Abstract
Isolated rat and mouse extensor digitorum longus (EDL) and soleus muscles were studied under isometric and isotonic conditions at temperatures from approximately 8 degrees -38 degrees C. The rate constant for the exponential rise of tension during an isometric tetanus had a Q10 of approximately 2.5 for all muscles (corresponding to an enthalpy of activation, delta H = 66 kJ/mol, if the rate was determined by a single chemical reaction). The half-contraction time, contraction time, and maximum rate of rise for tension in an isometric twitch and the maximum shortening velocity in an isotonic contraction all had a similar temperature dependence (i.e., delta H approximately 66 kJ/mol). The Mg++ ATPase rates of myofibrils prepared from rat EDL and soleus muscles had a steeper temperature dependence (delta H = 130 kJ/mol), but absolute rates at 20 degrees C were lower than the rate of rise of tension. This suggests that the Mg++ ATPase cycle rate is not limiting for force generation. A substantial fraction of cross-bridges may exist in a resting state that converts to the force-producing state at a rate faster than required to complete the cycle and repopulate the resting state. The temperature dependence for the rate constant of the exponential decay of tension during an isometric twitch or short tetanus (and the half-fall time of a twitch) had a break point at approximately 20 degrees C, with apparent enthalpy values of delta H = 117 kJ/mol below 20 degrees C and delta H = 70 kJ/mol above 20 degrees C. The break point and the values of delta H at high and low temperatures agree closely with published values for the delta H of the sarcoplasmic reticulum (SR) Ca++ ATPase. Thus, the temperature dependence for the relaxation rate of a twitch or a short tetanus is consistent with that for the reabsorption rate of Ca++ into the SR.
Full text
PDF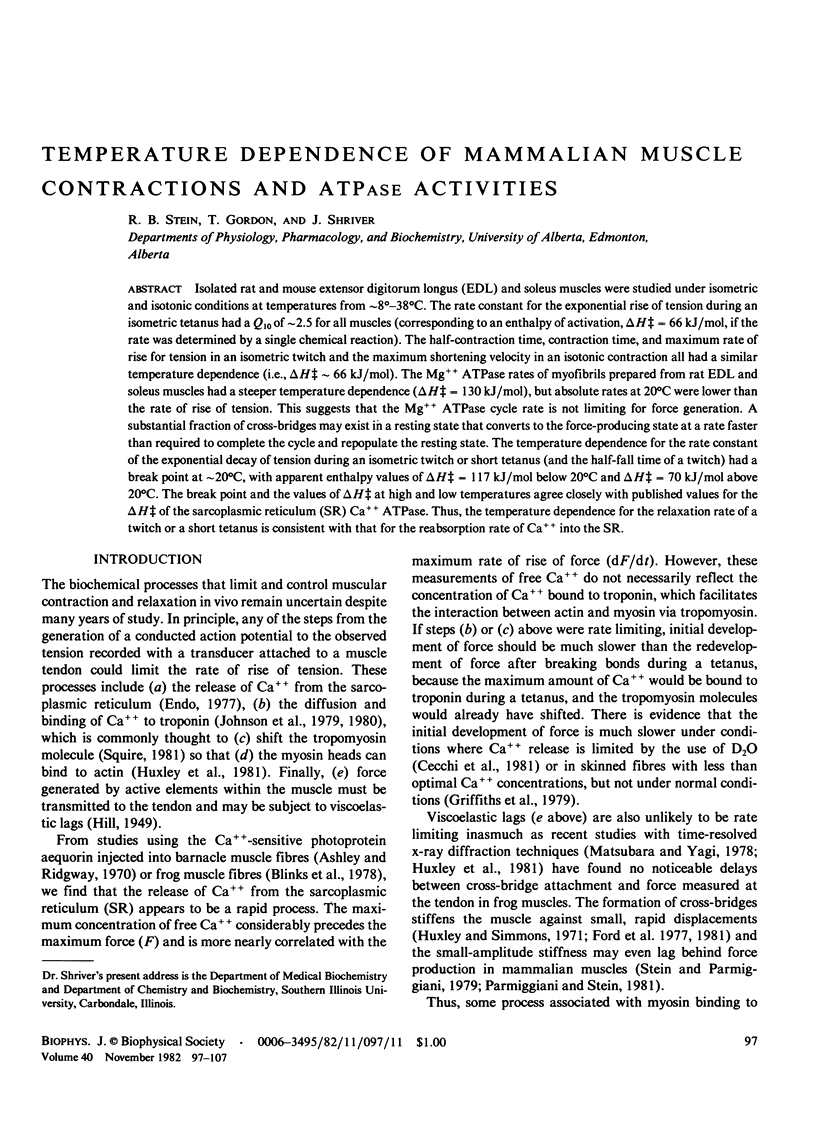
