Abstract
The presence of small amounts of weakly immobilized probes can result in large systematic errors in the measurement of correlation times (tau r) from saturation transfer EPR spectra. However, we have recently developed experimental methodology to minimize these errors (Squier and Thomas, Biophys. J., 49:921-935). In the present study we have applied this methodology to the measurement of the rotational motion of the Ca-ATPase in sarcoplasmic reticulum. This analysis involves the estimate of tau r from line-shape parameters (spectral line-height ratios) and intensity parameters (spectral integral), coupled with digital subtractions to remove spectral components corresponding to weakly immobilized probes. We have analyzed the ST-EPR spectra of the Ca-ATPase over a range of temperatures and find that, unlike line-shape parameters, intensity parameters are little affected by the subtraction of the weakly immobilized spectral component (W). Thus, tau r values from intensity parameters are a more reliable measurement of rotational motion. As reported previously, an analysis with line-shape parameters yields a nonlinear Arrhenius plot of protein mobility. However, the plot is linear when intensity parameters or corrected spectra are used, consistent with the theory for the hydrodynamic properties of a membrane protein of unchanging size and shape in a fluid bilayer. An analysis with line-shape parameters yields different effective tau r values in different spectral regions, and these tau r values are temperature-dependent. However, correction of spectra for W yields temperature-independent tau r ratios, indicating that the motional anisotropy is temperature-independent. Obtaining a good match for the weakly immobilized spectral component remains a major difficulty in the quantitative analysis of ST-EPR spectra using line-shape parameters. This study shows that intensity parameters can be used to avoid this problem, making the ST-EPR technique applicable in cases that were previously resistant to analysis.
Full text
PDF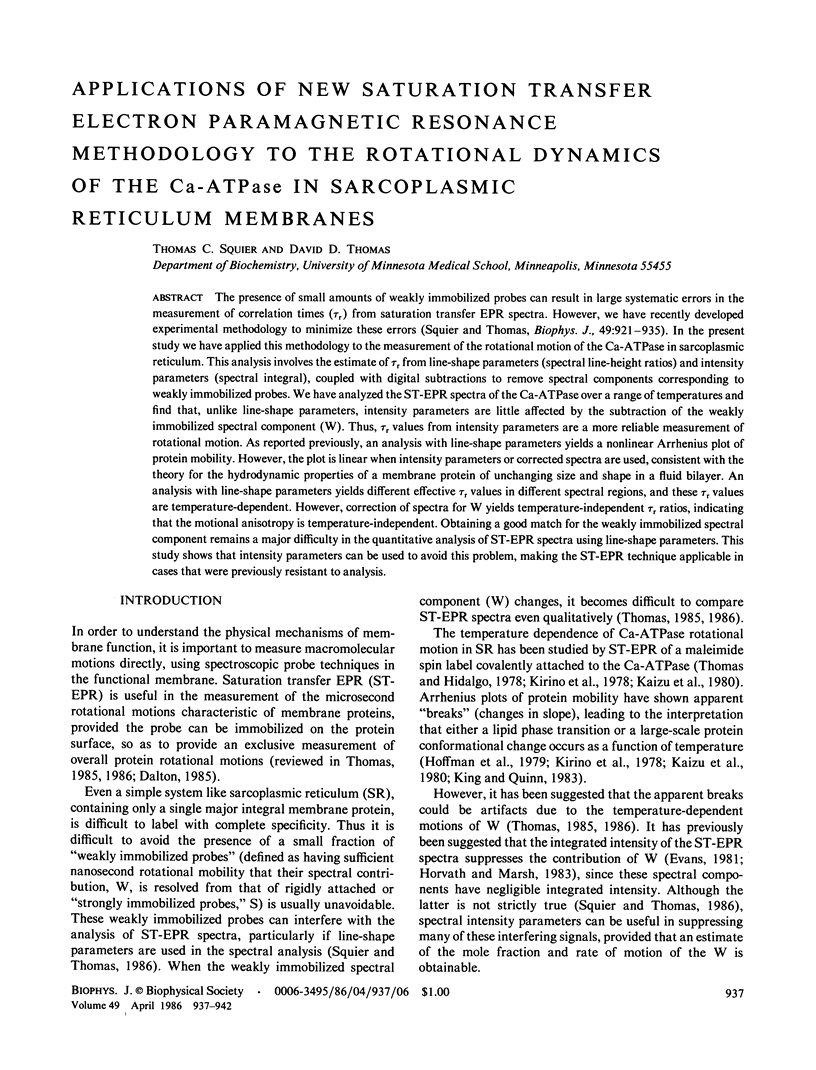
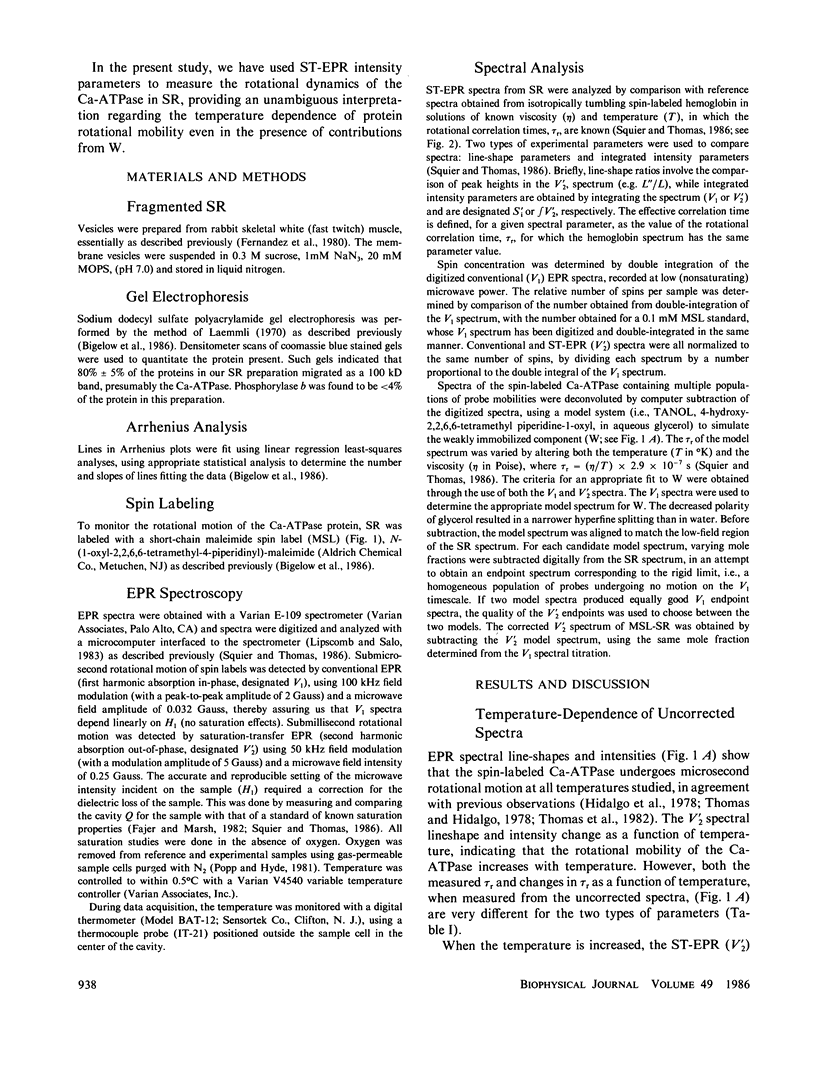
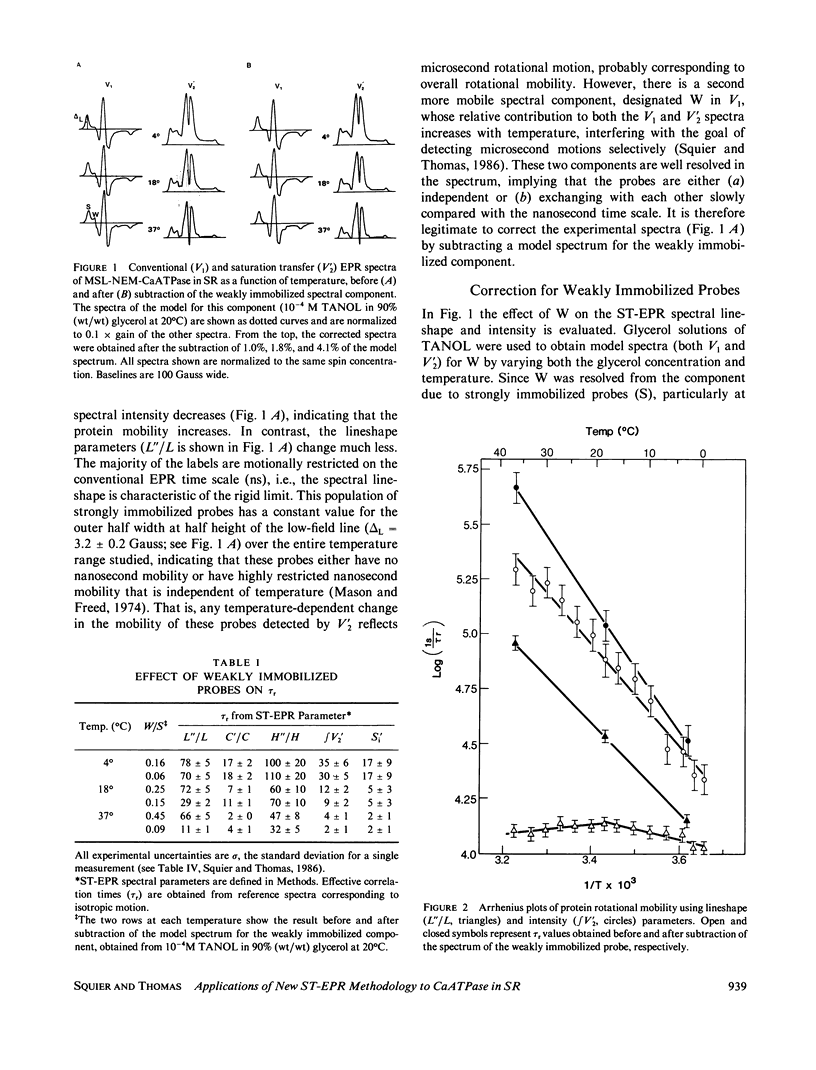
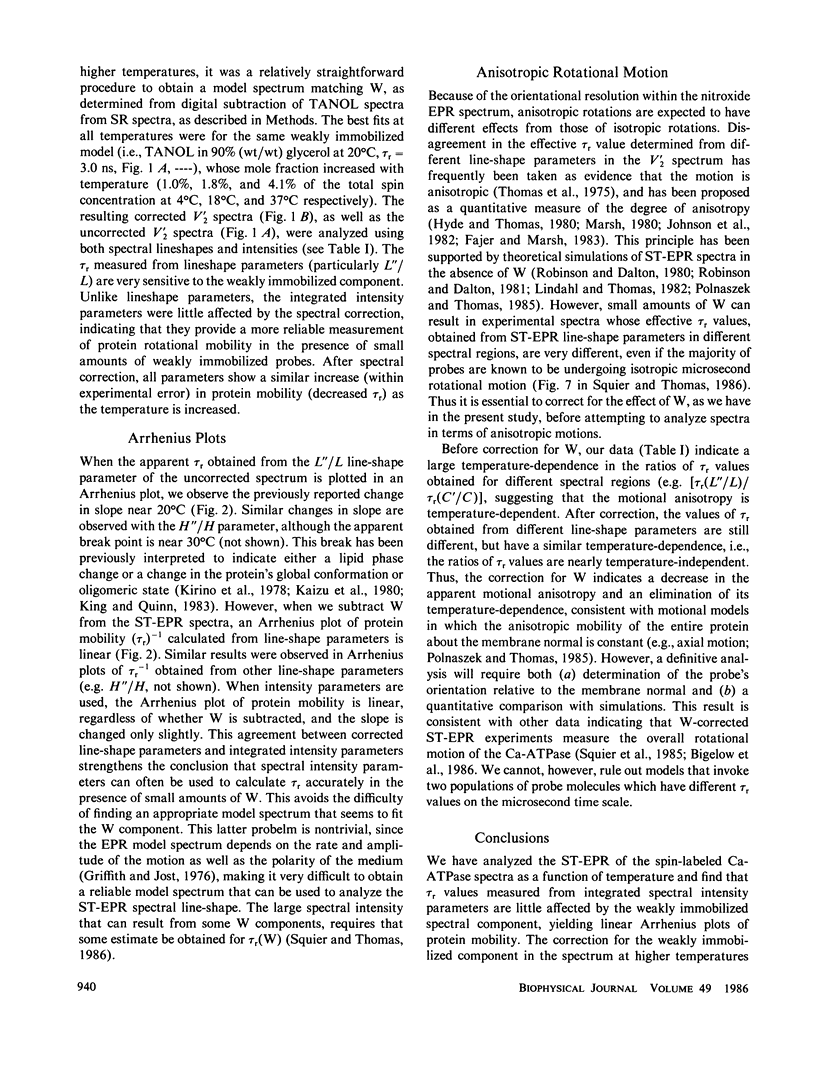
