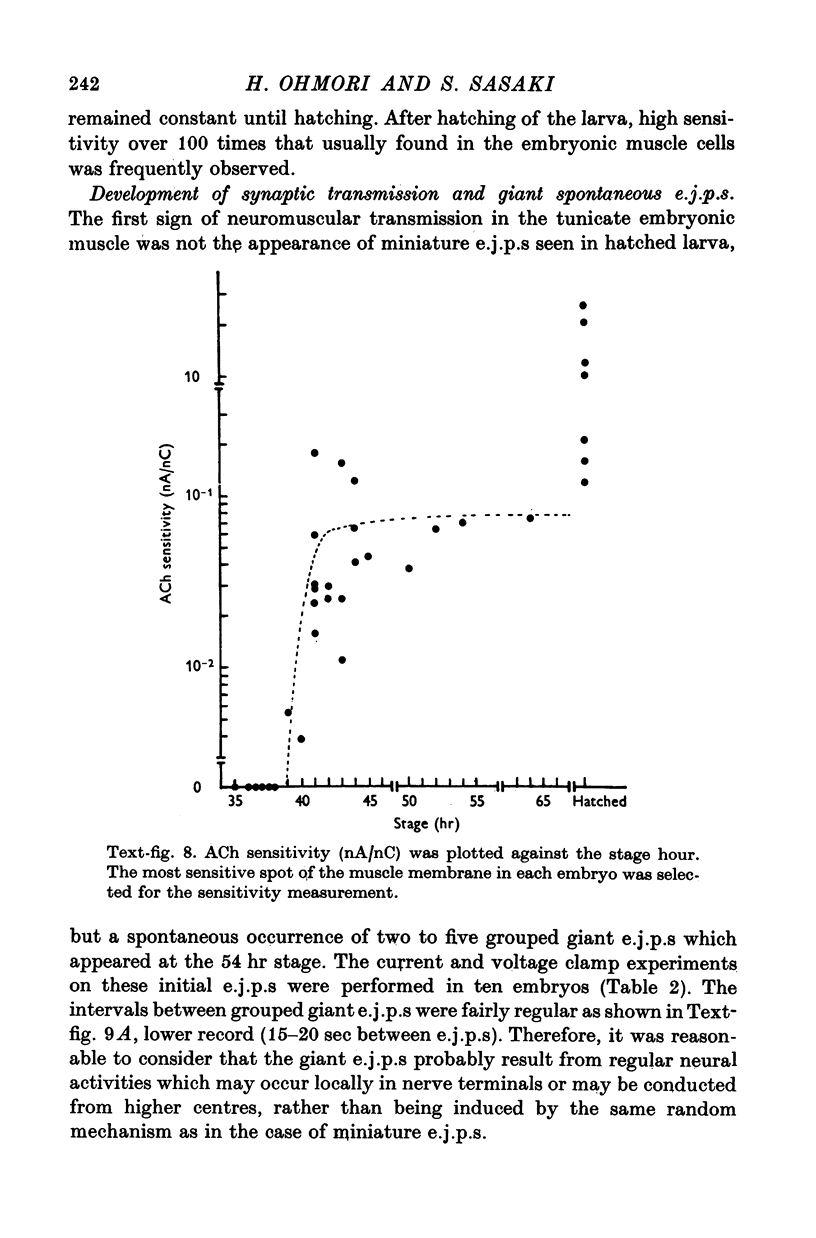
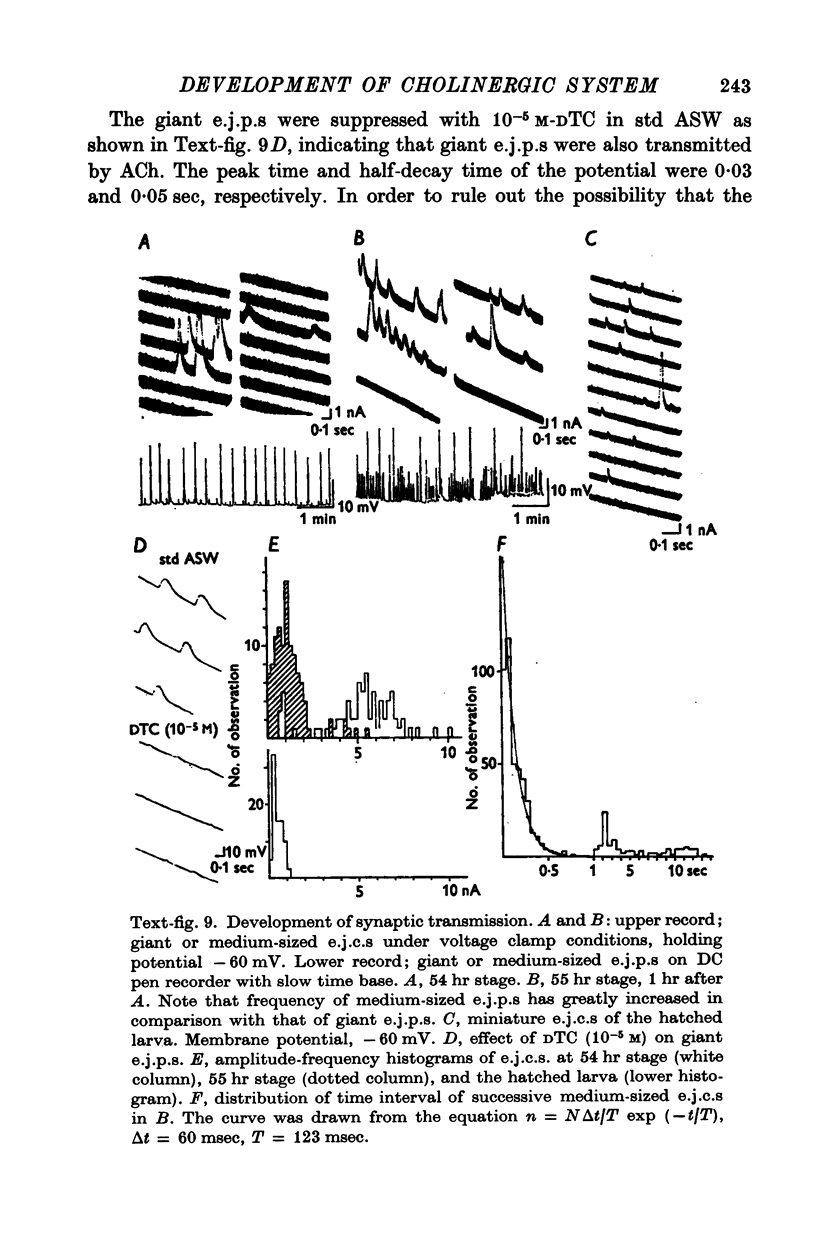
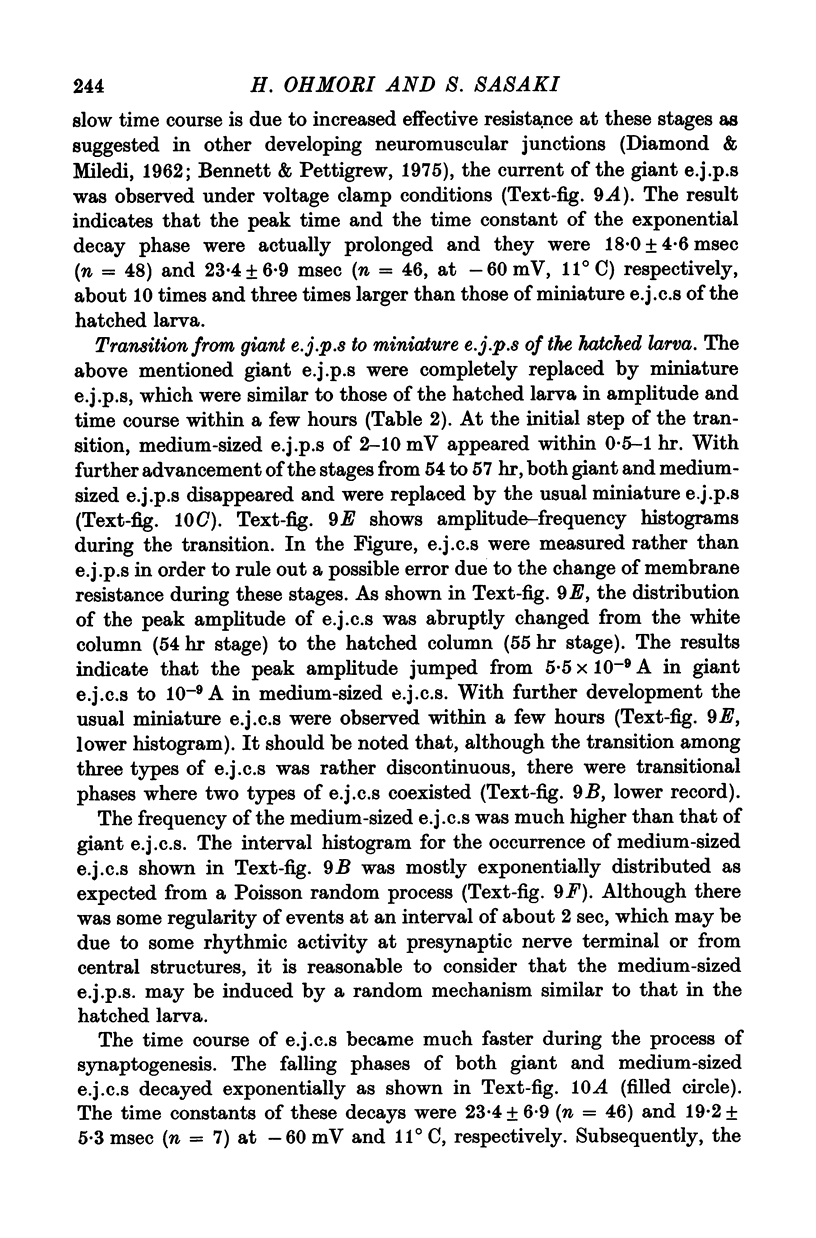