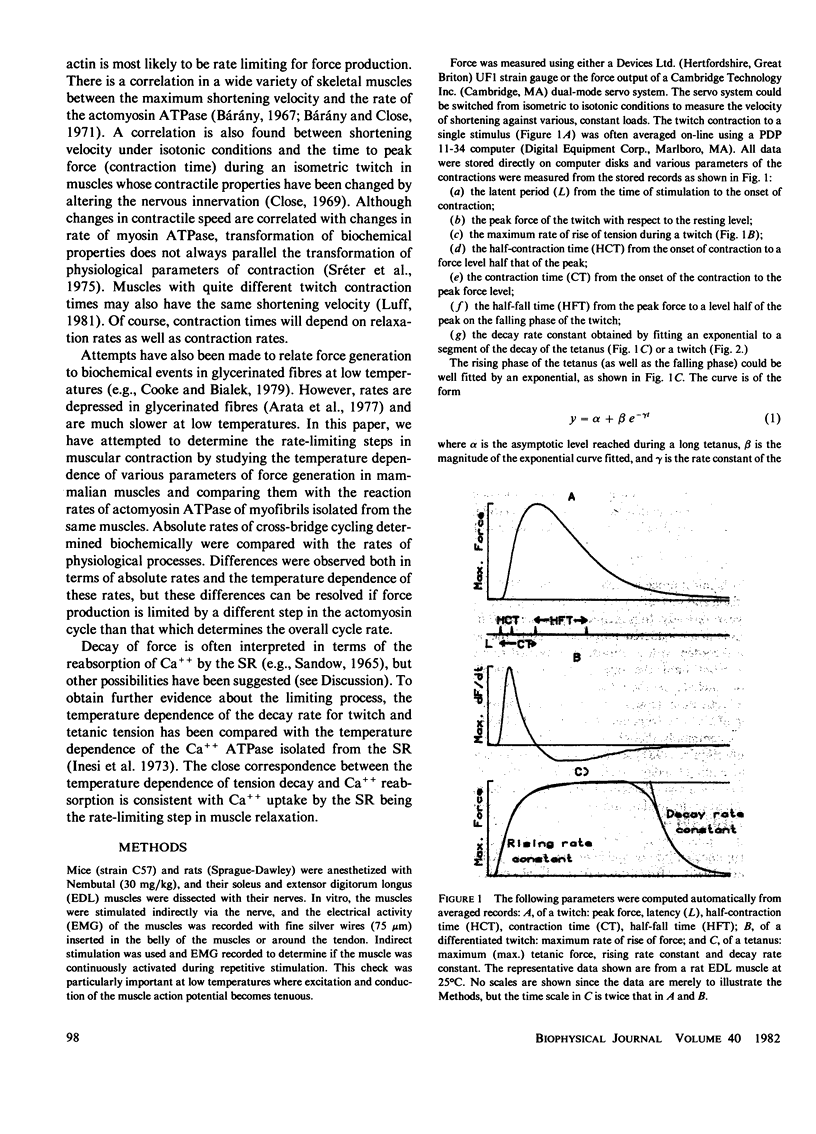
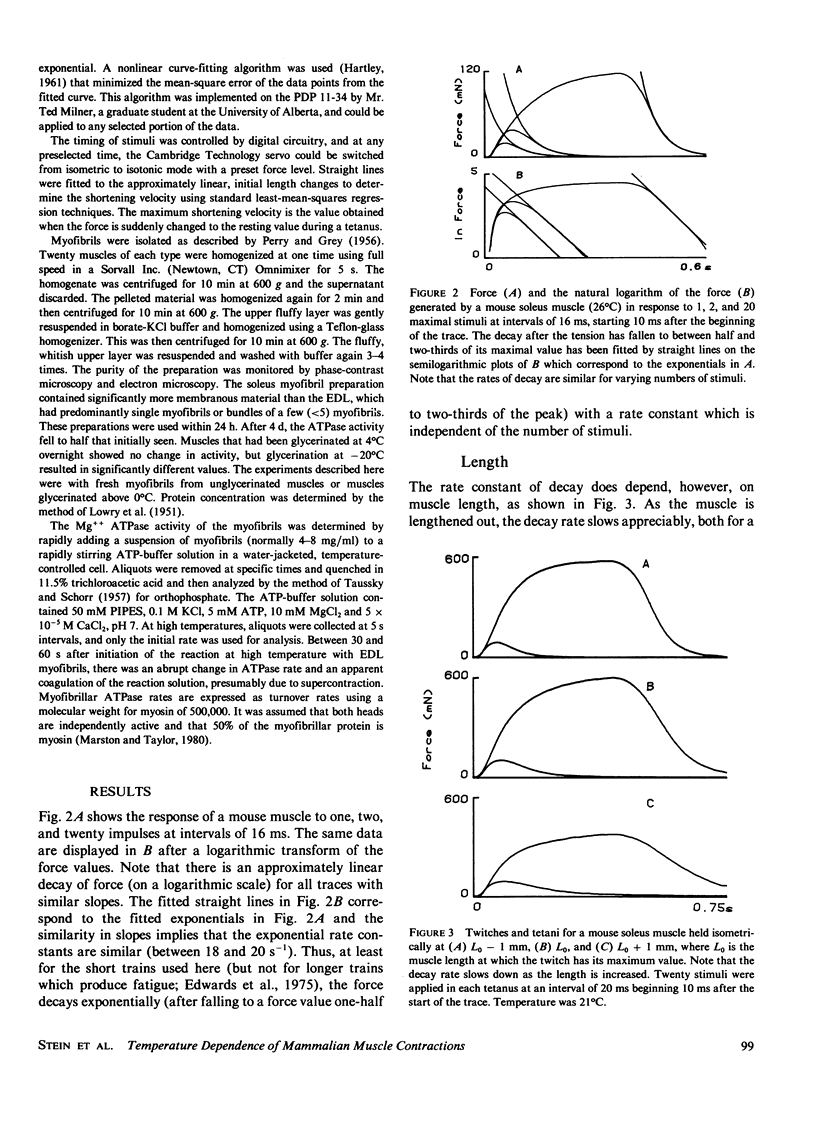
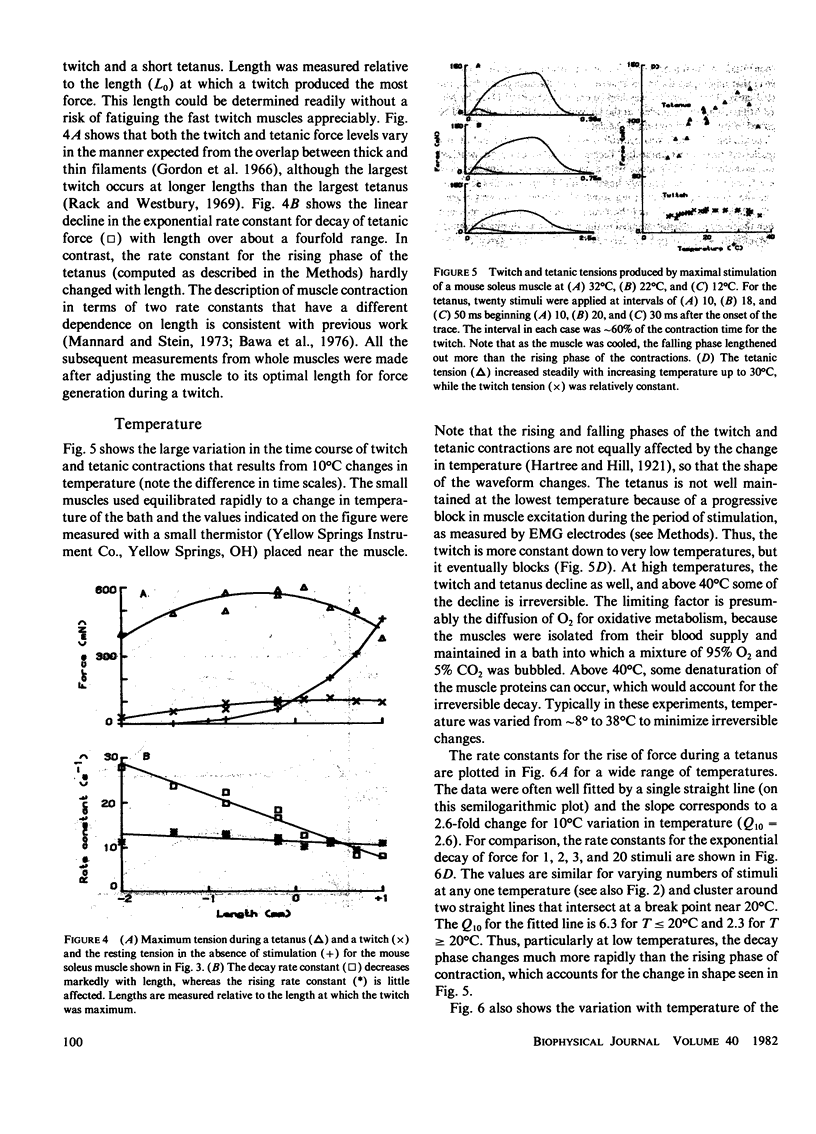
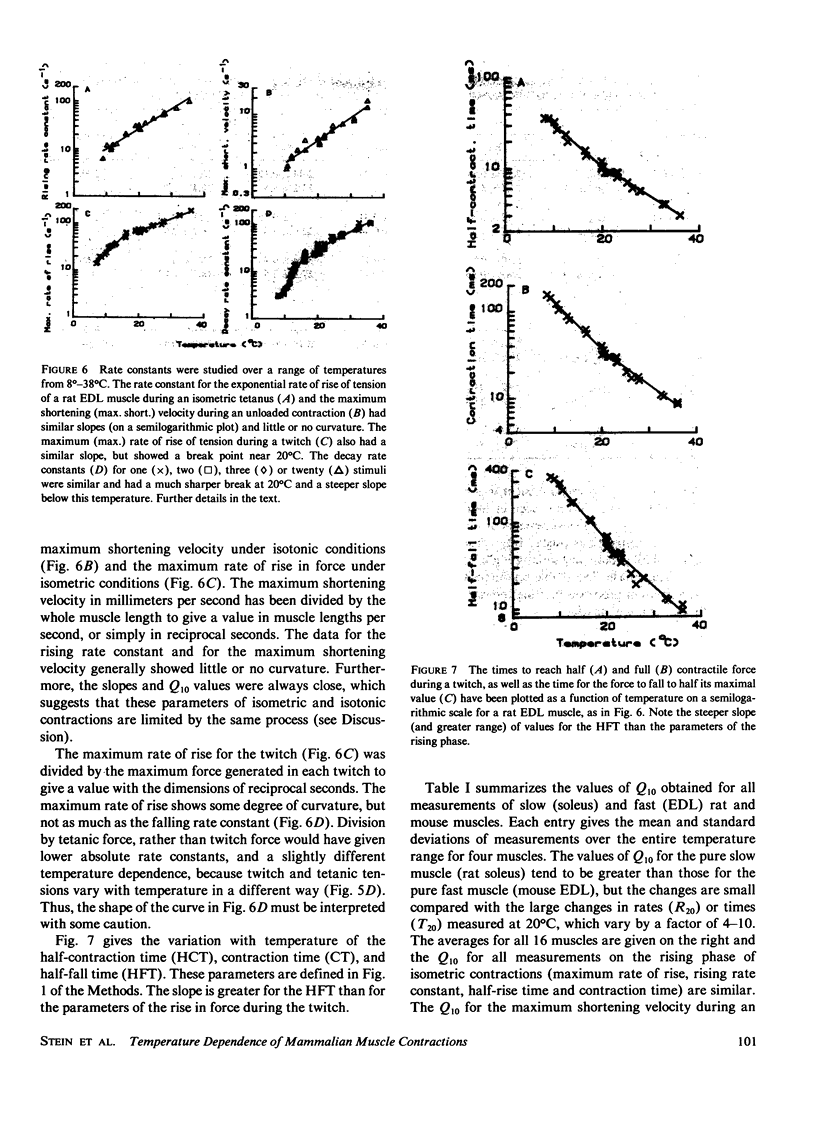
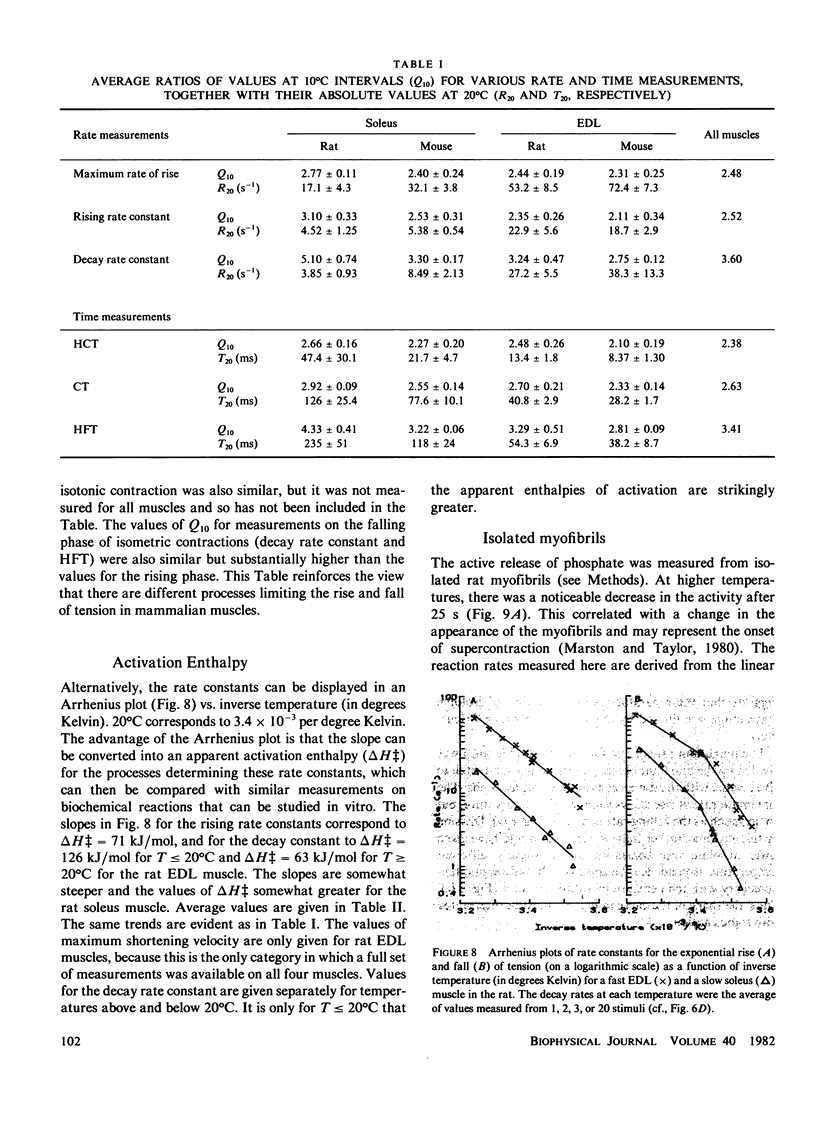
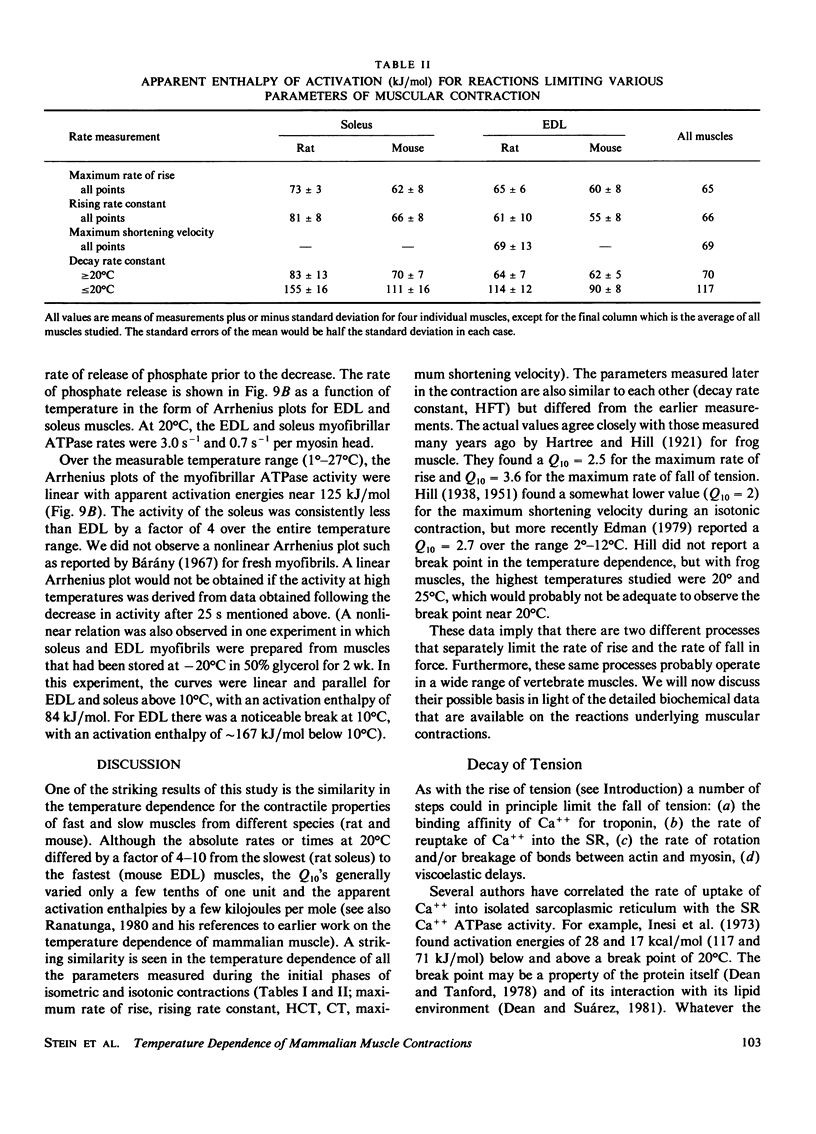
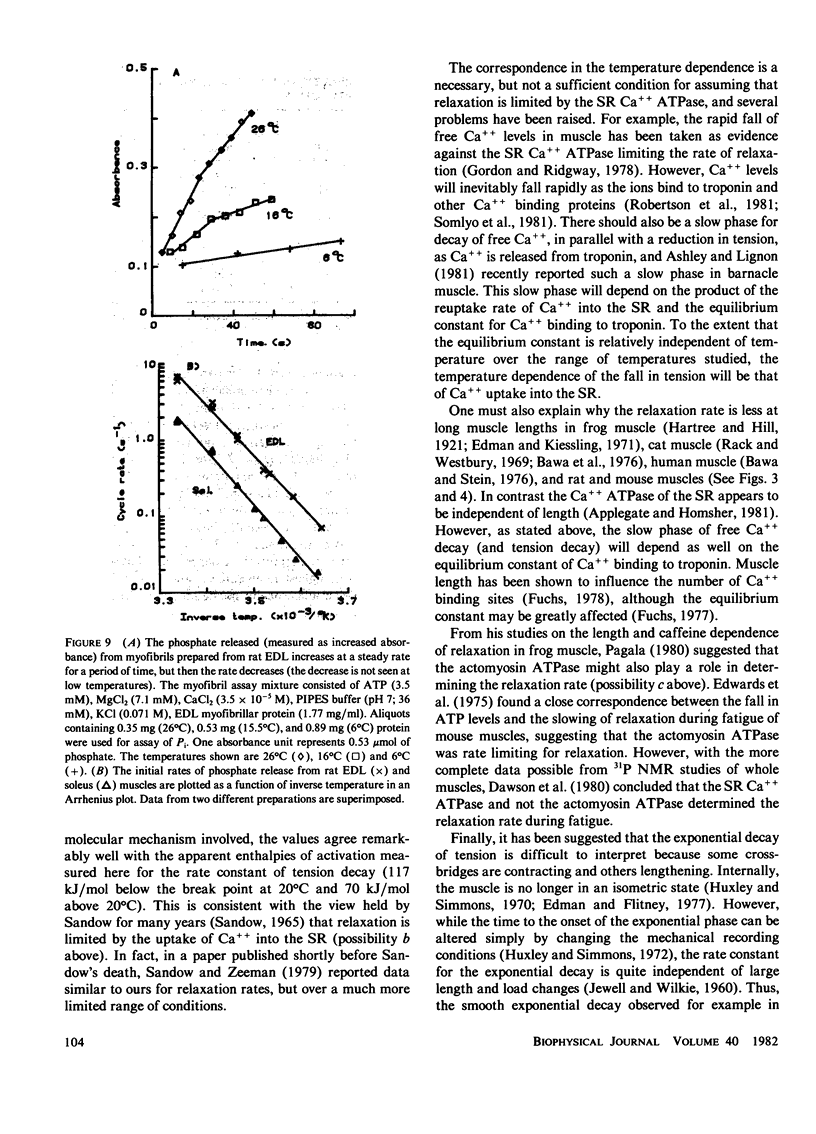
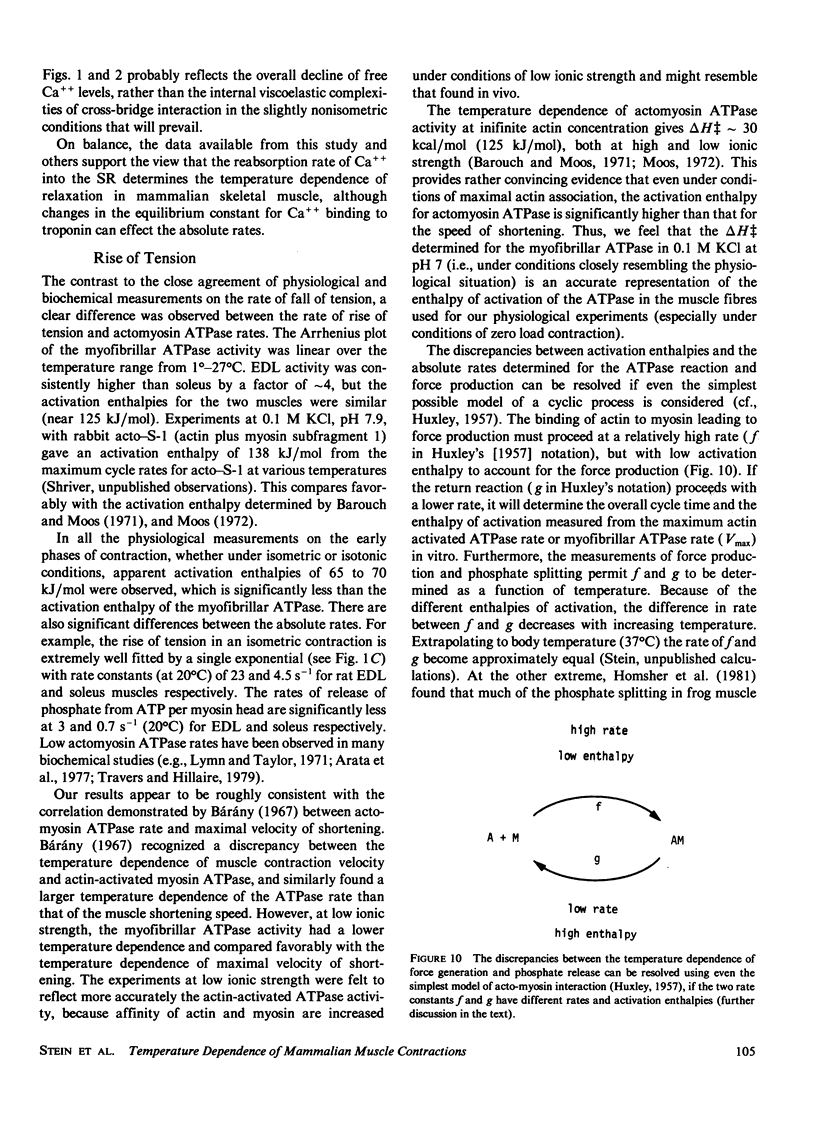
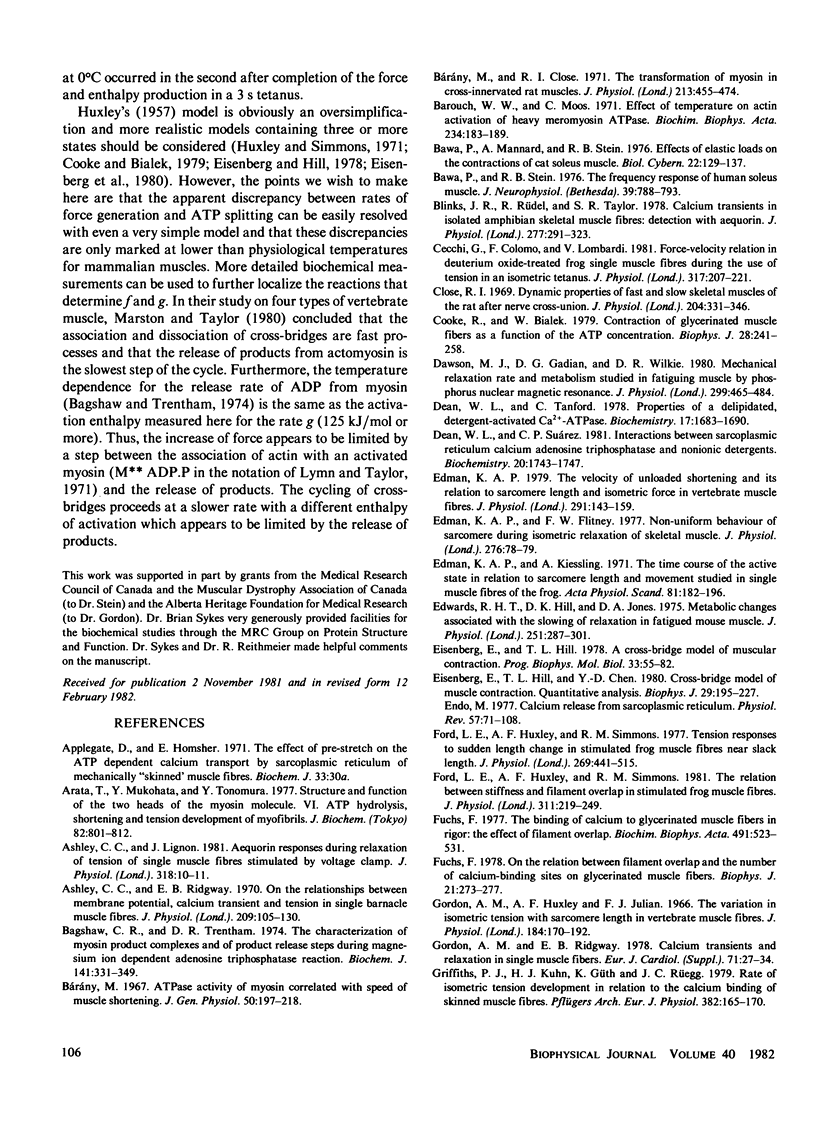
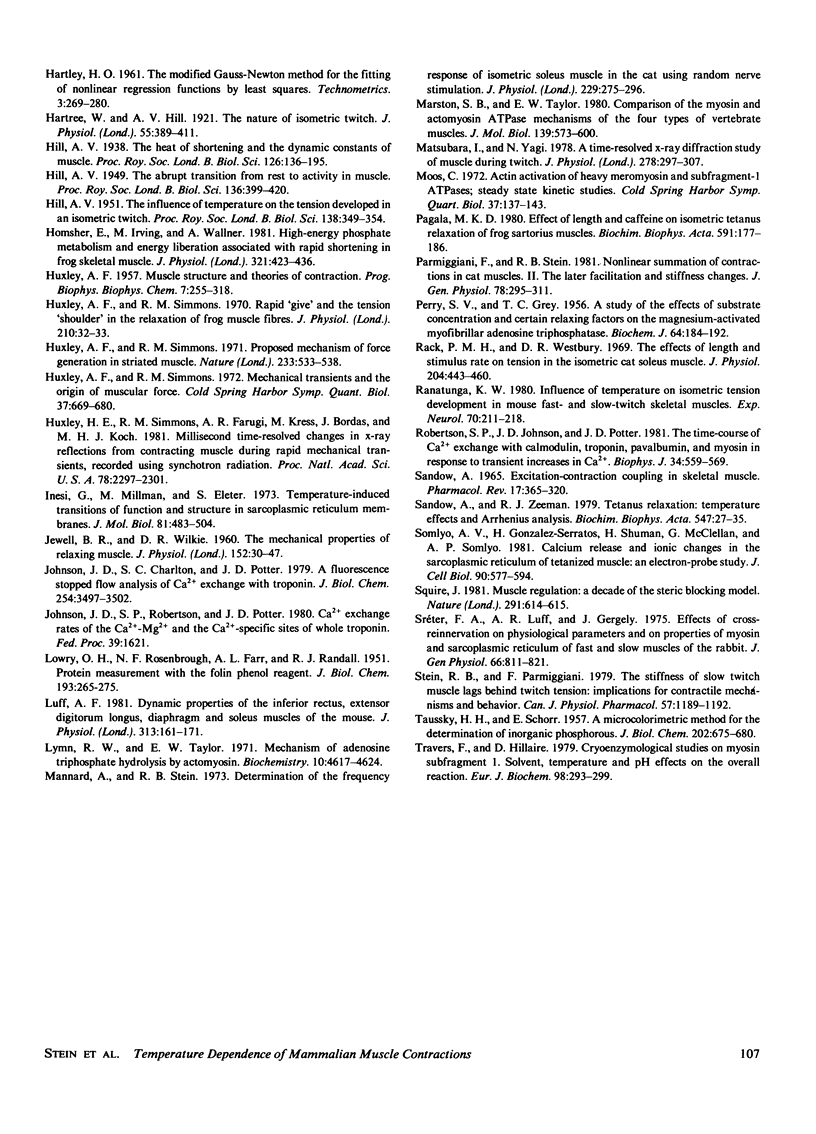
Selected References
These references are in PubMed. This may not be the complete list of references from this article.
- Arata T., Mukohata Y., Tonomura Y. Structure and function of the two heads of the myosin molecule. VI. ATP hydrolysis, shortening, and tension development of myofibrils. J Biochem. 1977 Sep;82(3):801–812. doi: 10.1093/oxfordjournals.jbchem.a131756. [DOI] [PubMed] [Google Scholar]
- Ashley C. C., Ridgway E. B. On the relationships between membrane potential, calcium transient and tension in single barnacle muscle fibres. J Physiol. 1970 Jul;209(1):105–130. doi: 10.1113/jphysiol.1970.sp009158. [DOI] [PMC free article] [PubMed] [Google Scholar]
- Bagshaw C. R., Trentham D. R. The characterization of myosin-product complexes and of product-release steps during the magnesium ion-dependent adenosine triphosphatase reaction. Biochem J. 1974 Aug;141(2):331–349. doi: 10.1042/bj1410331. [DOI] [PMC free article] [PubMed] [Google Scholar]
- Barouch W. W., Moos C. Effect of temperature on actin activation of heavy meromyosin ATPase. Biochim Biophys Acta. 1971 May 11;234(2):183–189. doi: 10.1016/0005-2728(71)90073-9. [DOI] [PubMed] [Google Scholar]
- Bawa P., Mannard A., Stein R. B. Effects of elastic loads on the contractions of cat muscles. Biol Cybern. 1976;22(3):129–137. doi: 10.1007/BF00365523. [DOI] [PubMed] [Google Scholar]
- Bawa P., Stein R. B. Frequency response of human soleus muscle. J Neurophysiol. 1976 Jul;39(4):788–793. doi: 10.1152/jn.1976.39.4.788. [DOI] [PubMed] [Google Scholar]
