Abstract
The intracellular reference phase (RP) method and ultra-low temperature micro-dissection were used for isothermal and isotopic phase distribution studies of Na+, K+, and water in amphibian oocyte cytoplasm. One-third of the cytoplasmic water is available as solvent for [3H]sucrose. This fraction, designated c1, quantitatively coincides with the water volume in which Na+ and K+ are freely diffusible. Two-thirds of the cytoplasmic water is inaccessible to sucrose and is designated c2. The Na+ and K+ associated with c2 are extremely slowly exchanging (bound) and at different concentrations than in c1. The cations in c1 are in mass-action equilibria with those in c2, each described by an equation of the form
Cci = Cc1i + Cc2i = qi·CRPi + maxCc2i·f(CRPi
in which Cci is the cytoplasmic Na+ or K+ concentration, Cc1i is the free, and Cc2i the bound cation concentration averaged over the cytoplasmic water. qi is the fractional free solute space, CRPi the RP concentration, maxCc2i the concentration of binding sites, and the function f is satisfied by the Langmuir isotherm. Numerical values for the variables of the isotherm are determined. Activity coefficients are calculated from RP data and provide a basis for generalizing the oocyte results to other cells. The conclusion is drawn that both c1 and c2 are widely distributed in cells, and that cellular ionic activities involve two distinct systems: the cell-membrane system and an adsorbed water ion-exchange-like buffering system. Alternative explanations for the