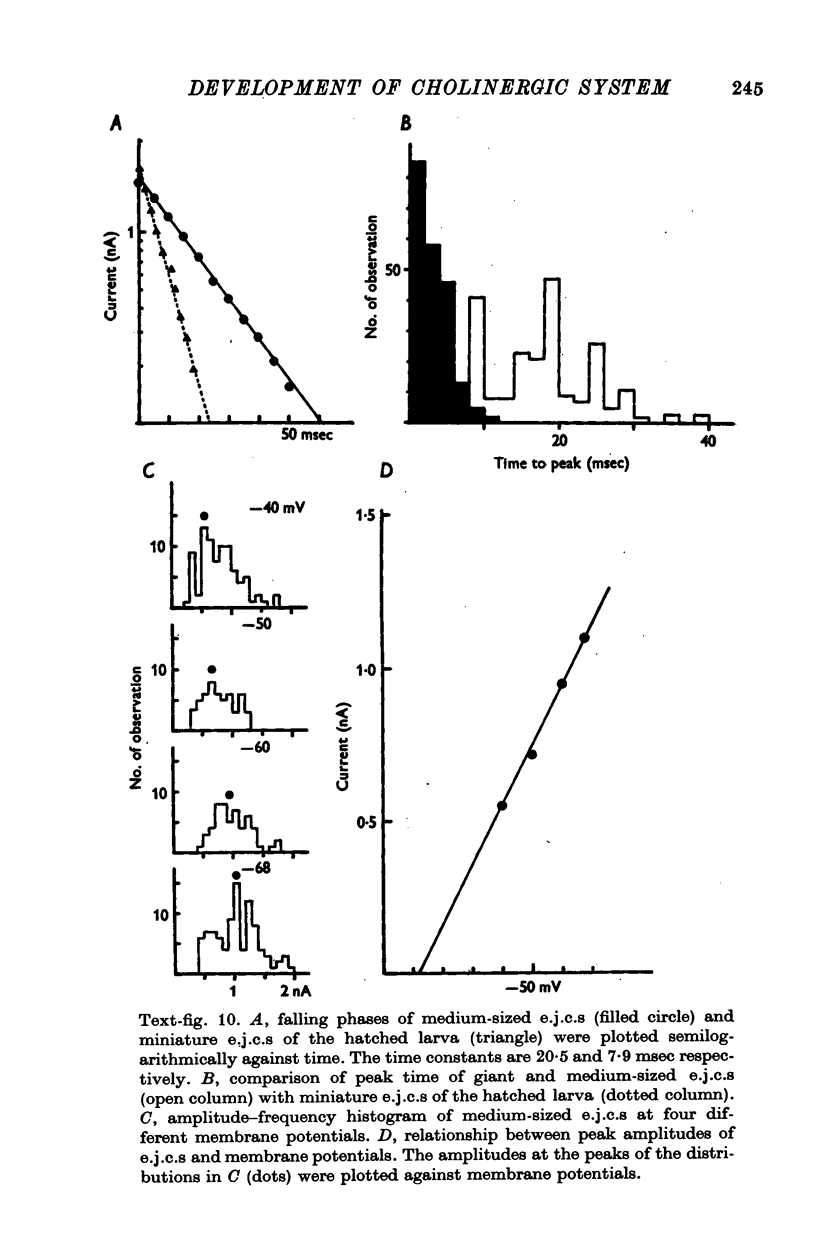
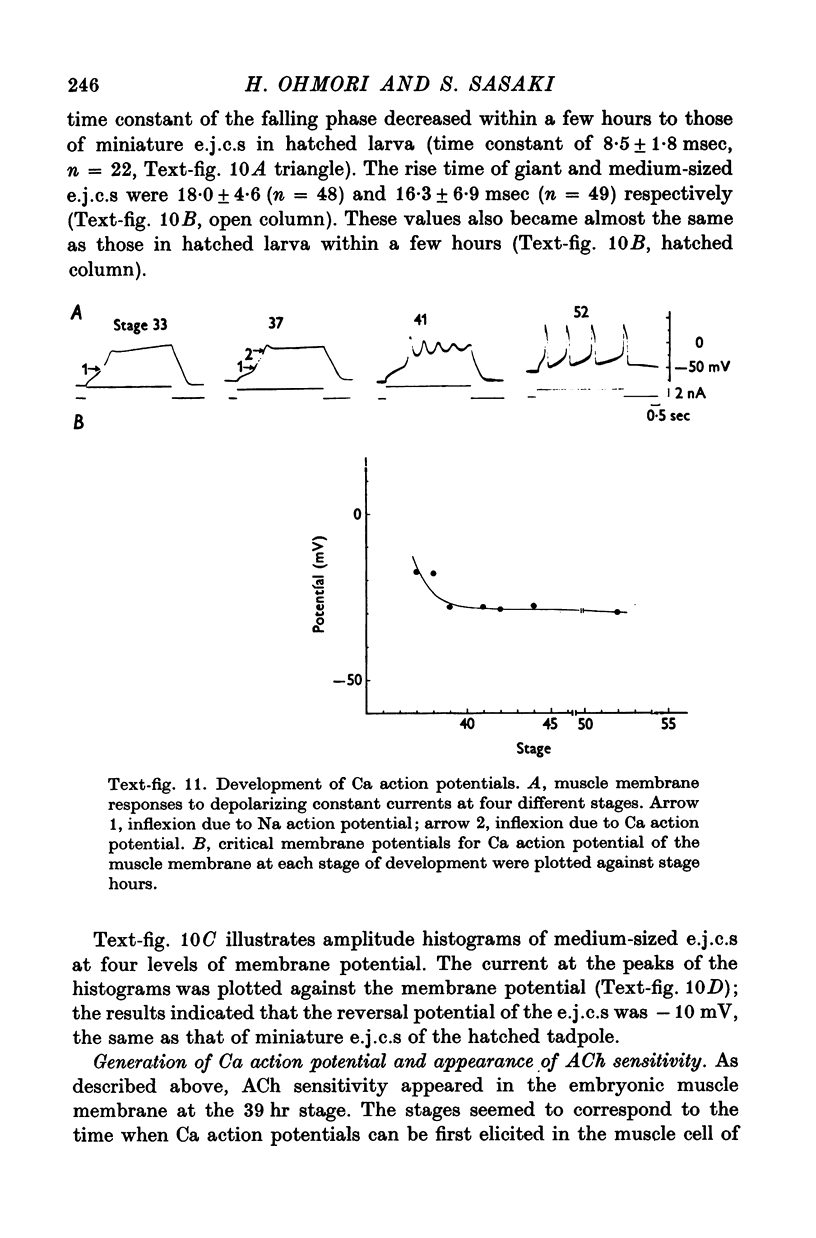
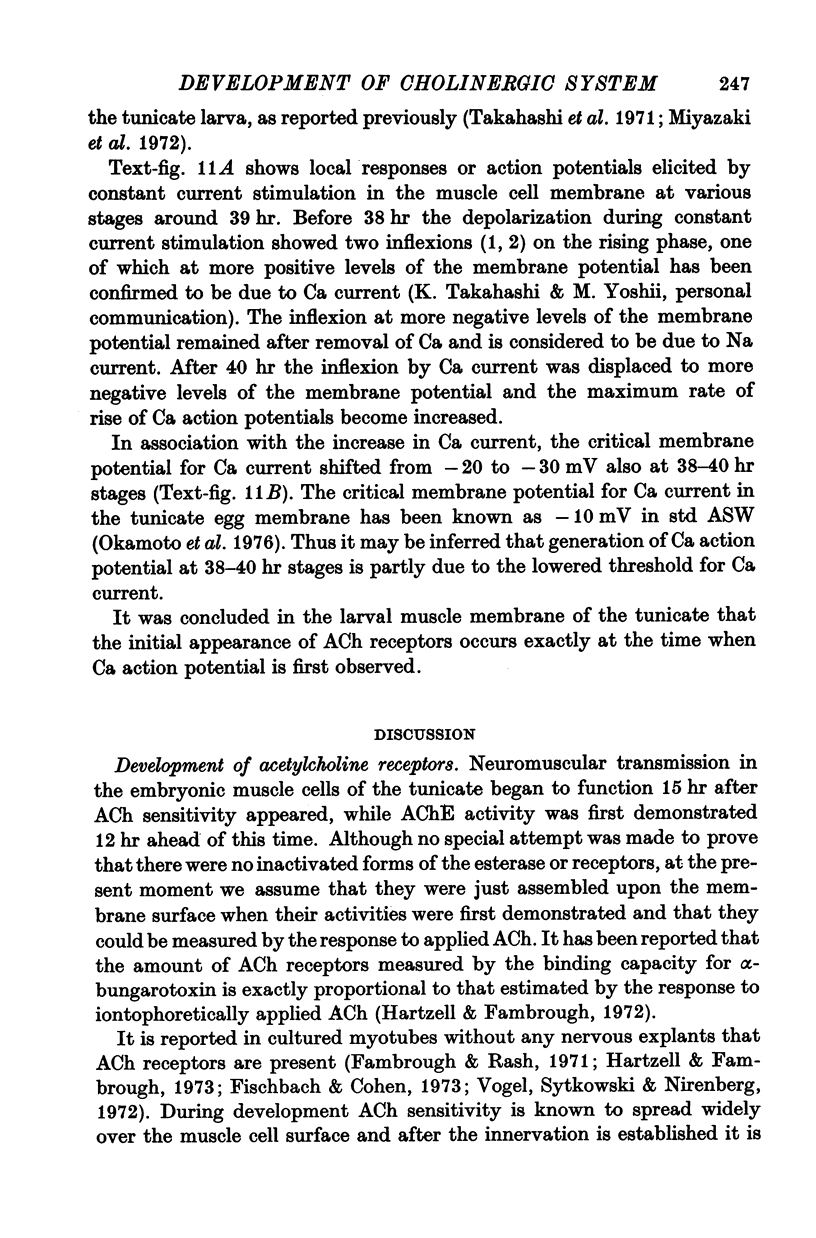