- Blinks J. R., Rüdel R., Taylor S. R. Calcium transients in isolated amphibian skeletal muscle fibres: detection with aequorin. J Physiol. 1978 Apr;277:291–323. doi: 10.1113/jphysiol.1978.sp012273. [DOI] [PMC free article] [PubMed] [Google Scholar]
- Bárány M. ATPase activity of myosin correlated with speed of muscle shortening. J Gen Physiol. 1967 Jul;50(6 Suppl):197–218. doi: 10.1085/jgp.50.6.197. [DOI] [PMC free article] [PubMed] [Google Scholar]
- Bárány M., Close R. I. The transformation of myosin in cross-innervated rat muscles. J Physiol. 1971 Mar;213(2):455–474. doi: 10.1113/jphysiol.1971.sp009393. [DOI] [PMC free article] [PubMed] [Google Scholar]
- Cecchi G., Colomo F., Lombardi V. Force-velocity relation in deuterium oxide-treated frog single muscle fibres during the rise of tension in an isometric tetanus. J Physiol. 1981 Aug;317:207–221. doi: 10.1113/jphysiol.1981.sp013821. [DOI] [PMC free article] [PubMed] [Google Scholar]
- Close R. Dynamic properties of fast and slow skeletal muscles of the rat after nerve cross-union. J Physiol. 1969 Oct;204(2):331–346. doi: 10.1113/jphysiol.1969.sp008916. [DOI] [PMC free article] [PubMed] [Google Scholar]
- Cooke R., Bialek W. Contraction of glycerinated muscle fibers as a function of the ATP concentration. Biophys J. 1979 Nov;28(2):241–258. doi: 10.1016/S0006-3495(79)85174-7. [DOI] [PMC free article] [PubMed] [Google Scholar]
- Dawson M. J., Gadian D. G., Wilkie D. R. Mechanical relaxation rate and metabolism studied in fatiguing muscle by phosphorus nuclear magnetic resonance. J Physiol. 1980 Feb;299:465–484. doi: 10.1113/jphysiol.1980.sp013137. [DOI] [PMC free article] [PubMed] [Google Scholar]
- Dean W. L., Suárez C. P. Interactions between sarcoplasmic reticulum calcium adenosintriphosphatase and nonionic detergents. Biochemistry. 1981 Mar 31;20(7):1743–1747. doi: 10.1021/bi00510a006. [DOI] [PubMed] [Google Scholar]
