Abstract
1. Maximum and submaximum Ca-activated tension in mechanically disrupted rat ventricular fibres was examined in solutions containing 30 micron, 100 micron and 4 mM-MgATP and either 50 micron or 1 mM ionized Mg. 2. In the absence of added Ca, significant amounts of base-line tension (up to 50% of maximum) develop in solutions containing less than 30 micron-MgATP. This effect is Mg-dependent; more tension is produced with 50 micron-Mg than with 1 mM. 3. Increasing the MgATP concentration shifts the pCa-% maximum tension relationship in the direction of increasing Ca required for activation. At 50 micron-Mg the pCa which produces 50% maximum tension is 5-8, 5-3 and 5-5 for the 30 micron, 100 micron and 4 mM-MgATP solutions. The effect of MgATP on position is relatively independent of the Mg concentration. 4. The steepness of the pCa-% maximum tension curve increases as MgATP is elevated to the millimolar range. The Hill coefficients for the different MgATP curves at 50 micron-Mg are 1-1, 1-3 and 3-0. This change in steepness accounts for the slightly lower Ca concentration needed for half-maximum tension as the MgATP concentration is increased to millimolar levels. Raising the Mg concentration to 1 mM greatly diminishes the effect of MgATP on the slope of the pCa-tension relationship. 5. The maximum tnesion a fibre bundle can produce decreases as the amount of MgATP is raised from micromolar to millimolar levels. For 50 muM-Mg, maximum tension drops about 35% as MgATP is raised from 30 micronM to 4 mM. For any