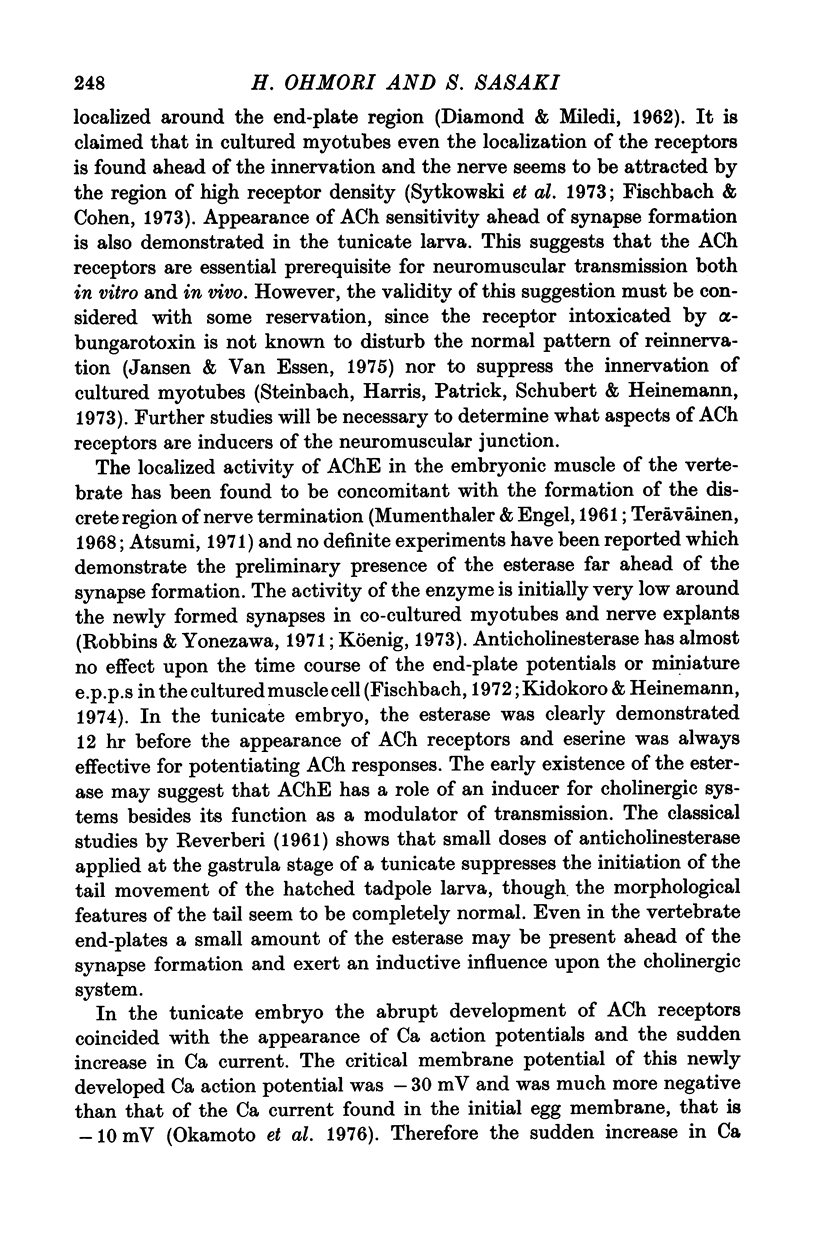
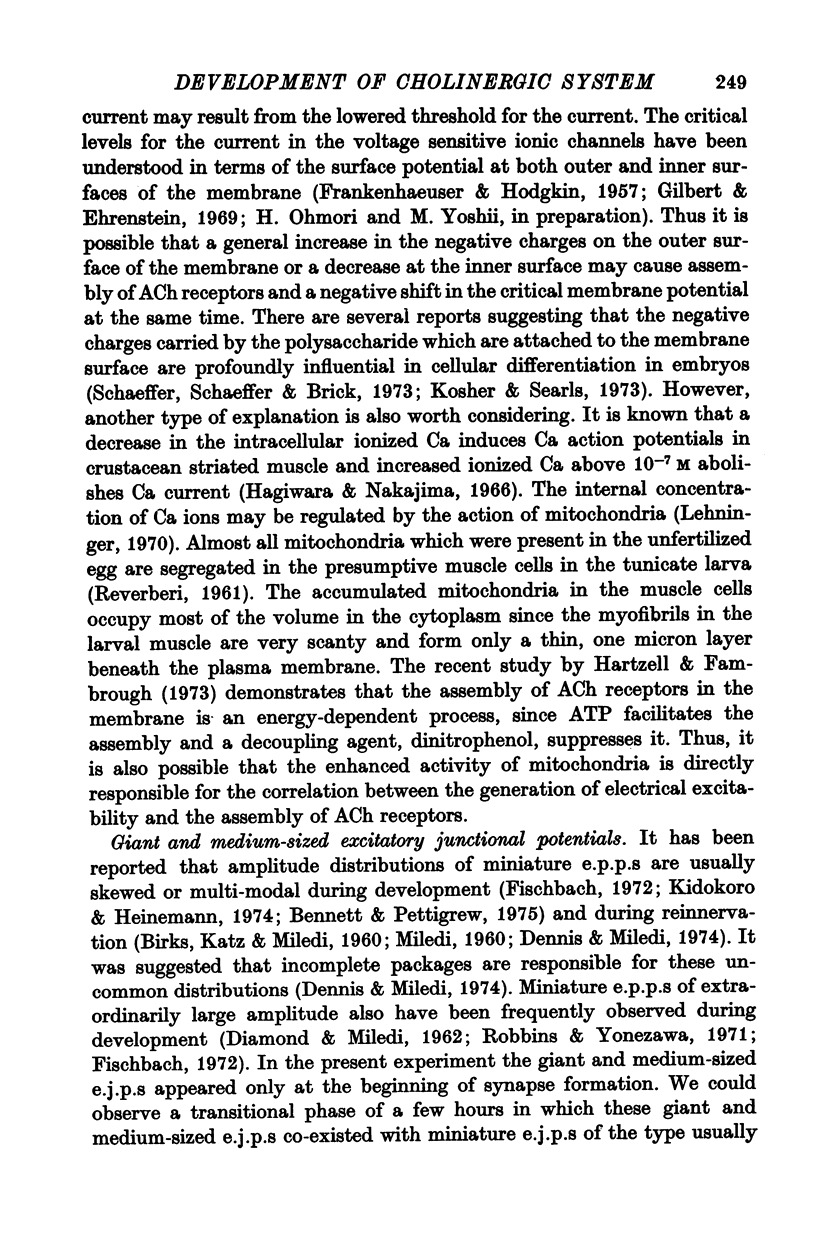
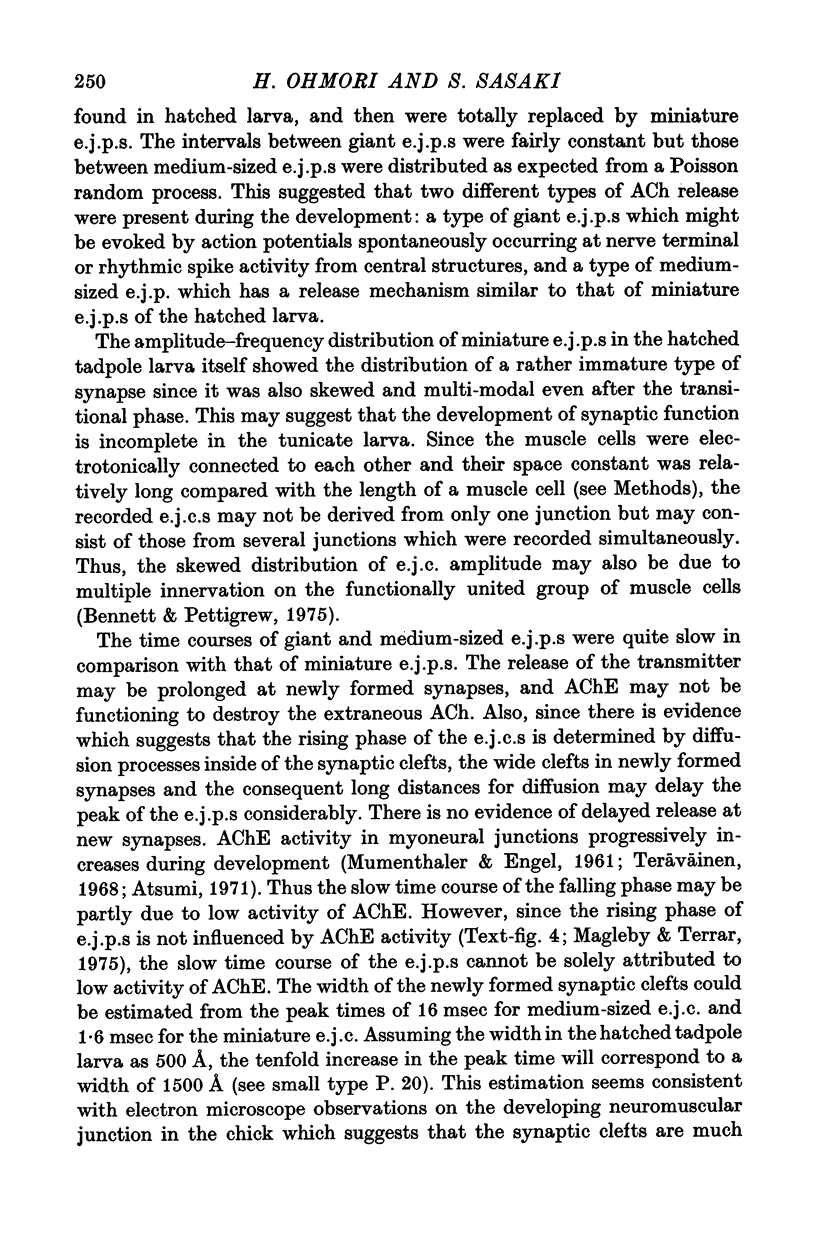