- Dean W. L., Tanford C. Properties of a delipidated, detergent-activated Ca2+--ATPase. Biochemistry. 1978 May 2;17(9):1683–1690. doi: 10.1021/bi00602a016. [DOI] [PubMed] [Google Scholar]
- Edman K. A., Kiessling A. The time course of the active state in relation to sarcomere length and movement studied in single skeletal muscle fibres of the frog. Acta Physiol Scand. 1971 Feb;81(2):182–196. doi: 10.1111/j.1748-1716.1971.tb04891.x. [DOI] [PubMed] [Google Scholar]
- Edman K. A. The velocity of unloaded shortening and its relation to sarcomere length and isometric force in vertebrate muscle fibres. J Physiol. 1979 Jun;291:143–159. doi: 10.1113/jphysiol.1979.sp012804. [DOI] [PMC free article] [PubMed] [Google Scholar]
- Edwards R. H., Hill D. K., Jones D. A. Metabolic changes associated with the slowing of relaxation in fatigued mouse muscle. J Physiol. 1975 Oct;251(2):287–301. doi: 10.1113/jphysiol.1975.sp011093. [DOI] [PMC free article] [PubMed] [Google Scholar]
- Eisenberg E., Hill T. L. A cross-bridge model of muscle contraction. Prog Biophys Mol Biol. 1978;33(1):55–82. doi: 10.1016/0079-6107(79)90025-7. [DOI] [PubMed] [Google Scholar]
- Eisenberg E., Hill T. L., Chen Y. Cross-bridge model of muscle contraction. Quantitative analysis. Biophys J. 1980 Feb;29(2):195–227. doi: 10.1016/S0006-3495(80)85126-5. [DOI] [PMC free article] [PubMed] [Google Scholar]
- Endo M. Calcium release from the sarcoplasmic reticulum. Physiol Rev. 1977 Jan;57(1):71–108. doi: 10.1152/physrev.1977.57.1.71. [DOI] [PubMed] [Google Scholar]
- Ford L. E., Huxley A. F., Simmons R. M. Tension responses to sudden length change in stimulated frog muscle fibres near slack length. J Physiol. 1977 Jul;269(2):441–515. doi: 10.1113/jphysiol.1977.sp011911. [DOI] [PMC free article] [PubMed] [Google Scholar]
- Ford L. E., Huxley A. F., Simmons R. M. The relation between stiffness and filament overlap in stimulated frog muscle fibres. J Physiol. 1981 Feb;311:219–249. doi: 10.1113/jphysiol.1981.sp013582. [DOI] [PMC free article] [PubMed] [Google Scholar]