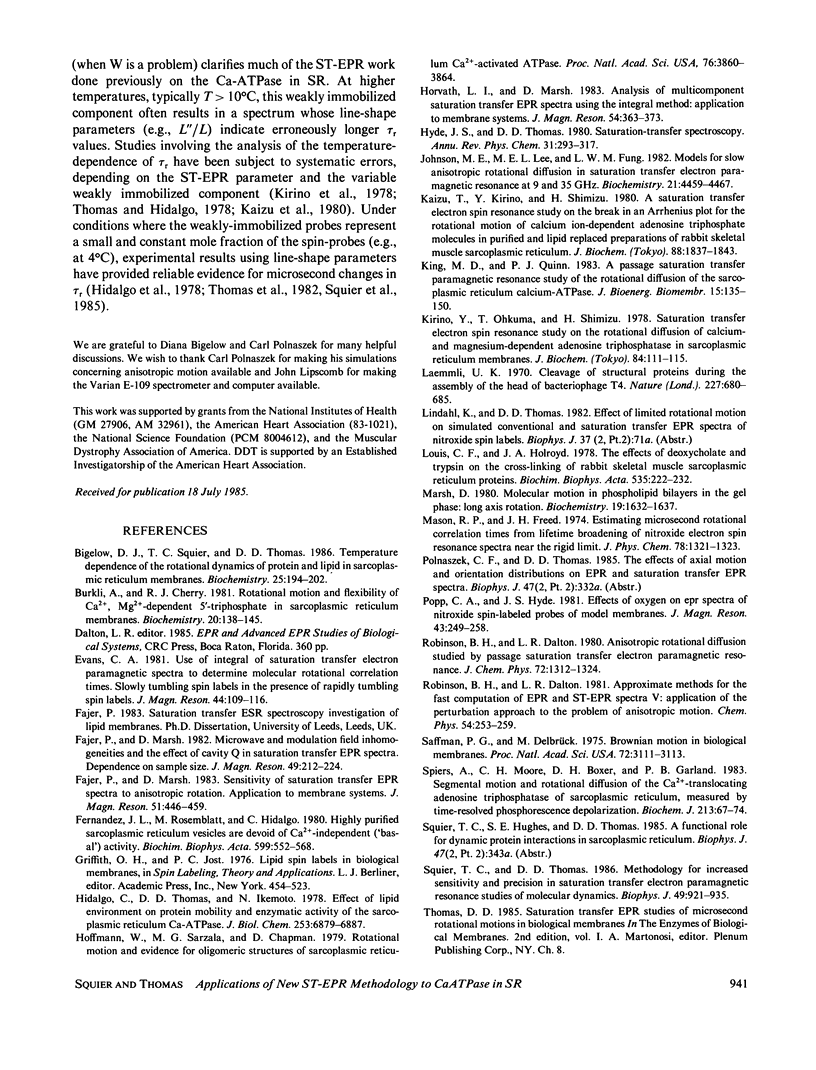
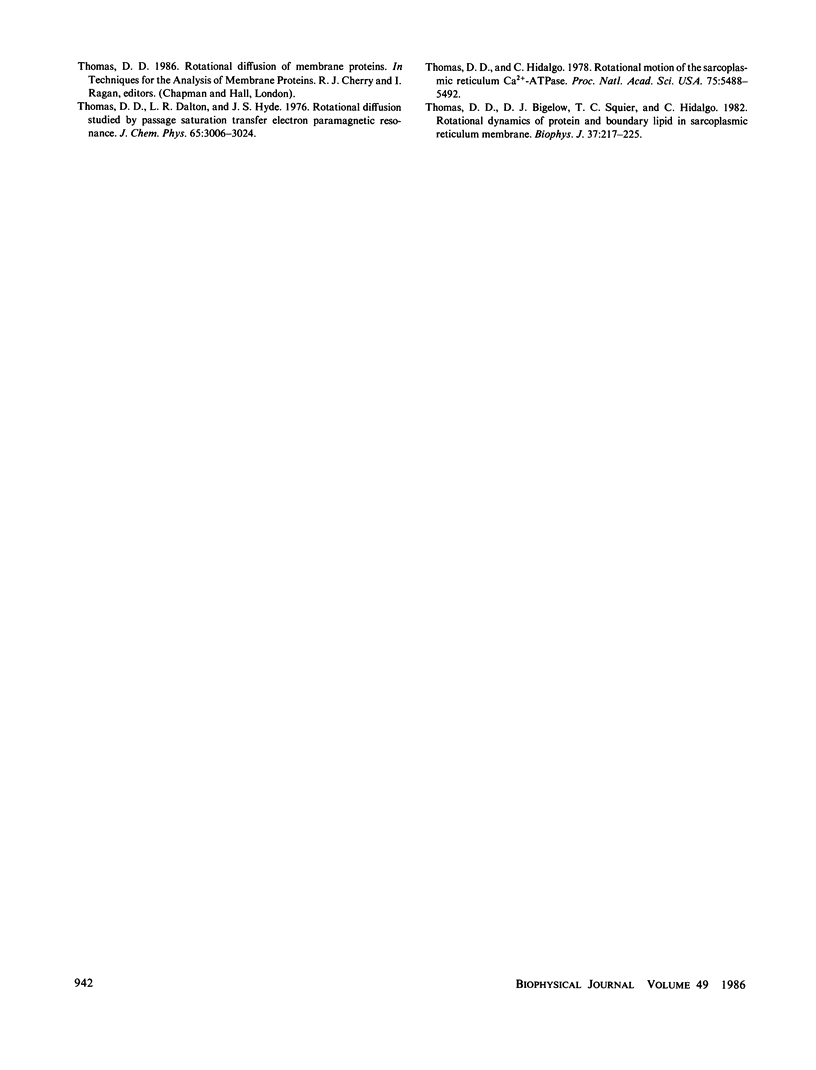
Selected References
These references are in PubMed. This may not be the complete list of references from this article.
- Bigelow D. J., Squier T. C., Thomas D. D. Temperature dependence of rotational dynamics of protein and lipid in sarcoplasmic reticulum membranes. Biochemistry. 1986 Jan 14;25(1):194–202. doi: 10.1021/bi00349a028. [DOI] [PubMed] [Google Scholar]
- Bürkli A., Cherry R. J. Rotational motion and flexibility of Ca2+,Mg2+-dependent adenosine 5'-triphosphatase in sarcoplasmic reticulum membranes. Biochemistry. 1981 Jan 6;20(1):138–145. doi: 10.1021/bi00504a023. [DOI] [PubMed] [Google Scholar]
- Fernandez J. L., Rosemblatt M., Hidalgo C. Highly purified sarcoplasmic reticulum vesicles are devoid of Ca2+-independent ('basal') ATPase activity. Biochim Biophys Acta. 1980 Jul;599(2):552–568. doi: 10.1016/0005-2736(80)90199-6. [DOI] [PubMed] [Google Scholar]
- Hidalgo C., Thomas D. D., Ikemoto N. Effect of the lipid environment on protein motion and enzymatic activity of sarcoplasmic reticulum calcium ATPase. J Biol Chem. 1978 Oct 10;253(19):6879–6887. [PubMed] [Google Scholar]