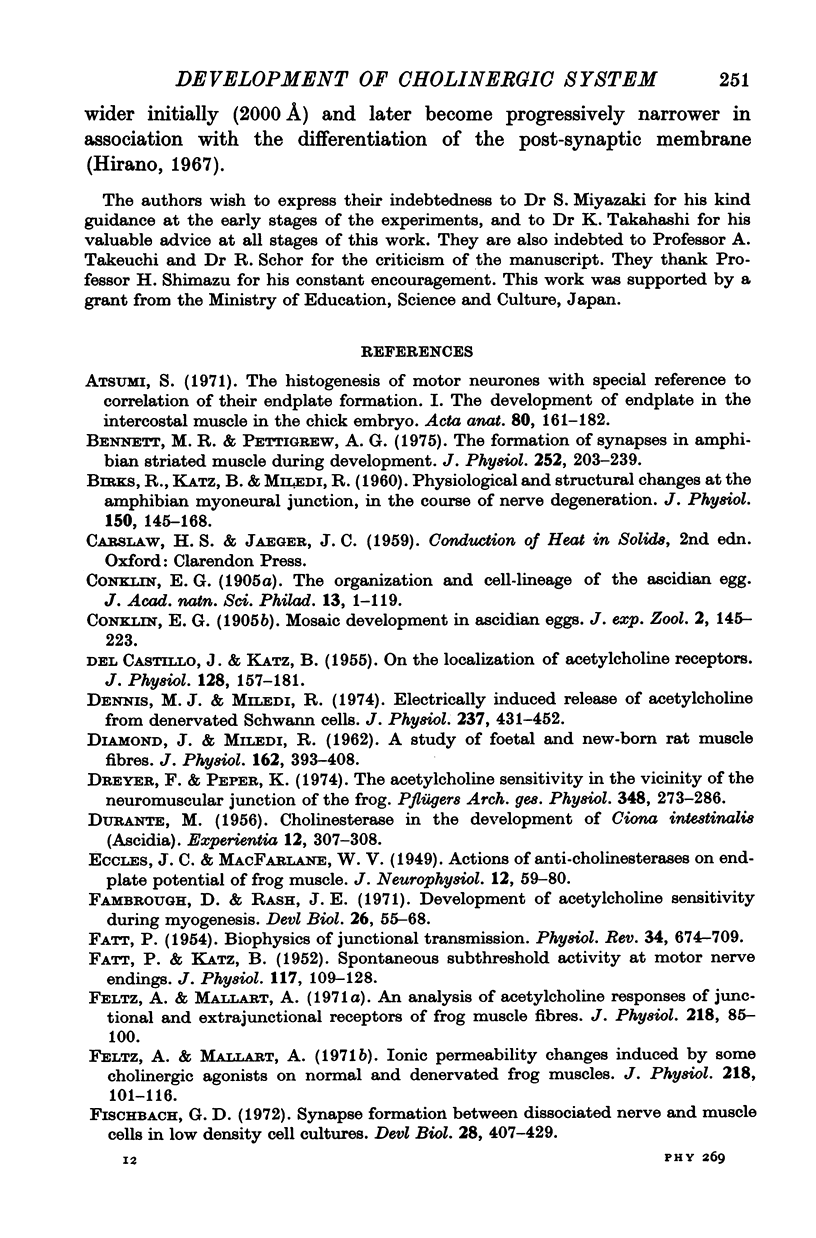
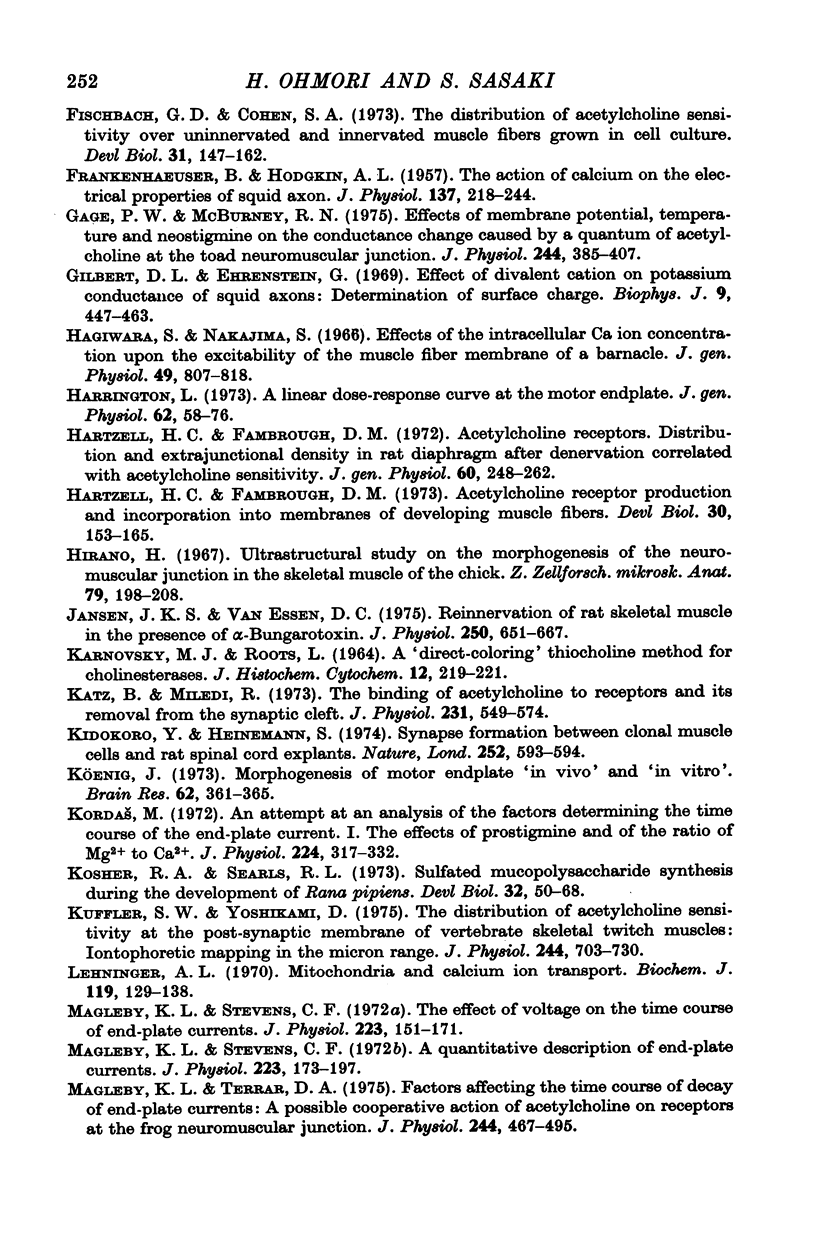
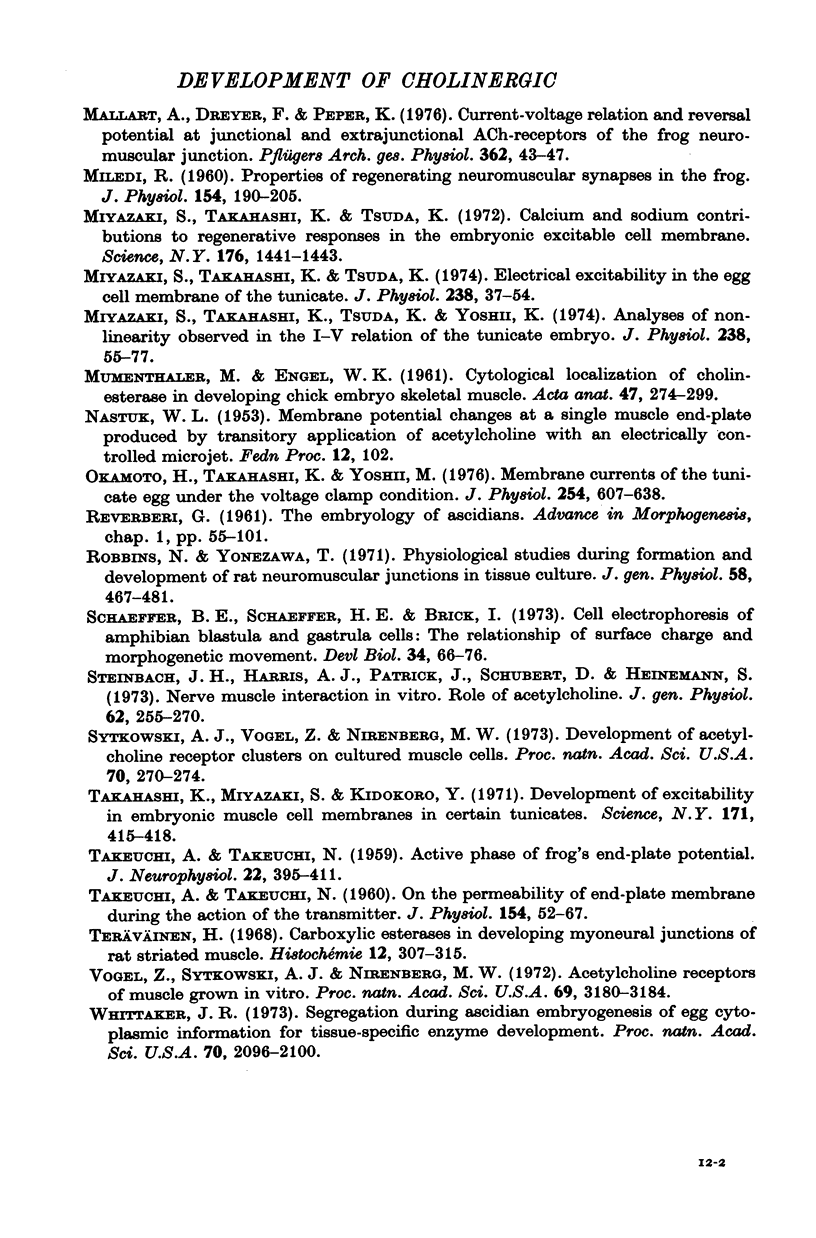
