Abstract
Single fibers, isolated intact from frog skeletal muscles, were held firmly very near to each end by stiff metal clasps fastened to the tendons. The fibers were then placed horizontally between two steel hooks inserted in eyelets of the tendon clasps. One hook was attached to a capacitance gauge force transducer (resonance frequency up to approximately 50 kHz) and the other was attached to a moving-coil length changer. This allowed us to impose small, rapid releases (complete in less than 0.15 ms) and high frequency oscillations (up to 13 kHz) to one end of a resting or contracting fiber and measure the consequences at the other end with fast time resolution at 4 to 6 degrees C. The stiffness of short fibers (1.8-2.6 mm) was determined directly from the ratio of force to length variations produced by the length changer. The resonance frequency of short fibers was so high (approximately 40 kHz) that intrinsic oscillations were not detectably excited. The stiffness of long fibers, on the other hand, was calculated from measurement of the mechanical resonance frequency of a fiber. Using both short and long fibers, we measured the sinusoids of force at one end of a contracting fiber that were produced by relatively small sinusoidal length changes at the other end. The amplitudes of the sinusoidal length changes were small compared with the size of step changes that produce nonlinear force-extension relations. The sinusoids of force from long fibers changed amplitude and shifted phase with changes in oscillation frequency in a manner expected of a transmission line composed of mass, compliance, and viscosity, similar to that modelled by (Ford, L. E., A. F. Huxley, and R. M. Simmons, 1981, J. Physiol. (Lond.), 311:219-249). A rapid release during the plateau of tetanic tension in short fibers caused a fall in force and stiffness, a relative change in stiffness that putatively was much smaller than that of force. Our results are, for the