two-component cytoplasm are considered. A model is proposed in which c1 is a normal intracellular aqueous phase controlled by the plasma membrane, whereas c2 consists of water and ions adsorbed in hydrate crystalline structures. In oocytes these structures are identified with yolk platelets.
Full text
PDF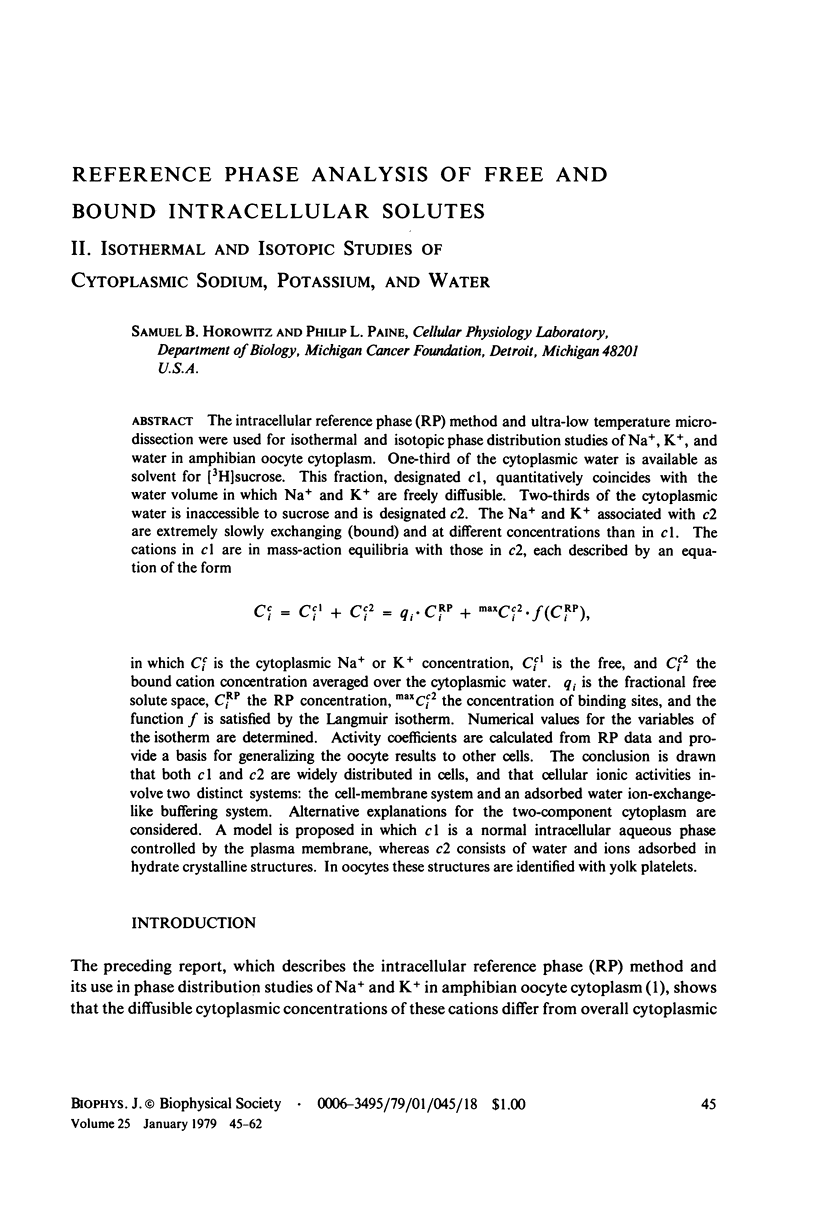
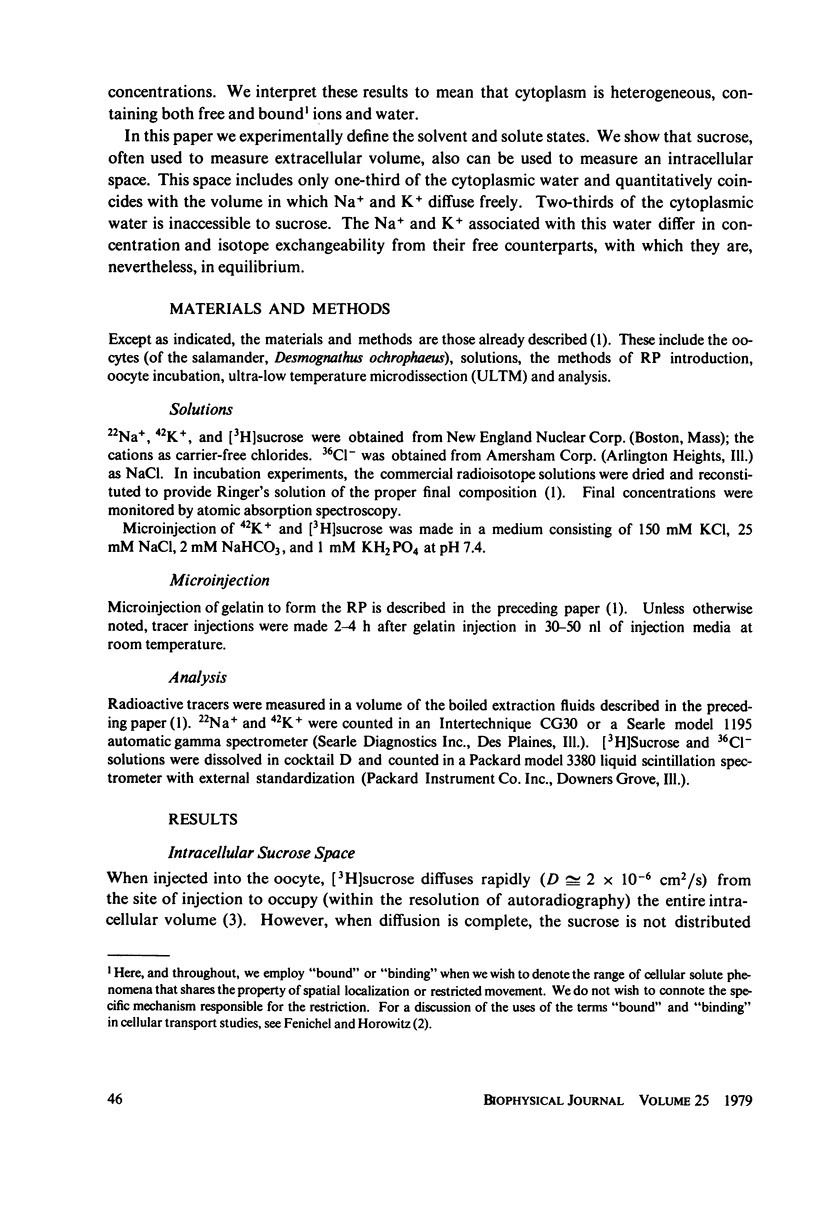