concentraiton of MgATP, maximum tension is higher at 1 mM-Mg than at 50 micron-Mg. 6. Existing theories of interaction between myosin heads and the thin filament are sufficient to account for the effects of MgATP on the position of the pCa-tension curves and on maximum tension. The effects on slope are less satisfactorily explained.
Full text
PDF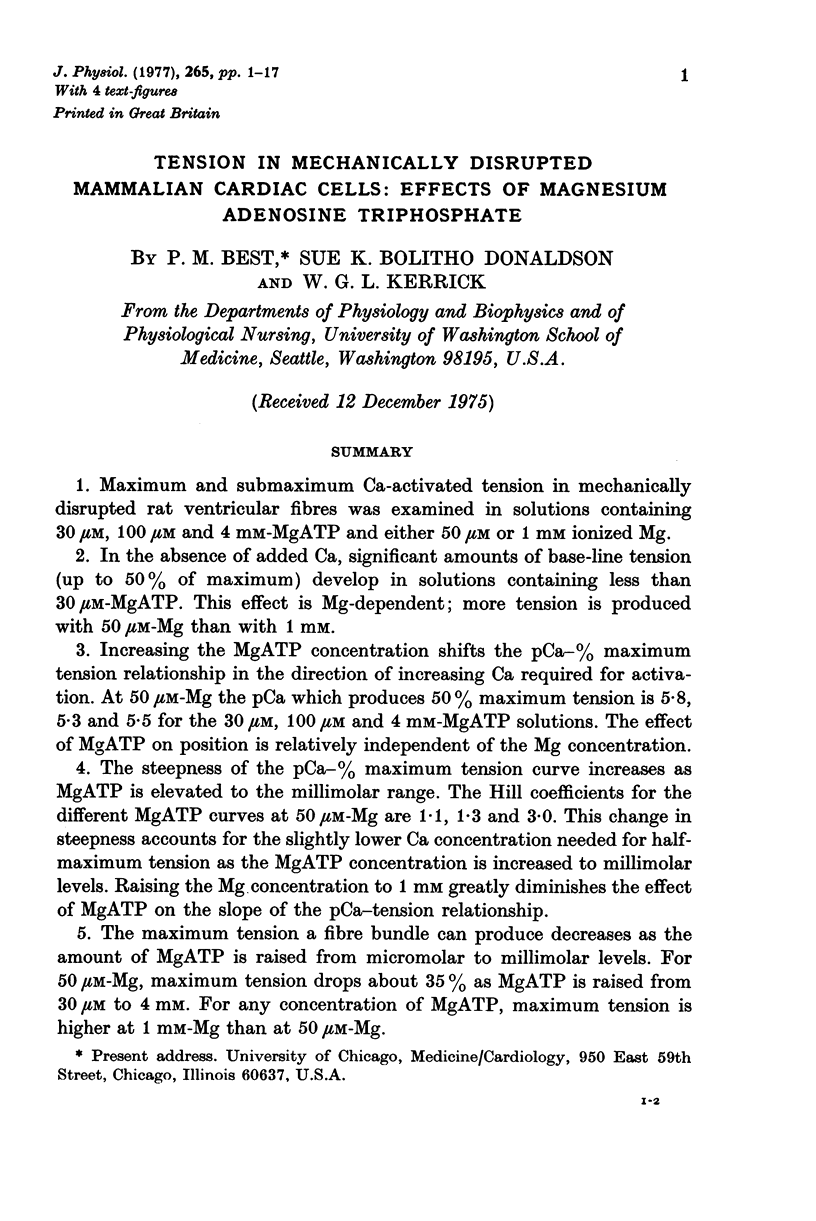
