Abstract
Total intensity and fine structure of first-order laser light diffraction maxima from single skinned rabbit psoas fibers were studied. Total intensity of the diffraction maxima was measured as a function of the incidence angle (omega-scan). In the most homogeneous fibers, most of the intensity in the diffraction maxima is confined to a rather narrow range of incidence angles. Fibers with less homogeneous striation patterns, apparently composed of several regions of distinct sarcomere length and tilt of striation (domains), give rise to several narrow intensity peaks in their omega-scans. Left and right first-order diffraction lines produce omega-scans of almost identical shape, composed of one or more intensity peaks, with each pair of corresponding peaks separated by about the same angle. The data indicated that in single skinned rabbit psoas fibers, light diffraction is dominated by Bragg diffraction and that the peaks within omega-scans can be directly correlated with domains within the illuminated fiber segment. In the most homogeneous fiber segments the diameter of domains, estimated from the width of the corresponding maxima in the omega-scans, could almost be as large as the fiber diameter. On average, from the number of peaks in the omega-scans two to three domains with an average length of approximately 250-350 microns can be identified in a fiber cross-section. Therefore, on average only a small number of domains (8 per mm) are found within skinned rabbit psoas fiber segments. In contrast, the number of substructural lines within the diffraction maxima is large even for microscopically homogeneous fibers. Substructural lines appear to be present only when several domains are illuminated simultaneously. Separation and width of these substructural lines are approximately inversely proportional to the length of the illuminated region of the fiber. These data suggest that the substructural lines are due to interference between domains, illuminated simultaneously by a light source with a high degree of spatial coherence (laser). The relevance of these findings for measurements of sarcomere length by laser light diffraction is discussed.