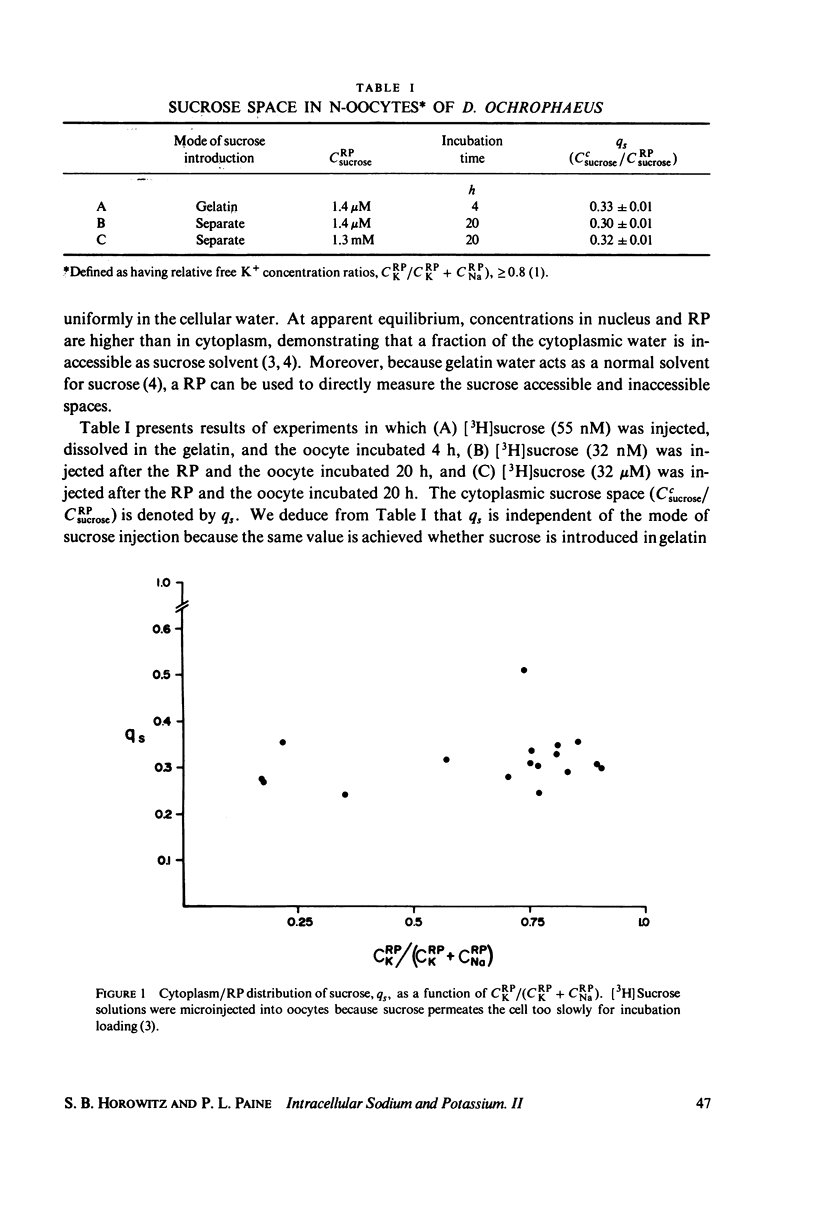
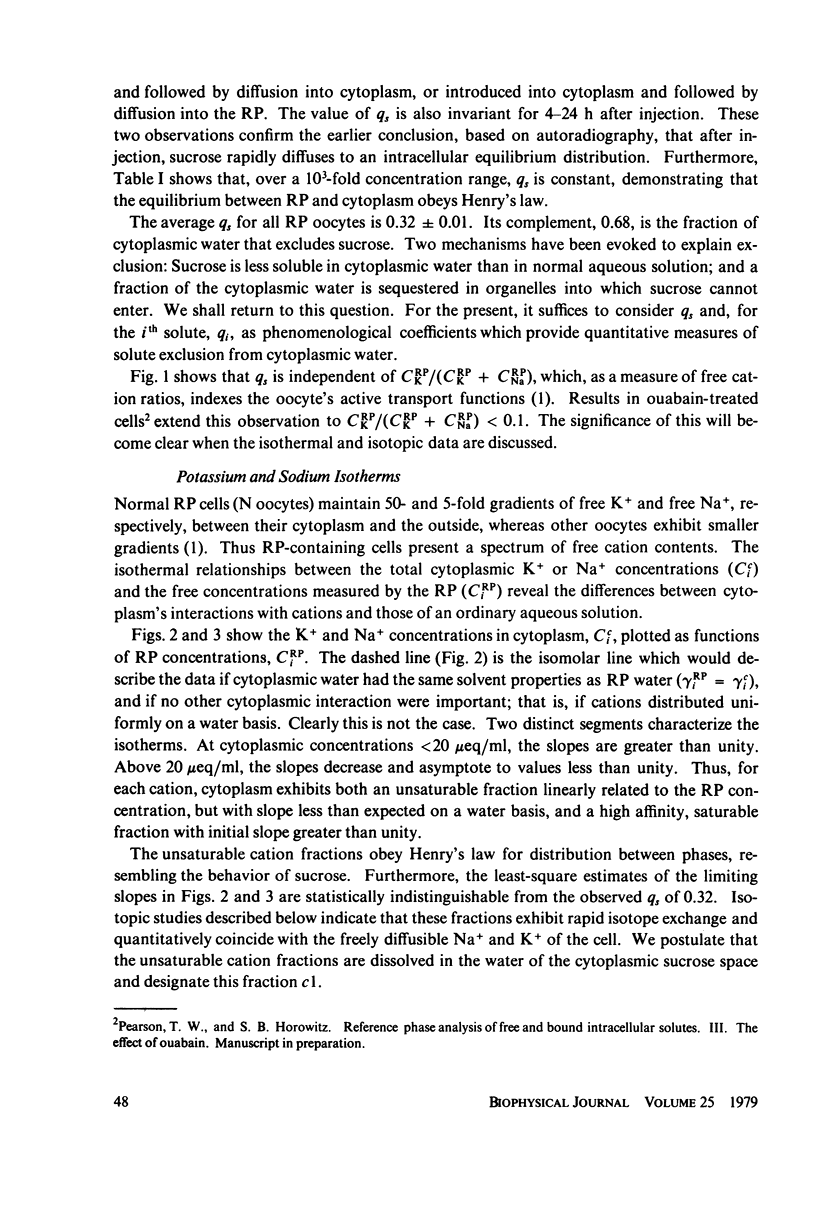
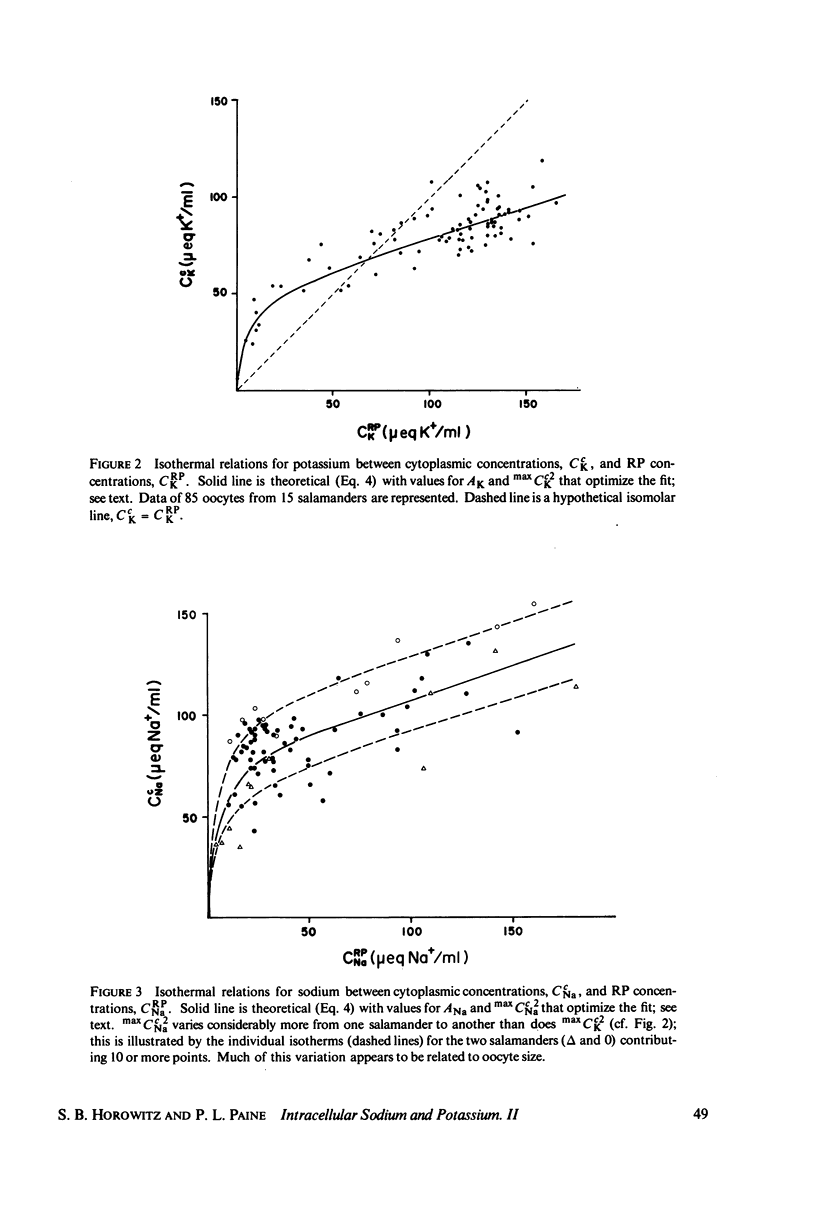
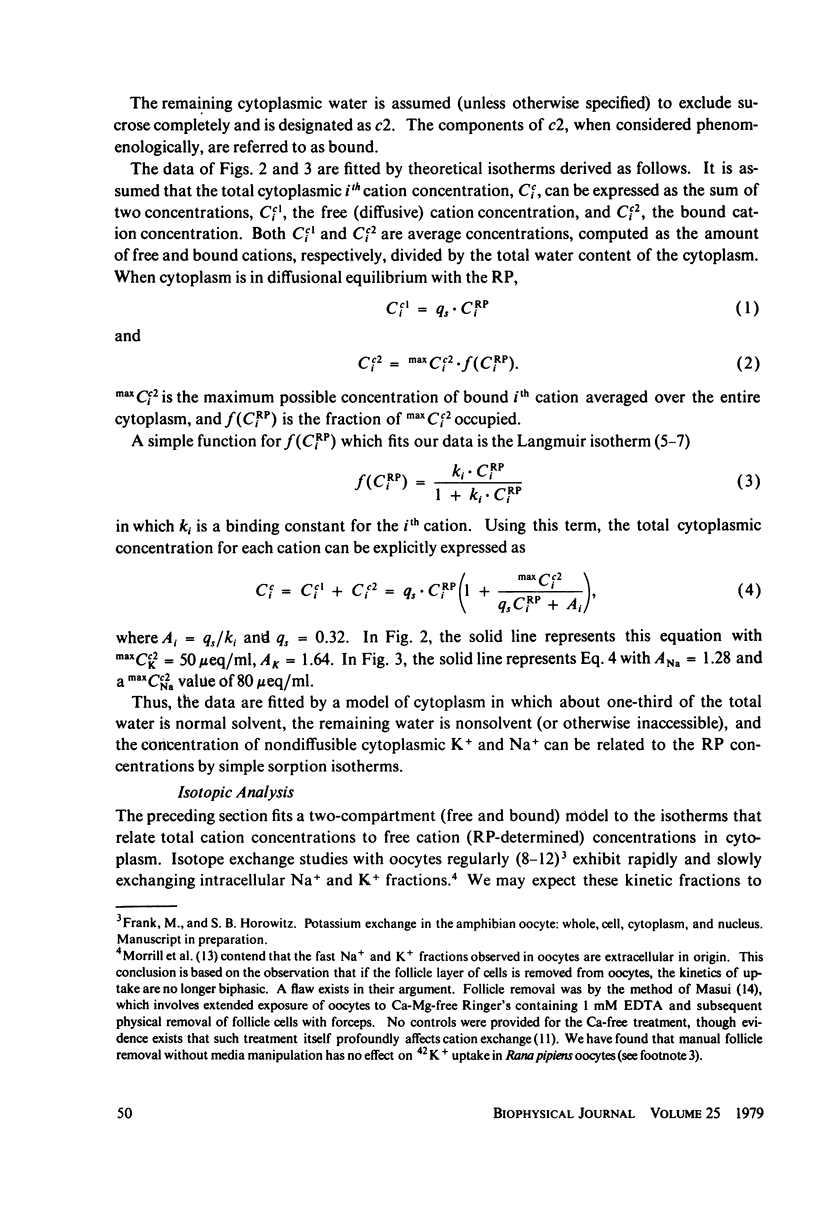
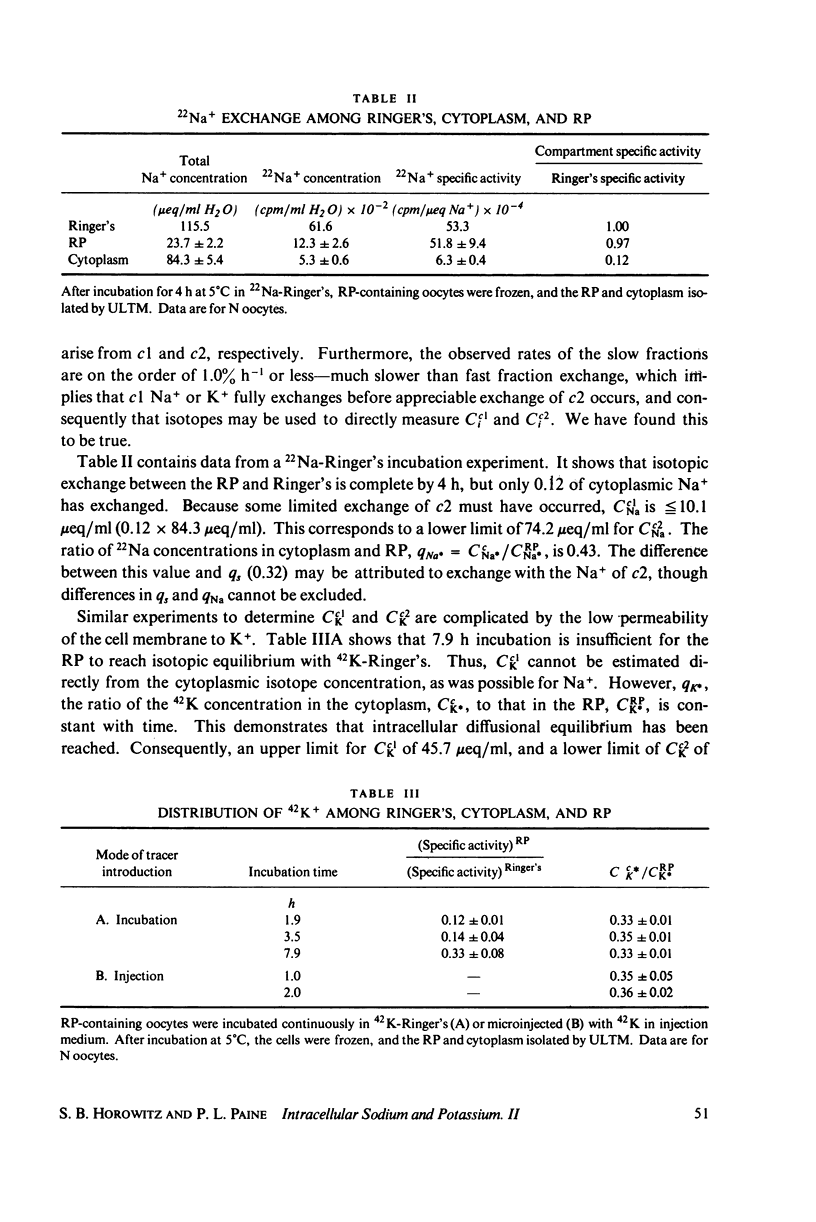
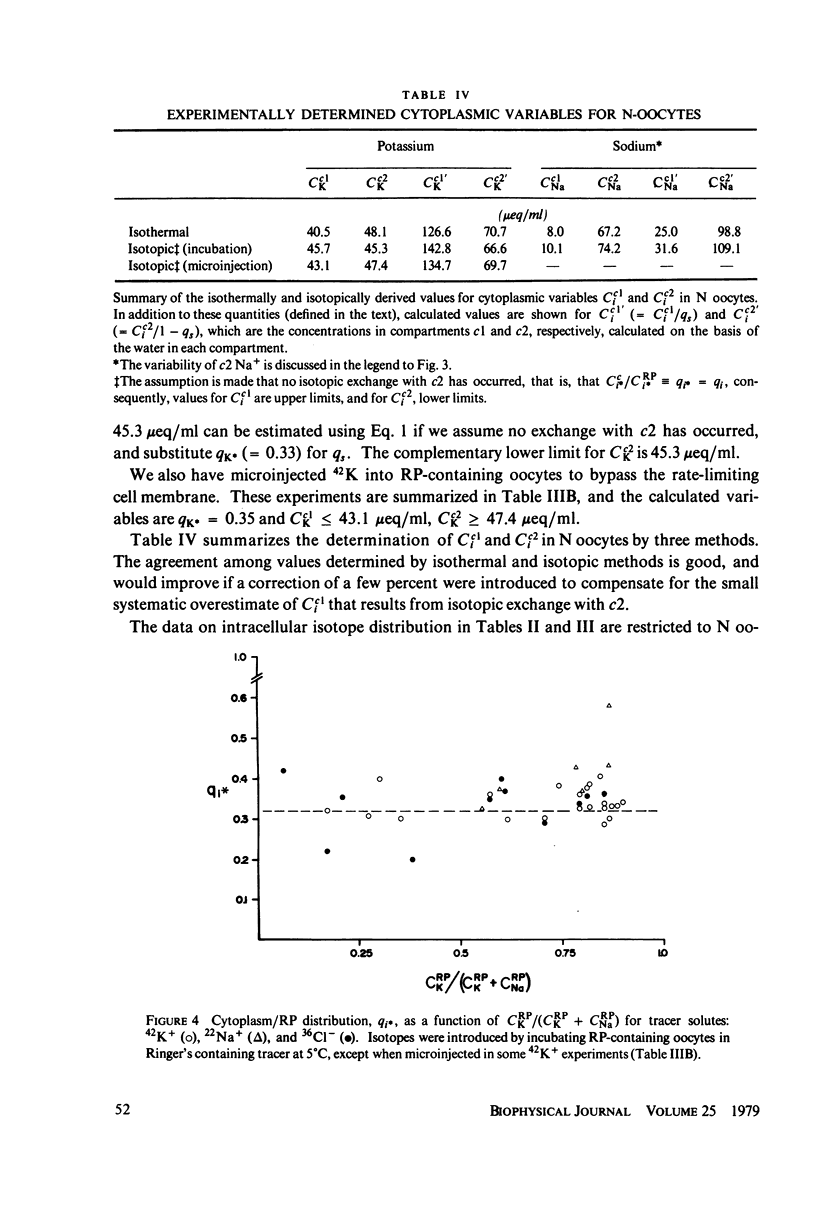
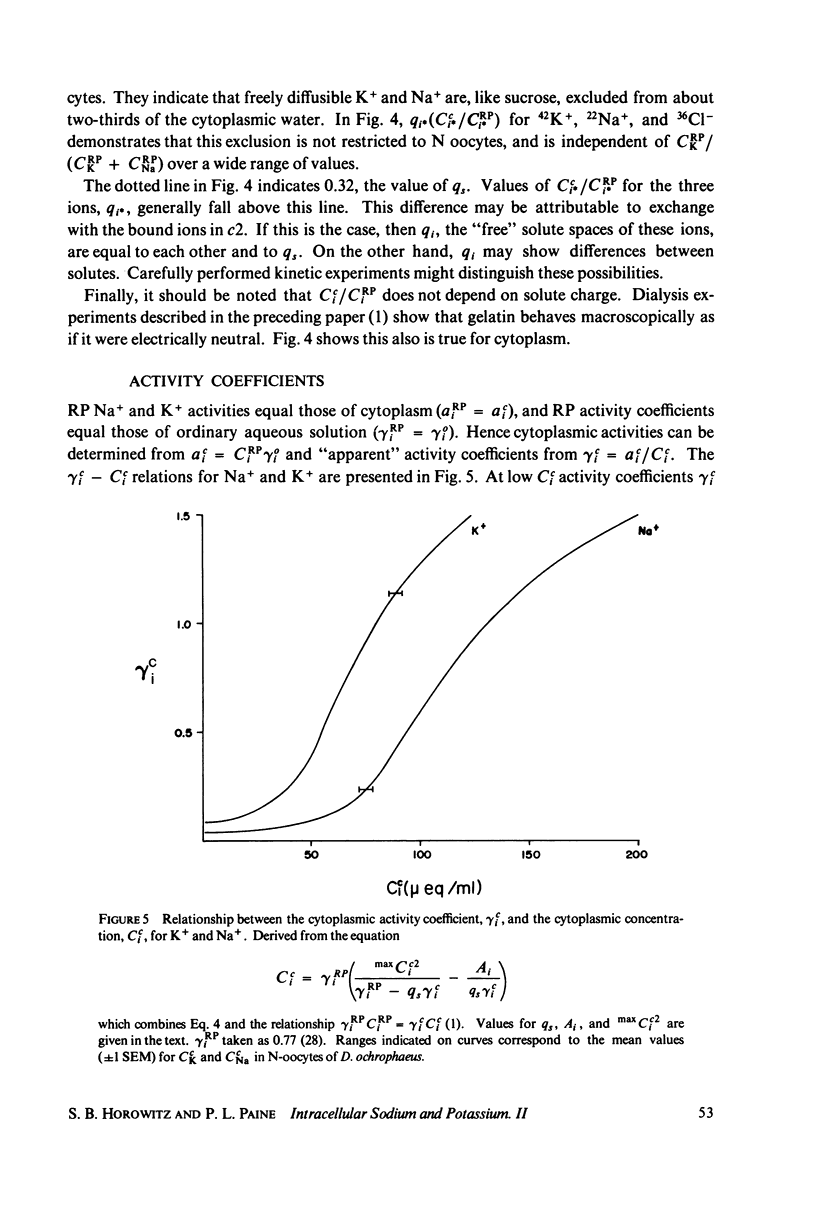
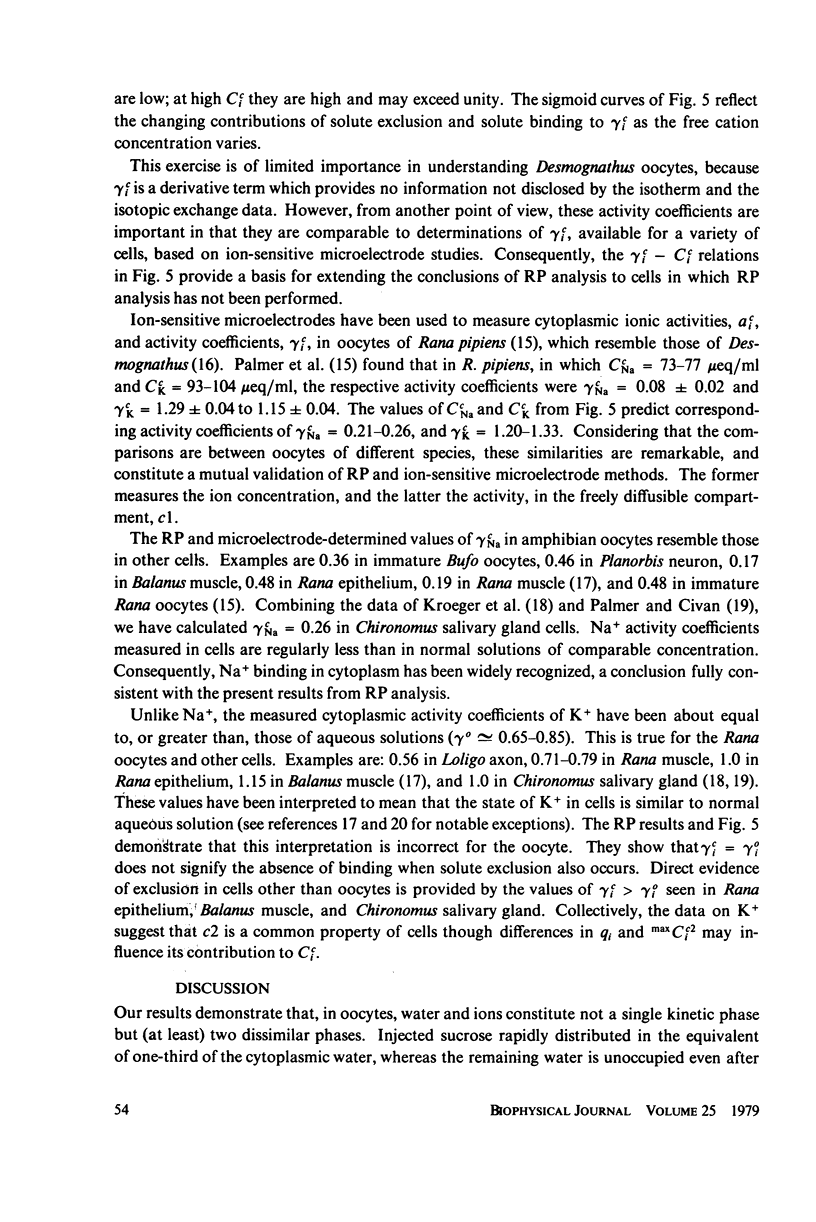
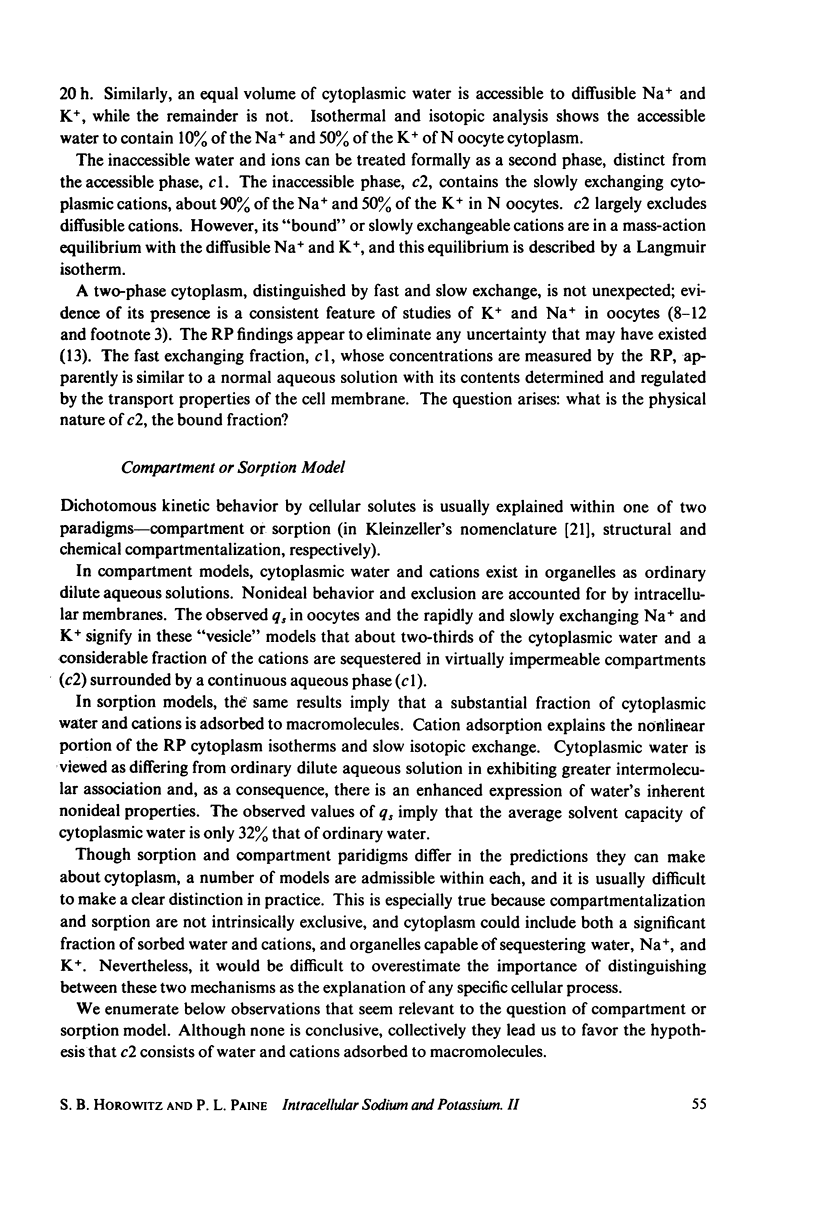