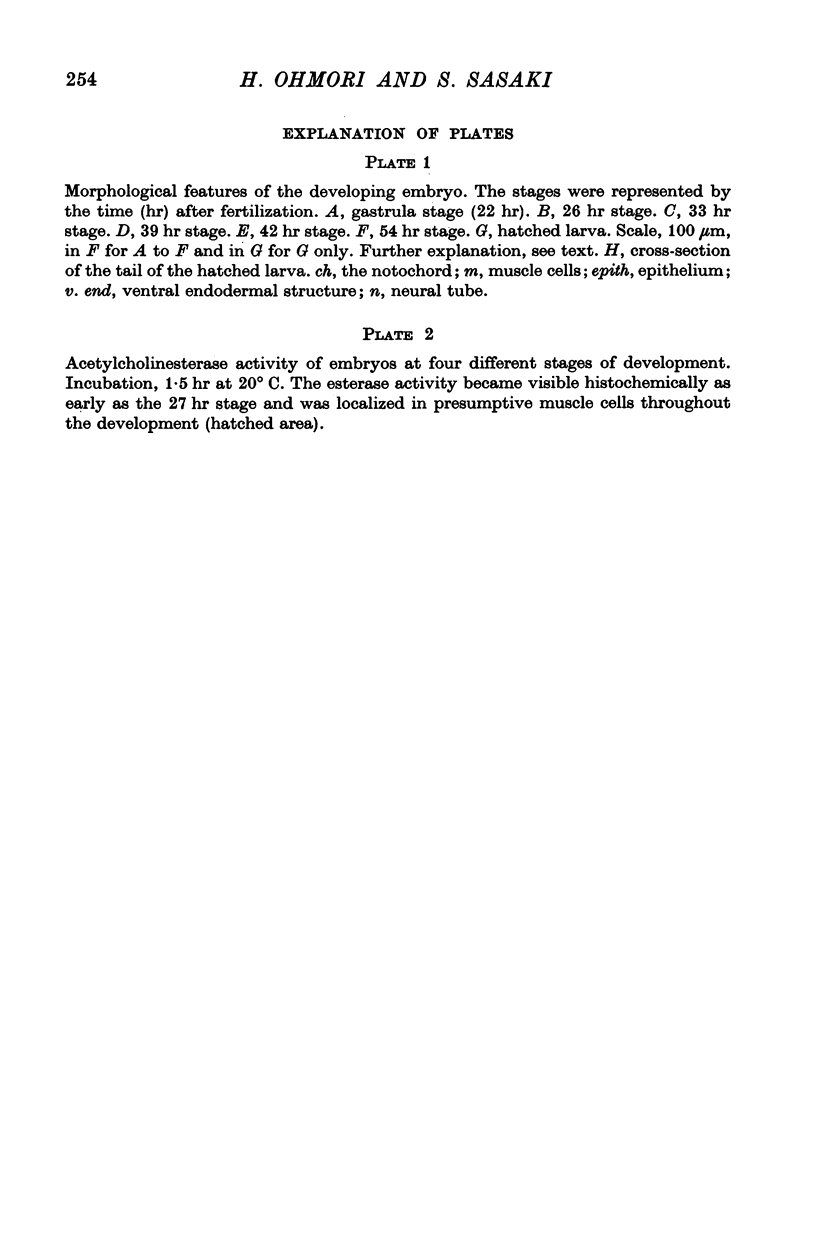
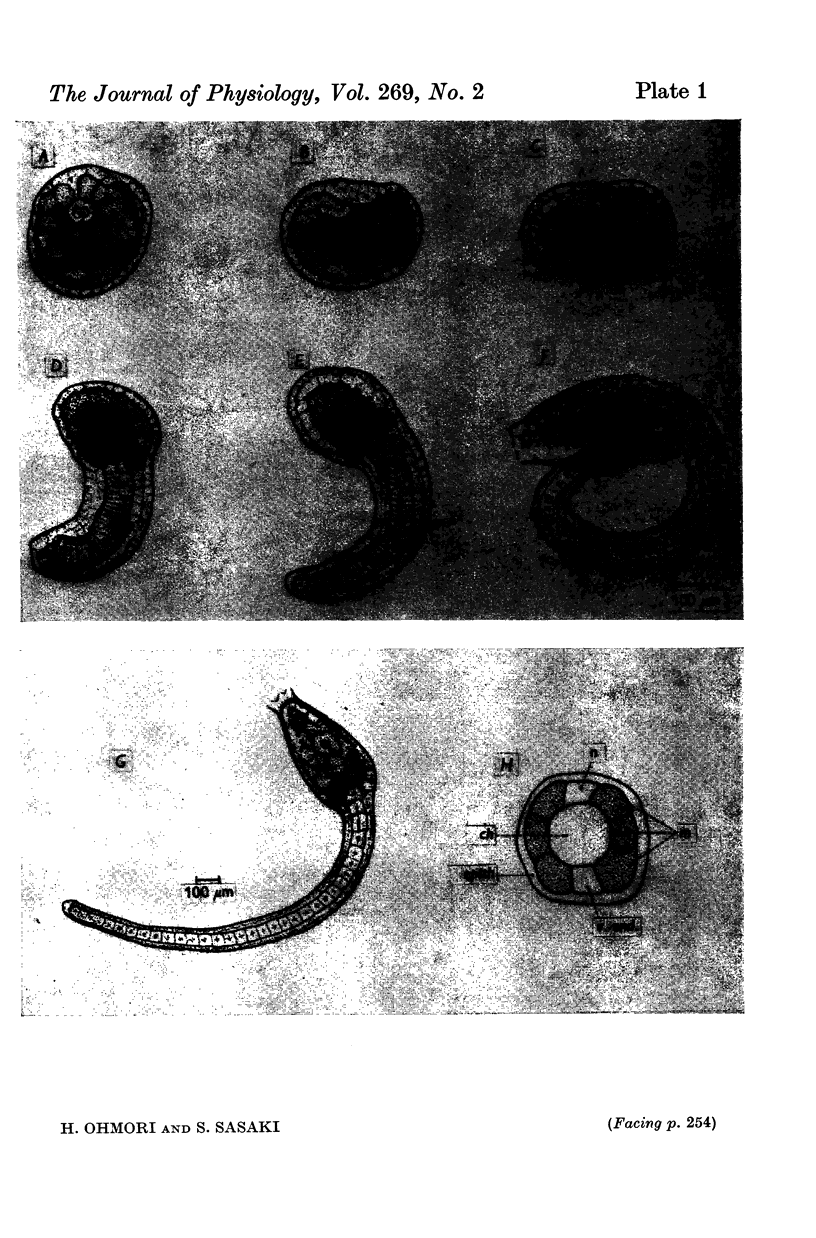
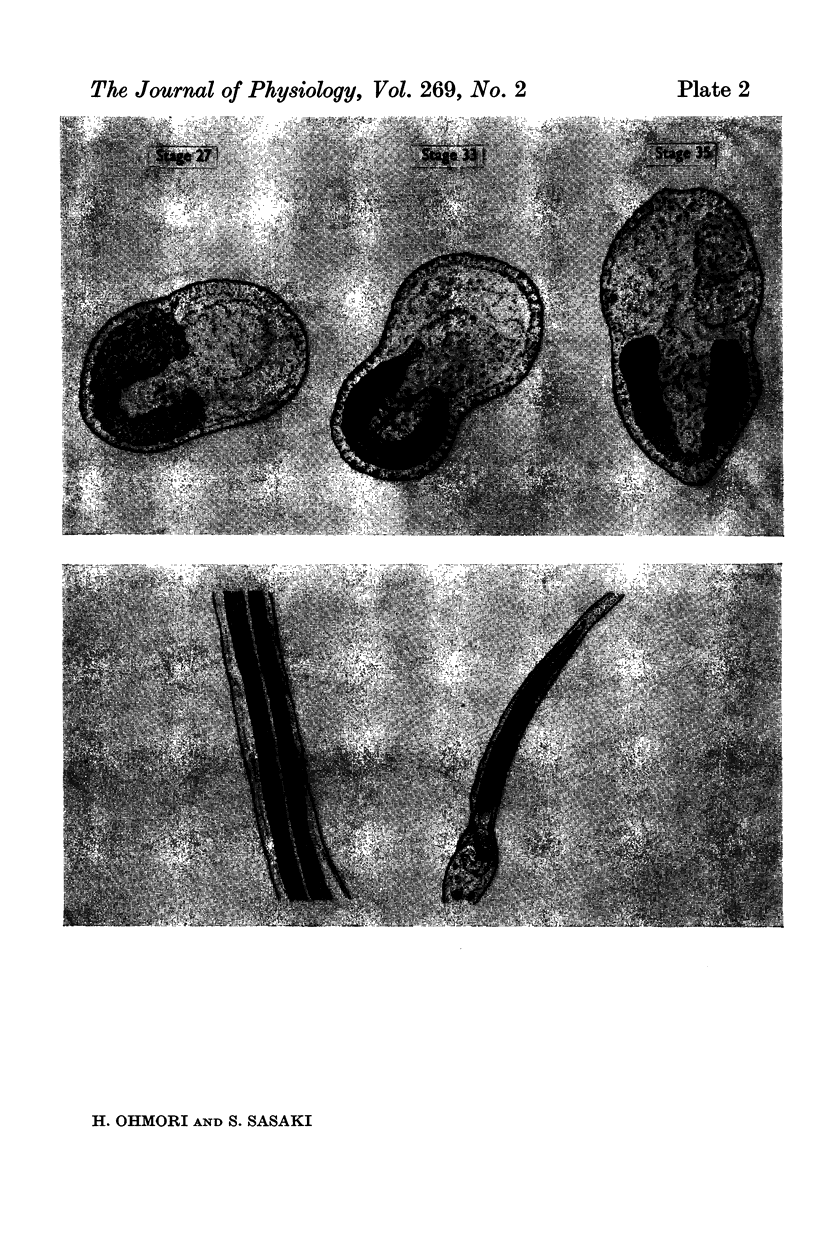
Images in this article
Selected References
These references are in PubMed. This may not be the complete list of references from this article.