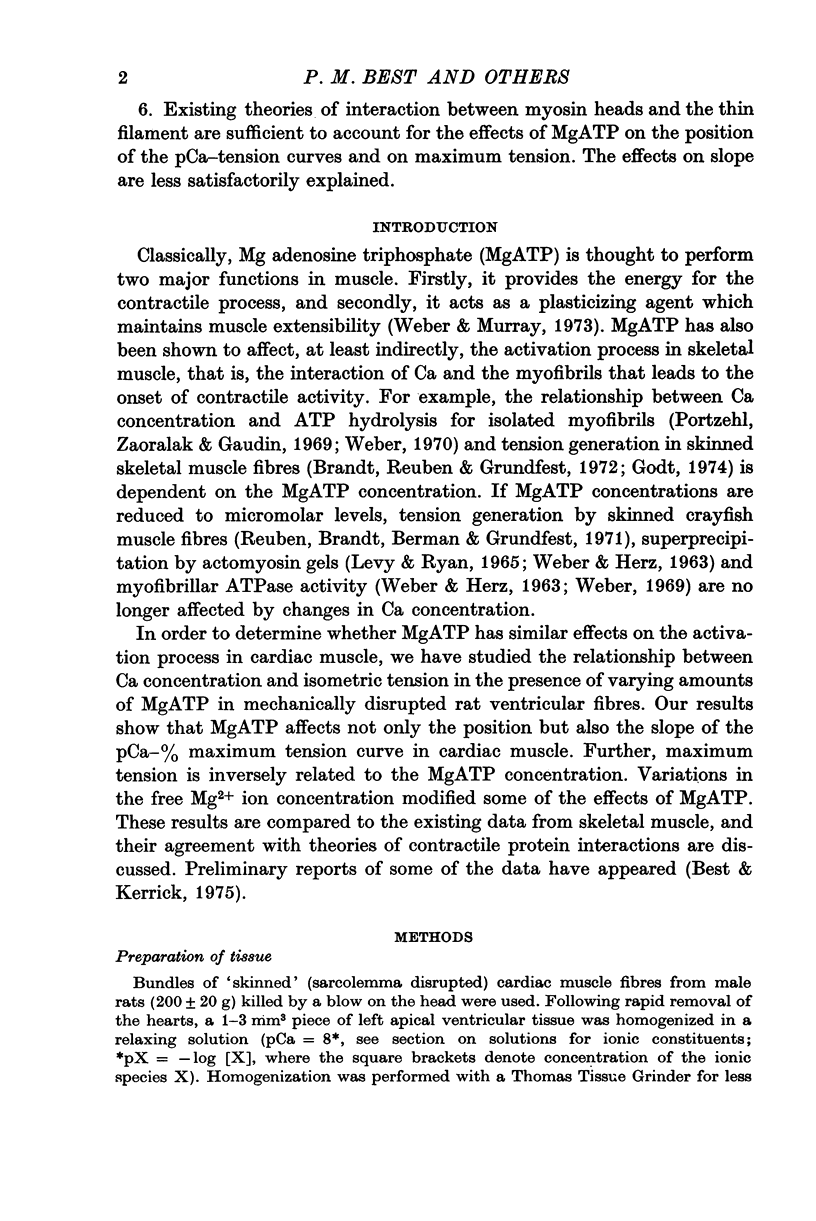
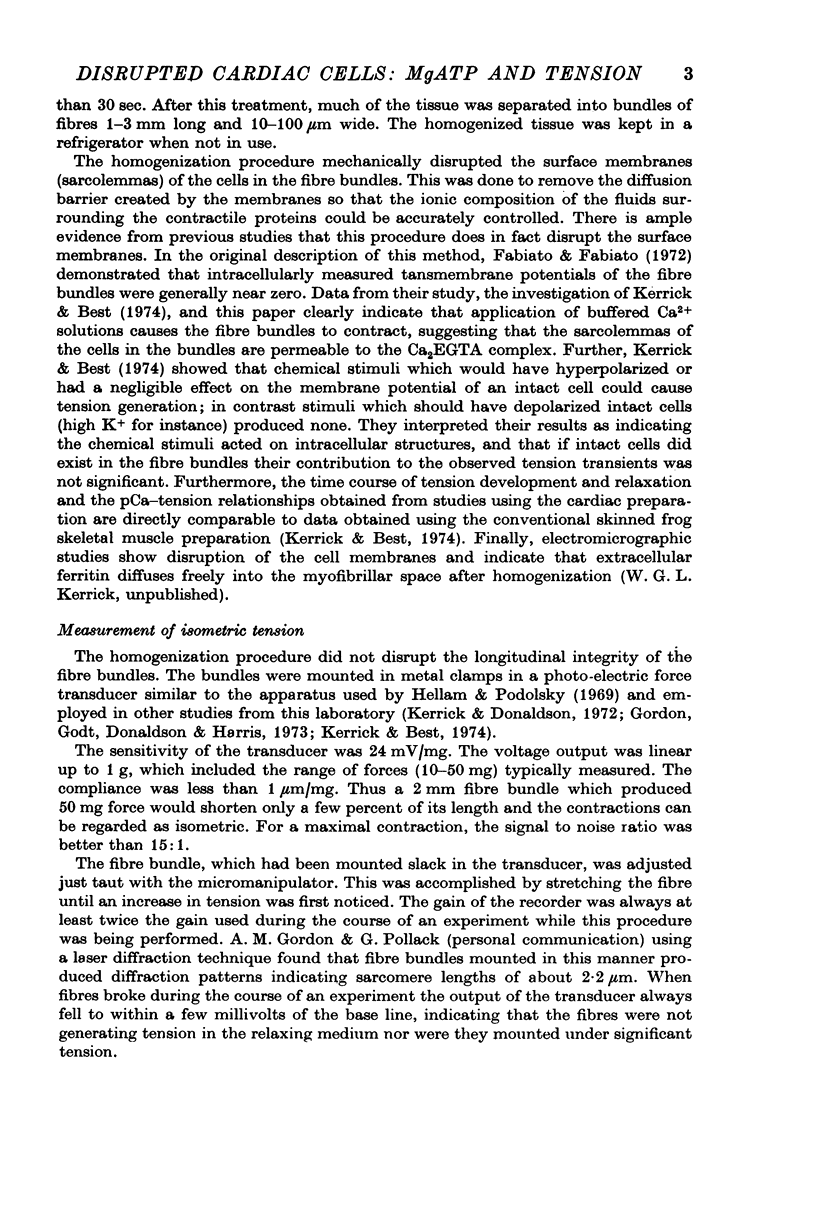
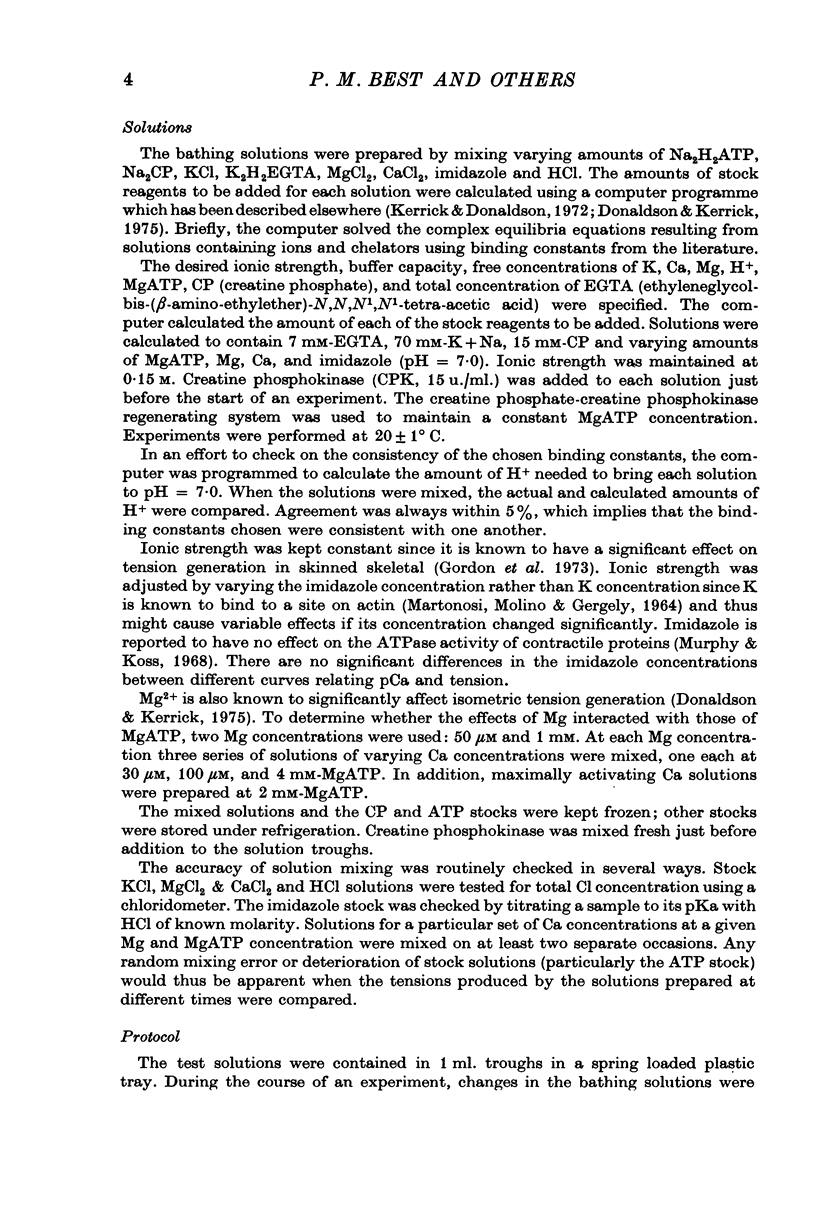
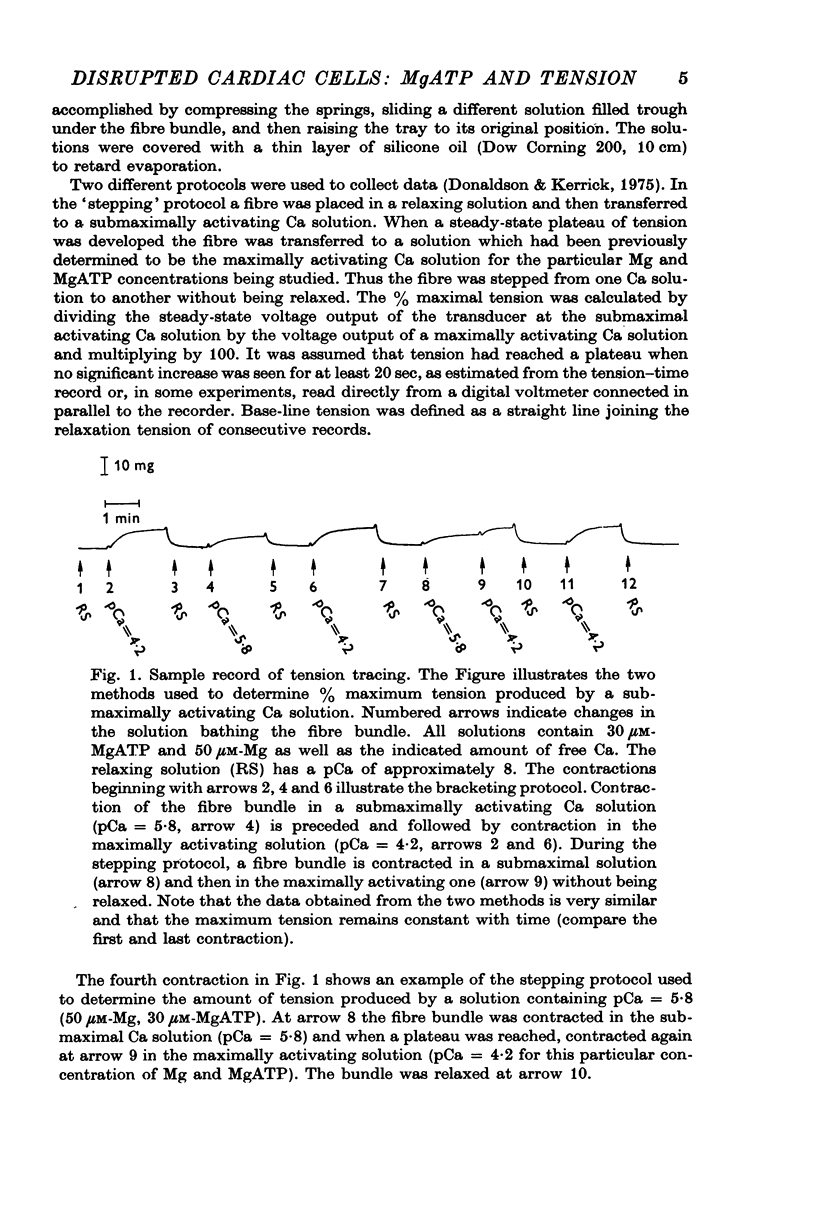
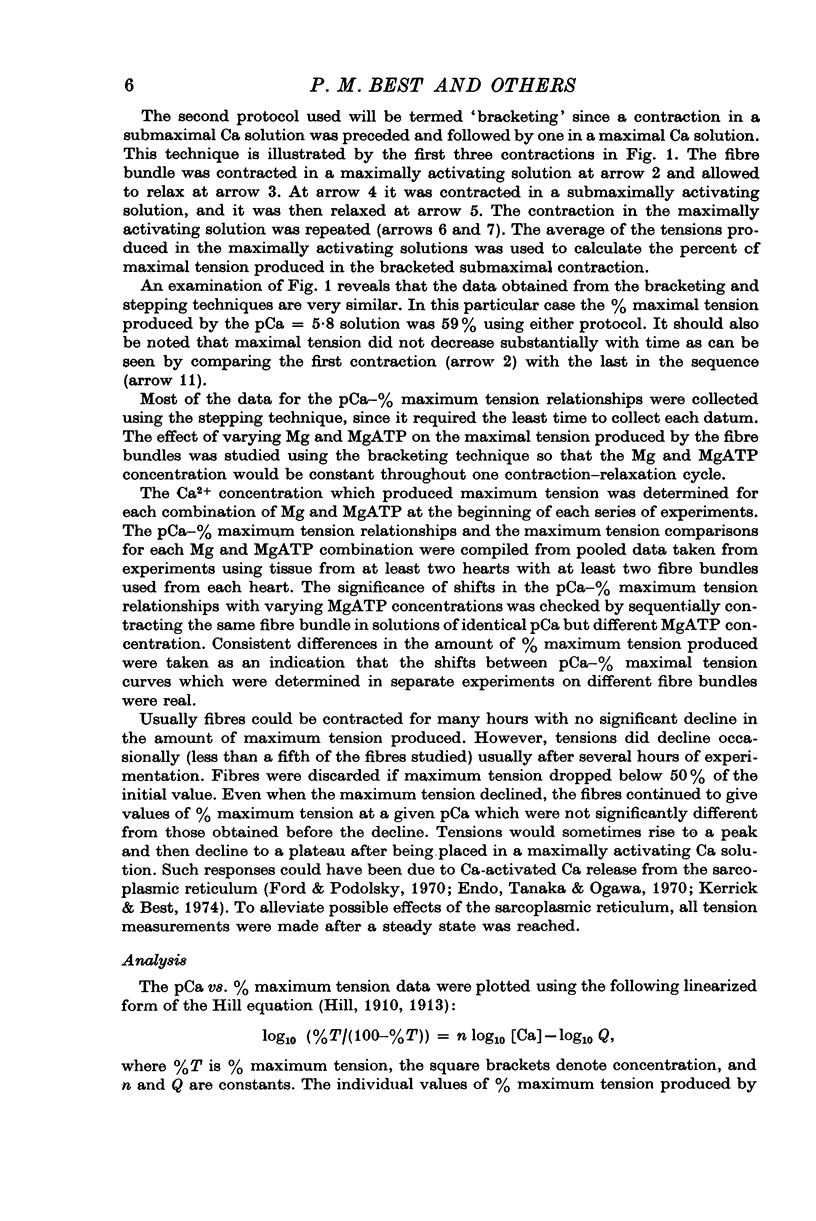
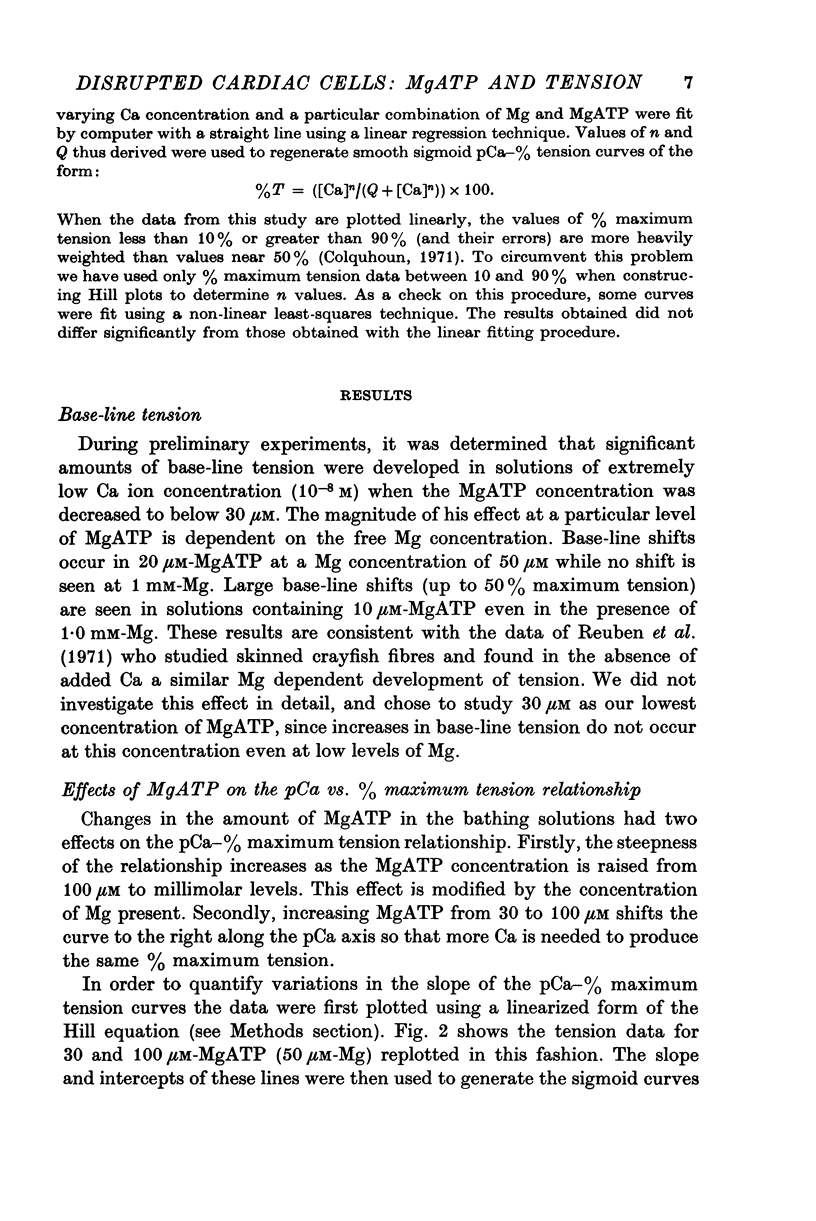
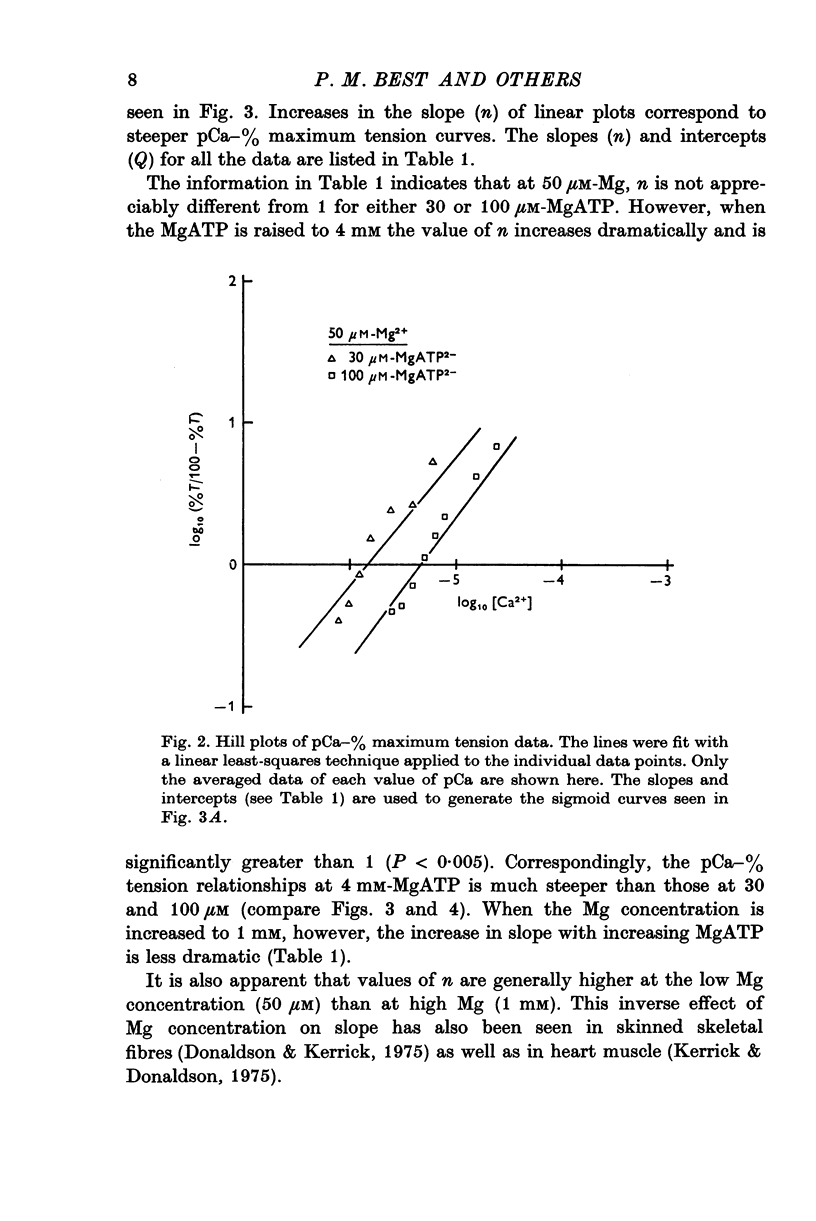
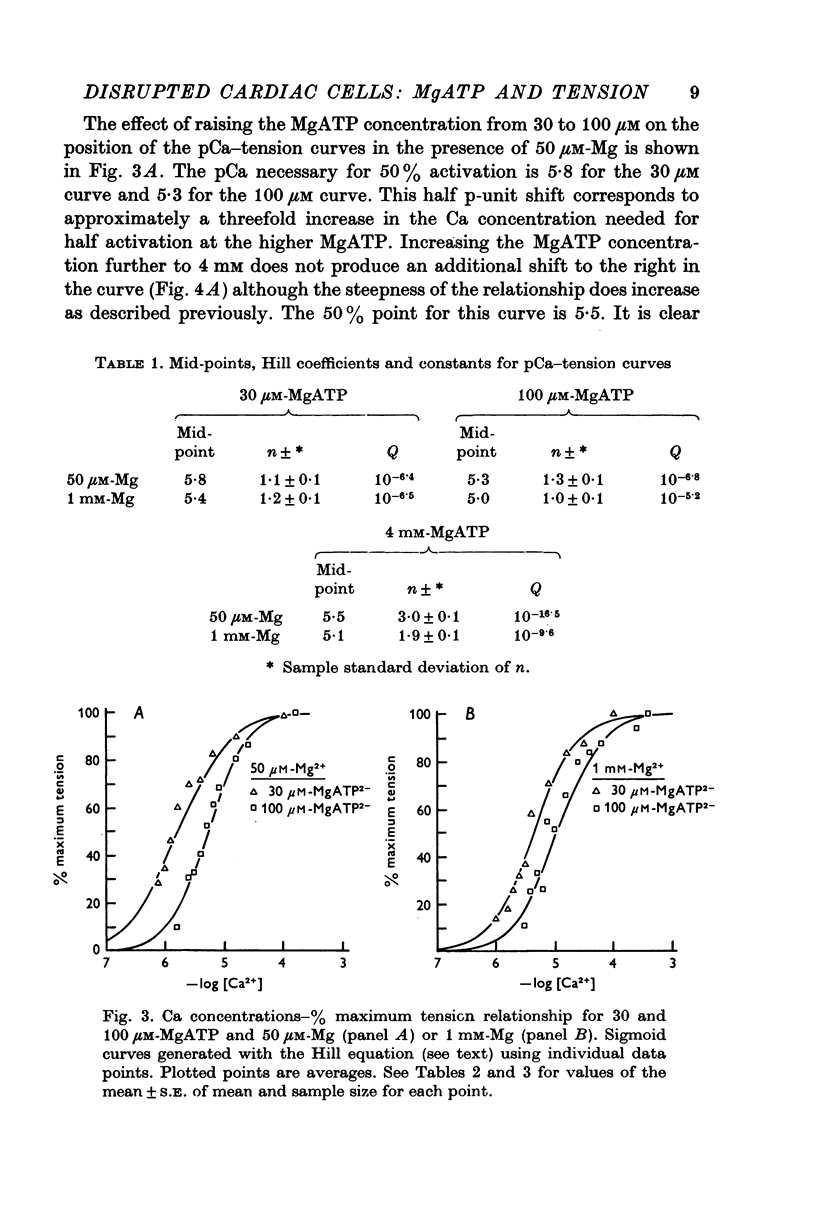
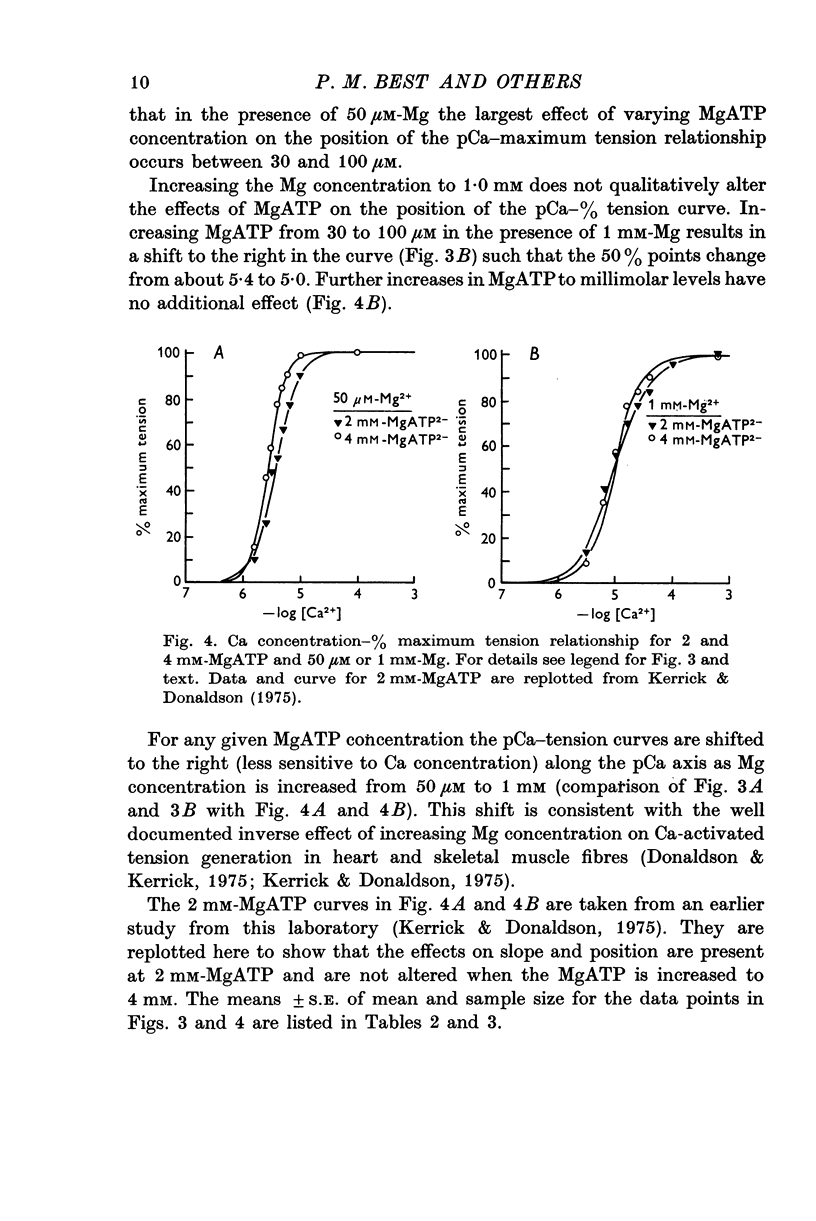