most part, consistent with the cross-bridge model of force generation proposed by Huxley, A. F., and R. M. Simmons (1971, Nature (Lond.), 213:533-538). However, stiffness in short fibers developed markedly faster than force during the tetanus rise. Thus our findings show the presence of one or more noteworthy cross-bridge states at the onset and during the rise of active tension towards a plateau in that attachment apparently is followed by a relatively long delay before force generation occurs. A set of equations is given in the Appendix that describes the frequency dependence of the applied sinusoid and its response. This model predicts that frequency dependent changes can be used as a measure of a change in stiffness.
Full text
PDF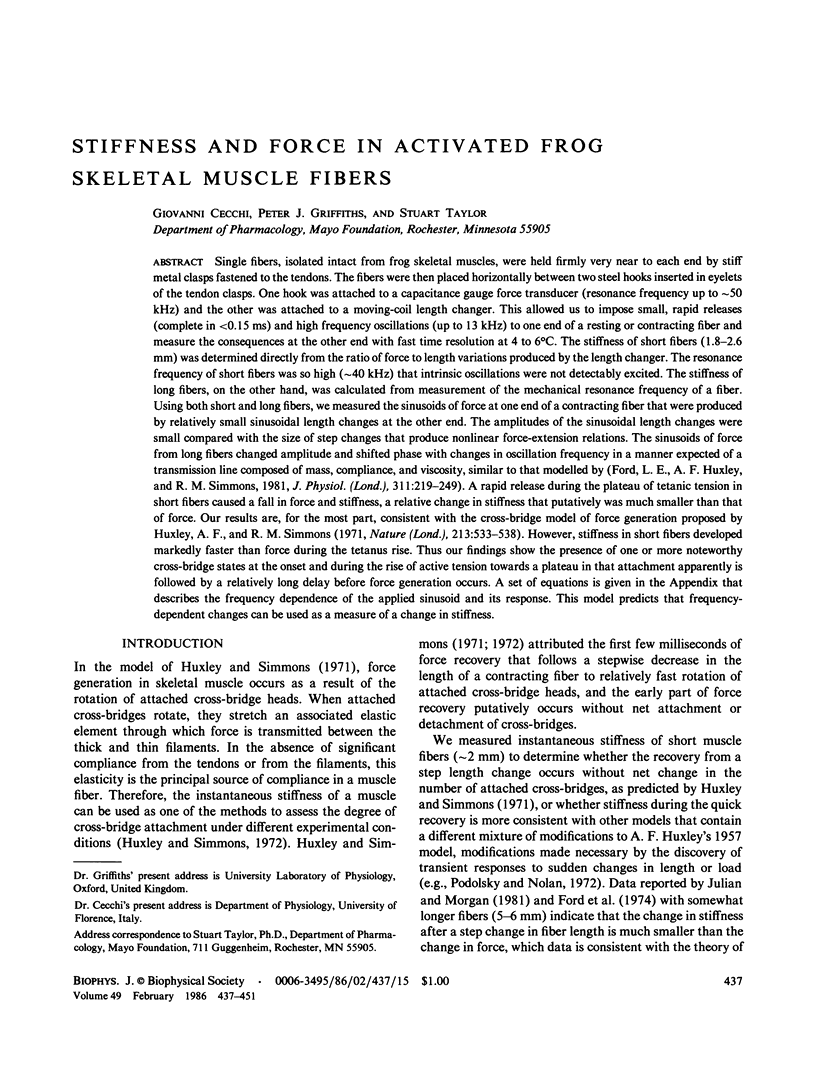
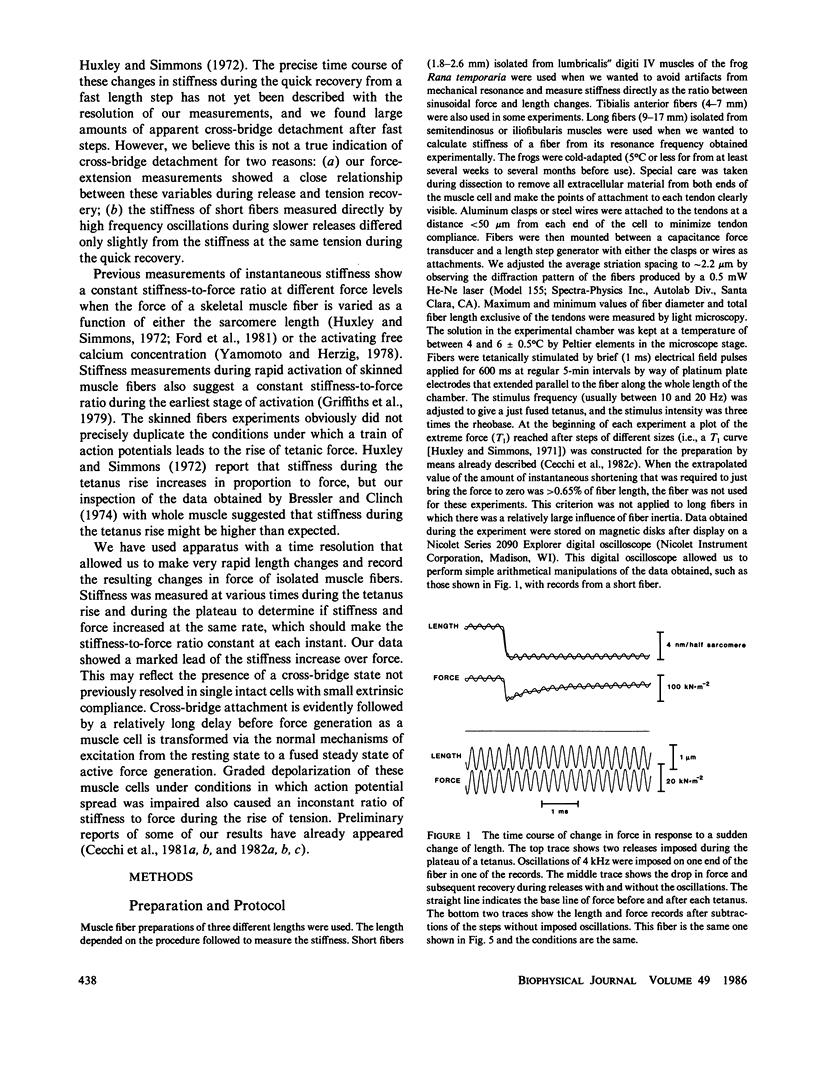
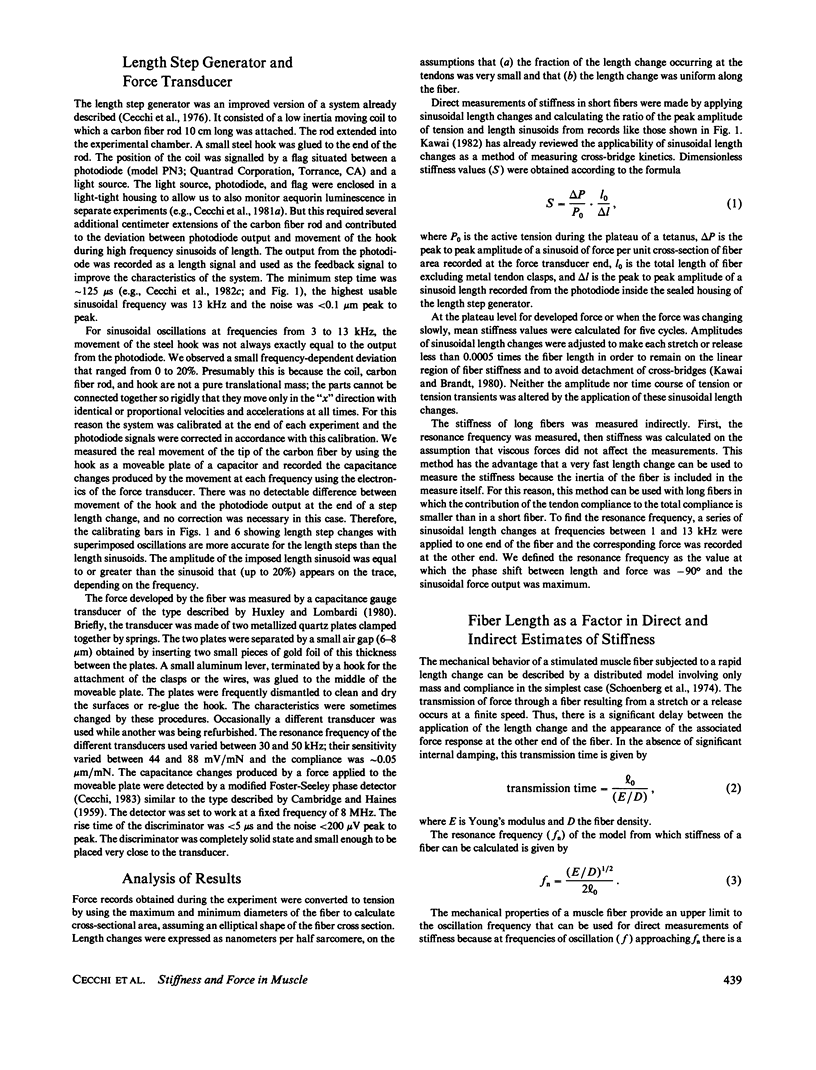
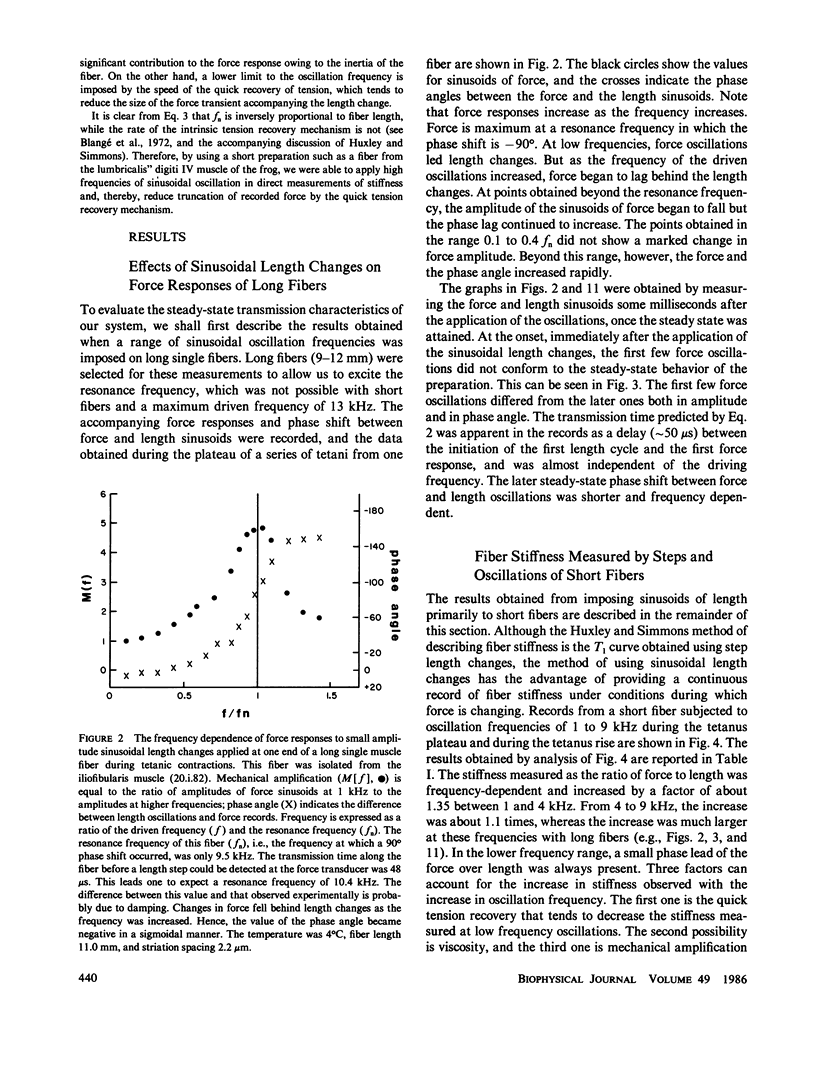
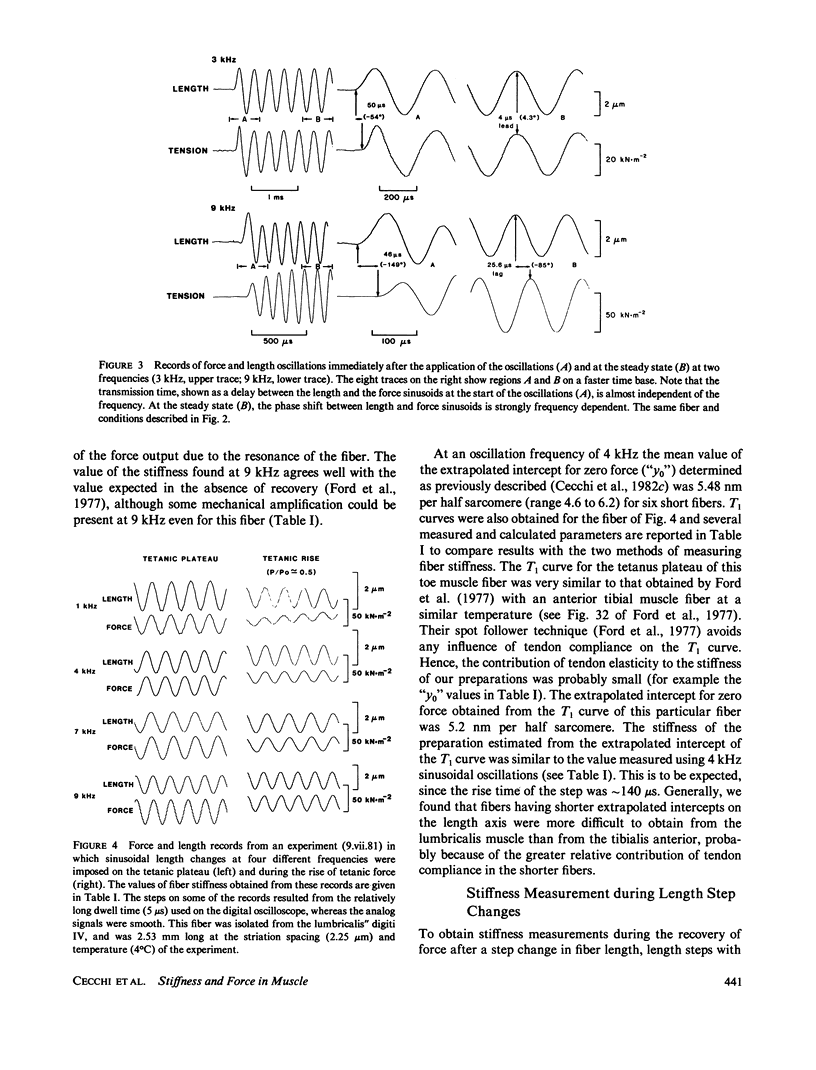
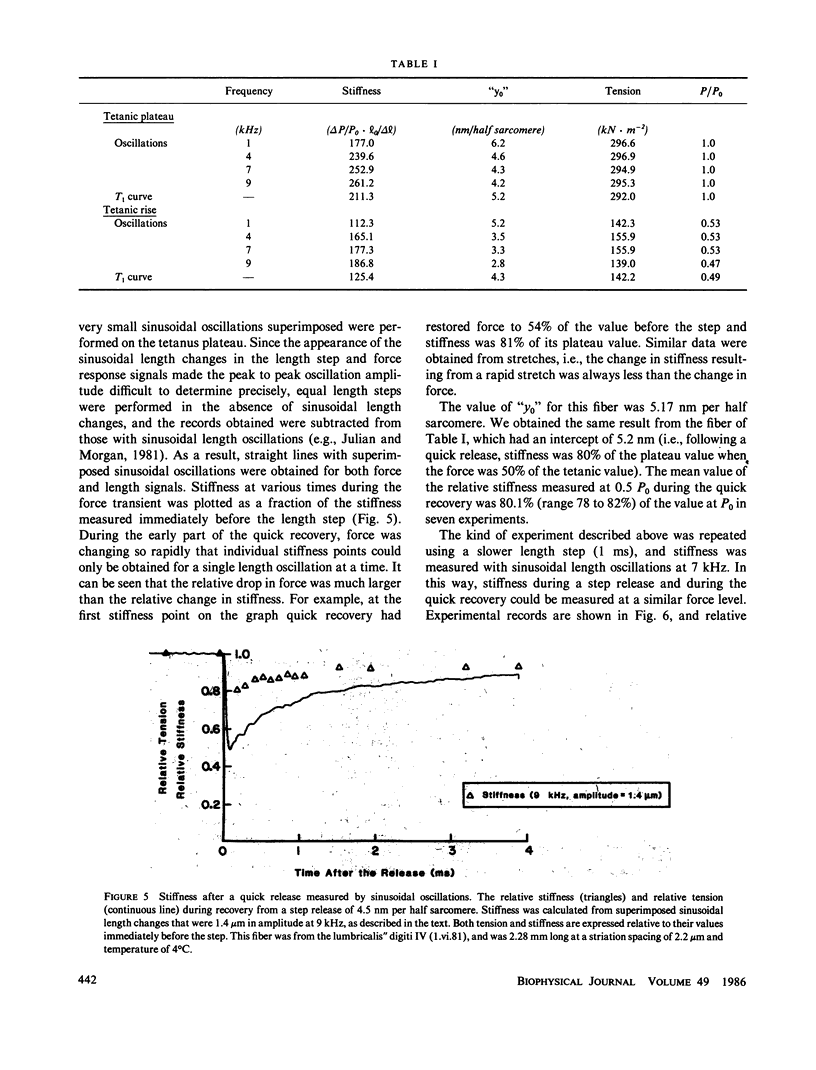