- Atsumi S. The histogenesis of motor neurons with special reference to the correlation of their endplate formation. I. The development of endplates in the intercostal muscle in the chick embryo. Acta Anat (Basel) 1971;80(2):161–182. doi: 10.1159/000143687. [DOI] [PubMed] [Google Scholar]
- BIRKS R., KATZ B., MILEDI R. Physiological and structural changes at the amphibian myoneural junction, in the course of nerve degeneration. J Physiol. 1960 Jan;150:145–168. doi: 10.1113/jphysiol.1960.sp006379. [DOI] [PMC free article] [PubMed] [Google Scholar]
- Bennett M. R., Pettigrew A. G. The formation of synapses in amphibian striated muscle during development. J Physiol. 1975 Oct;252(1):203–239. doi: 10.1113/jphysiol.1975.sp011141. [DOI] [PMC free article] [PubMed] [Google Scholar]
- DEL CASTILLO J., KATZ B. On the localization of acetylcholine receptors. J Physiol. 1955 Apr 28;128(1):157–181. doi: 10.1113/jphysiol.1955.sp005297. [DOI] [PMC free article] [PubMed] [Google Scholar]
- DIAMOND J., MILEDI R. A study of foetal and new-born rat muscle fibres. J Physiol. 1962 Aug;162:393–408. doi: 10.1113/jphysiol.1962.sp006941. [DOI] [PMC free article] [PubMed] [Google Scholar]
- DURANTE M. Cholinesterase in the development of Ciona intestinalis (Ascidia). Experientia. 1956 Aug 15;12(8):307–308. doi: 10.1007/BF02159626. [DOI] [PubMed] [Google Scholar]
- Dennis M. J., Miledi R. Electrically induced release of acetylcholine from denervated Schwann cells. J Physiol. 1974 Mar;237(2):431–452. doi: 10.1113/jphysiol.1974.sp010490. [DOI] [PMC free article] [PubMed] [Google Scholar]
- Dreyer F., Peper K. The acetylcholine sensitivity in the vicinity of the neuromuscular junction of the frog. Pflugers Arch. 1974 May 6;348(4):273–286. doi: 10.1007/BF00589217. [DOI] [PubMed] [Google Scholar]
- ECCLES J. C., MacFARLANE W. V. Actions of anti-cholinesterases on endplate potential of frog muscle. J Neurophysiol. 1949 Jan;12(1):59–80. doi: 10.1152/jn.1949.12.1.59. [DOI] [PubMed] [Google Scholar]
- FATT P. Biophysics of junctional transmission. Physiol Rev. 1954 Oct;34(4):674–710. doi: 10.1152/physrev.1954.34.4.674. [DOI] [PubMed] [Google Scholar]
- FATT P., KATZ B. Spontaneous subthreshold activity at motor nerve endings. J Physiol. 1952 May;117(1):109–128. [PMC free article] [PubMed] [Google Scholar]
- FRANKENHAEUSER B., HODGKIN A. L. The action of calcium on the electrical properties of squid axons. J Physiol. 1957 Jul 11;137(2):218–244. doi: 10.1113/jphysiol.1957.sp005808. [DOI] [PMC free article] [PubMed] [Google Scholar]
- Fambrough D., Rash J. E. Development of acetylcholine sensitivity during myogenesis. Dev Biol. 1971 Sep;26(1):55–68. doi: 10.1016/0012-1606(71)90107-2. [DOI] [PubMed] [Google Scholar]
- Feltz A., Mallart A. An analysis of acetylcholine responses of junctional and extrajunctional receptors of frog muscle fibres. J Physiol. 1971 Oct;218(1):85–100. doi: 10.1113/jphysiol.1971.sp009605. [DOI] [PMC free article] [PubMed] [Google Scholar]
- Feltz A., Mallart A. Ionic permeability changes induced by some cholinergic agonists on normal and denervated frog muscles. J Physiol. 1971 Oct;218(1):101–116. doi: 10.1113/jphysiol.1971.sp009606. [DOI] [PMC free article] [PubMed] [Google Scholar]
- Fischbach G. D., Cohen S. A. The distribution of acetylcholine sensitivity over uninnervated and innervated muscle fibers grown in cell culture. Dev Biol. 1973 Mar;31(1):147–162. doi: 10.1016/0012-1606(73)90326-6. [DOI] [PubMed] [Google Scholar]
- Fischbach G. D. Synapse formation between dissociated nerve and muscle cells in low density cell cultures. Dev Biol. 1972 Jun;28(2):407–429. doi: 10.1016/0012-1606(72)90023-1. [DOI] [PubMed] [Google Scholar]
- Gage P. W., McBurney R. N. Effects of membrane potential, temperature and neostigmine on the conductance change caused by a quantum or acetylcholine at the toad neuromuscular junction. J Physiol. 1975 Jan;244(2):385–407. doi: 10.1113/jphysiol.1975.sp010805. [DOI] [PMC free article] [PubMed] [Google Scholar]
- Gilbert D. L., Ehrenstein G. Effect of divalent cations on potassium conductance of squid axons: determination of surface charge. Biophys J. 1969 Mar;9(3):447–463. doi: 10.1016/S0006-3495(69)86396-4. [DOI] [PMC free article] [PubMed] [Google Scholar]
- Hagiwara S., Nakajima S. Effects of the intracellular Ca ion concentration upon the excitability of the muscle fiber membrane of a barnacle. J Gen Physiol. 1966 Mar;49(4):807–818. doi: 10.1085/jgp.49.4.807. [DOI] [PMC free article] [PubMed] [Google Scholar]
- Hartzell H. C., Fambrough D. M. Acetycholine receptor production and incorporation into membranes of developing muscle fibers. Dev Biol. 1973 Jan;30(1):153–165. doi: 10.1016/0012-1606(73)90054-7. [DOI] [PubMed] [Google Scholar]
- Hartzell H. C., Fambrough D. M. Acetylcholine receptors. Distribution and extrajunctional density in rat diaphragm after denervation correlated with acetylcholine sensitivity. J Gen Physiol. 1972 Sep;60(3):248–262. doi: 10.1085/jgp.60.3.248. [DOI] [PMC free article] [PubMed] [Google Scholar]
- Hirano H. Ultrastructural study on the morphogenesis of the neuromuscular junction in the skeletal muscle of the chick. Z Zellforsch Mikrosk Anat. 1967;79(2):198–208. [PubMed] [Google Scholar]
- Jansen J. K., Van Essen D. C. Re-innervation of rat skeleton muscle in the presence of alpha-bungarotoxin. J Physiol. 1975 Sep;250(3):651–667. doi: 10.1113/jphysiol.1975.sp011075. [DOI] [PMC free article] [PubMed] [Google Scholar]
- KARNOVSKY M. J., ROOTS L. A "DIRECT-COLORING" THIOCHOLINE METHOD FOR CHOLINESTERASES. J Histochem Cytochem. 1964 Mar;12:219–221. doi: 10.1177/12.3.219. [DOI] [PubMed] [Google Scholar]