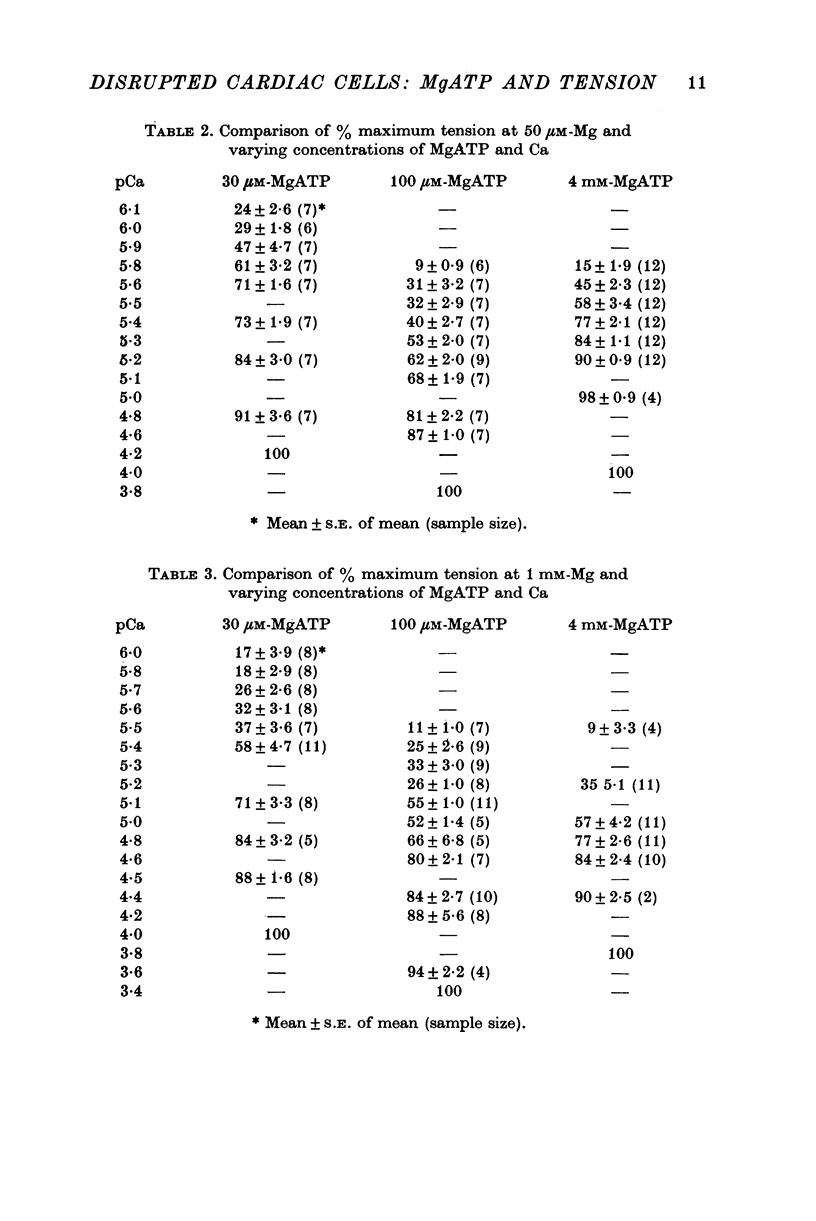
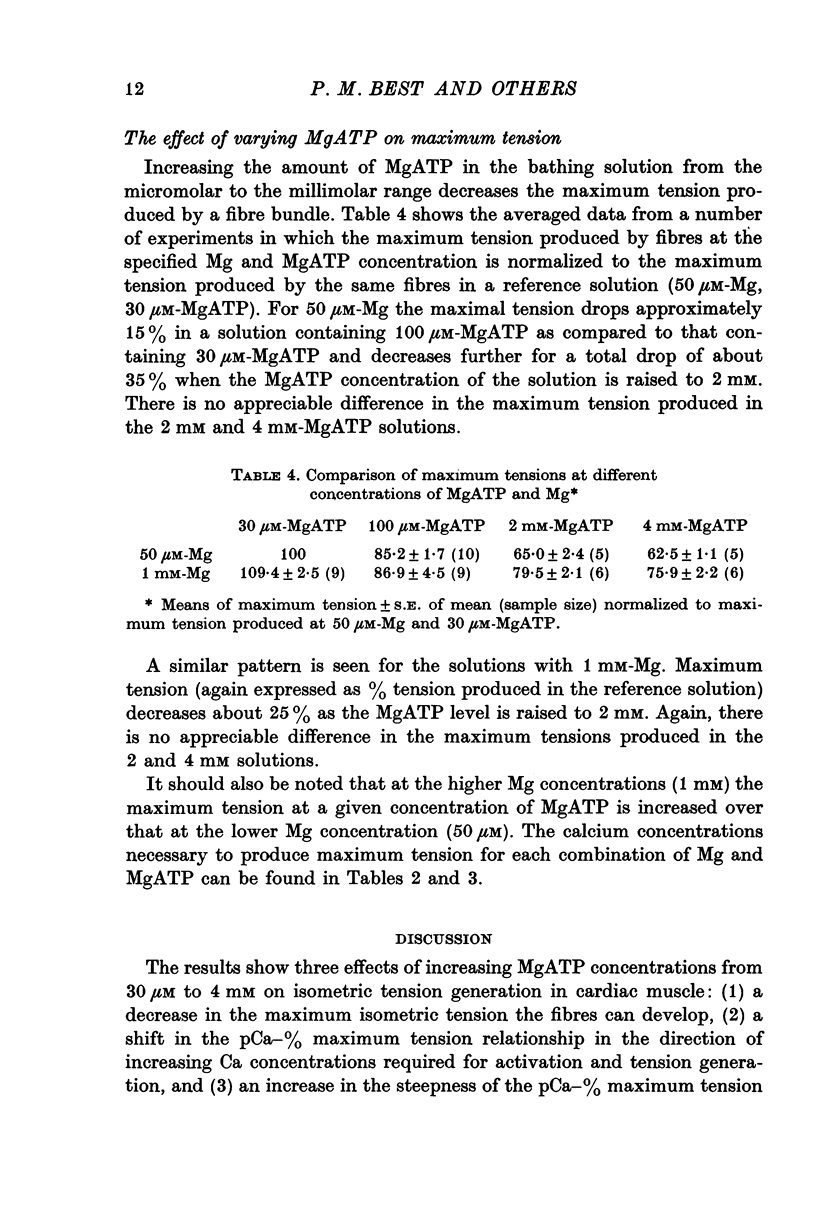
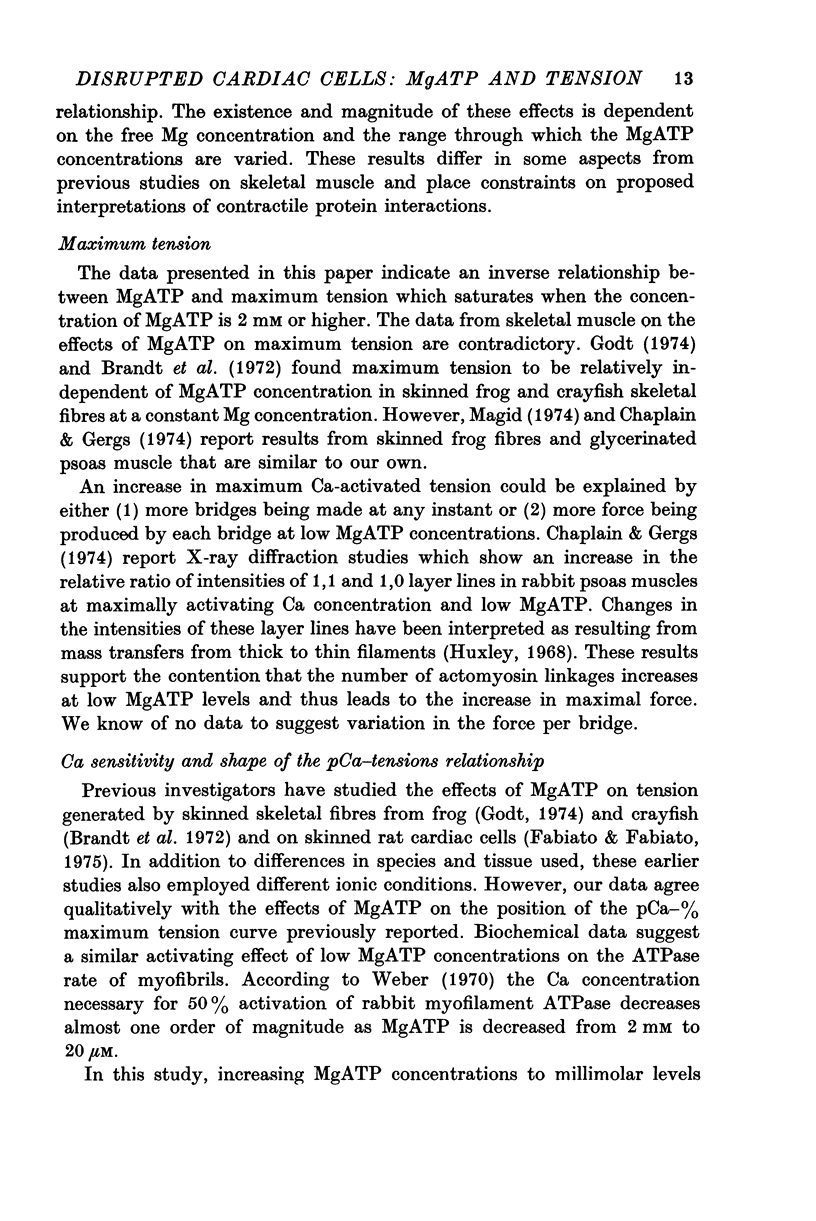
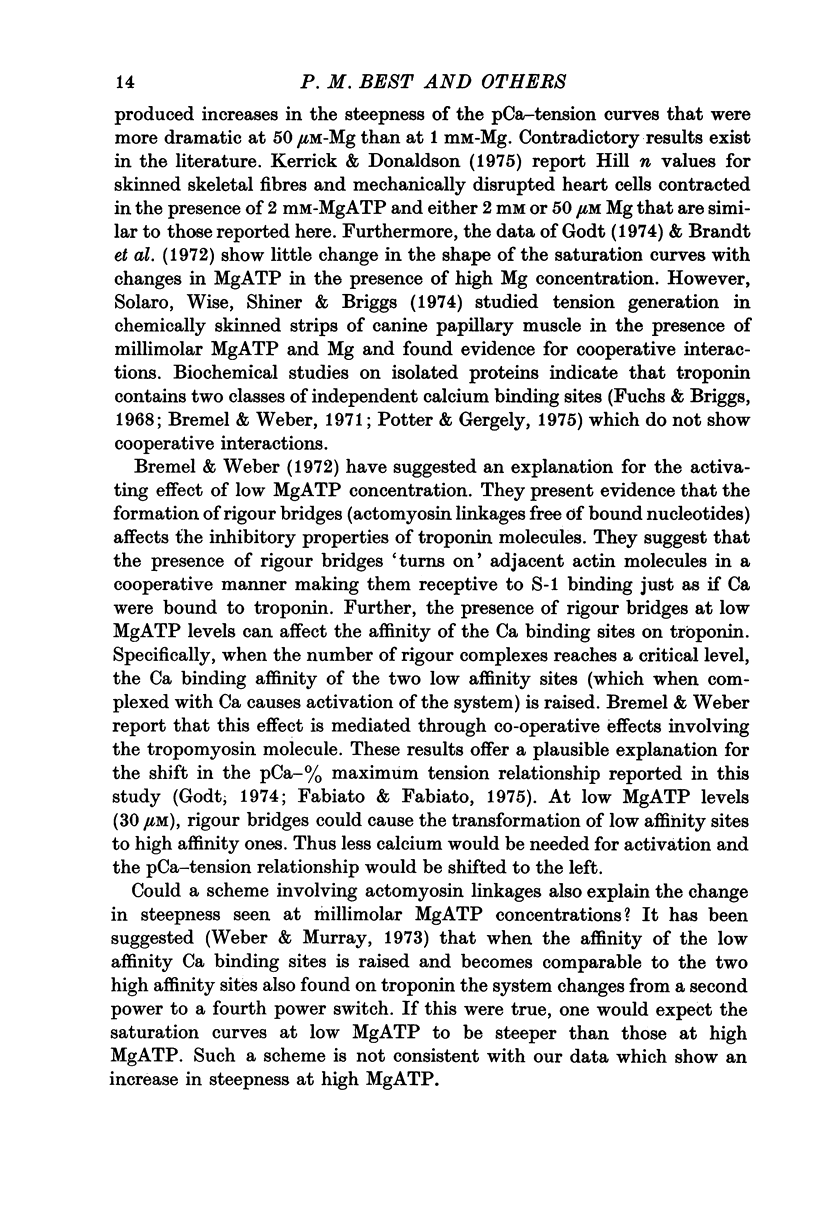
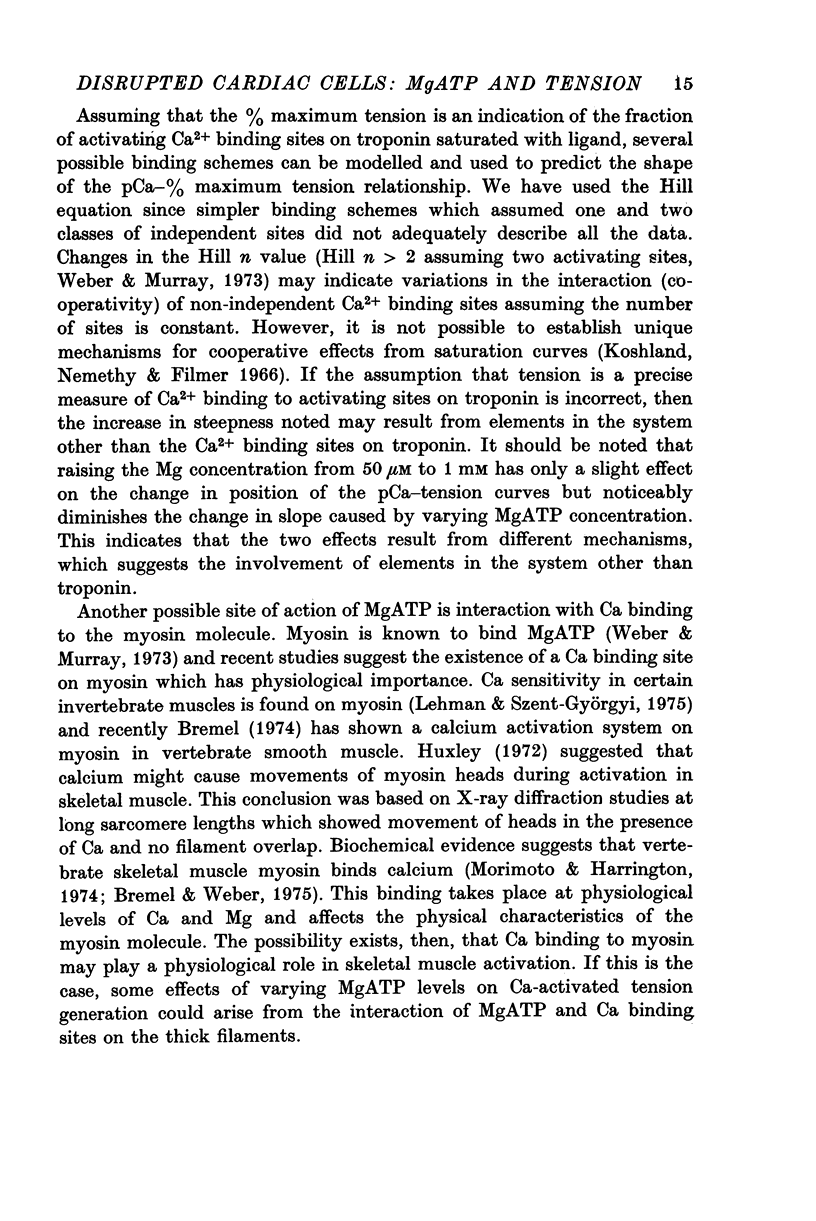
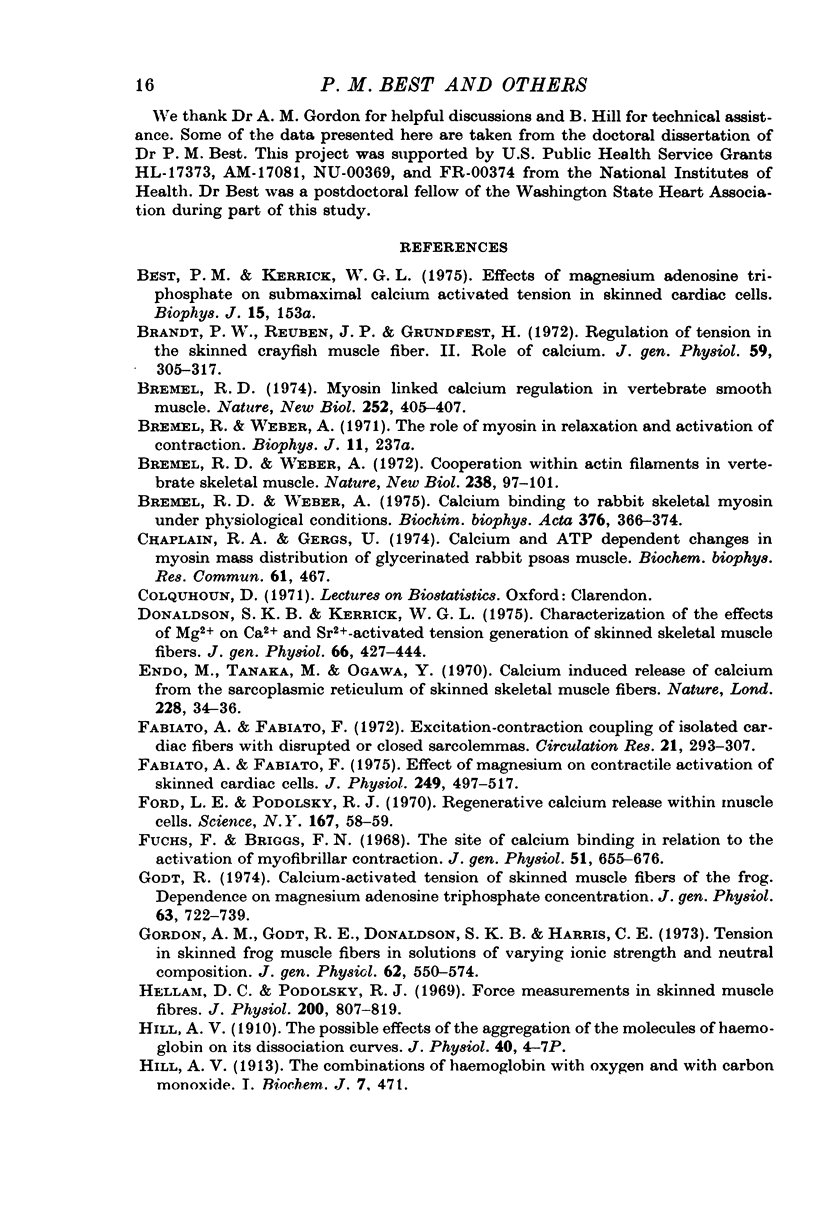
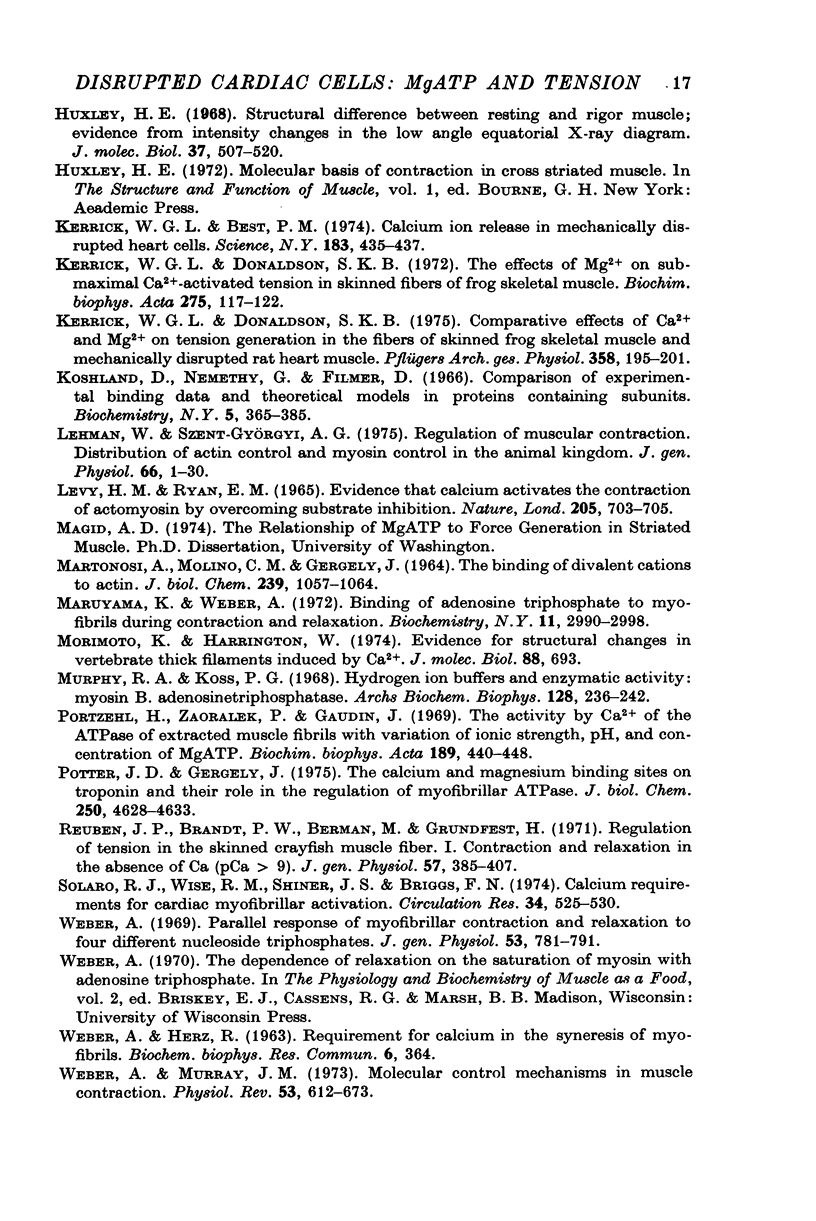
Selected References
These references are in PubMed. This may not be the complete list of references from this article.