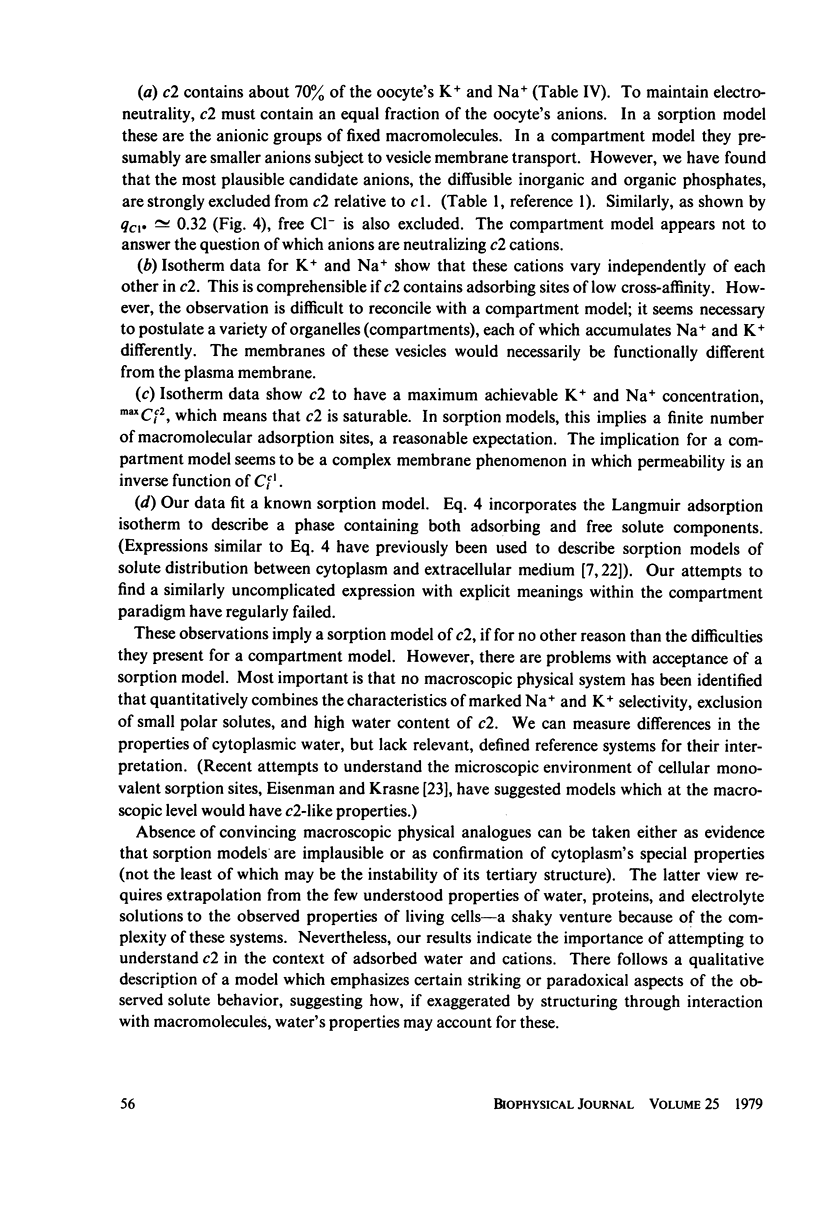
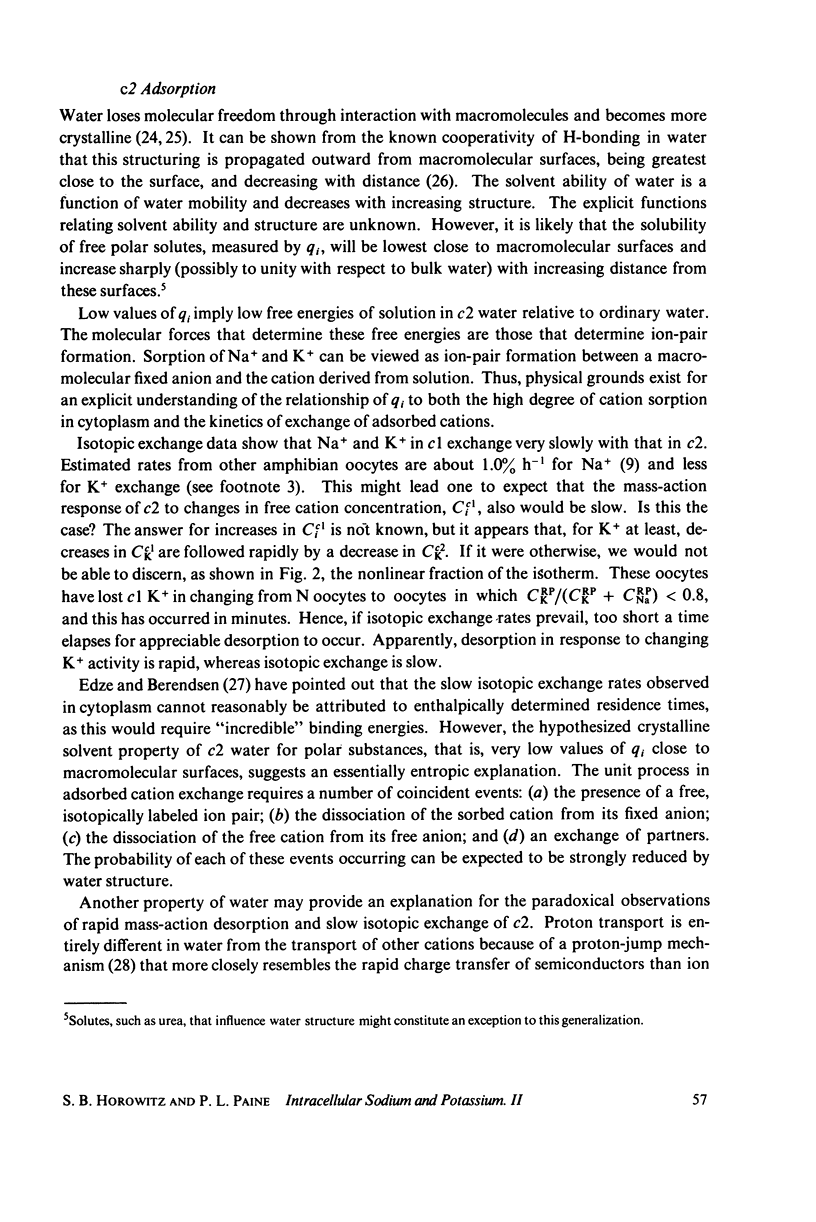
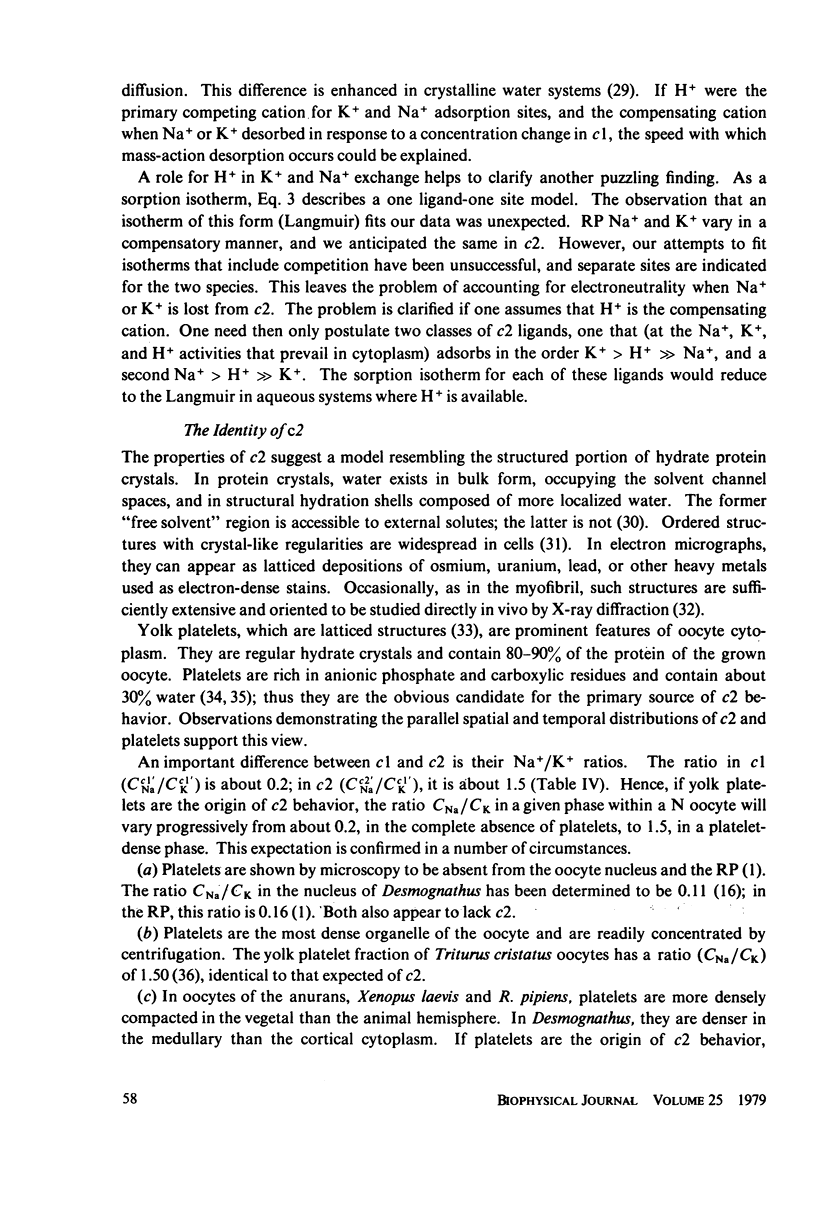
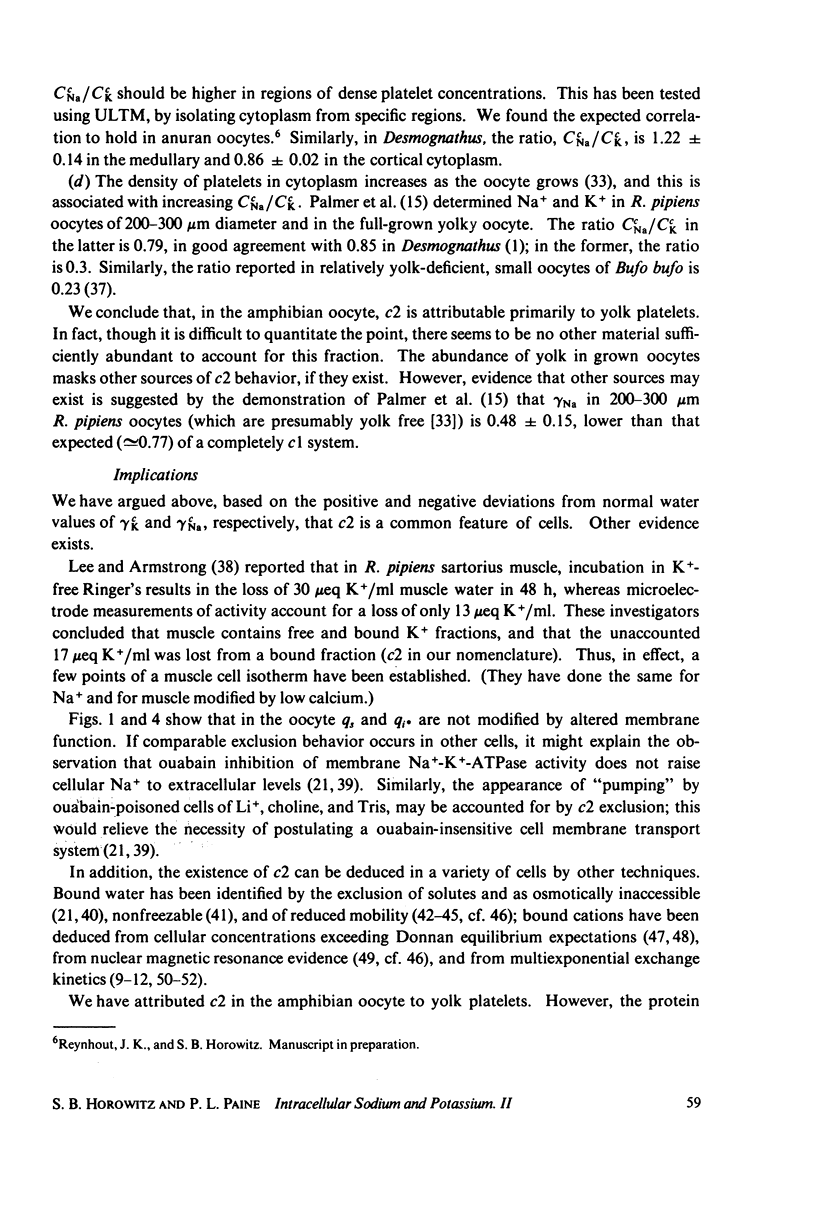
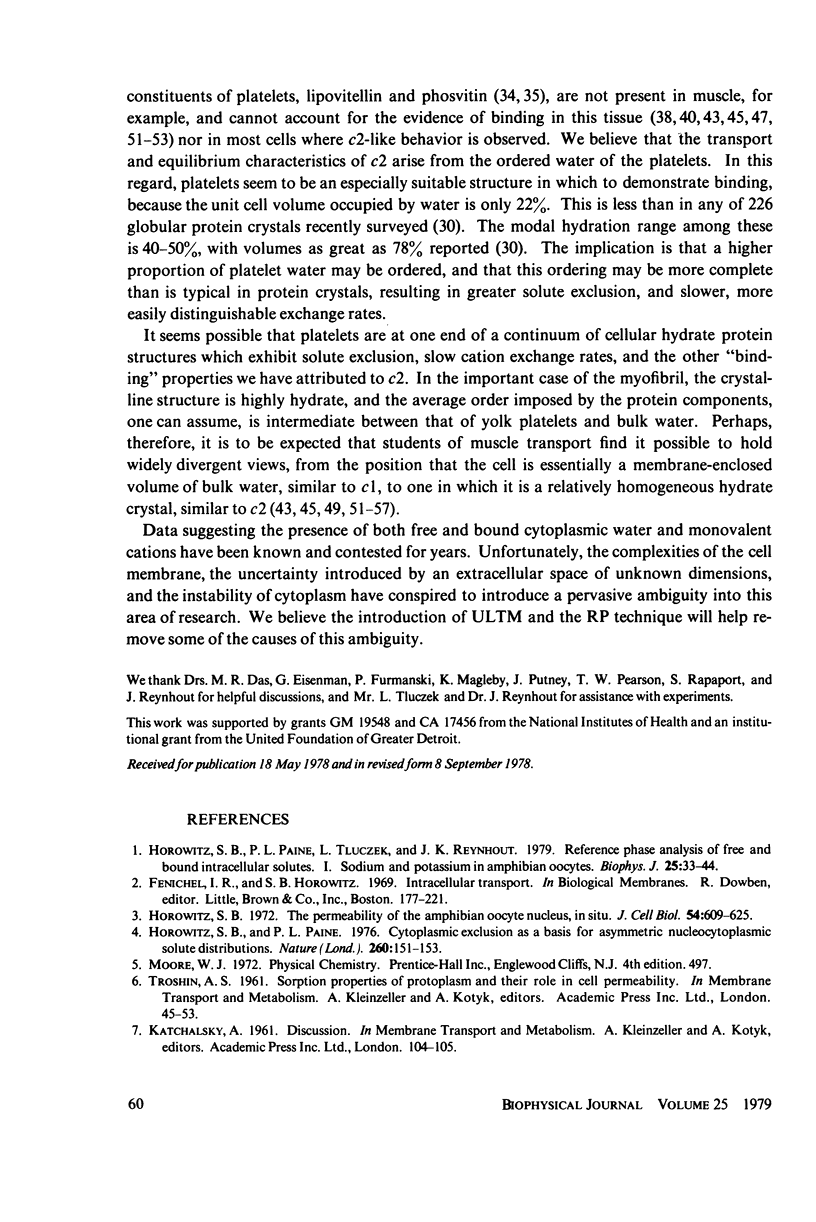
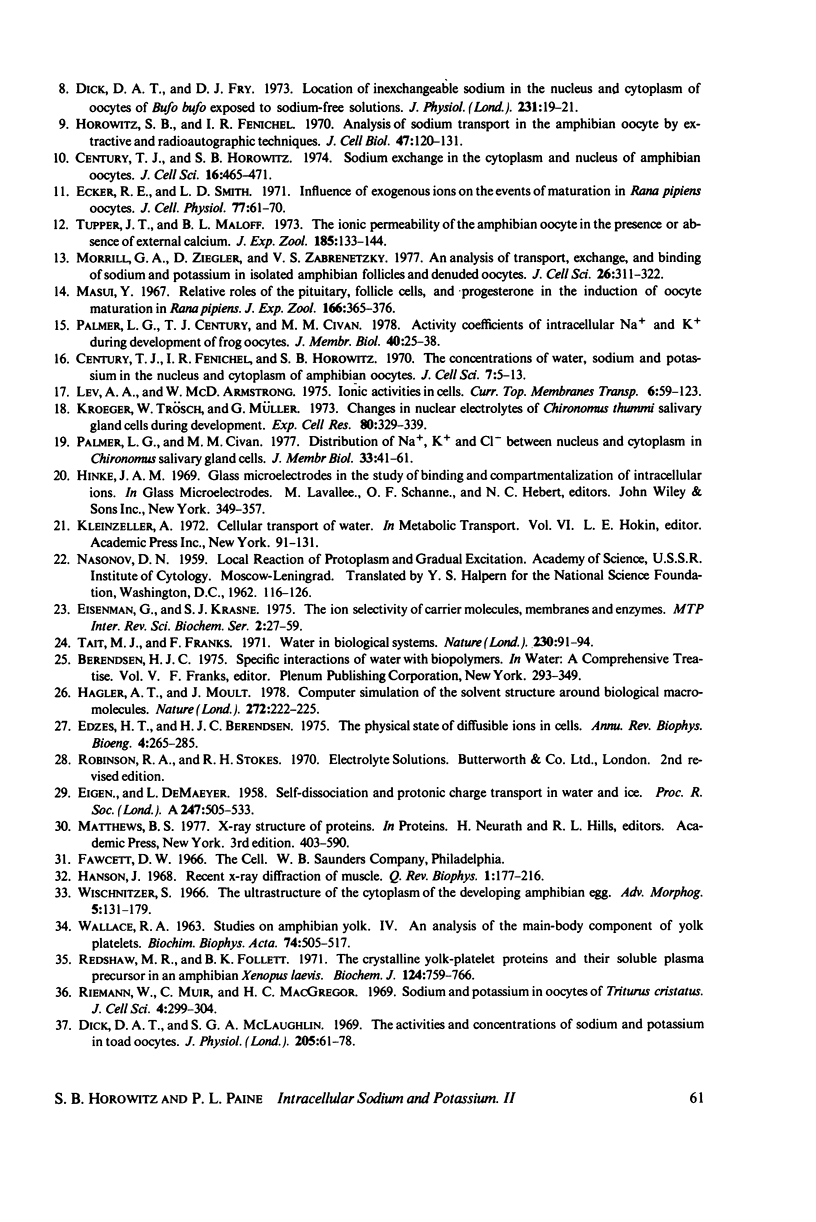
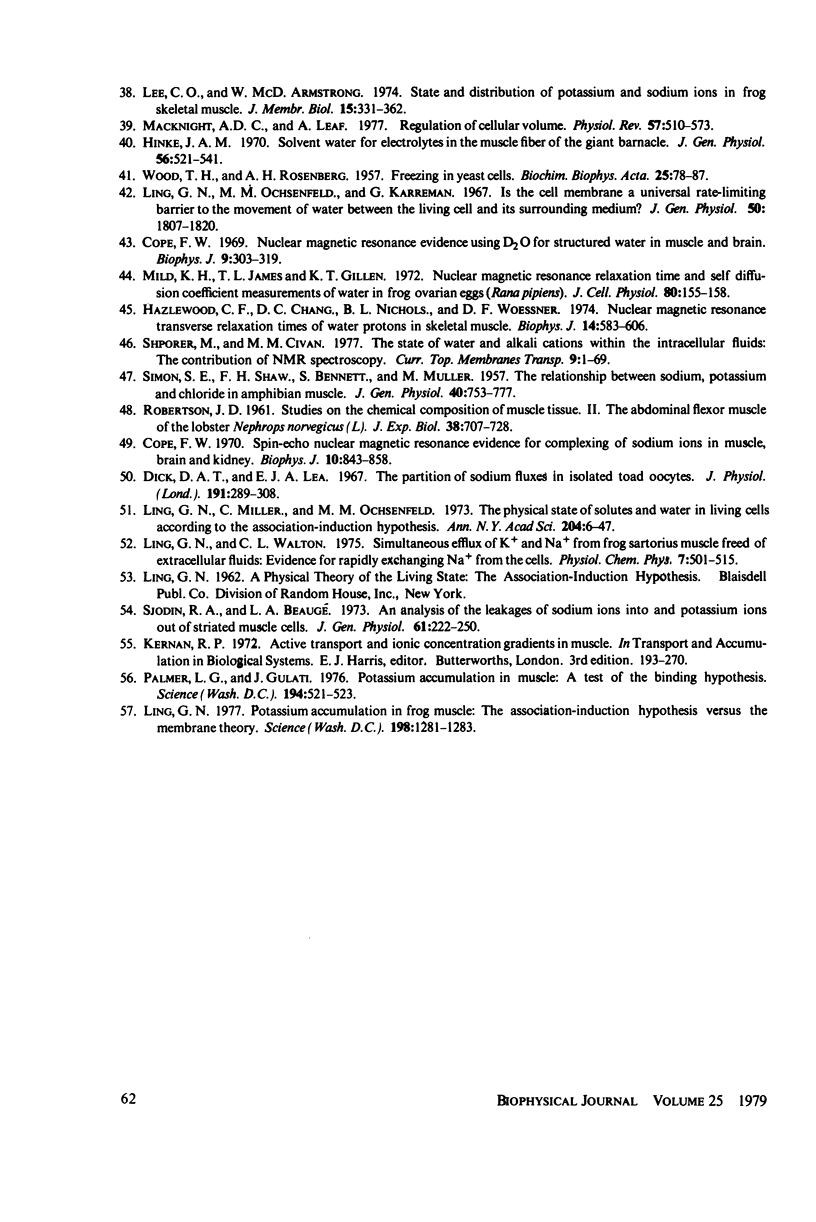
Selected References
These references are in PubMed. This may not be the complete list of references from this article.