- Fuchs F. On the relation between filament overlap and the number of calcium-binding sites on glycerinated muscle fibers. Biophys J. 1978 Mar;21(3):273–277. doi: 10.1016/S0006-3495(78)85524-6. [DOI] [PMC free article] [PubMed] [Google Scholar]
- Fuchs F. The binding of calcium to glycerinated muscle fibers in rigor. The effect of filament overlap. Biochim Biophys Acta. 1977 Apr 25;491(2):523–531. doi: 10.1016/0005-2795(77)90297-5. [DOI] [PubMed] [Google Scholar]
- GREY T. C., PERRY S. V. A study of the effects of substrate concentration and certain relaxing factors on the magnesium-activated myofibrillar adenosine triphosphatase. Biochem J. 1956 Sep;64(1):184–192. doi: 10.1042/bj0640184. [DOI] [PMC free article] [PubMed] [Google Scholar]
- Gordon A. M., Huxley A. F., Julian F. J. The variation in isometric tension with sarcomere length in vertebrate muscle fibres. J Physiol. 1966 May;184(1):170–192. doi: 10.1113/jphysiol.1966.sp007909. [DOI] [PMC free article] [PubMed] [Google Scholar]
- Gordon A. M., Ridgway E. B. Calcium transients and relaxation in single muscle fibers. Eur J Cardiol. 1978 Jun;7 (Suppl):27–34. [PubMed] [Google Scholar]
- Griffiths P. J., Kuhn H. J., Güth K., Rüegg J. C. Rate of isometric tension development in relation to calcium binding of skinned muscle fibres. Pflugers Arch. 1979 Nov;382(2):165–170. doi: 10.1007/BF00584218. [DOI] [PubMed] [Google Scholar]
- HILL A. V. The abrupt transition from rest to activity in muscle. Proc R Soc Lond B Biol Sci. 1949 Oct;136(884):399–420. doi: 10.1098/rspb.1949.0033. [DOI] [PubMed] [Google Scholar]
- HUXLEY A. F. Muscle structure and theories of contraction. Prog Biophys Biophys Chem. 1957;7:255–318. [PubMed] [Google Scholar]
- Hartree W., Hill A. V. The nature of the isometric twitch. J Physiol. 1921 Nov 18;55(5-6):389–411. doi: 10.1113/jphysiol.1921.sp001984. [DOI] [PMC free article] [PubMed] [Google Scholar]