Full text
PDF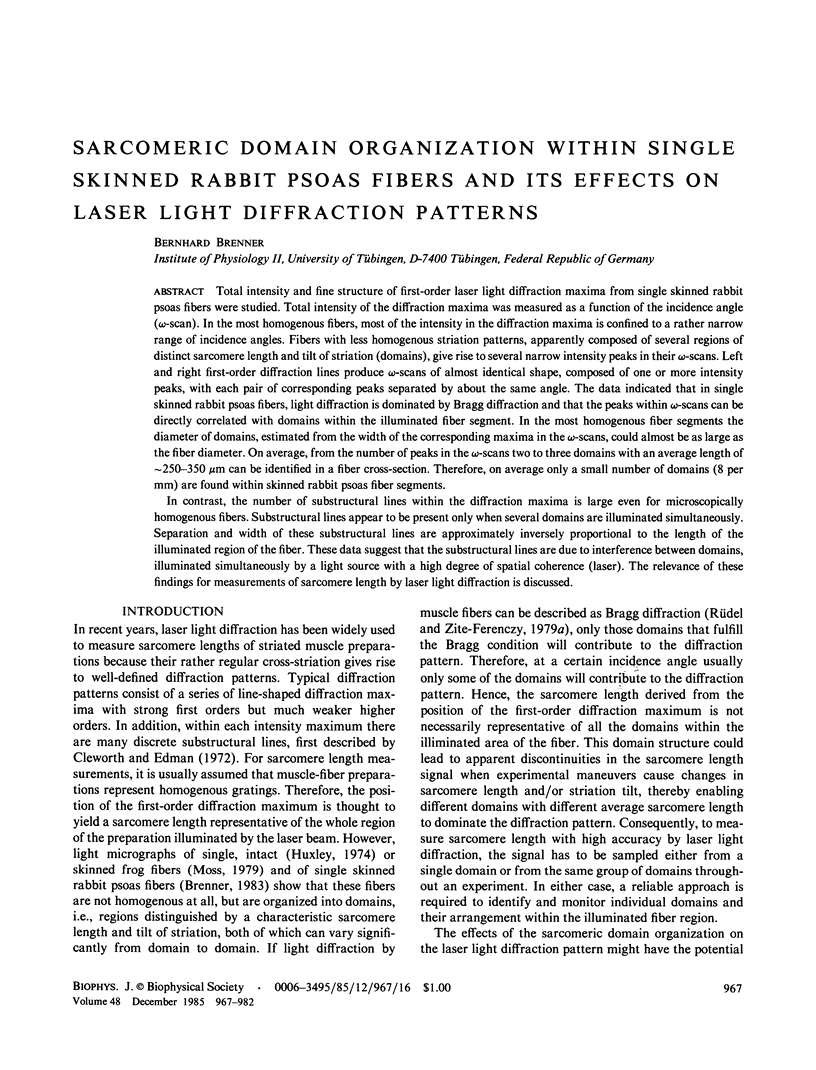
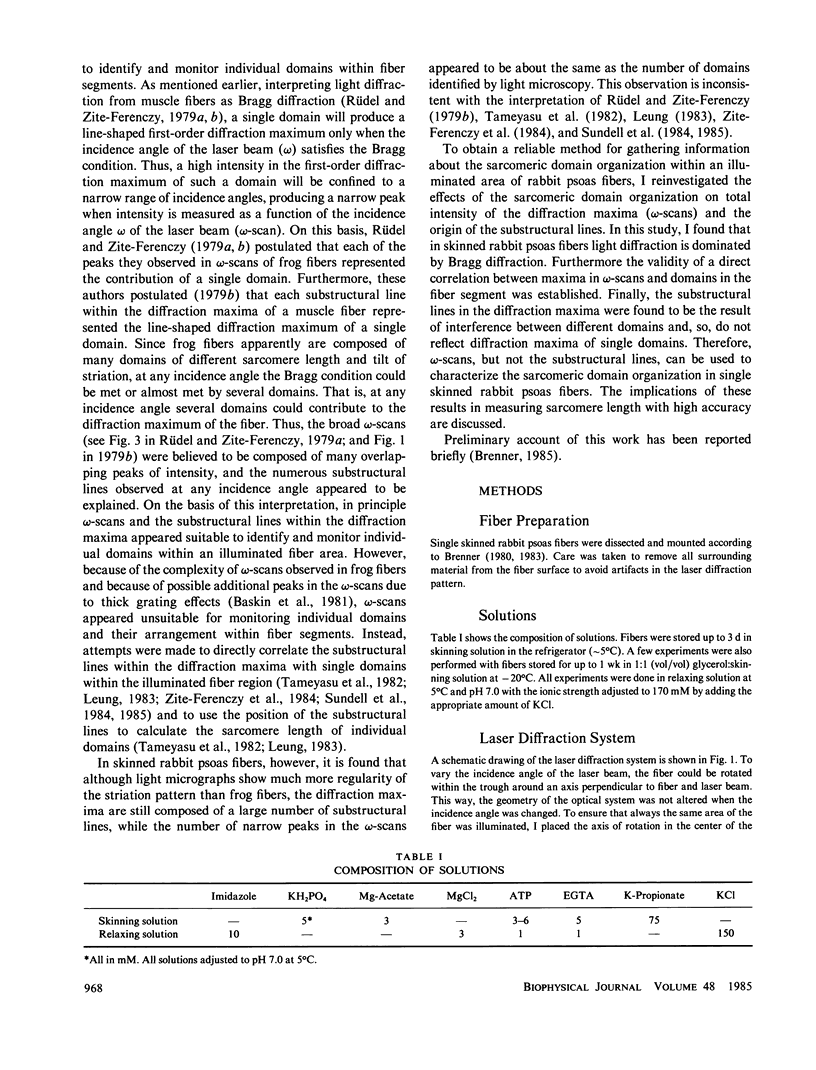
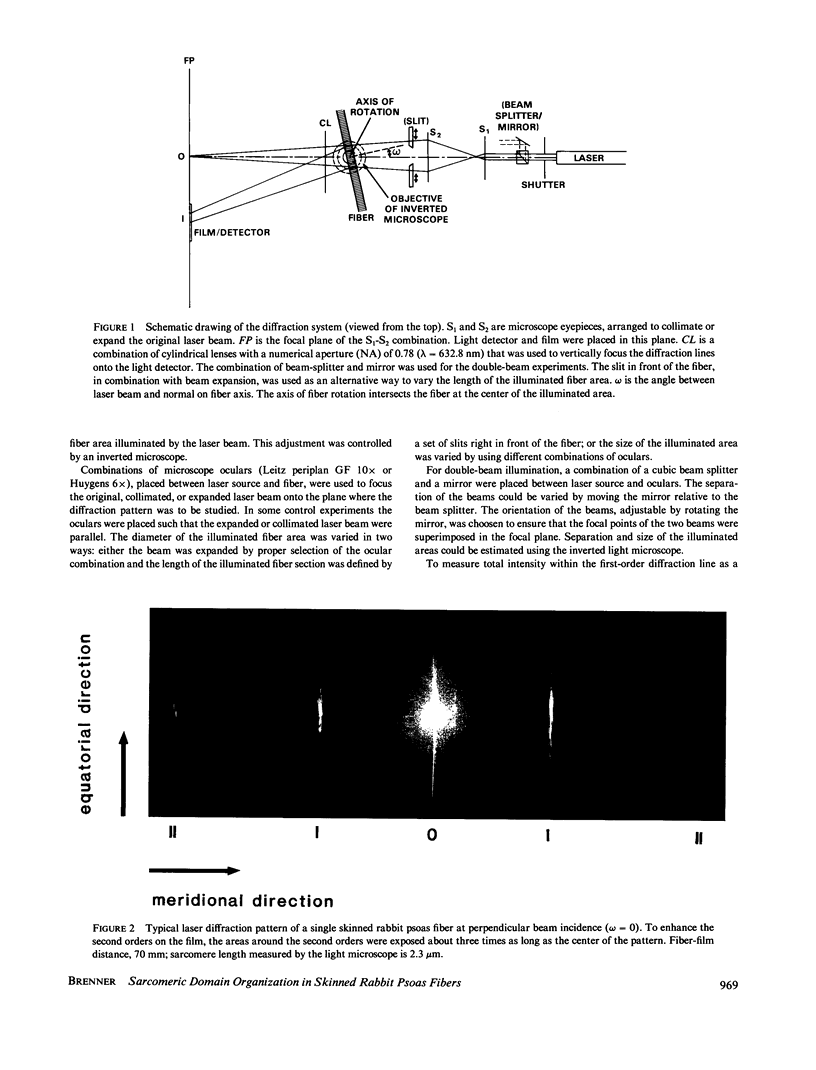
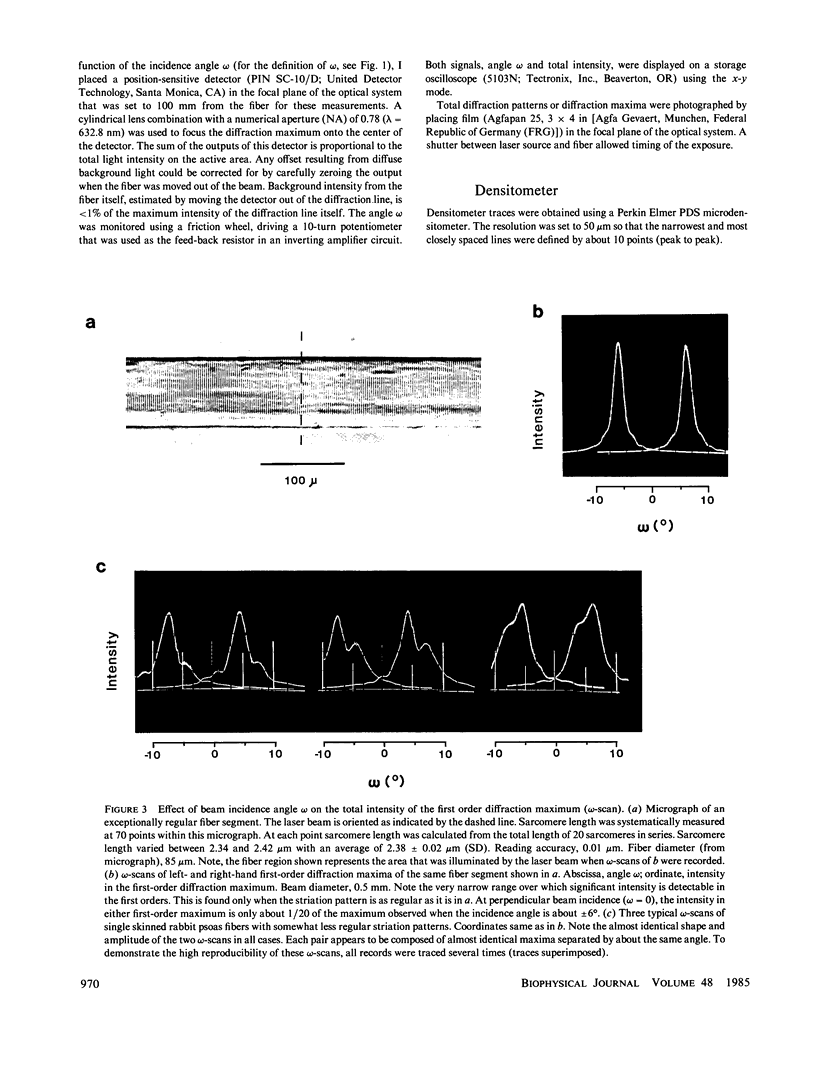
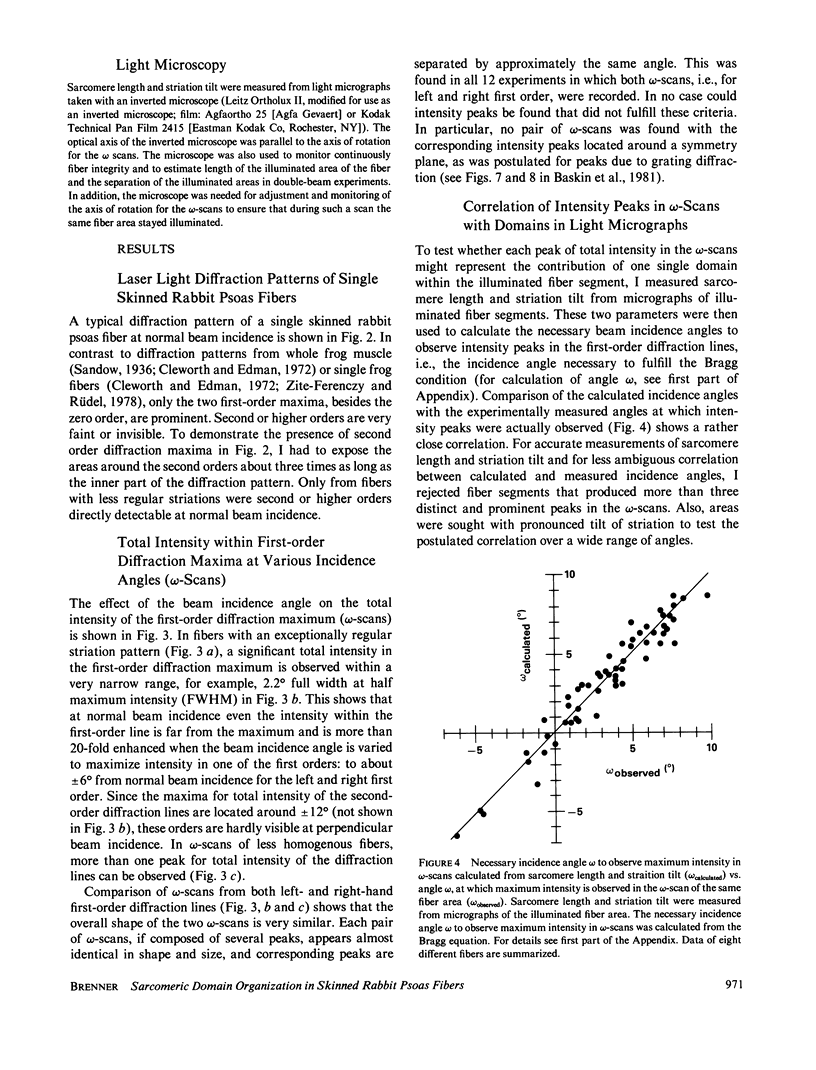
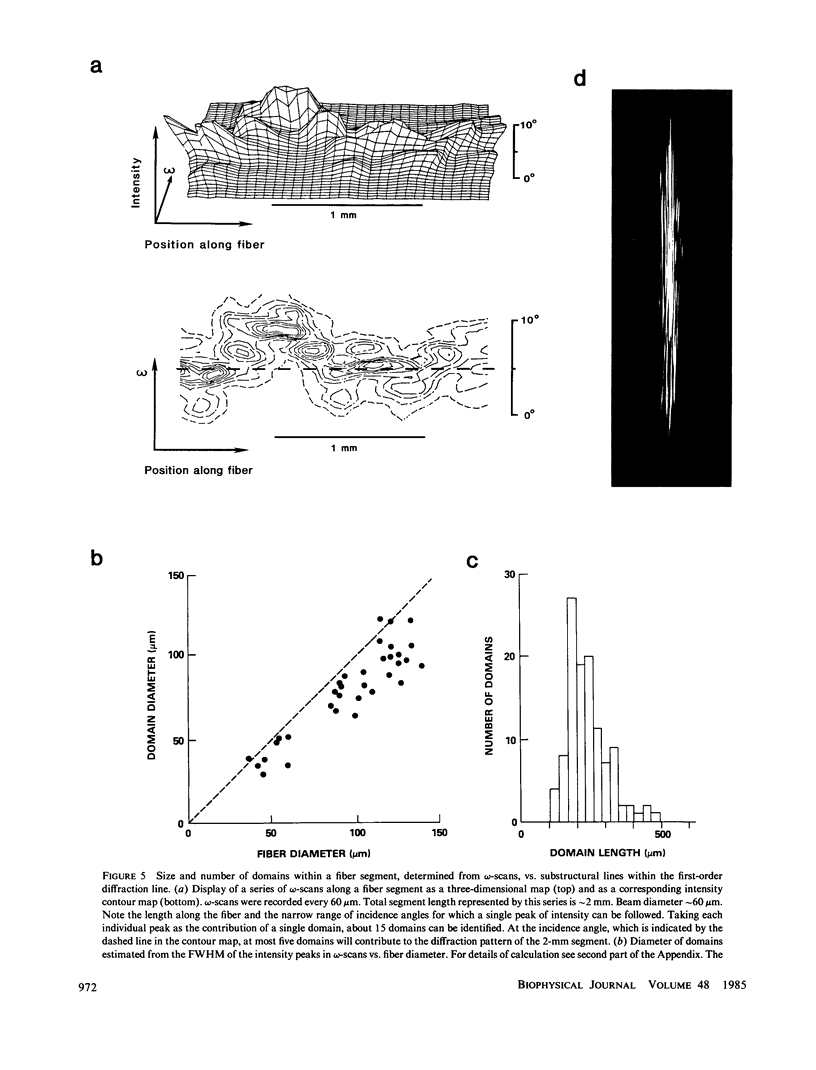
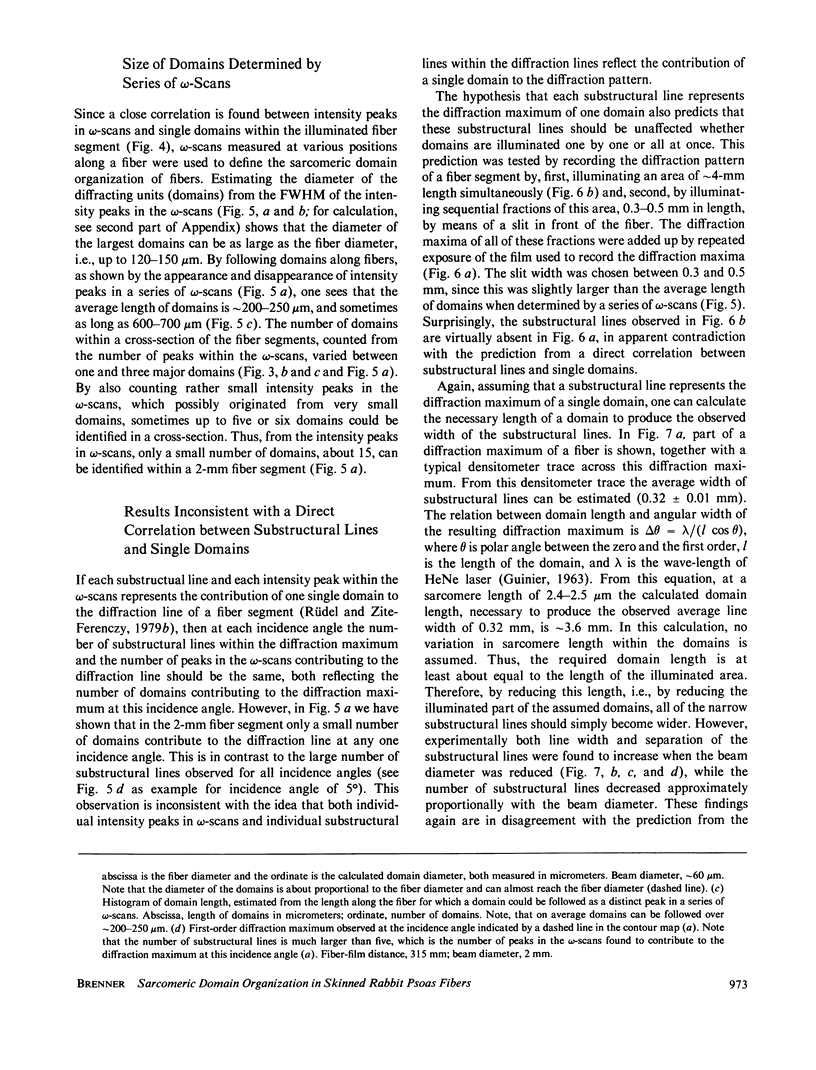
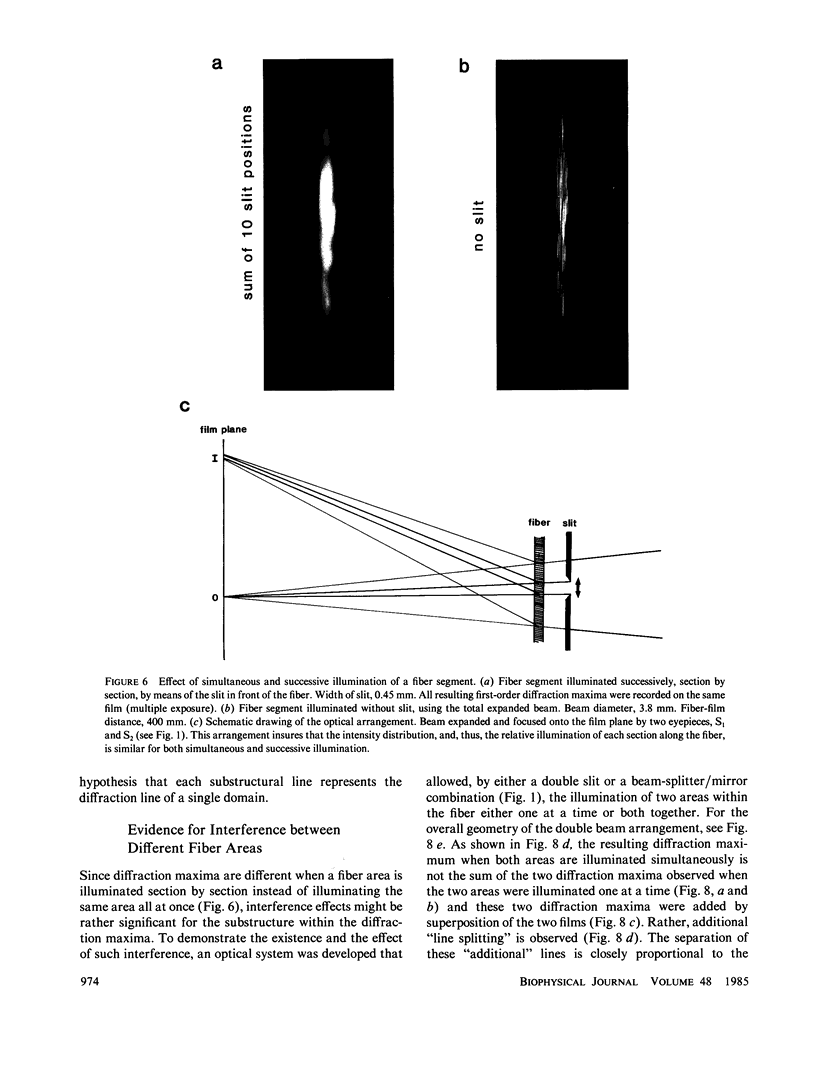
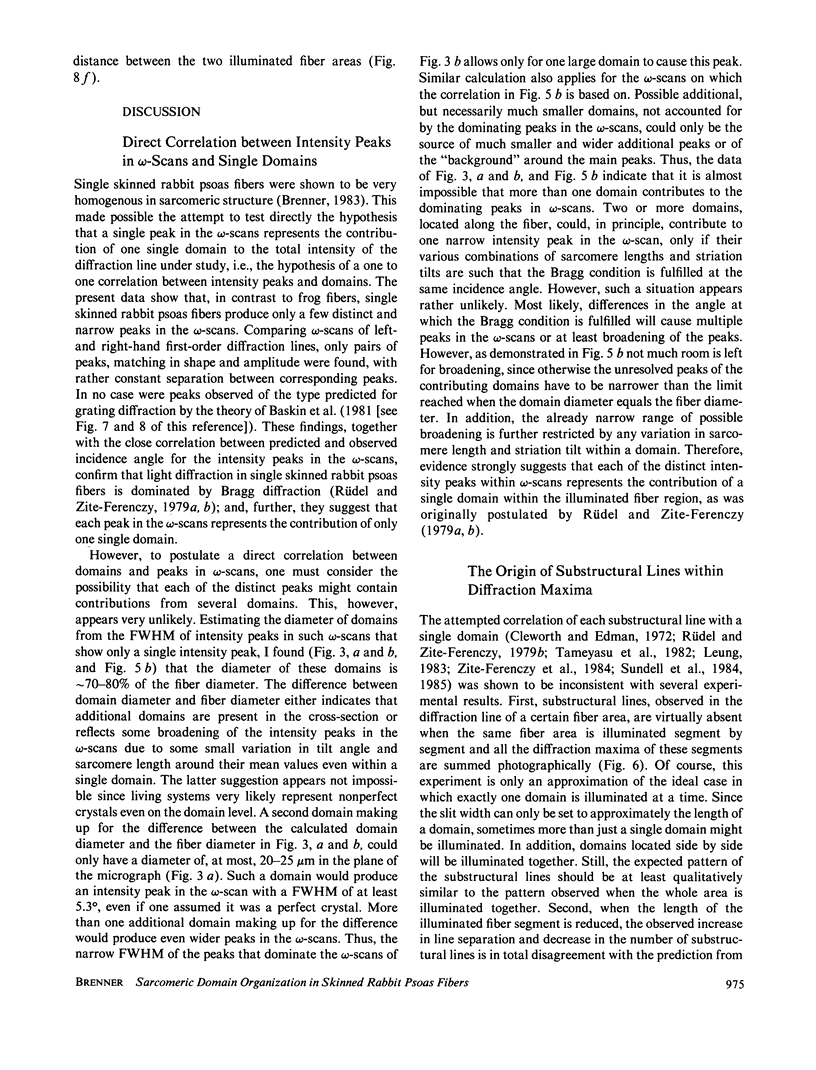
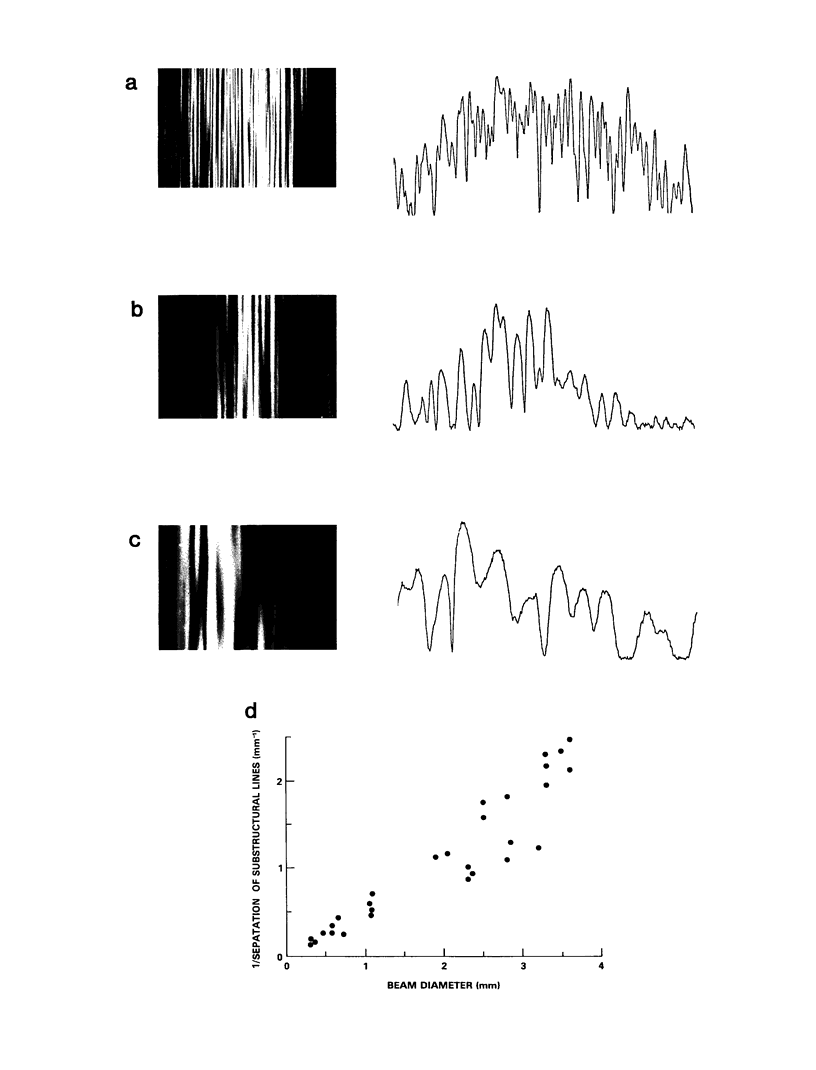
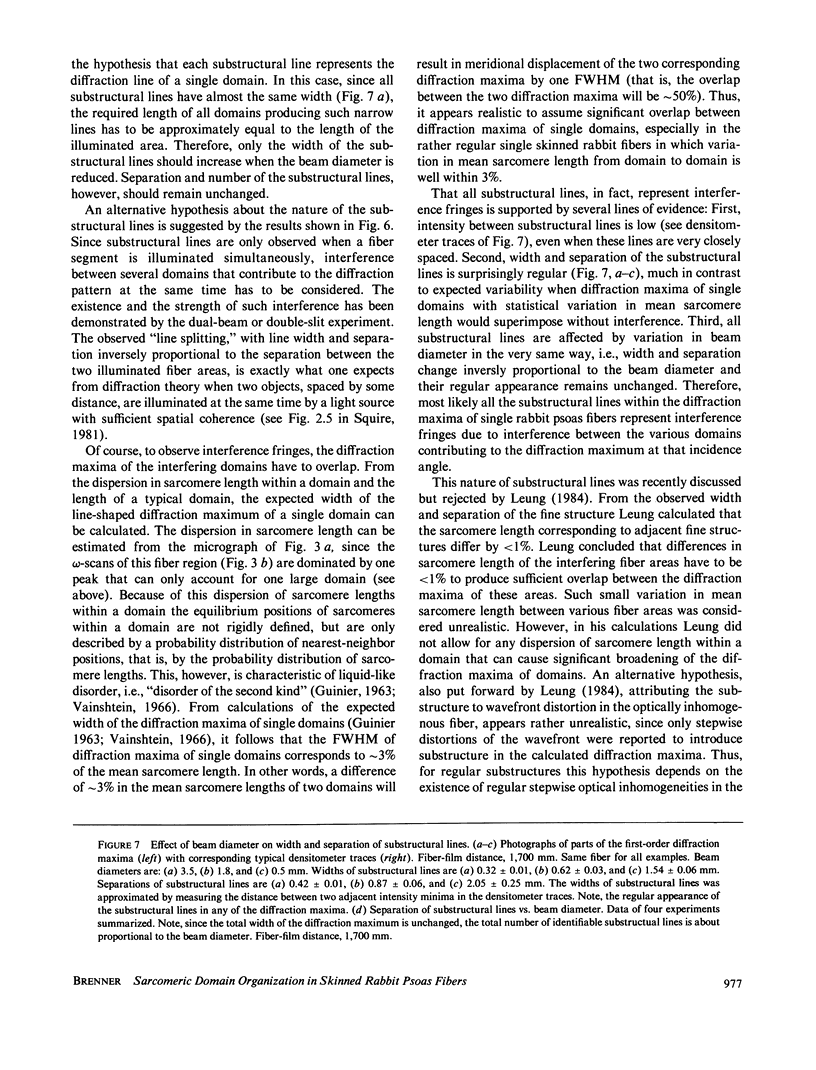
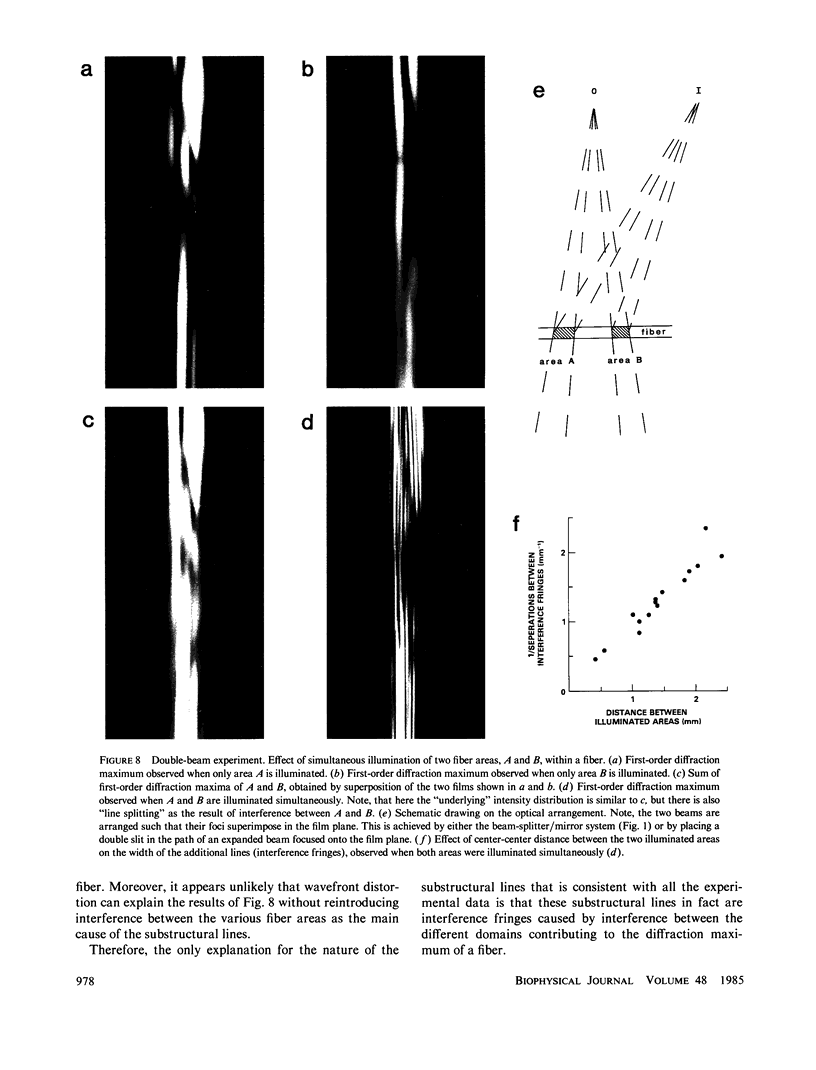
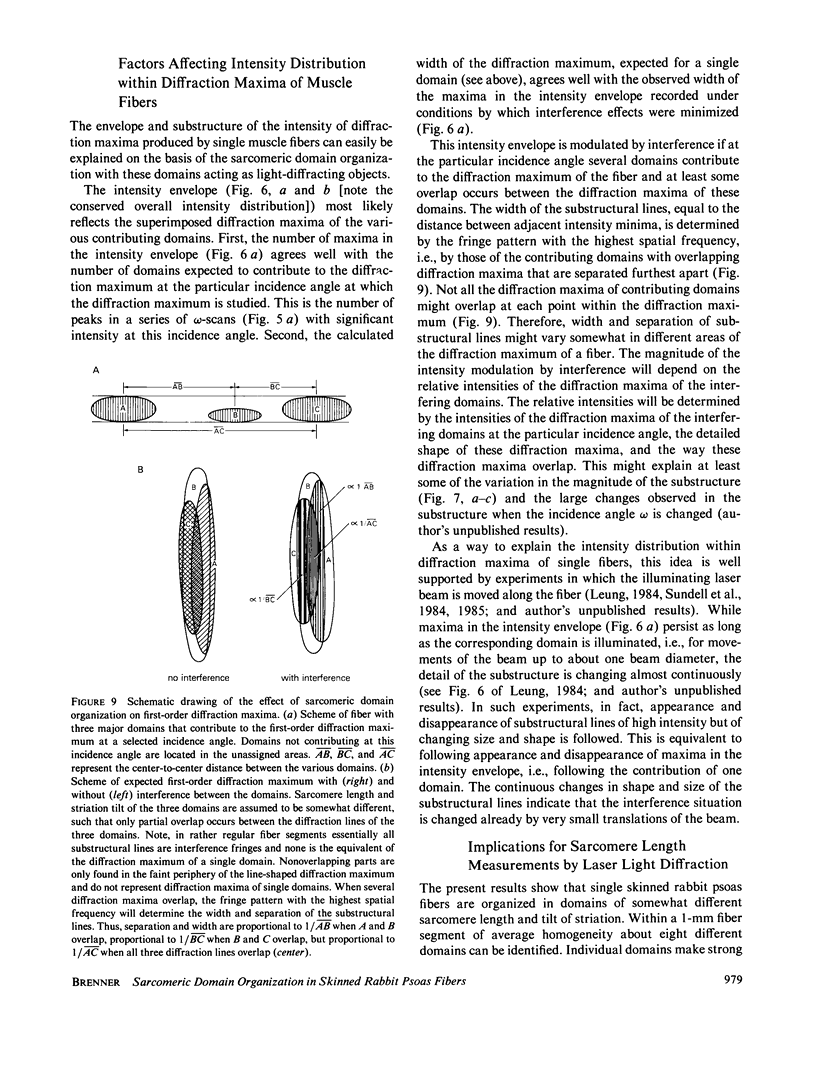
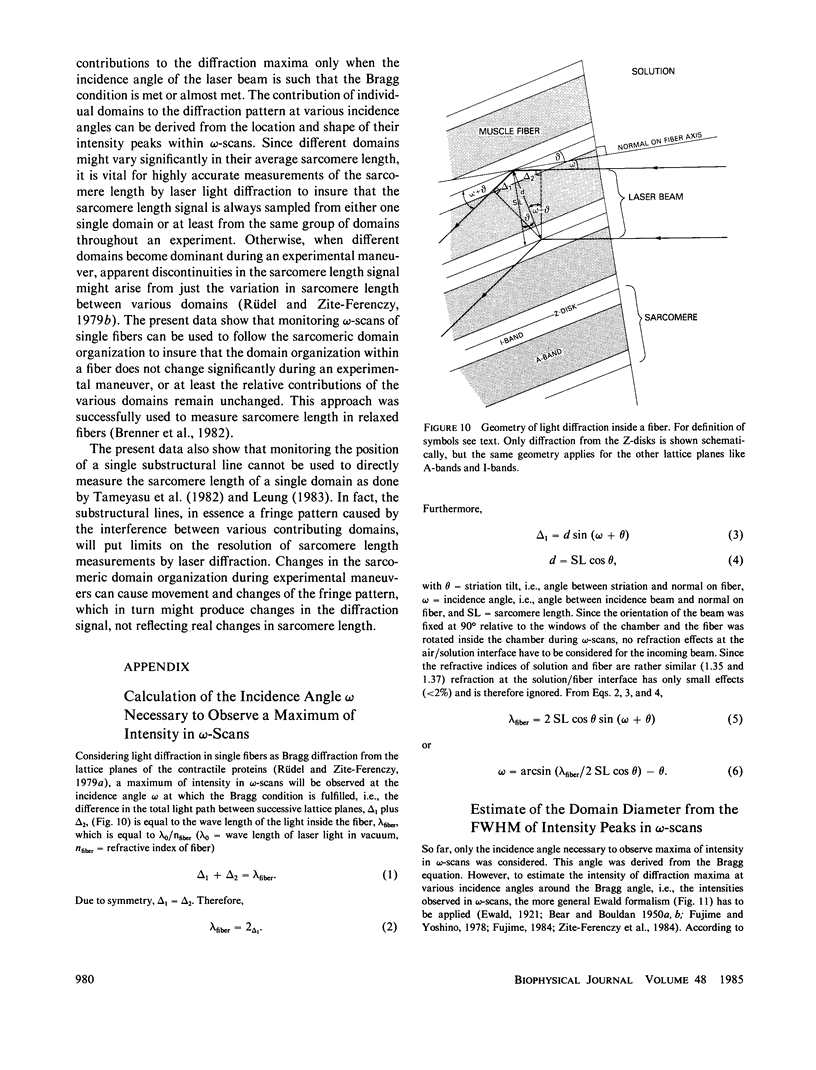
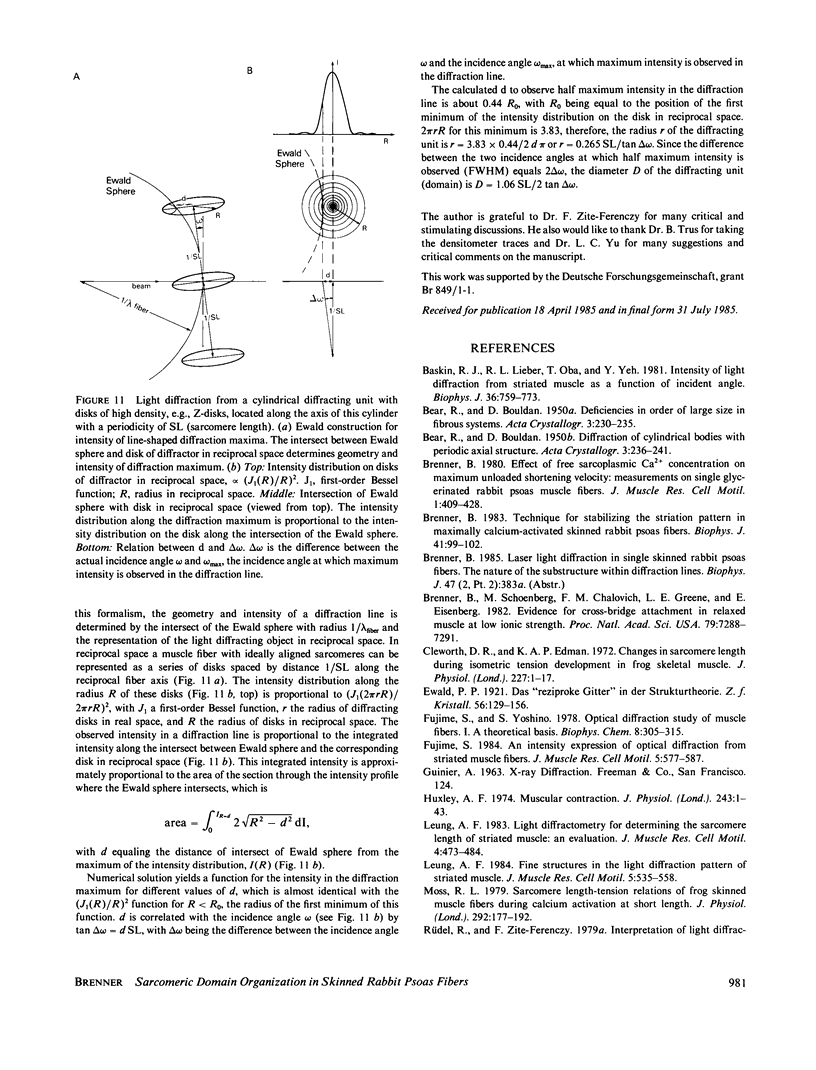
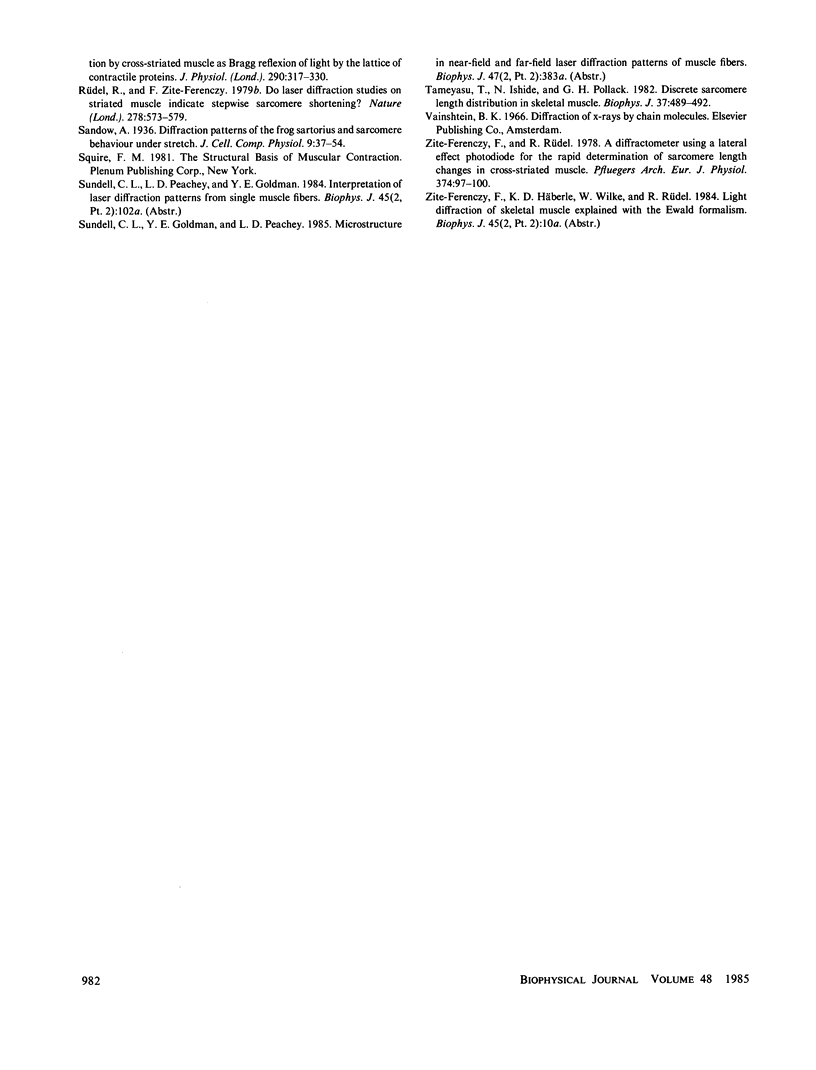
Images in this article