- Brandt P. W., Reuben J. P., Grundfest H. Regulation of tension in the skinned crayfish muscle fiber. II. Role of calcium. J Gen Physiol. 1972 Mar;59(3):305–317. doi: 10.1085/jgp.59.3.305. [DOI] [PMC free article] [PubMed] [Google Scholar]
- Bremel R. D. Myosin linked calcium regulation in vertebrate smooth muscle. Nature. 1974 Nov 29;252(5482):405–407. doi: 10.1038/252405a0. [DOI] [PubMed] [Google Scholar]
- Bremel R. D., Weber A. Calcium binding to rabbit skeletal myosin under physiological conditions. Biochim Biophys Acta. 1975 Feb 17;376(2):366–374. doi: 10.1016/0005-2728(75)90028-6. [DOI] [PubMed] [Google Scholar]
- Bremel R. D., Weber A. Cooperation within actin filament in vertebrate skeletal muscle. Nat New Biol. 1972 Jul 26;238(82):97–101. doi: 10.1038/newbio238097a0. [DOI] [PubMed] [Google Scholar]
- Donaldson S. K., Kerrick W. G. Characterization of the effects of Mg2+ on Ca2+- and Sr2+-activated tension generation of skinned skeletal muscle fibers. J Gen Physiol. 1975 Oct;66(4):427–444. doi: 10.1085/jgp.66.4.427. [DOI] [PMC free article] [PubMed] [Google Scholar]
- Endo M., Tanaka M., Ogawa Y. Calcium induced release of calcium from the sarcoplasmic reticulum of skinned skeletal muscle fibres. Nature. 1970 Oct 3;228(5266):34–36. doi: 10.1038/228034a0. [DOI] [PubMed] [Google Scholar]
- Fabiato A., Fabiato F. Effects of magnesium on contractile activation of skinned cardiac cells. J Physiol. 1975 Aug;249(3):497–517. doi: 10.1113/jphysiol.1975.sp011027. [DOI] [PMC free article] [PubMed] [Google Scholar]
- Fabiato A., Fabiato F. Excitation-contraction coupling of isolated cardiac fibers with disrupted or closed sarcolemmas. Calcium-dependent cyclic and tonic contractions. Circ Res. 1972 Sep;31(3):293–307. doi: 10.1161/01.res.31.3.293. [DOI] [PubMed] [Google Scholar]
- Ford L. E., Podolsky R. J. Regenerative calcium release within muscle cells. Science. 1970 Jan 2;167(3914):58–59. doi: 10.1126/science.167.3914.58. [DOI] [PubMed] [Google Scholar]
- Fuchs F., Briggs F. N. The site of calcium binding in relation to the activation of myofibrillar contraction. J Gen Physiol. 1968 May;51(5):655–676. doi: 10.1085/jgp.51.5.655. [DOI] [PMC free article] [PubMed] [Google Scholar]
- Glenn W., Kerrick L., Donaldson S. K. The comparative effects of (Ca2+) and (Mg2+) on on tension generation in the fibers of skinned frog skeletal muscle and mechanically disrupted rat ventricular cardiac muscle. Pflugers Arch. 1975 Jul 28;358(3):195–201. doi: 10.1007/BF00587216. [DOI] [PubMed] [Google Scholar]
- Godt R. E. Calcium-activated tension of skinned muscle fibers of the frog. Dependence on magnesium adenosine triphosphate concentration. J Gen Physiol. 1974 Jun;63(6):722–739. doi: 10.1085/jgp.63.6.722. [DOI] [PMC free article] [PubMed] [Google Scholar]
- Gordon A. M., Godt R. E., Donaldson S. K., Harris C. E. Tension in skinned frog muscle fibers in solutions of varying ionic strength and neutral salt composition. J Gen Physiol. 1973 Nov;62(5):550–574. doi: 10.1085/jgp.62.5.550. [DOI] [PMC free article] [PubMed] [Google Scholar]
- Hellam D. C., Podolsky R. J. Force measurements in skinned muscle fibres. J Physiol. 1969 Feb;200(3):807–819. doi: 10.1113/jphysiol.1969.sp008723. [DOI] [PMC free article] [PubMed] [Google Scholar]
- Hill A. V. The Combinations of Haemoglobin with Oxygen and with Carbon Monoxide. I. Biochem J. 1913 Oct;7(5):471–480. doi: 10.1042/bj0070471. [DOI] [PMC free article] [PubMed] [Google Scholar]
- Huxley H. E. Structural difference between resting and rigor muscle; evidence from intensity changes in the lowangle equatorial x-ray diagram. J Mol Biol. 1968 Nov 14;37(3):507–520. doi: 10.1016/0022-2836(68)90118-6. [DOI] [PubMed] [Google Scholar]
- Kerrick W. G., Best P. M. Calcium ion release in mechanically disrupted heart cells. Science. 1974 Feb 1;183(4123):435–437. doi: 10.1126/science.183.4123.435. [DOI] [PubMed] [Google Scholar]
- Kerrick W. G., Donaldson S. K. The effects of Mg 2+ on submaximum Ca 2+ -activated tension in skinned fibers of frog skeletal muscle. Biochim Biophys Acta. 1972 Jul 12;275(1):117–122. doi: 10.1016/0005-2728(72)90030-8. [DOI] [PubMed] [Google Scholar]
- Koshland D. E., Jr, Némethy G., Filmer D. Comparison of experimental binding data and theoretical models in proteins containing subunits. Biochemistry. 1966 Jan;5(1):365–385. doi: 10.1021/bi00865a047. [DOI] [PubMed] [Google Scholar]
- LEVY H. M., RYAN E. M. EVIDENCE THAT CALCIUM ACTIVATES THE CONTRACTION OF ACTOMYOSIN BY OVERCOMING SUBSTRATE INHIBITION. Nature. 1965 Feb 13;205:703–705. doi: 10.1038/205703b0. [DOI] [PubMed] [Google Scholar]
- Lehman W., Szent-Györgyi A. G. Regulation of muscular contraction. Distribution of actin control and myosin control in the animal kingdom. J Gen Physiol. 1975 Jul;66(1):1–30. doi: 10.1085/jgp.66.1.1. [DOI] [PMC free article] [PubMed] [Google Scholar]
- MARTONOSII A., MOLINO C. M., GERGELY J. THE BINDING OF DIVALENT CATIONS TO ACTIN. J Biol Chem. 1964 Apr;239:1057–1064. [PubMed] [Google Scholar]
- Maruyama K., Weber A. Binding of adenosine triphosphate to myofibrils during contraction and relaxation. Biochemistry. 1972 Aug 1;11(16):2990–2998. doi: 10.1021/bi00766a010. [DOI] [PubMed] [Google Scholar]
- Morimoto K., Harrington W. F. Evidence for structural changes in vertebrate thick filaments induced by calcium. J Mol Biol. 1974 Sep 25;88(3):693–709. doi: 10.1016/0022-2836(74)90417-3. [DOI] [PubMed] [Google Scholar]
- Murphy R. A., Koss P. G. Hydrogen ion buffers and enzymatic activity: myosin B adenosinetriphosphatase. Arch Biochem Biophys. 1968 Oct;128(1):236–242. doi: 10.1016/0003-9861(68)90027-1. [DOI] [PubMed] [Google Scholar]
- Portzehl H., Zaoralek P., Gaudin J. The activation by Ca2+ of the ATPase of extracted muscle fibrilsith variation of ionic strength, pH and concentration of MgATP. Biochim Biophys Acta. 1969;189(3):440–448. doi: 10.1016/0005-2728(69)90175-3. [DOI] [PubMed] [Google Scholar]
- Potter J. D., Gergely J. The calcium and magnesium binding sites on troponin and their role in the regulation of myofibrillar adenosine triphosphatase. J Biol Chem. 1975 Jun 25;250(12):4628–4633. [PubMed] [Google Scholar]
- Reuben J. P., Brandt P. W., Berman M., Grundfest H. Regulation of tension in the skinned crayfish muscle fiber. I. Contraction and relaxation in the absence of Ca (pCa is greater than 9). J Gen Physiol. 1971 Apr;57(4):385–407. doi: 10.1085/jgp.57.4.385. [DOI] [PMC free article] [PubMed] [Google Scholar]
- Solaro R. J., Wise R. M., Shiner J. S., Briggs F. N. Calcium requirements for cardiac myofibrillar activation. Circ Res. 1974 Apr;34(4):525–530. doi: 10.1161/01.res.34.4.525. [DOI] [PubMed] [Google Scholar]
- WEBER A., HERZ R. Requirement for calcium in the synaeresis of myofibrils. Biochem Biophys Res Commun. 1961 Dec 20;6:364–368. doi: 10.1016/0006-291x(61)90146-2. [DOI] [PubMed] [Google Scholar]
- Weber A., Murray J. M. Molecular control mechanisms in muscle contraction. Physiol Rev. 1973 Jul;53(3):612–673. doi: 10.1152/physrev.1973.53.3.612. [DOI] [PubMed] [Google Scholar]
- Weber A. Parallel response of myofibrillar contraction and relaxation to four different nucleoside triphophates. J Gen Physiol. 1969 Jun;53(6):781–791. doi: 10.1085/jgp.53.6.781. [DOI] [PMC free article] [PubMed] [Google Scholar]