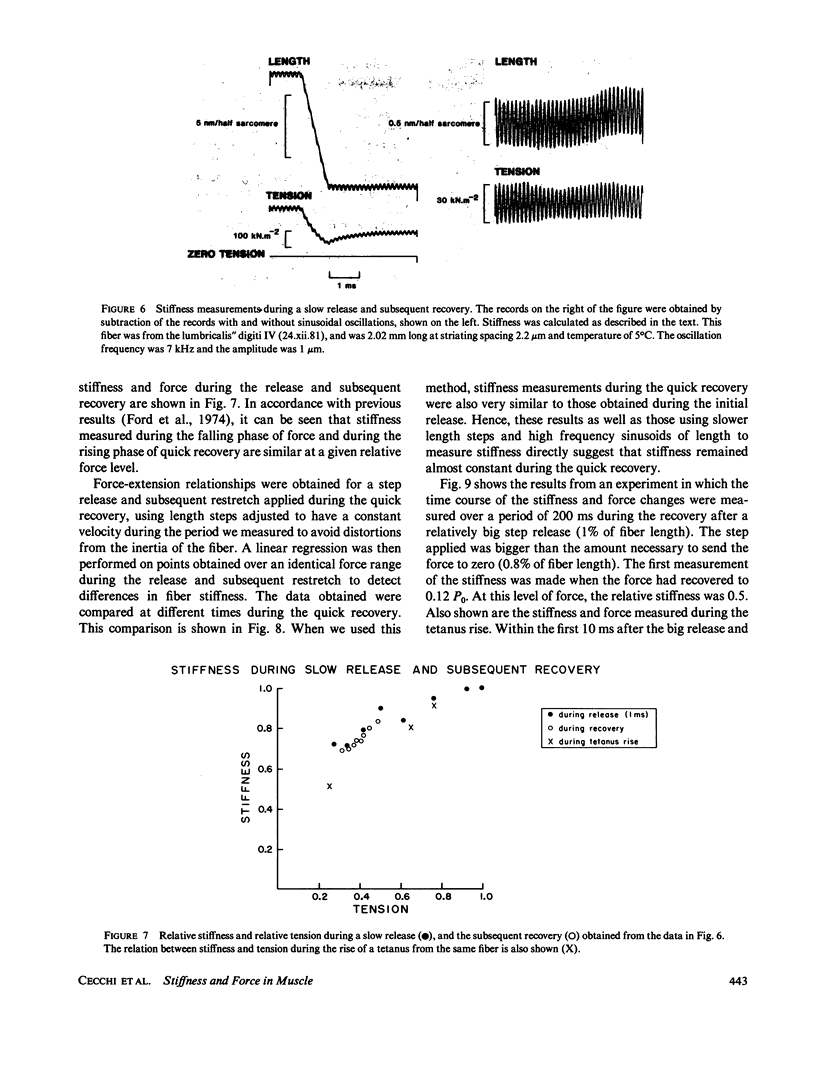
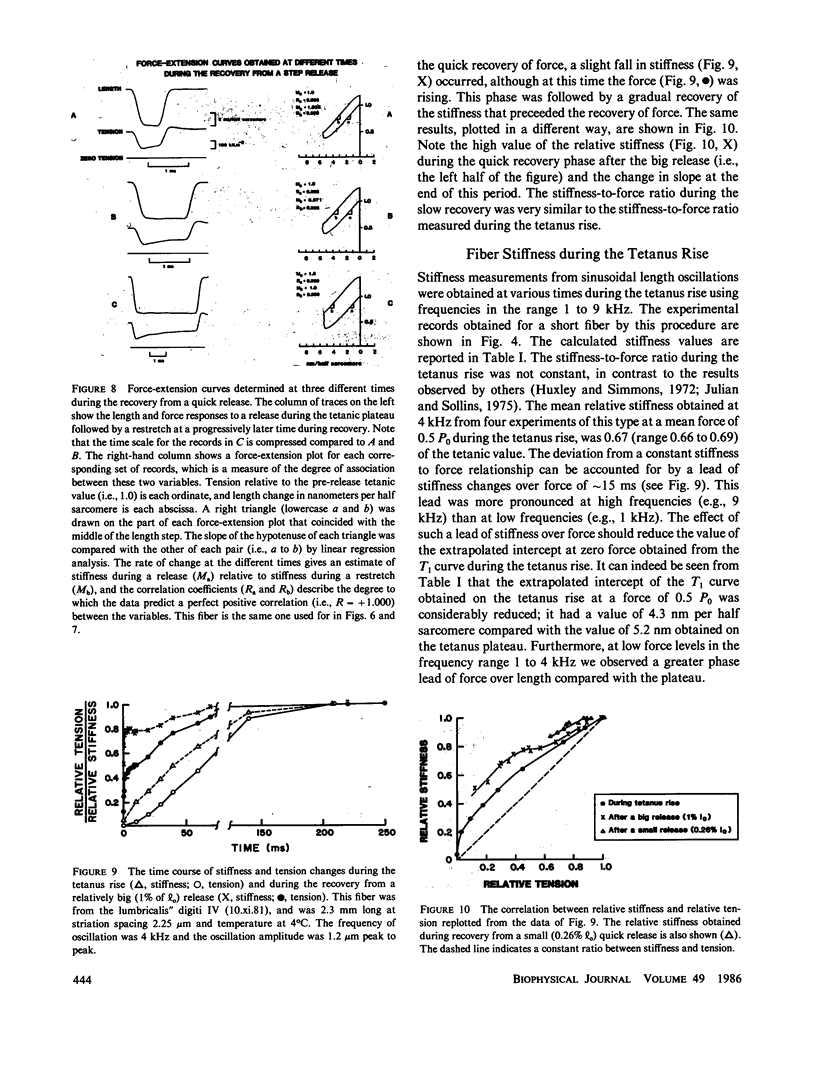
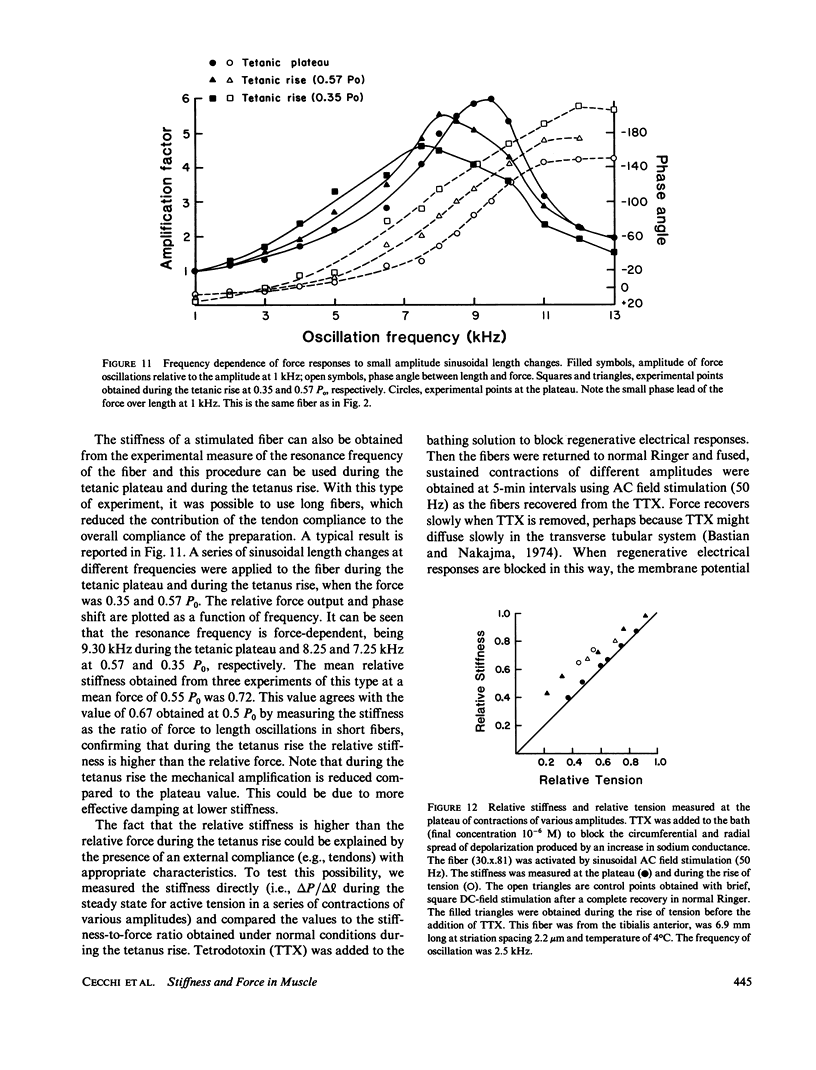
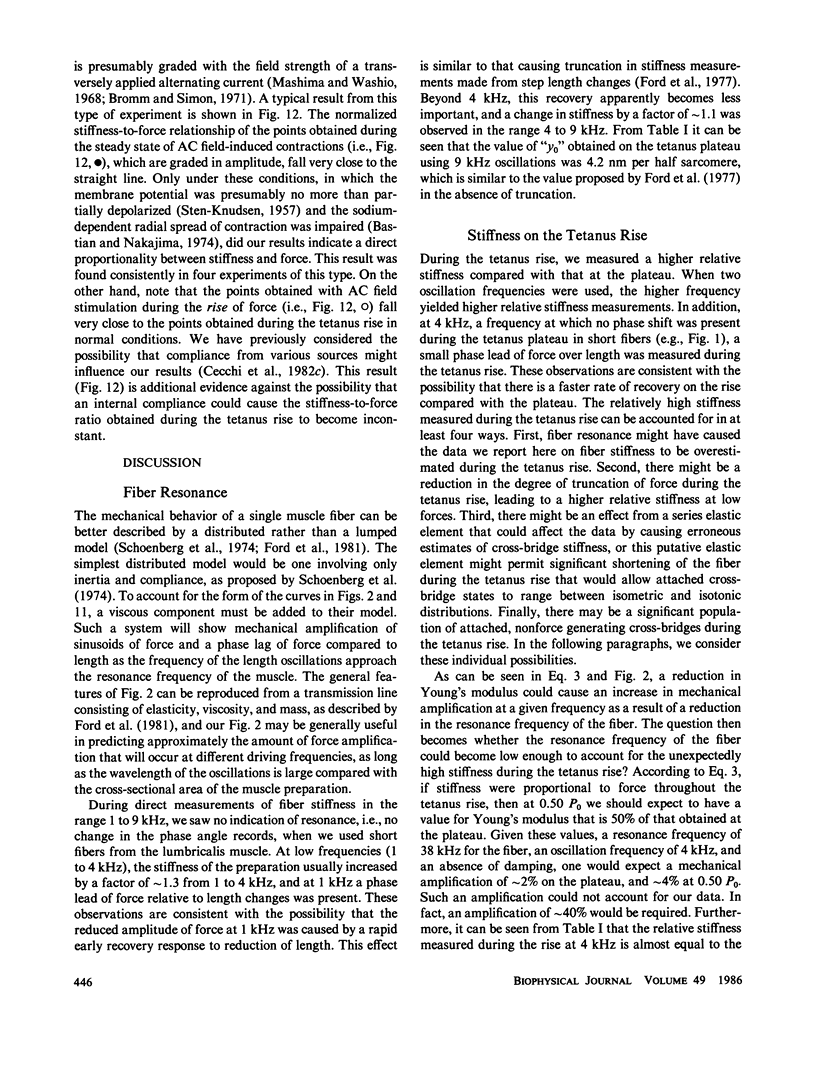
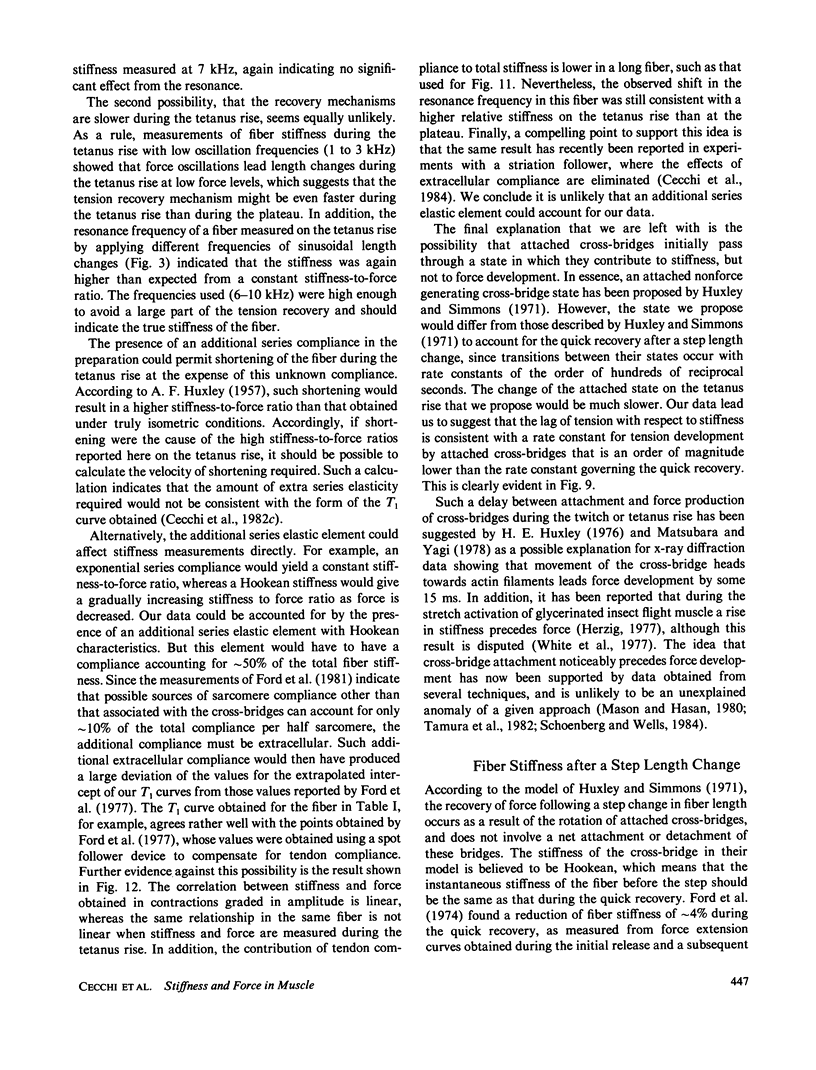
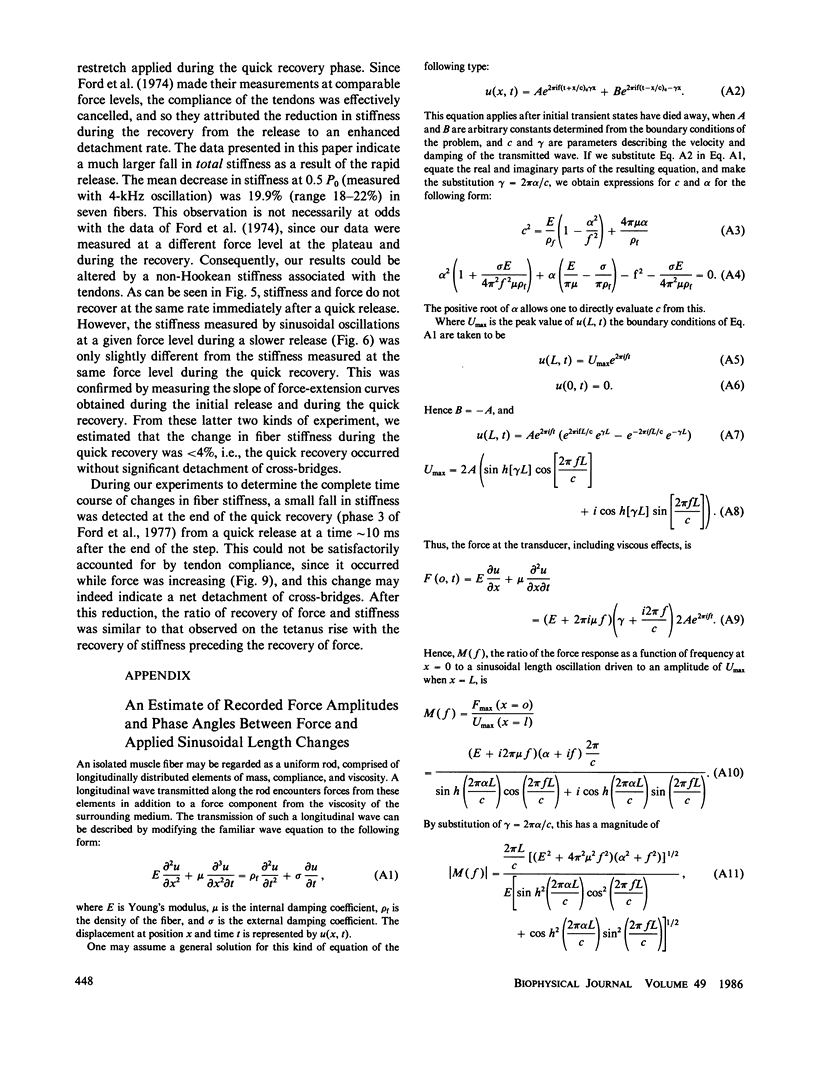
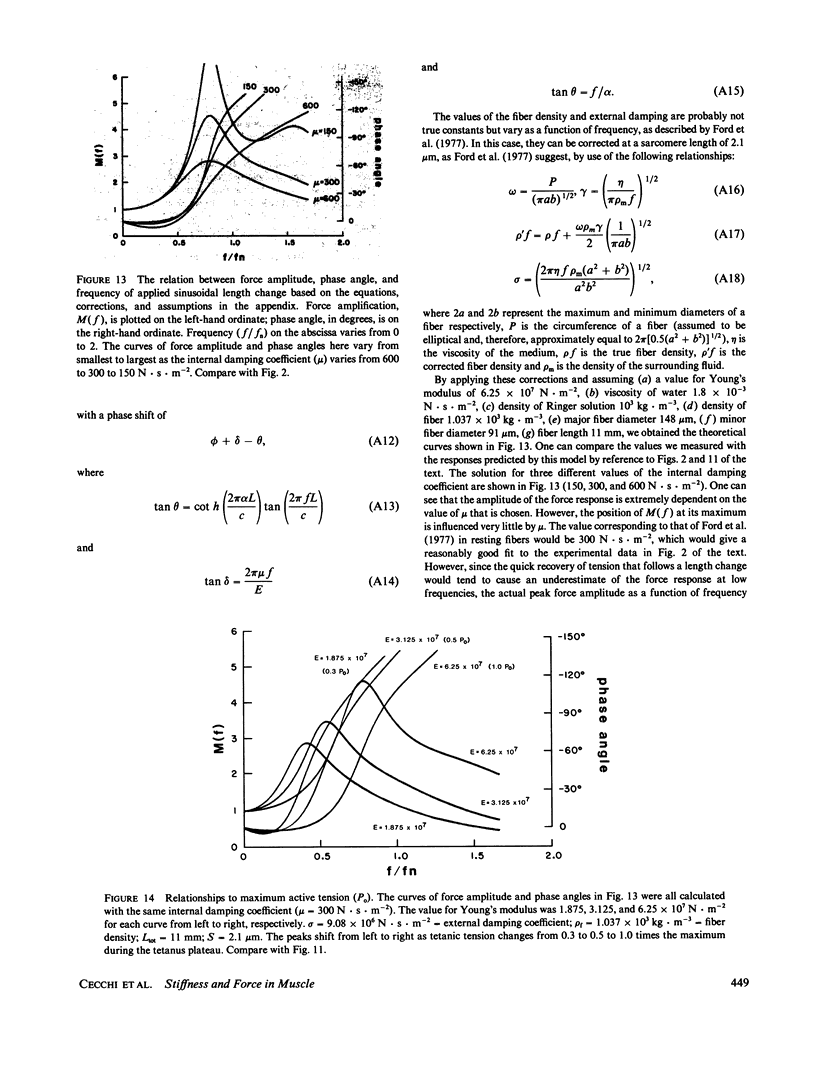
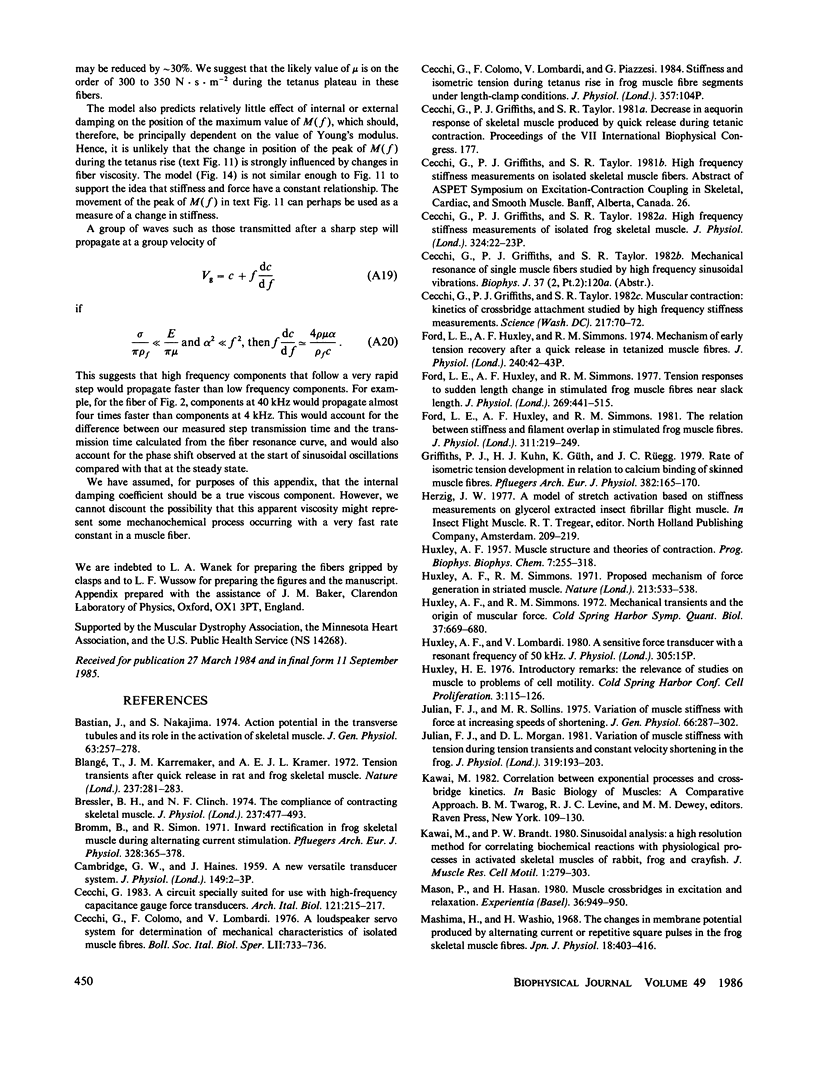
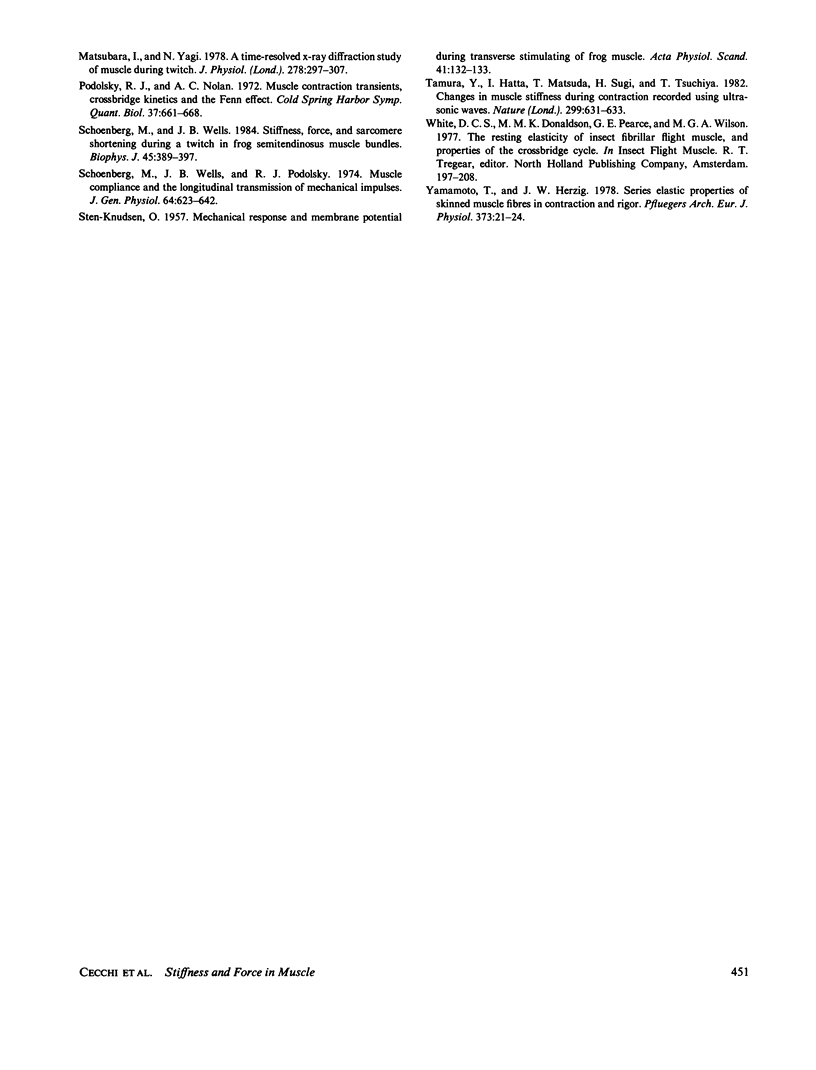
Selected References
These references are in PubMed. This may not be the complete list of references from this article.