- Hoffmann W., Sarzala M. G., Chapman D. Rotational motion and evidence for oligomeric structures of sarcoplasmic reticulum Ca2+-activated ATPase. Proc Natl Acad Sci U S A. 1979 Aug;76(8):3860–3864. doi: 10.1073/pnas.76.8.3860. [DOI] [PMC free article] [PubMed] [Google Scholar]
- Johnson M. E., Lee L., Fung L. W. Models for slow anisotropic rotational diffusion in saturation transfer electron paramagnetic resonance at 9 and 35 GHz. Biochemistry. 1982 Aug 31;21(18):4459–4467. doi: 10.1021/bi00261a041. [DOI] [PubMed] [Google Scholar]
- Kaizu T., Kirino Y., Shimizu H. A saturation transfer electron spin resonance study on the break in the Arrhenius plot for the rotational motion of Ca2+-dependent adenosine triphosphatase molecules in purified and lipid-replaced preparations of rabbit skeletal muscle sarcoplasmic reticulum. J Biochem. 1980 Dec;88(6):1837–1843. doi: 10.1093/oxfordjournals.jbchem.a133159. [DOI] [PubMed] [Google Scholar]
- Kirino Y., Ohkuma T., Shimizu H. Saturation transfer electron spin resonance study on the rotational diffusion of calcium- and magnesium-dependent adenosine triphosphatase in sarcoplasmic reticulum membranes. J Biochem. 1978 Jul;84(1):111–115. doi: 10.1093/oxfordjournals.jbchem.a132099. [DOI] [PubMed] [Google Scholar]
- Laemmli U. K. Cleavage of structural proteins during the assembly of the head of bacteriophage T4. Nature. 1970 Aug 15;227(5259):680–685. doi: 10.1038/227680a0. [DOI] [PubMed] [Google Scholar]
- Louis C. F., Holroyd J. A. The effects of deoxycholate and trypsin on the cross-linking of rabbit skeletal muscle sarcoplasmic reticulum proteins. Biochim Biophys Acta. 1978 Aug 21;535(2):222–232. doi: 10.1016/0005-2795(78)90088-0. [DOI] [PubMed] [Google Scholar]
- Marsh D. Molecular motion in phospholipid bilayers in the gel phase: long axis rotation. Biochemistry. 1980 Apr 15;19(8):1632–1637. doi: 10.1021/bi00549a017. [DOI] [PubMed] [Google Scholar]
- Saffman P. G., Delbrück M. Brownian motion in biological membranes. Proc Natl Acad Sci U S A. 1975 Aug;72(8):3111–3113. doi: 10.1073/pnas.72.8.3111. [DOI] [PMC free article] [PubMed] [Google Scholar]