Selected References
These references are in PubMed. This may not be the complete list of references from this article.
- Baskin R. J., Lieber R. L., Oba T., Yeh Y. Intensity of light diffraction from striated muscle as a function of incident angle. Biophys J. 1981 Dec;36(3):759–773. doi: 10.1016/S0006-3495(81)84764-9. [DOI] [PMC free article] [PubMed] [Google Scholar]
- Brenner B., Schoenberg M., Chalovich J. M., Greene L. E., Eisenberg E. Evidence for cross-bridge attachment in relaxed muscle at low ionic strength. Proc Natl Acad Sci U S A. 1982 Dec;79(23):7288–7291. doi: 10.1073/pnas.79.23.7288. [DOI] [PMC free article] [PubMed] [Google Scholar]
- Brenner B. Technique for stabilizing the striation pattern in maximally calcium-activated skinned rabbit psoas fibers. Biophys J. 1983 Jan;41(1):99–102. doi: 10.1016/S0006-3495(83)84411-7. [DOI] [PMC free article] [PubMed] [Google Scholar]
- Cleworth D. R., Edman K. A. Changes in sarcomere length during isometric tension development in frog skeletal muscle. J Physiol. 1972 Dec;227(1):1–17. doi: 10.1113/jphysiol.1972.sp010016. [DOI] [PMC free article] [PubMed] [Google Scholar]
- Fujime S. An intensity expression of optical diffraction from striated muscle fibres. J Muscle Res Cell Motil. 1984 Oct;5(5):577–587. doi: 10.1007/BF00713262. [DOI] [PubMed] [Google Scholar]