- Century T. J., Fenichel I. R., Horowitz S. B. The concentrations of water, sodium and potassium in the nucleus and cytoplasm of amphibian oocytes. J Cell Sci. 1970 Jul;7(1):5–13. doi: 10.1242/jcs.7.1.5. [DOI] [PubMed] [Google Scholar]
- Century T. J., Horowitz S. B. Sodium exchange in the cytoplasm and nucleus of amphibian oocytes. J Cell Sci. 1974 Nov;16(2):465–471. doi: 10.1242/jcs.16.2.465. [DOI] [PubMed] [Google Scholar]
- Cope F. W. Nuclear magnetic resonance evidence using D2O for structured water in muscle and brain. Biophys J. 1969 Mar;9(3):303–319. doi: 10.1016/S0006-3495(69)86388-5. [DOI] [PMC free article] [PubMed] [Google Scholar]
- Cope F. W. Spin-echo nuclear magnetic resonance evidence for complexing of sodium ions in muscle, brain, and kidney. Biophys J. 1970 Sep;10(9):843–858. doi: 10.1016/S0006-3495(70)86339-1. [DOI] [PMC free article] [PubMed] [Google Scholar]
- Dick D. A., Lea E. J. Partition of sodium fluxes in isolated toad oocytes. J Physiol. 1967 Jul;191(2):289–308. doi: 10.1113/jphysiol.1967.sp008251. [DOI] [PMC free article] [PubMed] [Google Scholar]
- Dick D. A., McLaughlin S. G. The activities and concentrations of sodium and potassium in toad oocytes. J Physiol. 1969 Nov;205(1):61–78. doi: 10.1113/jphysiol.1969.sp008951. [DOI] [PMC free article] [PubMed] [Google Scholar]
- Ecker R. E., Smith L. D. Influence of exogenous ions on the events of maturation in Rana pipiens oocytes. J Cell Physiol. 1971 Feb;77(1):61–70. doi: 10.1002/jcp.1040770108. [DOI] [PubMed] [Google Scholar]
- Edzes H. T., Berendsen H. J. The physical state of diffusible ions in cells. Annu Rev Biophys Bioeng. 1975;4(00):265–285. doi: 10.1146/annurev.bb.04.060175.001405. [DOI] [PubMed] [Google Scholar]
- Hagler A. T., Moult J. Computer simulation of the solvent structure around biological macromolecules. Nature. 1978 Mar 16;272(5650):222–226. doi: 10.1038/272222a0. [DOI] [PubMed] [Google Scholar]
- Hanson J. Recent x-ray diffraction studies of muscle. Q Rev Biophys. 1968 Jun;1(2):177–216. doi: 10.1017/s0033583500000536. [DOI] [PubMed] [Google Scholar]
- Hazlewood C. F., Chang D. C., Nichols B. L., Woessner D. E. Nuclear magnetic resonance transverse relaxation times of water protons in skeletal muscle. Biophys J. 1974 Aug;14(8):583–606. doi: 10.1016/S0006-3495(74)85937-0. [DOI] [PMC free article] [PubMed] [Google Scholar]
- Hinke J. A. Solvent water for electrolytes in the muscle fiber of the giant barnacle. J Gen Physiol. 1970 Oct;56(4):521–541. doi: 10.1085/jgp.56.4.521. [DOI] [PMC free article] [PubMed] [Google Scholar]
- Horowitz S. B., Fenichel I. R. Analysis of sodium transport in the amphibian oocyte by extractive and radioautographic techniques. J Cell Biol. 1970 Oct;47(1):120–131. doi: 10.1083/jcb.47.1.120. [DOI] [PMC free article] [PubMed] [Google Scholar]
- Horowitz S. B., Paine P. L. Cytoplasmic exclusion as a basis for asymmetric nucleocytoplasmic solute distributions. Nature. 1976 Mar 11;260(5547):151–153. doi: 10.1038/260151a0. [DOI] [PubMed] [Google Scholar]
- Horowitz S. B., Paine P. L., Tluczek L., Reynhout J. K. Reference phase analysis of free and bound intracellular solutes. I. Sodium and potassium in amphibian oocytes. Biophys J. 1979 Jan;25(1):33–44. doi: 10.1016/S0006-3495(79)85276-5. [DOI] [PMC free article] [PubMed] [Google Scholar]
- Horowitz S. B. The permeability of the amphibian oocyte nucleus, in situ. J Cell Biol. 1972 Sep;54(3):609–625. doi: 10.1083/jcb.54.3.609. [DOI] [PMC free article] [PubMed] [Google Scholar]
- Kroeger H., Trösch W., Müller G. Changes in nuclear electrolytes of Chironomus thummi salivary gland cells during development. Exp Cell Res. 1973 Aug;80(2):329–339. doi: 10.1016/0014-4827(73)90304-2. [DOI] [PubMed] [Google Scholar]
- Lee C. O., Armstrong W. M. State and distribution of potassium and sodium ions in frog skeletal muscle. J Membr Biol. 1974;15(4):331–362. doi: 10.1007/BF01870094. [DOI] [PubMed] [Google Scholar]
- Ling G. N., Ochsenfeld M. M., Karreman G. Is the cell membrane a universal rate-limiting barrier to the movement of water between the living cell and its surrounding medium? J Gen Physiol. 1967 Jul;50(6):1807–1820. doi: 10.1085/jgp.50.6.1807. [DOI] [PMC free article] [PubMed] [Google Scholar]
- Ling G. N. Potassium accumulation frog muscle: the association-induction hypothesis versus the membrane theory. Science. 1977 Dec 23;198(4323):1281–1284. doi: 10.1126/science.929204. [DOI] [PubMed] [Google Scholar]
- Ling G. N., Walton C. L. Simultaneous efflux of K+ and Na+ from frog sartorius muscle freed of extracellular fluids: evidence for rapidly exchanging Na+ from the cells. Physiol Chem Phys. 1975;7(6):501–515. [PubMed] [Google Scholar]
- Macknight A. D., Leaf A. Regulation of cellular volume. Physiol Rev. 1977 Jul;57(3):510–573. doi: 10.1152/physrev.1977.57.3.510. [DOI] [PubMed] [Google Scholar]
- Masui Y. Relative roles of the pituitary, follicle cells, and progesterone in the induction of oocyte maturation in Rana pipiens. J Exp Zool. 1967 Dec;166(3):365–375. doi: 10.1002/jez.1401660309. [DOI] [PubMed] [Google Scholar]
- Mild K. H., James T. L., Gillen K. T. Nuclear magnetic resonance relaxation time and self-diffusion coefficient measurements of water in frog ovarian eggs (Rana pipiens). J Cell Physiol. 1972 Aug;80(1):155–158. doi: 10.1002/jcp.1040800117. [DOI] [PubMed] [Google Scholar]
- Morrill G. A., Ziegler D., Zabrenetzky V. S. An analysis of transport, exchange, and binding of sodium and potassium in isolated amphibian follicles and denuded oocytes. J Cell Sci. 1977 Aug;26:311–322. doi: 10.1242/jcs.26.1.311. [DOI] [PubMed] [Google Scholar]
- Palmer L. G., Century T. J., Civan M. M. Activity coefficients of intracellular Na+ and K+ during development of frog oocytes. J Membr Biol. 1978 Apr 20;40(1):25–38. doi: 10.1007/BF01909737. [DOI] [PubMed] [Google Scholar]
- Palmer L. G., Civan M. M. Distribution of Na+, K+ and Cl- between nucleus and cytoplasm in Chironomus salivary gland cells. J Membr Biol. 1977 May 6;33(1-2):41–61. doi: 10.1007/BF01869511. [DOI] [PubMed] [Google Scholar]
- Palmer L. G., Gulati J. Potassium accumulation in muscle: a test of the binding hypothesis. Science. 1976 Oct 29;194(4264):521–523. doi: 10.1126/science.1085986. [DOI] [PubMed] [Google Scholar]
- Redshaw M. R., Follett B. K. The crystalline yolk-platelet proteins and their soluble plasma precursor in an amphibian, Xenopus laevis. Biochem J. 1971 Oct;124(4):759–766. doi: 10.1042/bj1240759. [DOI] [PMC free article] [PubMed] [Google Scholar]
- Riemann W., Muir C., Macgregor H. C. Sodium and potassium in oocytes of Triturus cristatus. J Cell Sci. 1969 Mar;4(2):299–304. doi: 10.1242/jcs.4.2.299. [DOI] [PubMed] [Google Scholar]
- SIMON S. E., SHAW F. H., BENNETT S., MULLER M. The relationship between sodium, potassium, and chloride in amphibian muscle. J Gen Physiol. 1957 May 20;40(5):753–777. doi: 10.1085/jgp.40.5.753. [DOI] [PMC free article] [PubMed] [Google Scholar]
- Sjodin R. A., Beaugé L. A. An analysis of the leakages of sodium ions into and potassium ions out of striated muscle cells. J Gen Physiol. 1973 Feb;61(2):222–250. doi: 10.1085/jgp.61.2.222. [DOI] [PMC free article] [PubMed] [Google Scholar]
- Tait M. J., Franks F. Water in biological systems. Nature. 1971 Mar 12;230(5289):91–94. doi: 10.1038/230091a0. [DOI] [PubMed] [Google Scholar]
- Tupper J. T., Maloff B. L. The ionic permeability of the amphibian oocyte in the presence or absence of external calcium. J Exp Zool. 1973 Jul;185(1):133–144. doi: 10.1002/jez.1401850113. [DOI] [PubMed] [Google Scholar]
- WALLACE R. A. STUDIES ON AMPHIBIAN YOLK. IV. AN ANALYSIS OF THE MAIN-BODY COMPONENT OF YOLK PLATELETS. Biochim Biophys Acta. 1963 Aug 13;74:505–518. doi: 10.1016/0006-3002(63)91393-3. [DOI] [PubMed] [Google Scholar]
- WOOD T. H., ROSENBERG A. M. Freezing in yeast cells. Biochim Biophys Acta. 1957 Jul;25(1):78–87. doi: 10.1016/0006-3002(57)90421-3. [DOI] [PubMed] [Google Scholar]
- Wischnitzer S. The ultrastructure of the cytoplasm of the developing amphibian egg. Adv Morphog. 1966;5:131–179. doi: 10.1016/b978-1-4831-9952-8.50008-0. [DOI] [PubMed] [Google Scholar]