- Katz B., Miledi R. The binding of acetylcholine to receptors and its removal from the synaptic cleft. J Physiol. 1973 Jun;231(3):549–574. doi: 10.1113/jphysiol.1973.sp010248. [DOI] [PMC free article] [PubMed] [Google Scholar]
- Kidokoro Y., Heinemann S. Synapse formation between clonal muscle cells and rat spinal cord explants. Nature. 1974 Dec 13;252(5484):593–594. doi: 10.1038/252593a0. [DOI] [PubMed] [Google Scholar]
- Koenig J. Morphogenesis of motor end-plates "in vivo" and "in vitro". Brain Res. 1973 Nov 23;62(2):361–365. doi: 10.1016/0006-8993(73)90697-5. [DOI] [PubMed] [Google Scholar]
- Kosher R. A., Searls R. L. Sulfated mucopolysaccharide synthesis during the development of Rana pipiens. Dev Biol. 1973 May;32(1):50–68. doi: 10.1016/0012-1606(73)90219-4. [DOI] [PubMed] [Google Scholar]
- Kuffler S. W., Yoshikami D. The distribution of acetylcholine sensitivity at the post-synaptic membrane of vertebrate skeletal twitch muscles: iontophoretic mapping in the micron range. J Physiol. 1975 Jan;244(3):703–730. doi: 10.1113/jphysiol.1975.sp010821. [DOI] [PMC free article] [PubMed] [Google Scholar]
- Lehninger A. L. Mitochondria and calcium ion transport. Biochem J. 1970 Sep;119(2):129–138. doi: 10.1042/bj1190129. [DOI] [PMC free article] [PubMed] [Google Scholar]
- MILEDI R. Properties of regenerating neuromuscular synapses in the frog. J Physiol. 1960 Nov;154:190–205. doi: 10.1113/jphysiol.1960.sp006573. [DOI] [PMC free article] [PubMed] [Google Scholar]
- MUMENTHALER M., ENGEL W. K. Cytological localization of cholinesterase in developing chick embryo skeletal muscle. Acta Anat (Basel) 1961;47:274–299. doi: 10.1159/000141814. [DOI] [PubMed] [Google Scholar]
- Magleby K. L., Stevens C. F. A quantitative description of end-plate currents. J Physiol. 1972 May;223(1):173–197. doi: 10.1113/jphysiol.1972.sp009840. [DOI] [PMC free article] [PubMed] [Google Scholar]
- Magleby K. L., Stevens C. F. The effect of voltage on the time course of end-plate currents. J Physiol. 1972 May;223(1):151–171. doi: 10.1113/jphysiol.1972.sp009839. [DOI] [PMC free article] [PubMed] [Google Scholar]
- Magleby K. L., Terrar D. A. Factors affecting the time course of decay of end-plate currents: a possible cooperative action of acetylcholine on receptors at the frog neuromuscular junction. J Physiol. 1975 Jan;244(2):467–495. doi: 10.1113/jphysiol.1975.sp010808. [DOI] [PMC free article] [PubMed] [Google Scholar]
- Mallart A., Dreyer F., Peper K. Current-voltage relation and reversal potential at junctional and extrajunctional ACh-receptors of the frog neuromuscular junction. Pflugers Arch. 1976 Mar 11;362(1):43–47. doi: 10.1007/BF00588679. [DOI] [PubMed] [Google Scholar]
- Miyazaki S. I., Takahashi K., Tsuda K. Electrical excitability in the egg cell membrane of the tunicate. J Physiol. 1974 Apr;238(1):37–54. doi: 10.1113/jphysiol.1974.sp010509. [DOI] [PMC free article] [PubMed] [Google Scholar]
- Miyazaki S. I., Takahashi K., Tsuda K., Yoshii M. Analysis of non-linearity observed in the current-voltage relation of the tunicate embryo. J Physiol. 1974 Apr;238(1):55–77. doi: 10.1113/jphysiol.1974.sp010510. [DOI] [PMC free article] [PubMed] [Google Scholar]
- Miyazaki S., Takahashi K., Tsuda K. Calcium and sodium contributions to regenerative responses in the embryonic excitable cell membrane. Science. 1972 Jun 30;176(4042):1441–1443. doi: 10.1126/science.176.4042.1441. [DOI] [PubMed] [Google Scholar]
- Okamoto H., Takahashi K., Yoshii M. Membrane currents of the tunicate egg under the voltage-clamp condition. J Physiol. 1976 Jan;254(3):607–638. doi: 10.1113/jphysiol.1976.sp011249. [DOI] [PMC free article] [PubMed] [Google Scholar]
- Robbins N., Yonezawa T. Physiological studies during formation and development of rat neuromuscular junctions in tissue culture. J Gen Physiol. 1971 Oct;58(4):467–481. doi: 10.1085/jgp.58.4.467. [DOI] [PMC free article] [PubMed] [Google Scholar]
- Schaeffer B. E., Schaeffer H. E., Brick I. Cell electrophoresis of amphibian blastula and gastrula cells; the relationship of surface charge and morphogenetic movement. Dev Biol. 1973 Sep;34(1):66–76. doi: 10.1016/0012-1606(73)90339-4. [DOI] [PubMed] [Google Scholar]
- Steinbach J. H., Harris A. J., Patrick J., Schubert D., Heinemann S. Nerve-muscle interaction in vitro. Role of acetylcholine. J Gen Physiol. 1973 Sep;62(3):255–270. doi: 10.1085/jgp.62.3.255. [DOI] [PMC free article] [PubMed] [Google Scholar]
- Sytkowski A. J., Vogel Z., Nirenberg M. W. Development of acetylcholine receptor clusters on cultured muscle cells. Proc Natl Acad Sci U S A. 1973 Jan;70(1):270–274. doi: 10.1073/pnas.70.1.270. [DOI] [PMC free article] [PubMed] [Google Scholar]
- TAKEUCHI A., TAKEUCHI N. Active phase of frog's end-plate potential. J Neurophysiol. 1959 Jul;22(4):395–411. doi: 10.1152/jn.1959.22.4.395. [DOI] [PubMed] [Google Scholar]
- TAKEUCHI A., TAKEUCHI N. On the permeability of end-plate membrane during the action of transmitter. J Physiol. 1960 Nov;154:52–67. doi: 10.1113/jphysiol.1960.sp006564. [DOI] [PMC free article] [PubMed] [Google Scholar]
- Takahashi K., Miyazaki S. I., Kidokoro Y. Development of excitability in embryonic muscle cell membranes in certain tunicates. Science. 1971 Jan 29;171(3969):415–418. doi: 10.1126/science.171.3969.415. [DOI] [PubMed] [Google Scholar]
- Vogel Z., Sytkowski A. J., Nirenberg M. W. Acetylcholine receptors of muscle grown in vitro. Proc Natl Acad Sci U S A. 1972 Nov;69(11):3180–3184. doi: 10.1073/pnas.69.11.3180. [DOI] [PMC free article] [PubMed] [Google Scholar]