- Homsher E., Irving M., Wallner A. High-energy phosphate metabolism and energy liberation associated with rapid shortening in frog skeletal muscle. J Physiol. 1981 Dec;321:423–436. doi: 10.1113/jphysiol.1981.sp013994. [DOI] [PMC free article] [PubMed] [Google Scholar]
- Huxley A. F., Simmons R. M. Proposed mechanism of force generation in striated muscle. Nature. 1971 Oct 22;233(5321):533–538. doi: 10.1038/233533a0. [DOI] [PubMed] [Google Scholar]
- Huxley H. E., Simmons R. M., Faruqi A. R., Kress M., Bordas J., Koch M. H. Millisecond time-resolved changes in x-ray reflections from contracting muscle during rapid mechanical transients, recorded using synchrotron radiation. Proc Natl Acad Sci U S A. 1981 Apr;78(4):2297–2301. doi: 10.1073/pnas.78.4.2297. [DOI] [PMC free article] [PubMed] [Google Scholar]
- Inesi G., Millman M., Eletr S. Temperature-induced transitions of function and structure in sarcoplasmic reticulum membranes. J Mol Biol. 1973 Dec 25;81(4):483–504. doi: 10.1016/0022-2836(73)90518-4. [DOI] [PubMed] [Google Scholar]
- JEWELL B. R., WILKIE D. R. The mechanical properties of relaxing muscle. J Physiol. 1960 Jun;152:30–47. doi: 10.1113/jphysiol.1960.sp006467. [DOI] [PMC free article] [PubMed] [Google Scholar]
- Johnson J. D., Charlton S. C., Potter J. D. A fluorescence stopped flow analysis of Ca2+ exchange with troponin C. J Biol Chem. 1979 May 10;254(9):3497–3502. [PubMed] [Google Scholar]
- LOWRY O. H., ROSEBROUGH N. J., FARR A. L., RANDALL R. J. Protein measurement with the Folin phenol reagent. J Biol Chem. 1951 Nov;193(1):265–275. [PubMed] [Google Scholar]
- Luff A. R. Dynamic properties of the inferior rectus, extensor digitorum longus, diaphragm and soleus muscles of the mouse. J Physiol. 1981;313:161–171. doi: 10.1113/jphysiol.1981.sp013656. [DOI] [PMC free article] [PubMed] [Google Scholar]