- Speirs A., Moore C. H., Boxer D. H., Garland P. B. Segmental motion and rotational diffusion of the Ca2+-translocating adenosine triphosphatase of sarcoplasmic reticulum, measured by time-resolved phosphorescence depolarization. Biochem J. 1983 Jul 1;213(1):67–74. doi: 10.1042/bj2130067. [DOI] [PMC free article] [PubMed] [Google Scholar]
- Squier T. C., Thomas D. D. Methodology for increased precision in saturation transfer electron paramagnetic resonance studies of rotational dynamics. Biophys J. 1986 Apr;49(4):921–935. doi: 10.1016/S0006-3495(86)83720-1. [DOI] [PMC free article] [PubMed] [Google Scholar]
- Thomas D. D., Bigelow D. J., Squier T. C., Hidalgo C. Rotational dynamics of protein and boundary lipid in sarcoplasmic reticulum membrane. Biophys J. 1982 Jan;37(1):217–225. doi: 10.1016/S0006-3495(82)84671-7. [DOI] [PMC free article] [PubMed] [Google Scholar]
- Thomas D. D., Hidalgo C. Rotational motion of the sarcoplasmic reticulum Ca2+-ATPase. Proc Natl Acad Sci U S A. 1978 Nov;75(11):5488–5492. doi: 10.1073/pnas.75.11.5488. [DOI] [PMC free article] [PubMed] [Google Scholar]