- Bastian J., Nakajima S. Action potential in the transverse tubules and its role in the activation of skeletal muscle. J Gen Physiol. 1974 Feb;63(2):257–278. doi: 10.1085/jgp.63.2.257. [DOI] [PMC free article] [PubMed] [Google Scholar]
- Blangé T., Karemaker J. M., Kramer A. E. Tension transients after quick release in rat and frog skeletal muscles. Nature. 1972 Jun 2;237(5353):281–283. doi: 10.1038/237281a0. [DOI] [PubMed] [Google Scholar]
- Bressler B. H., Clinch N. F. The compliance of contracting skeletal muscle. J Physiol. 1974 Mar;237(3):477–493. doi: 10.1113/jphysiol.1974.sp010493. [DOI] [PMC free article] [PubMed] [Google Scholar]
- Cecchi G. A circuit specially suited for use with high-frequency capacitance gauge force transducers. Arch Ital Biol. 1983 Aug;121(3):215–217. [PubMed] [Google Scholar]
- Cecchi G., Colomo F., Lombardi V. A loudspeaker servo system for determination of mechanical characteristics of isolated muscle fibres. Boll Soc Ital Biol Sper. 1976 May 30;52(10):733–736. [PubMed] [Google Scholar]
- Cecchi G., Griffiths P. J., Taylor S. Muscular contraction: kinetics of crossbridge attachment studied by high-frequency stiffness measurements. Science. 1982 Jul 2;217(4554):70–72. doi: 10.1126/science.6979780. [DOI] [PubMed] [Google Scholar]
- Ford L. E., Huxley A. F., Simmons R. M. Tension responses to sudden length change in stimulated frog muscle fibres near slack length. J Physiol. 1977 Jul;269(2):441–515. doi: 10.1113/jphysiol.1977.sp011911. [DOI] [PMC free article] [PubMed] [Google Scholar]
- Ford L. E., Huxley A. F., Simmons R. M. The relation between stiffness and filament overlap in stimulated frog muscle fibres. J Physiol. 1981 Feb;311:219–249. doi: 10.1113/jphysiol.1981.sp013582. [DOI] [PMC free article] [PubMed] [Google Scholar]
- Griffiths P. J., Kuhn H. J., Güth K., Rüegg J. C. Rate of isometric tension development in relation to calcium binding of skinned muscle fibres. Pflugers Arch. 1979 Nov;382(2):165–170. doi: 10.1007/BF00584218. [DOI] [PubMed] [Google Scholar]
- HUXLEY A. F. Muscle structure and theories of contraction. Prog Biophys Biophys Chem. 1957;7:255–318. [PubMed] [Google Scholar]
- Huxley A. F., Simmons R. M. Proposed mechanism of force generation in striated muscle. Nature. 1971 Oct 22;233(5321):533–538. doi: 10.1038/233533a0. [DOI] [PubMed] [Google Scholar]
- Julian F. J., Morgan D. L. Variation of muscle stiffness with tension during tension transients and constant velocity shortening in the frog. J Physiol. 1981;319:193–203. doi: 10.1113/jphysiol.1981.sp013901. [DOI] [PMC free article] [PubMed] [Google Scholar]
- Julian F. J., Sollins M. R. Variation of muscle stiffness with force at increasing speeds of shortening. J Gen Physiol. 1975 Sep;66(3):287–302. doi: 10.1085/jgp.66.3.287. [DOI] [PMC free article] [PubMed] [Google Scholar]
- Kawai M., Brandt P. W. Sinusoidal analysis: a high resolution method for correlating biochemical reactions with physiological processes in activated skeletal muscles of rabbit, frog and crayfish. J Muscle Res Cell Motil. 1980 Sep;1(3):279–303. doi: 10.1007/BF00711932. [DOI] [PubMed] [Google Scholar]
- Mashima H., Washio H. The changes in membrane potential produced by alternating current or repetitive square pulses in the frog skeletal muscle fibres. Jpn J Physiol. 1968 Aug 15;18(4):403–416. doi: 10.2170/jjphysiol.18.403. [DOI] [PubMed] [Google Scholar]
- Mason P., Hasan H. Muscle crossbridge action in excitation and relaxation. Experientia. 1980 Aug 15;36(8):949–950. doi: 10.1007/BF01953810. [DOI] [PubMed] [Google Scholar]
- Matsubara I., Yagi N. A time-resolved X-ray diffraction study of muscle during twitch. J Physiol. 1978 May;278:297–307. doi: 10.1113/jphysiol.1978.sp012305. [DOI] [PMC free article] [PubMed] [Google Scholar]
- Schoenberg M., Wells J. B., Podolsky R. J. Muscle compliance and the longitudinal transmission of mechanical impulses. J Gen Physiol. 1974 Dec;64(6):623–642. doi: 10.1085/jgp.64.6.623. [DOI] [PMC free article] [PubMed] [Google Scholar]
- Schoenberg M., Wells J. B. Stiffness, force, and sarcomere shortening during a twitch in frog semitendinosus muscle bundles. Biophys J. 1984 Feb;45(2):389–397. doi: 10.1016/S0006-3495(84)84163-6. [DOI] [PMC free article] [PubMed] [Google Scholar]
- Tamura Y., Hatta I., Matsuda T., Sugi H., Tsuchiya T. Changes in muscle stiffness during contraction recorded using ultrasonic waves. Nature. 1982 Oct 14;299(5884):631–633. doi: 10.1038/299631a0. [DOI] [PubMed] [Google Scholar]
- Yamamoto T., Herzig J. W. Series elastic properties of skinned muscle fibres in contraction and rigor. Pflugers Arch. 1978 Jan 31;373(1):21–24. doi: 10.1007/BF00581144. [DOI] [PubMed] [Google Scholar]