- Lymn R. W., Taylor E. W. Mechanism of adenosine triphosphate hydrolysis by actomyosin. Biochemistry. 1971 Dec 7;10(25):4617–4624. doi: 10.1021/bi00801a004. [DOI] [PubMed] [Google Scholar]
- Mannard A., Stein R. B. Determination of the frequency response of isometric soleus muscle in the cat using random nerve stimulation. J Physiol. 1973 Mar;229(2):275–296. doi: 10.1113/jphysiol.1973.sp010138. [DOI] [PMC free article] [PubMed] [Google Scholar]
- Marston S. B., Taylor E. W. Comparison of the myosin and actomyosin ATPase mechanisms of the four types of vertebrate muscles. J Mol Biol. 1980 Jun 5;139(4):573–600. doi: 10.1016/0022-2836(80)90050-9. [DOI] [PubMed] [Google Scholar]
- Matsubara I., Yagi N. A time-resolved X-ray diffraction study of muscle during twitch. J Physiol. 1978 May;278:297–307. doi: 10.1113/jphysiol.1978.sp012305. [DOI] [PMC free article] [PubMed] [Google Scholar]
- Pagala M. K. Effect of length and caffeine on isometric tetanus relaxation of frog sartorius muscles. Biochim Biophys Acta. 1980 Jun 10;591(1):177–186. doi: 10.1016/0005-2728(80)90231-5. [DOI] [PubMed] [Google Scholar]
- Parmiggiani F., Stein R. B. Nonlinear summation of contractions in cat muscles. II. Later facilitation and stiffness changes. J Gen Physiol. 1981 Sep;78(3):295–311. doi: 10.1085/jgp.78.3.295. [DOI] [PMC free article] [PubMed] [Google Scholar]
- Rack P. M., Westbury D. R. The effects of length and stimulus rate on tension in the isometric cat soleus muscle. J Physiol. 1969 Oct;204(2):443–460. doi: 10.1113/jphysiol.1969.sp008923. [DOI] [PMC free article] [PubMed] [Google Scholar]
- Ranatunga K. W. Influence of temperature on isometric tension development in mouse fast- and slow-twitch skeletal muscles. Exp Neurol. 1980 Nov;70(2):211–218. doi: 10.1016/0014-4886(80)90021-7. [DOI] [PubMed] [Google Scholar]