- Fujime S., Yoshino S. Optical diffraction study of muscle fibers. I. A theoretical basis. Biophys Chem. 1978 Sep;8(4):305–315. doi: 10.1016/0301-4622(78)80013-1. [DOI] [PubMed] [Google Scholar]
- Leung A. F. Fine structures in the light diffraction pattern of striated muscle. J Muscle Res Cell Motil. 1984 Oct;5(5):535–558. doi: 10.1007/BF00713260. [DOI] [PubMed] [Google Scholar]
- Leung A. F. Light diffractometry for determining the sarcomere length of striated muscle: an evaluation. J Muscle Res Cell Motil. 1983 Aug;4(4):473–484. doi: 10.1007/BF00711950. [DOI] [PubMed] [Google Scholar]
- Moss R. L. Sarcomere length-tension relations of frog skinned muscle fibres during calcium activation at short lengths. J Physiol. 1979 Jul;292:177–192. doi: 10.1113/jphysiol.1979.sp012845. [DOI] [PMC free article] [PubMed] [Google Scholar]
- Rüdel R., Zite-Ferenczy F. Do laser diffraction studies on striated muscle indicate stepwise sarcomere shortening? Nature. 1979 Apr 5;278(5704):573–575. doi: 10.1038/278573a0. [DOI] [PubMed] [Google Scholar]
- Tameyasu T., Ishide N., Pollack G. H. Discrete sarcomere length distribution in skeletal muscle. Biophys J. 1982 Feb;37(2):489–492. doi: 10.1016/S0006-3495(82)84695-X. [DOI] [PMC free article] [PubMed] [Google Scholar]
- Zite-Ferenczy F., Rüdel R. A diffractometer using a lateral effect photodiode for the rapid determination of sarcomere length changes in cross-striated muscle. Pflugers Arch. 1978 Apr 25;374(1):97–100. doi: 10.1007/BF00585702. [DOI] [PubMed] [Google Scholar]