- Robertson S. P., Johnson J. D., Potter J. D. The time-course of Ca2+ exchange with calmodulin, troponin, parvalbumin, and myosin in response to transient increases in Ca2+. Biophys J. 1981 Jun;34(3):559–569. doi: 10.1016/S0006-3495(81)84868-0. [DOI] [PMC free article] [PubMed] [Google Scholar]
- Sandow A. Excitation-contraction coupling in skeletal muscle. Pharmacol Rev. 1965 Sep;17(3):265–320. [PubMed] [Google Scholar]
- Sandow A., Zeman R. J. Tetanus relaxation. Temperature effects and Arrhenius analysis. Biochim Biophys Acta. 1979 Jul 10;547(1):27–35. doi: 10.1016/0005-2728(79)90092-6. [DOI] [PubMed] [Google Scholar]
- Somlyo A. V., Gonzalez-Serratos H. G., Shuman H., McClellan G., Somlyo A. P. Calcium release and ionic changes in the sarcoplasmic reticulum of tetanized muscle: an electron-probe study. J Cell Biol. 1981 Sep;90(3):577–594. doi: 10.1083/jcb.90.3.577. [DOI] [PMC free article] [PubMed] [Google Scholar]
- Squire J. Muscle regulation: a decade of the steric blocking model. Nature. 1981 Jun 25;291(5817):614–615. doi: 10.1038/291614a0. [DOI] [PubMed] [Google Scholar]
- Sréter F. A., Luff A. R., Gergely J. Effect of cross-reinnervation on physiological parameters and on properties of myosin and sarcoplasmic reticulum of fast and slow muscles of the rabbit. J Gen Physiol. 1975 Dec;66(6):811–821. doi: 10.1085/jgp.66.6.811. [DOI] [PMC free article] [PubMed] [Google Scholar]
- Stein R. B., Parmiggiani F. The stiffness of slow-twitch muscle lags behind twitch tension: implications for contractile mechanisms and behavior. Can J Physiol Pharmacol. 1979 Oct;57(10):1189–1192. doi: 10.1139/y79-178. [DOI] [PubMed] [Google Scholar]
- TAUSSKY H. H., SHORR E. A microcolorimetric method for the determination of inorganic phosphorus. J Biol Chem. 1953 Jun;202(2):675–685. [PubMed] [Google Scholar]
- Travers F., Hillaire D. Cryoenzymological studies on myosin subfragment 1. Solvent, temperature and pH effects on the overall reaction. Eur J Biochem. 1979 Jul;98(1):293–299. doi: 10.1111/j.1432-1033.1979.tb13188.x. [DOI] [PubMed] [Google Scholar]